DOI:
10.1039/C4MD00281D
(Concise Article)
Med. Chem. Commun., 2015,
6, 222-229
Received 29th June 2014 , Accepted 7th October 2014
First published on 8th October 2014
1. Introduction
Quinolones are a well known class of synthetic antimicrobial drugs with excellent safety profiles, favorable pharmacokinetic characteristics and a broad antibacterial spectrum. It is generally considered that quinolones can bind with the enzyme–DNA binary complex to form ternary complexes, thereby blocking DNA replication and leading to the death of bacterial cells. Since the first generation of COMPOUND LINKS
Read more about this on ChemSpider
Download mol file of compound
Explore further on Open PHACTSquinolone drugs was found to be active in the early 1960s, four generations of quinolones have been clinically used in succession, such as COMPOUND LINKS
Read more about this on ChemSpider
Download mol file of compound
Explore further on Open PHACTSnalidixic acid, COMPOUND LINKS
Read more about this on ChemSpider
Download mol file of compound
Explore further on Open PHACTSpipemidic acid, COMPOUND LINKS
Read more about this on ChemSpider
Download mol file of compound
Explore further on Open PHACTSlomefloxacin, and COMPOUND LINKS
Read more about this on ChemSpider
Download mol file of compound
Explore further on Open PHACTSmoxifloxacin.1 Nevertheless, the prevalently clinical use of this class of anti-infective drugs has led to increasingly worrisome resistance at a disturbing rate.2 Furthermore, their shortcomings, including phototoxicity, lipid peroxidation, photohemolysis and poor COMPOUND LINKS
Read more about this on ChemSpider
Download mol file of compound
Explore further on Open PHACTSwater solubility, also limit their administrable modes.3 This situation stimulates the urgent need to design and develop powerful antimicrobial COMPOUND LINKS
Read more about this on ChemSpider
Download mol file of compound
Explore further on Open PHACTSquinolone agents,4 especially for the exploration of new COMPOUND LINKS
Read more about this on ChemSpider
Download mol file of compound
Explore further on Open PHACTSquinolone-like compounds. Quinazolinones with a benzopyrimidone skeleton are an important class of compounds structurally similar to clinical benzopyridone quinolone drugs. Reasonably, a great deal of work has recently been directed towards quinazolinone compounds and the related research has become increasingly active.5 Quinazolinones have been found to possess extensive biological activities5 such as antibacterial,6 antifungal,7 antiviral,8 antiinflammatory,9 antimalarial,10 antioxidant,11 antimycobacterial,12 and anticancer.13 Numerous efforts have been devoted to the research and development of quinazolinones as potential medicinal drugs by the separation and purification of naturally occurring alkaloids from a variety of plants as well as artificial synthesis of quinazolinone compounds with novel structures and properties. So far, some quinazolinones, for example, methaqualone as a hypnotic and anticonvulsant agent and COMPOUND LINKS
Read more about this on ChemSpider
Download mol file of compound
Explore further on Open PHACTSquinethazone as a diuretic drug, have been used in the clinic.5a More importantly, an increasing number of quinazolinone compounds have displayed great potency in the treatment of microbial infections.5,14
Azole compounds such as mono-nitrogen thiazoles,15 oxazoles16 and carbazoles,17 bis-nitrogen imidazoles18 and benzimidazoles,19 tri-nitrogen triazoles20 and benzotriazoles,21 and tetra-nitrogen tetrazoles22 are an important class of nitrogen heterocycles with multiple heteroatoms, aromaticity and electron rich properties. Their special structure enables COMPOUND LINKS
Read more about this on ChemSpider
Download mol file of compound
Explore further on Open PHACTSazole-based derivatives to easily bind with the enzymes and receptors in organisms through noncovalent interactions such as coordination and hydrogen bonds, thereby giving them various applications in medicinal chemistry;23 for example, imidazoles4a,18,24 and triazoles4b,20,25 are notably effective against microbial strains. In recent years, research has established that the introduction of nitrogen-containing heterocyclic moieties such as COMPOUND LINKS
Read more about this on ChemSpider
Download mol file of compound
Explore further on Open PHACTSpyrazole and COMPOUND LINKS
Read more about this on ChemSpider
Download mol file of compound
Explore further on Open PHACTSthiazole into the quinazolinone backbone can significantly increase antimicrobial efficiency and broaden their antimicrobial spectrum.5,26 However, to the best of our knowledge, other COMPOUND LINKS
Read more about this on ChemSpider
Download mol file of compound
Explore further on Open PHACTSquinazolinone azoles have been seldom reported. In view of the above observations and as an extension of our studies on the development of azole compounds, herein we incorporated different azole fragments into the N-3 position of COMPOUND LINKS
Read more about this on ChemSpider
Download mol file of compound
Explore further on Open PHACTSquinazolinone to generate a novel class of COMPOUND LINKS
Read more about this on ChemSpider
Download mol file of compound
Explore further on Open PHACTSquinazolinone azoles. Research provides evidence that alkyl linkers can modulate physicochemical properties and thus improve biological potency.27 With the aim of better understanding structure–activity relationships and increasing flexibilities, different lengths of alkyl chains were introduced into the target compounds to investigate the influences of linkers on bioactive profiles. The prepared compounds might be expected to exhibit potential activities against bacterial and fungal strains, including drug-resistant microorganisms. Therefore, the antibacterial and antifungal potencies of all newly synthesized compounds were evaluated in vitro against four Gram-positive bacteria including COMPOUND LINKS
Read more about this on ChemSpider
Download mol file of compound
Explore further on Open PHACTSmethicillin-resistant Staphylococcus aureus N315 (MRSA), four Gram-negative bacteria and five fungi. The preliminary antimicrobial mechanism was investigated by evaluating the interactions of the prepared highly active compounds with calf thymus DNA.28 It is well accepted that the overall distribution, metabolism, and efficacies of drugs can be changed by their affinity to human serum albumin (HSA), and many promising new drugs have been rendered ineffective because of their unusually high affinity to this protein.29 Therefore, the full investigations of interactions between drugs or bioactive small molecules and HSA not only provide a proper understanding of the absorption, transportation, distribution, metabolism and excretion properties of drugs, but also are helpful in the design, modification and screening of drug molecules.30 Thus, further binding behaviors between the synthesized active compound and HSA were investigated to evaluate their transportation and pharmacokinetic properties by fluorescence and UV-vis absorption spectroscopy.
2. Chemistry
The target quinazolinone azoles were synthesized according to the synthetic route outlined in Scheme 1. Commercially available fluoro or chloro substituted COMPOUND LINKS
Read more about this on ChemSpider
Download mol file of compound
Explore further on Open PHACTS2-aminobenzoic acid was reacted with formamidine acetate in the presence of COMPOUND LINKS
Read more about this on ChemSpider
Download mol file of compound
Explore further on Open PHACTS2-methoxyethanol to produce COMPOUND LINKS
Read more about this on ChemSpider
Download mol file of compound
Explore further on Open PHACTS7-fluoroquinazolin-4(3H)-one or 7-chloroquinazolin-4(3H)-one COMPOUND LINKS
Read more about this on ChemSpider
Download mol file of compound1a and b in a high yield of 80%; compounds COMPOUND LINKS
Read more about this on ChemSpider
Download mol file of compound1a and b were then further treated with a series of alkyl dibromides in COMPOUND LINKS
Read more about this on ChemSpider
Download mol file of compound
Explore further on Open PHACTSacetone using potassium carbonate as base to afford bromides 2a–j with yields of 50–76% according to a previously reported method.31 The target quinazolinone azoles 3a–j, 4a–j and 5a–d were conveniently and efficiently obtained in 50–67% yields by the reaction of bromides 2a–j respectively with COMPOUND LINKS
Read more about this on ChemSpider
Download mol file of compound
Explore further on Open PHACTS2-methyl-5-nitroimidazole, COMPOUND LINKS
Read more about this on ChemSpider
Download mol file of compound
Explore further on Open PHACTS4-nitroimidazole, COMPOUND LINKS
Read more about this on ChemSpider
Download mol file of compound
Explore further on Open PHACTSimidazole or COMPOUND LINKS
Read more about this on ChemSpider
Download mol file of compound
Explore further on Open PHACTStriazole in COMPOUND LINKS
Read more about this on ChemSpider
Download mol file of compound
Explore further on Open PHACTSacetonitrile at 50 °C in the presence of potassium carbonate as base. Unfortunately, under the same conditions above, imidazoles 5a and b were obtained in relatively low yields, which demonstrated that potassium carbonate was unfavorable for this reaction; probably its basicity was too weak to form the imidazole carbanion. Therefore, COMPOUND LINKS
Read more about this on ChemSpider
Download mol file of compound
Explore further on Open PHACTSsodium hydride was selected as base in the reaction of COMPOUND LINKS
Read more about this on ChemSpider
Download mol file of compound
Explore further on Open PHACTSimidazole with bromides, which easily afforded the imidazole derivatives 5a and b with good yields ranging from 58% to 69% at 50 °C in anhydrous COMPOUND LINKS
Read more about this on ChemSpider
Download mol file of compound
Explore further on Open PHACTStetrahydrofuran under a stream of nitrogen. All the new compounds were confirmed by 1H NMR, 13C NMR, IR, MS and HRMS spectra (ESI section 1).† 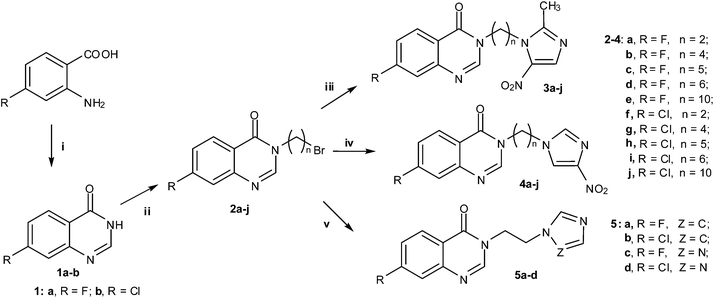 |
| Scheme 1 Synthetic routes of COMPOUND LINKS
Read more about this on ChemSpider
Download mol file of compound
Explore further on Open PHACTSquinazolinone azoles. Reagents and conditions: (i) formamidine acetate, COMPOUND LINKS
Read more about this on ChemSpider
Download mol file of compound
Explore further on Open PHACTS2-methoxyethanol, reflux, 18 h; (ii) alkyl dibromides, COMPOUND LINKS
Read more about this on ChemSpider
Download mol file of compound
Explore further on Open PHACTSK2CO3, COMPOUND LINKS
Read more about this on ChemSpider
Download mol file of compound
Explore further on Open PHACTSacetone, 50 °C, 5 h; (iii) 2-methyl-5-nitroimidazole, COMPOUND LINKS
Read more about this on ChemSpider
Download mol file of compound
Explore further on Open PHACTSK2CO3, COMPOUND LINKS
Read more about this on ChemSpider
Download mol file of compound
Explore further on Open PHACTSacetonitrile, 50 °C, 5 h; (iv) COMPOUND LINKS
Read more about this on ChemSpider
Download mol file of compound
Explore further on Open PHACTS4-nitroimidazole, COMPOUND LINKS
Read more about this on ChemSpider
Download mol file of compound
Explore further on Open PHACTSK2CO3, COMPOUND LINKS
Read more about this on ChemSpider
Download mol file of compound
Explore further on Open PHACTSacetonitrile, 50 °C, 5 h; (v) COMPOUND LINKS
Read more about this on ChemSpider
Download mol file of compound
Explore further on Open PHACTSimidazole or COMPOUND LINKS
Read more about this on ChemSpider
Download mol file of compound
Explore further on Open PHACTStriazole, COMPOUND LINKS
Read more about this on ChemSpider
Download mol file of compound
Explore further on Open PHACTSK2CO3, COMPOUND LINKS
Read more about this on ChemSpider
Download mol file of compound
Explore further on Open PHACTSacetonitrile, 50 °C, 5 h. | |
3. Results and discussion
The obtained results (ESI section 2)† as depicted in Table 1 revealed that COMPOUND LINKS
Read more about this on ChemSpider
Download mol file of compound
Explore further on Open PHACTSquinazolinone nitroimidazoles 3a–j could effectively inhibit the growth of all the tested bacterial strains except for P. aeruginosa, while triazole compounds 5c and d showed potent activity against M. luteus and P. aeruginosa.
Compds | Fungi | Gram-positive bacteria | Gram-negative bacteria |
C. utilis | A. flavus | B. yeast | C. albicans | C. mycoderma | M. luteus | MRSA | S. aureus | B. subtilis | P. aeruginosa | E. coli | B. proteus | E. typhosa |
Minimum inhibitory concentrations were determined by micro broth dilution method for microdilution plates. C. utilis, Candida utilis ATCC9950; A. flavus, Aspergillus flavus ATCC204304; B. yeast, Beer yeast ATCC9763; C. albicans, Candida albicans ATCC10231; C. mycoderma, Candida mycoderma ATCC9888; M. luteus, Micrococcus luteus ATCC4698; MRSA, COMPOUND LINKS
Read more about this on ChemSpider
Download mol file of compound
Explore further on Open PHACTSMethicillin-Resistant Staphylococcus aureus N315; S. aureus, Staphylococcus aureus ATCC25923; B. subtilis, Bacillus subtilis ATCC6633; P. aeruginosa, Pseudomonas aeruginosa ATCC27853; E. Coli, Escherichia coli DH52; B. proteus, Bacillus proteus ATCC13315; E. typhosa, Eberthella typhosa ATCC14028. A = chloromycin; B = COMPOUND LINKS
Read more about this on ChemSpider
Download mol file of compound
Explore further on Open PHACTSnorfloxacin; C = COMPOUND LINKS
Read more about this on ChemSpider
Download mol file of compound
Explore further on Open PHACTSfluconazole. |
2a | 0.94 | 1.89 | 0.94 | 0.94 | >1.89 | 0.47 | 0.94 | >1.89 | 1.89 | >1.89 | 1.89 | 0.47 | >1.89 |
2b | 0.43 | 1.71 | 0.86 | 0.86 | >1.71 | 0.86 | 0.43 | >1.71 | 1.71 | >1.71 | 1.71 | 0.86 | >1.71 |
2c | 0.41 | 0.82 | 0.82 | 0.82 | >1.63 | 0.41 | 0.82 | >1.63 | 1.63 | >1.63 | 1.63 | 0.41 | >1.63 |
2d | 0.39 | 1.56 | 0.78 | 0.78 | >1.56 | 0.39 | 0.39 | >1.56 | 1.56 | >1.56 | 0.78 | 0.39 | >1.56 |
2e | 0.33 | 1.34 | 0.67 | 0.67 | >1.34 | 0.67 | 0.33 | >1.34 | 1.34 | >1.34 | 1.34 | 0.33 | >1.34 |
2f | 0.89 | >1.78 | 0.89 | >1.78 | >1.78 | 0.45 | 0.89 | >1.78 | 1.78 | >1.78 | 1.78 | 0.89 | >1.78 |
2g | 0.41 | >1.62 | 0.81 | >1.62 | >1.62 | 0.81 | 0.81 | >1.62 | 1.62 | >1.62 | 0.81 | 0.41 | >1.62 |
2h | 0.39 | >1.55 | 0.78 | >1.55 | >1.55 | 0.39 | 0.78 | >1.55 | 1.55 | >1.55 | 1.55 | 0.39 | >1.55 |
2i | 0.74 | >1.49 | 0.74 | >1.49 | >1.49 | 0.37 | 0.74 | >1.49 | 1.49 | >1.49 | 1.49 | 0.74 | >1.49 |
2j | 0.32 | >1.28 | 0.64 | >1.28 | >1.28 | 0.32 | 0.64 | >1.28 | 1.28 | >1.28 | 0.64 | 0.32 | >1.28 |
3a | 0.03 | 0.03 | 0.03 | 0.03 | 0.03 | 0.05 | 0.03 | 0.03 | 0.03 | >1.61 | 0.03 | 0.05 | 0.03 |
3b | 0.05 | 0.02 | 0.05 | 0.02 | 0.05 | 0.05 | 0.02 | 0.05 | 0.05 | >1.48 | 0.05 | 0.02 | 0.02 |
3c | 0.02 | 0.02 | 0.02 | 0.04 | 0.04 | 0.02 | 0.02 | 0.04 | 0.02 | >1.42 | 0.04 | 0.02 | 0.02 |
3d | 0.02 | 0.01 | 0.04 | 0.02 | 0.02 | 0.04 | 0.04 | 0.04 | 0.02 | >1.37 | 0.04 | 0.04 | 0.02 |
3e | 0.02 | 0.04 | 0.02 | 0.04 | 0.02 | 0.04 | 0.02 | 0.02 | 0.02 | >1.19 | 0.02 | 0.02 | 0.02 |
3f | 0.05 | 0.02 | 0.05 | 0.05 | 0.05 | 0.10 | 0.05 | 0.10 | 0.05 | >1.53 | 0.10 | 0.05 | 0.02 |
3g | 0.02 | 0.04 | 0.04 | 0.02 | 0.04 | 0.04 | 0.02 | 0.04 | 0.04 | >1.42 | 0.04 | 0.02 | 0.02 |
3h | 0.02 | 0.02 | 0.04 | 0.02 | 0.02 | 0.04 | 0.02 | 0.04 | 0.02 | >1.36 | 0.02 | 0.02 | 0.02 |
3i | 0.02 | 0.04 | 0.02 | 0.02 | 0.02 | 0.04 | 0.02 | 0.02 | 0.04 | >1.31 | 0.04 | 0.02 | 0.02 |
3j | 0.02 | 0.02 | 0.04 | 0.02 | 0.04 | 0.02 | 0.04 | 0.02 | 0.02 | >1.15 | 0.02 | 0.02 | 0.02 |
4a | 0.42 | >1.69 | 0.21 | 0.05 | 0.84 | 0.05 | 0.21 | 0.84 | 0.05 | >1.69 | 0.84 | 0.42 | 0.42 |
4b | 0.39 | 0.77 | 0.10 | 0.10 | 0.77 | 0.05 | 0.19 | 0.39 | 0.05 | 0.77 | 0.77 | 0.39 | 0.39 |
4c | 0.37 | 0.74 | 0.09 | 0.09 | 0.19 | 0.05 | 0.19 | 0.19 | 0.02 | 0.37 | 0.37 | 0.37 | 0.37 |
4d | 0.36 | 0.18 | 0.09 | 0.04 | 0.04 | 0.04 | 0.18 | 0.36 | 0.02 | 0.36 | 0.18 | 0.09 | 0.36 |
4e | 0.15 | 0.15 | 0.08 | 0.04 | 0.04 | 0.04 | 0.15 | 0.31 | 0.02 | 0.08 | 0.15 | 0.08 | 0.31 |
4f | 0.40 | >1.60 | 0.20 | 0.10 | 0.80 | 0.05 | 0.20 | 0.80 | 0.05 | >1.60 | 0.80 | 0.40 | 0.40 |
4g | 0.37 | 0.74 | 0.09 | 0.09 | 0.74 | 0.05 | 0.18 | 0.37 | 0.05 | 0.74 | 0.74 | 0.37 | 0.37 |
4h | 0.35 | 0.71 | 0.09 | 0.09 | 0.18 | 0.04 | 0.18 | 0.18 | 0.04 | 0.35 | 0.35 | 0.35 | 0.35 |
4i | 0.34 | 0.17 | 0.09 | 0.04 | 0.04 | 0.04 | 0.17 | 0.34 | 0.04 | 0.34 | 0.17 | 0.09 | 0.34 |
4j | 0.15 | 0.15 | 0.07 | 0.04 | 0.04 | 0.04 | 0.15 | 0.30 | 0.04 | 0.07 | 0.15 | 0.07 | 0.30 |
5a | 0.99 | 0.50 | 0.50 | 0.99 | >1.98 | 0.50 | 0.99 | >1.98 | >1.98 | 0.99 | 1.98 | 1.98 | >1.98 |
5b | 0.47 | 0.93 | 0.93 | 0.93 | >1.86 | 0.47 | 0.93 | >1.86 | >1.86 | 0.93 | 1.86 | 1.86 | >1.86 |
5c | 0.99 | 0.99 | 0.49 | 0.99 | 0.49 | 0.03 | 0.49 | 0.99 | 0.49 | 0.03 | 0.25 | 0.99 | 0.99 |
5d | 0.46 | 0.93 | 0.46 | 0.93 | 0.46 | 0.03 | 0.46 | 0.93 | 0.46 | 0.03 | 0.23 | 0.93 | 0.93 |
A | >1.58 | >1.58 | >1.58 | >1.58 | >1.58 | 0.02 | 0.05 | 0.05 | 0.10 | 0.10 | 0.10 | 0.10 | 0.10 |
B | >1.60 | >1.60 | >1.60 | >1.60 | >1.60 | 0.01 | 0.03 | 0.01 | 0.01 | 0.01 | 0.05 | 0.01 | 0.01 |
C | 0.03 | 0.84 | 0.05 | <0.01 | 0.01 | >1.67 | >1.67 | >1.67 | >1.67 | >1.67 | >1.67 | >1.67 | >1.67 |
Notably, the hybrid of COMPOUND LINKS
Read more about this on ChemSpider
Download mol file of compound
Explore further on Open PHACTSquinazolinone and 2-methyl-5-nitroimidazole 3a gave comparable or even superior antibacterial efficacies (MIC = 0.03–0.05 μmol mL−1) to COMPOUND LINKS
Read more about this on ChemSpider
Download mol file of compound
Explore further on Open PHACTSnorfloxacin (MIC = 0.01–0.05 μmol mL−1) and chloromycin (MIC = 0.02–0.10 μmol mL−1). 4-Nitroimidazoles 4a–j showed stronger anti-B. subtilis activity (MIC = 0.02–0.05 μmol mL−1) than chloromycin (MIC = 0.10 μmol mL−1). The results also showed that intermediates 2a–j and imidazoles 5a and b displayed weak or no obvious activities in inhibiting the growth of microorganisms. The fluoro or chloro substituent of COMPOUND LINKS
Read more about this on ChemSpider
Download mol file of compound
Explore further on Open PHACTSquinazolinone at the C-7 position did not exert any significantly different influence on antimicrobial potency.
It is known that MRSA is one of the most virulent organisms that shows severe multi-drug resistance to numerous currently available agents. Excitingly, 2-methyl-5-nitroimidazoles 3a–j effectively inhibited the growth of MRSA at concentrations of 0.02–0.05 μmol mL−1, and were comparable or even more active in comparison with clinical chloromycin (MIC = 0.05 μmol mL−1). The results in Table 1 also demonstrated the great effects of alkyl chain lengths on biological activities. Long-chain alkyl 4-nitroimidazoles 4c–e and 4h–j possessed better antimicrobial activities than short-chain derivatives 4a and b and 4f and g against most of the tested strains, respectively. Particularly for B. subtilis, compounds 4c–e with (CH2)5, (CH2)6 and (CH2)10 linkers respectively were five-fold more potent (MIC = 0.02 μmol mL−1) than chloromycin (MIC = 0.10 μmol mL−1). This might be explained by the high hydrophobicity of the long alkyl chain, which facilitated penetration of the cytomembrane and therefore improved the antibacterial activity.
The antifungal evaluation in vitro revealed that most of nitroimidazole compounds showed antifungal efficiency to some extent, similar to the antibacterial results. Noticeably, 2-methyl-5-nitroimidazoles 3a–j showed good inhibitory efficiency against all the tested fungal strains, which further implied that the nitroimidazole nucleus has favorable antimicrobial potency. Especially, compound 3d with the (CH2)6 linker efficiently inhibited the growth of A. flavus strain in vitro with an MIC value of 0.01 μmol mL−1, which was 84-fold more potent than COMPOUND LINKS
Read more about this on ChemSpider
Download mol file of compound
Explore further on Open PHACTSfluconazole (MIC = 0.84 μmol mL−1). The results suggested that this compound may be further investigated as an anti-A. flavus agent.
3.2 Interactions with calf thymus DNA
Deoxyribonucleic acid (DNA) is the informational molecule encoding the genetic instructions used in the development and function of almost all known living organisms. It is one of the targets for the studies of biologically important small molecules such as antimicrobial drugs. The binding studies of small molecules with DNA are important and helpful for developing novel and more efficient drugs, which have been attracting considerable attention in biomedical science. To explore the possible antimicrobial action mechanism, the binding behavior of active compound 3a with calf thymus DNA (a DNA model with medical importance, low cost and ready availability properties) was studied on the molecular level in vitro using COMPOUND LINKS
Read more about this on ChemSpider
Download mol file of compound
Explore further on Open PHACTSneutral red (NR) dye as a spectral probe by UV-vis spectroscopic methods.32 3.2.1 Absorption spectra of DNA in the presence of compound 3a. Absorption spectroscopy is a very useful technique in DNA-binding studies. In absorption spectroscopy, hypochromism and hyperchromism are very important spectral features to distinguish changes in DNA double-helical structure.33 Due to the strong interactions between the electronic states of the intercalating chromophore and that of the DNA base, the observed large hyperchromism strongly suggests a close proximity of the aromatic chromophore to the DNA bases. With a fixed concentration of DNA, UV-vis absorption spectra were recorded with increasing amounts of compound 3a. As shown in Fig. 1, UV-vis spectra displayed that the maximum absorption peak of DNA at 260 nm exhibited a proportional increase with the increasing concentration of compound 3a. Meanwhile, the absorption value of 3a–DNA complex was slightly greater than the measured value of the simple sum of free DNA and free compound 3a. This indicates that a weak hyperchromism effect existed between DNA and compound 3a. Furthermore, the intercalation of the aromatic chromophore of compound 3a into the helix and the strong overlap of π–π* states in the large π-conjugated system with the electronic states of DNA bases were consistent with the observed spectral changes. 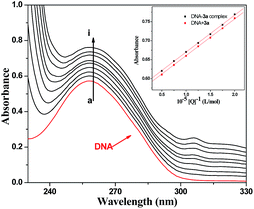 |
| Fig. 1 UV absorption spectra of DNA with different concentrations of compound 3a (pH = 7.4, T = 290 K). Inset: comparison of absorption at 260 nm between the 3a–DNA complex and the sum values of free DNA and free compound 3a. c(DNA) = 8.62 × 10−5 mol L−1, and c(compound 3a) = 0–2.0 × 10−5 mol L−1 for curves a–i respectively at increments of 0.25 × 10−5. | |
On the basis of the variations in the absorption spectra of DNA upon binding to 3a, eqn (1) can be utilized to calculate the intrinsic binding constant (K):
| 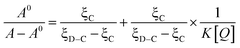 | (1) |
where
A0 and
A represent the absorbance of
DNA in the absence and presence of compound
3a at 260 nm, and
ξC and
ξD–C are the absorption coefficients of compound
3a and
3a–DNA complex respectively. The plot of
A0/(
A −
A0)
versus 1/[compound
3a] was constructed using the absorption titration data and linear fitting (
Fig. 2), yielding the binding constant,
K = 1.41 × 10
4 L mol
−1,
R = 0.998, SD = 0.34 (R is the correlation coefficient, and SD is the standard deviation).
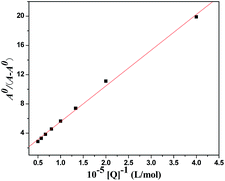 |
| Fig. 2 The plot of A0/(A − A0) versus 1/[compound 3a]. | |
3.2.2 Absorption spectra of NR interactions with DNA. COMPOUND LINKS
Read more about this on ChemSpider
Download mol file of compound
Explore further on Open PHACTSNeutral Red (NR) is a planar phenazine dye that is structurally similar to other planar dyes such as acridines, thiazines and xanthenes, but possesses lower toxicity, higher stability and convenient application. Furthermore, it has been sufficiently demonstrated by spectrophotometric and electrochemical techniques that the binding of NR with DNA is an intercalation binding.28b Therefore, NR was employed as a spectral probe to investigate the binding mode of 3a with DNA in the present work. The absorption spectra of the NR dye upon the addition of DNA are shown in Fig. 3. It was apparent that the absorption peak of NR at around 460 nm displayed a gradual decrease with the increasing concentration of DNA, and a new band developed at around 530 nm. This was attributed to the formation of the new DNA–NR complex. An isosbestic point at about 504 nm provided evidence of DNA–NR complex formation. 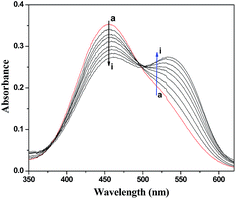 |
| Fig. 3 UV absorption spectra of NR in the presence of DNA at pH 7.4 and room temperature. c(NR) = 2.0 × 10−5 mol L−1, and c(DNA) = 0–5.25 × 10−5 mol L−1 for curves a–i respectively at increments of 0.66 × 10−5. | |
3.2.3 Absorption spectra of competitive interactions of compound 3a and NR with DNA. Fig. 4 displays the absorption spectra of a competitive binding assay between NR and 3a with DNA. As shown, with increasing concentration of 3a, the maximum absorption around 460 nm of the DNA–NR complex increased. Compared with the absorption band at around 460 nm of the free NR in the presence of increasing concentrations of DNA (Fig. 3), the spectra in Fig. 4 (inset) exhibited the reverse process. The results suggested that compound 3a intercalated into the double helix of DNA by substituting for NR in the DNA–NR complex. 3.3 Interactions of compound 3a with HSA
HSA is the most abundant serum protein in systemic circulation, comprising approximately 60% of plasma and providing approximately 80% of the colloid osmotic pressure of blood. Full investigations of interactions of drugs with HSA are not only beneficial to thoroughly understand the pharmacokinetic behavior of drugs, but also to aid in the design, modification and screening of drug molecules.30 3.3.1 UV-vis absorption spectral study. UV-vis absorption measurement is a very facile operational method applicable to explore the structural changes of protein and to identify complex formation. In our binding experiment, UV-vis absorption spectroscopy was adopted to evaluate the binding behaviors between compound 3a and HSA. As shown in Fig. 5, the absorption peak observed at 278 nm was attributed to the aromatic rings in tryptophan (Trp-214), tyrosine (Tyr-411) and phenylalanine (Phe) residues in HSA. With the addition of compound 3a, the peak intensity increased, indicating that compound 3a could interact with HSA and that the peptide strands of HSA were extended. However, the maximum absorption wavelength remained unchanged, implying that the interactions of compound 3a and HSA were noncovalent interactions. These occurred via π–π stacking between the aromatic rings of compound 3a and Trp, Tyr and Phe residues, which possess conjugated π-electrons and are located in the binding cavity of HSA.34 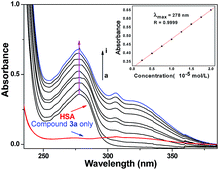 |
| Fig. 5 Effect of compound 3a on HSA UV-vis absorption, c(HSA) = 1.0 × 10−5 mol L−1; c(compound 3a)/(10−5 mol L−1): 0, 0.25, 0.5, 0.75, 1, 1.25, 1.5, 1.75, 2 (T = 298 K, pH = 7.40). The inset corresponds to the absorbance at 278 nm with different concentrations of compound 3a. | |
3.3.2 Fluorescence quenching mechanism. Fluorescence spectroscopy is also an effective method to study the interactions of small molecules with HSA. The fluorescence intensity of Trp-214 may change when HSA interacts with other small molecules, which could be reflected in the fluorescence spectra of HSA in the UV region. The effect of compound 3a on the fluorescence intensity of HSA at 298 K is shown in Fig. S6 (ESI section 3).† It was obvious that HSA had a strong fluorescence emission with a peak at 358 nm owing to the single Try-214 residue. The intensity of this characteristic broad emission band regularly decreased with increased concentration of compound 3a; however, the maximum emission wavelength of HSA remained unchanged. This suggested that Trp-214 did not undergo any change in polarity.29b The fluorescence quenching data can be analyzed by the well-known Stern–Volmer equation:35
| 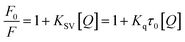 | (2) |
where
F0 and
F represent fluorescence intensity in the absence and presence of compound
3a, respectively.
KSV (L mol
−1) is the Stern–Volmer quenching constant,
Kq is the bimolecular quenching rate constant (L mol
−1 s
−1),
τ0 is the fluorescence lifetime of the fluorophore in the absence of quencher, assumed to be 6.4 × 10
−9 s for HSA, and [
Q] is the concentration of compound
3a. Hence, the Stern–Volmer plots of HSA in the presence of compound
3a at different concentrations and temperatures could be calculated and were shown in
Fig. 6.
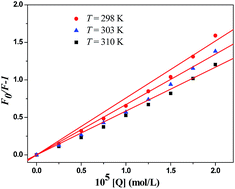 |
| Fig. 6 Stern–Volmer plots of 3a–HSA system at different temperatures. | |
Fluorescence quenching occurs by different mechanisms, usually classified as dynamic quenching and static quenching depending on temperature and viscosity. Because higher temperatures result in larger diffusion coefficients, the quenching constants are expected to increase with a gradually increasing temperature in dynamic quenching. However, the increase of temperature is likely to result in a smaller static quenching constant due to the dissociation of weakly bound complexes.
The values of KSV and Kq for the interactions of compound 3a with HSA at different temperatures are shown in Table S2 (ESI section 3).† The KSV values were inversely correlated with the temperature, which indicated that the fluorescence quenching of HSA was probably initiated by the formation of 3a–HSA complex rather than dynamic collisions. The Kq values obtained at different temperatures were in the range of 1012–1013 L mol−1 s−1, which far exceeded the diffusion controlled rate constants of various quenchers with a biopolymer (2.0 × 1010 L mol−1 s−1), and indicated that the quenching was not initiated by the dynamic diffusion process but occurred in the statical formation of 3a–HSA complex.
3.3.3 Binding constant and site. For a static quenching process, the data can be described by the modified Stern–Volmer equation:36 | 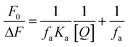 | (3) |
where ΔF is the difference in fluorescence intensity in the absence and presence of compound 3a at concentration [Q], fa is the fraction of accessible fluorescence, and Ka is the effective quenching constant for the accessible fluorophores, which are analogous to associative binding constants for the quencher-acceptor system. The dependence of F0/ΔF on the reciprocal value of quencher concentration [Q]−1 is linear with the slope equal to the value of (faKa)−1. The value fa−1 is fixed on the ordinate. The constant Ka is a quotient of the ordinate fa−1 and the slope (faKa)−1. The modified Stern–Volmer plots are shown in Fig. S8† and the calculated results are depicted in Table S3 (ESI section 3).† When small molecules bind to a set of equivalent sites on a macromolecule, the equilibrium binding constants and the numbers of binding sites can also be calculated according to the Scatchard equation:37
where
Df is the molar concentration of free small molecules,
r is the moles of small molecules bound per mole of
protein,
n is
binding site multiplicity per class of
binding site, and
Kb is the equilibrium binding constant. The Scatchard plots are shown in Fig. S9
† and the
Kb and
n are listed in Table S3 (ESI section 3).
† Fig. S9† shows the modified Stern–Volmer and Scatchard plots for the 3a–HSA system at different temperatures. The decreased trend of Ka and Kb with increased temperature was in accordance with the temperature dependence of KSV. The value of the binding site n was approximately 1, which showed that one high affinity binding site was present in the interactions of compound 3a with HSA. The results also showed that the binding constants were moderate and the effects of temperature were not significant; thus, compound 3a might be stored and carried by this protein.
3.3.4 Binding mode and thermodynamic parameters. Generally, there are four types of non-covalent interactions, including hydrogen bonds, van der Waals forces, electrostatic interactions and hydrophobic bonds, which play important roles in the binding of small molecules to proteins.38 Changes in the thermodynamic parameters enthalpy (ΔH) and entropy (ΔS) of the binding reaction are the main evidence for confirming the interactions between small molecules and proteins. If ΔH does not vary significantly over the studied temperature range, then its value and ΔS can be evaluated from the van't Hoff equation: | 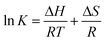 | (5) |
where K is analogous to the associative binding constants at the corresponding temperature and R is the gas constant. In order to explain the binding model between compound 3a and HSA, the thermodynamic parameters were calculated from the van't Hoff plots. ΔH was estimated from the slope of the van't Hoff relationship (Fig. S10, ESI section 3).† The free energy change (ΔG) was then calculated from the following equation:Table S4 (ESI section 3)† summarizes the values of ΔH, ΔG and ΔS. The negative values of free energy ΔG of the interactions between compound 3a and HSA suggested that the binding process was spontaneous. The negative entropy ΔS and enthalpy ΔH values of the interactions of compound 3a with HSA indicated that the binding was mainly enthalpy-driven and the entropy was unfavorable; moreover, hydrogen bonds and van der Waals forces played a major role in the reaction. 4. Conclusion
In conclusion, a novel class of COMPOUND LINKS
Read more about this on ChemSpider
Download mol file of compound
Explore further on Open PHACTSquinazolinone azoles were successfully synthesized in good yields via an easy, convenient and efficient synthetic route. All the newly synthesized compounds were characterized by 1H NMR, 13C NMR, MS, IR and HRMS spectra. The biological results revealed that some target compounds exhibited significant antibacterial and antifungal activities against most of the tested strains. Notably, nitroimidazole derivative 3a showed comparable or even superior antibacterial efficacies (MIC = 0.03–0.05 μmol mL−1) in contrast with COMPOUND LINKS
Read more about this on ChemSpider
Download mol file of compound
Explore further on Open PHACTSnorfloxacin (MIC = 0.01–0.05 μmol mL−1) and chloromycin (MIC = 0.02–0.10 μmol mL−1). The specific interactions of compound 3a with DNA were studied by UV-vis absorption spectroscopy. Experimental results displayed that compound 3a could intercalate DNA to form compound 3a–DNA complex which might further block DNA replication to exert powerful antibacterial and antifungal activities. The calculated parameters indicated that the binding process was spontaneous, and hydrogen bonds and van der Waals forces played important roles in the strong association of compound 3a–HSA. Further research, including the in vivo bioactive evaluation along with toxicity investigation and the effect factors on antimicrobial activities such as other heterocyclic rings23a,39 (benzotriazole, COMPOUND LINKS
Read more about this on ChemSpider
Download mol file of compound
Explore further on Open PHACTSbenzimidazole and their derivatives, etc.) as well as spacers with different types of linkers (aralkyl, aryl and heterocyclic types and their lengths of chains) are actively in progress. All these will be discussed in a future paper. Acknowledgements
This work was partially supported by National Natural Science Foundation of China (no. 21172181, 21372186), the Research Fellowship for International Young Scientists from International (Regional) Cooperation and Exchange Program (no. 81350110523, 81450110095), the key program from Natural Science Foundation of Chongqing (CSTC2012jjB10026) and the Specialized Research Fund for the Doctoral Program of Higher Education of China (SRFDP 20110182110007) and Chongqing Special Fund for Postdoctoral Research Proposal (Xm2014127). Notes and references
-
(a) C. Mugnaini, S. Pasquini and F. Corelli, Curr. Med. Chem., 2009, 16, 1746–1767 CrossRef CAS PubMed;
(b) A. Ahmed and M. Daneshtalab, J. Pharm. Pharm. Sci., 2012, 15, 52–72 CAS.
- K. J. Aldred, R. J. Kerns and N. Osheroff, Biochemistry, 2014, 53, 1565–1574 CrossRef CAS PubMed.
- J. A. Wiles, B. J. Bradbury and M. J. Pucci, Expert Opin. Ther. Pat., 2010, 20, 1295–1319 CrossRef CAS PubMed.
-
(a) S. F. Cui, L. P. Peng, H. Z. Zhang, S. Rasheed, K. V. Kumar and C. H. Zhou, Eur. J. Med. Chem., 2014, 86, 318–334 CrossRef CAS PubMed;
(b) F. W. Zhou, H. S. Lei, L. Fan, L. Jiang, J. Liu, X. M. Peng, X. R. Xu, L. Chen, C. H. Zhou, Y. Y. Zou, C. P. Liu, Z. Q. He and D. C. Yang, Bioorg. Med. Chem. Lett., 2014, 24, 1912–1917 CrossRef CAS PubMed;
(c) S. F. Cui, Y. Ren, S. L. Zhang, X. M. Peng, G. L. V. Damu, R. X. Geng and C. H. Zhou, Bioorg. Med. Chem. Lett., 2013, 23, 3267–3272 CrossRef CAS PubMed;
(d) Y. Wang, G. L. V. Damu, J. S. Lv, R. X. Geng, D. C. Yang and C. H. Zhou, Bioorg. Med. Chem. Lett., 2012, 22, 5363–5366 CrossRef CAS PubMed.
-
(a) P. C. Sharma, G. Kaur, R. Pahwa, A. Sharma and H. Rajak, Curr. Med. Chem., 2011, 18, 4786–4812 CrossRef CAS PubMed;
(b) I. Khan, A. Ibrar, N. Abbas and A. Saeed, Eur. J. Med. Chem., 2014, 76, 193–244 CrossRef CAS PubMed;
(c) R. Rajput and A. P. Mishra, Int. J. Pharm. Pharm. Sci., 2012, 4, 66–70 CAS.
- X. Wang, J. Yin, L. Shi, G. P. Zhang and B. A. Song, Eur. J. Med. Chem., 2014, 77, 65–74 CrossRef CAS PubMed.
- X. Wang, Z. N. Li, J. Yin, M. He, W. Xue, Z. W. Chen and B. A. Song, J. Agric. Food Chem., 2013, 61, 9575–9582 CAS.
-
(a) Z. W. Wang, M. X. Wang, X. Yao, Y. Li, J. Tan, L. Z. Wang, W. T. Qiao, Y. Q. Geng, Y. X. Liu and Q. M. Wang, Eur. J. Med. Chem., 2012, 53, 275–282 CrossRef CAS PubMed;
(b) A. L. Leivers, M. Tallant, J. B. Shotwell, S. Dickerson, M. R. Leivers, O. B. McDonald, J. Gobel, K. L. Creech, S. L. Strum, A. Mathis, S. Rogers, C. B. Moore and J. Botyanszki, J. Med. Chem., 2014, 57, 2091–2106 CrossRef CAS PubMed.
- M. F. Zayed and M. H. Hassan, Saudi Pharm. J., 2014, 22, 157–162 CrossRef PubMed.
- Y. Takeuchi, M. Koike, K. Azuma, H. Nishioka, H. Abe, S. H. Kim, Y. Wataya and T. Harayama, Chem. Pharm. Bull., 2001, 49, 721–725 CrossRef CAS PubMed.
- A. A. Al-Amiery, A. A. H. Kadhum, M. Shamel, M. Satar, Y. Khalid and A. B. Mohamad, Med. Chem. Res., 2014, 23, 236–242 CrossRef CAS.
- M. B. Patel, U. Harikrishnan, N. N. Valand, N. R. Modi and S. K. Menon, Arch. Pharm., 2013, 346, 210–220 CrossRef CAS PubMed.
- C. Carmi, E. Galvani, F. Vacondio, S. Rivara, A. Lodola, S. Russo, S. Aiello, F. Bordi, G. Costantino, A. Cavazzoni, R. R. Alfieri, A. Ardizzoni, P. G. Petronini and M. Mor, J. Med. Chem., 2012, 55, 2251–2264 CrossRef CAS PubMed.
-
(a) F. A. M. Al-Omary, G. S. Hassan, S. M. El-Messery, M. N. Nagid, E. E. Habib and H. I. El-Subbagh, Eur. J. Med. Chem., 2013, 63, 33–45 CrossRef CAS PubMed;
(b) L. P. Shi, K. M. Jiang, J. J. Jiang, Y. Jin, Y. H. Tao, K. Li, X. H. Wang and J. Lin, Bioorg. Med. Chem. Lett., 2013, 23, 5958–5963 CrossRef CAS PubMed;
(c) N. C. Desai, H. V. Vaghani and P. N. Shihora, J. Fluorine Chem., 2013, 153, 39–47 CrossRef CAS.
-
(a) S. F. Cui, Y. Wang, J. S. Lv, G. L. V. Damu and C. H. Zhou, Sci. Sin.: Chim., 2012, 42, 1105–1131 CrossRef CAS;
(b) S. F. Cui, C. H. Zhou, R. X. Geng and Q. G. Ji, Chin. J. Biochem. Pharm., 2012, 33, 311–315 CAS.
- H. Z. Zhang, C. H. Zhou, R. X. Geng and Q. G. Ji, Chin. J. Org. Chem., 2011, 31, 1963–1976 CAS.
-
(a) F. F. Zhang, C. H. Zhou and J. P. Yan, Chin. J. Org. Chem., 2010, 30, 783–796 CAS;
(b) F. F. Zhang, L. L. Gan and C. H. Zhou, Bioorg. Med. Chem. Lett., 2010, 20, 1881–1884 CrossRef CAS PubMed.
-
(a) L. Zhang, X. M. Peng, G. L. V. Damu, R. X. Geng and C. H. Zhou, Med. Res. Rev., 2014, 34, 340–437 CrossRef CAS PubMed;
(b) L. Zhang, J. J. Chang, S. L. Zhang, G. L. V. Damu, R. X. Geng and C. H. Zhou, Bioorg. Med. Chem., 2013, 21, 4158–4169 CrossRef CAS PubMed.
-
(a) H. Z. Zhang, S. F. Cui, S. Nagarajan, S. Rasheed, G. X. Cai and C. H. Zhou, Tetrahedron Lett., 2014, 55, 4105–4109 CrossRef CAS;
(b) H. Z. Zhang, J. M. Lin, S. Rasheed and C. H. Zhou, Sci. China: Chem., 2014, 57, 807–822 CrossRef CAS;
(c) S. L. Zhang, G. L. V. Damu, L. Zhang, R. X. Geng and C. H. Zhou, Eur. J. Med. Chem., 2012, 55, 164–175 CrossRef CAS PubMed.
-
(a) C. H. Zhou and Y. Wang, Curr. Med. Chem., 2012, 19, 239–280 CrossRef CAS PubMed;
(b) H. Z. Zhang, G. L. V. Damu, G. X. Cai and C. H. Zhou, Curr. Org. Chem., 2014, 18, 359–406 CrossRef CAS;
(c) Y. Y. Zhang, J. L. Mi, C. H. Zhou and X. D. Zhou, Eur. J. Med. Chem., 2011, 46, 4391–4402 CrossRef CAS PubMed;
(d) Q. P. Wang, J. Q. Zhang, G. L. V. Damu, K. Wan, H. Z. Zhang and C. H. Zhou, Sci. China: Chem., 2012, 55, 2134–2153 CrossRef CAS.
-
(a) Y. Shi, C. H. Zhou, X. D. Zhou, R. X. Geng and Q. G. Ji, Acta Pharm. Sin. B, 2011, 46, 798–810 CAS;
(b) Y. Shi and C. H. Zhou, Bioorg. Med. Chem. Lett., 2011, 21, 956–960 CrossRef CAS PubMed.
-
(a) L. L. Dai, S. F. Cui, G. L. V. Damu and C. H. Zhou, Chin. J. Org. Chem., 2013, 33, 224–244 CrossRef CAS;
(b) L. L. Dai, H. Z. Zhang, S. Nagarajan, S. Rasheed and C. H. Zhou, MedChemComm, 2015 10.1039/c4md00266k.
-
(a) X. M. Peng, G. X. Cai and C. H. Zhou, Curr. Top. Med. Chem., 2013, 13, 1963–2010 CrossRef CAS PubMed;
(b) S. L. Zhang, J. J. Chang, G. L. V. Damu, R. X. Geng and C. H. Zhou, MedChemComm, 2013, 4, 839–846 RSC;
(c) Y. Y. Zhang and C. H. Zhou, Bioorg. Med. Chem. Lett., 2011, 21, 4349–4352 CrossRef CAS PubMed;
(d) S. Li, J. X. Chen, Q. X. Xiang, L. Q. Zhang, C. H. Zhou, J. Q. Xie, L. Yu and F. Z. Li, Eur. J. Med. Chem., 2014, 84, 677–686 CrossRef CAS PubMed;
(e) C. H. Zhou, Y. Y. Zhang, C. Y. Yan, K. Wan, L. L. Gan and Y. Shi, Anti-Cancer Agents Med. Chem., 2010, 10, 371–395 CrossRef CAS;
(f) C. H. Zhou, L. L. Gan, Y. Y. Zhang, F. F. Zhang, G. Z. Wang, L. Jin and R. X. Geng, Sci. China, Ser. B: Chem., 2009, 52, 415–458 CrossRef CAS.
-
(a) G. L. V. Damu, S. F. Cui, X. M. Peng, Q. M. Wen, G. X. Cai and C. H. Zhou, Bioorg. Med. Chem. Lett., 2014, 24, 3605–3608 CrossRef CAS PubMed;
(b) B. Fang, C. H. Zhou and X. C. Rao, Eur. J. Med. Chem., 2010, 45, 4388–4398 CrossRef CAS PubMed;
(c) G. L. V. Damu, Q. P. Wang, H. Z. Zhang, Y. Y. Zhang, J. S. Lv and C. H. Zhou, Sci. China: Chem., 2013, 56, 952–969 CrossRef CAS.
-
(a) Y. Wang and C. H. Zhou, Sci. Sin.: Chim., 2011, 41, 1429–1456 CrossRef;
(b) J. J. Wei, Y. Wang, X. L. Wang, C. H. Zhou and Q. G. Ji, Chin. Pharm. J., 2011, 46, 481–485 CAS;
(c) S. L. Zhang, J. J. Chang, G. L. V. Damu, B. Fang, X. D. Zhou, R. X. Geng and C. H. Zhou, Bioorg. Med. Chem. Lett., 2013, 23, 1008–1012 CrossRef CAS PubMed;
(d) J. S. Lv, X. M. Peng, B. Kishore and C. H. Zhou, Bioorg. Med. Chem. Lett., 2014, 24, 308–313 CrossRef CAS PubMed;
(e) H. Z. Zhang, J. J. Wei, K. V. Kumar, S. Rasheed and C. H. Zhou, Med. Chem. Res., 2014 DOI:10.1007/s00044–014–1123–9.
- K. A. M. El-Bayouki, M. M. Aly, Y. A. Mohamed, W. M. Basyouni and Y. S. Abbas, World J. Chem., 2009, 4, 161–170 CAS.
-
(a) X. L. Wang, K. Wan and C. H. Zhou, Eur. J. Med. Chem., 2010, 45, 4631–4639 CrossRef CAS PubMed;
(b) Y. Luo, Y. H. Lu, L. L. Gan, C. H. Zhou, J. Wu, R. X. Geng and Y. Y. Zhang, Arch. Pharm., 2009, 342, 386–393 CrossRef CAS PubMed.
-
(a) G. W. Zhang, P. Fu, L. Wang and M. M. Hu, J. Agric. Food Chem., 2011, 59, 8944–8952 CrossRef CAS PubMed;
(b) X. L. Li, Y. J. Hu, H. Wang, B. Q. Yu and H. L. Yue, Biomacromolecules, 2012, 13, 873–880 CrossRef CAS PubMed.
-
(a) F. Berti, S. Bincoletto, I. Donati, G. Fontanive, M. Fregonese and F. Benedetti, Org. Biomol. Chem., 2011, 9, 1987–1999 RSC;
(b) Y. J. Hu, Y. Liu and X. H. Xiao, Biomacromolecules, 2009, 10, 517–521 CrossRef CAS PubMed.
- D. C. Carter and J. X. Ho, Adv. Protein Chem., 1994, 45, 153–203 CrossRef CAS PubMed.
-
(a) J. W. Cusic and E. F. Levon, N-[2-(nitro-1-imidazolyl)ethyl]naphthal imides, US Pat. 3997572, 1976;
(b) K. Lee, J. Kim, K. Jeong, K. W. Lee, Y. Lee, J. Y. Song, M. S. Kim, G. S. Lee and Y. Kim, Bioorg. Med. Chem., 2007, 17, 3152–3161 CrossRef PubMed.
- A. J. Berdis, Biochemistry, 2008, 47, 8253–8260 CrossRef CAS PubMed.
- C. D. Kanakis, S. Nafisi, M. Rajabi, A. Shadaloi, P. A. Tarantilis, M. G. Polissiou, J. Bariyanga and H. A. Tajmir-Riahi, Spectroscopy, 2009, 23, 29–43 CrossRef CAS.
- V. D. Suryawanshi, P. V. Anbhule, A. H. Gore, S. R. Patil and G. B. Kolekar, Ind. Eng. Chem. Res., 2012, 51, 95–102 CrossRef CAS.
- J. R. Lakowicz, Principles of fluorescence spectroscopy, Springer, New York, 3rd edn, 2006, pp. 11–12 Search PubMed.
- B. T. Yin, C. Y. Yan, X. M. Peng, S. L. Zhang, S. Rasheed, R. X. Geng and C. H. Zhou, Eur. J. Med. Chem., 2014, 71, 148–159 CrossRef CAS PubMed.
- J. Y. Yang and W. Y. Yang, J. Am. Chem. Soc., 2009, 131, 11644–11645 CrossRef CAS PubMed.
- U. S. Mote, S. R. Patil, S. H. Bhosale, S. H. Han and G. B. Kolekar, J. Photochem. Photobiol., B, 2011, 103, 16–21 CrossRef CAS PubMed.
-
(a) X. M. Peng, G. L. V. Damu and C. H. Zhou, Curr. Pharm. Des., 2013, 19, 3884–3930 CrossRef CAS PubMed;
(b) H. Z. Zhang, G. L. V. Damu, G. X. Cai and C. H. Zhou, Eur. J. Med. Chem., 2013, 64, 329–344 CrossRef CAS PubMed.
Footnotes |
† Electronic supplementary information (ESI) available. See DOI: 10.1039/c4md00281d |
‡ Postdoctoral fellow from School of Chemistry, Madurai Kamaraj University, India. |
§ Postdoctoral fellow from Department of Chemistry, Hyderabad University, India. |
|
This journal is © The Royal Society of Chemistry 2015 |
Click here to see how this site uses Cookies. View our privacy policy here.