DOI:
10.1039/C5LC00080G
(Technical Innovation)
Lab Chip, 2015,
15, 1994-1997
Electromechanical cell lysis using a portable audio device: enabling challenging sample preparation at the point-of-care†
Received
22nd January 2015
, Accepted 13th March 2015
First published on 13th March 2015
Abstract
Audio sources are ubiquitously available on portable electronic devices, including cell phones. Here we demonstrate lysis of Mycobacterium marinum and Staphylococcus epidermidis bacteria utilizing a portable audio device coupled with a simple and inexpensive electromagnetic coil. The resulting alternating magnetic field rotates a magnet in a tube with the sample and glass beads, lysing the cells and enabling sample preparation for these bacteria anywhere there is a cell phone, mp3 player, laptop, or other device with a headphone jack.
Diagnosis is the first hurdle in disease management, expediting appropriate treatment in developed settings where sophisticated equipment and trained personnel are available. For example, in the US in vitro diagnostic procedures represent about 1.6% of Medicare spending, yet influence 60–70% of medical decisions.1 Nucleic acid amplification tests (NAATs) performed in the laboratory represent the pinnacle of sensitive and specific pathogen detection. Unfortunately, this state of the art is also expensive and complex, requiring infrastructure and instrumentation not available in all settings.
The lack of adequate diagnostics is especially troublesome in the case of tuberculosis (TB), which infects 1/3 of the world's population according to the WHO.2 Sixty percent of TB patients only have access to the peripheral level of the health system, where no suitable TB diagnostics exist.3 Conventional TB diagnostics in lower-resource settings, mainly sputum smear microscopy and cell culture, lack the ideal specificity and timeliness. Also, required equipment isn't always available.3 Microfluidic platforms have shown promise to enable the type of point-of-care devices that could bring NAATs directly to patients in lower resource settings, but sample preparation remains the weak link in microfluidics-based bioassays.4
Existing microfluidic systems such as the Cepheid GeneXpert, consisting of sophisticated instrumentation into which a disposable cartridge containing the assay reagents is inserted, are capable of sample-to-result nucleic acid testing, and can obtain results within two hours. While the GeneXpert has been shown to expedite TB treatment,5 due to cost and infrastructure requirements it remains primarily a solution for centralized laboratories. Therefore, further advances are needed in point-of-care sample preparation that is compatible with low resource settings and downstream NAATs.
Cell phones have provided a new tool used to interpret and communicate health data. In addition to telemedicine applications, cell phones have shown utility for applications ranging from the interpretation of diagnostic tests6,7 to digitizing information originally recorded on paper forms.8 If cell phones could also assist in sample preparation, it may become more feasible to perform NAATs completely free of laboratory equipment.
If a low-cost point-of-care NAAT were available, not only could disease diagnostics be performed more rapidly, but also water and food safety could be evaluated quickly and inexpensively. The development of isothermal NAATs, such as loop-mediated amplification (LAMP) and recombinase polymerase amplification, is a large step towards enabling simpler devices. These isothermal assays do not require precision thermal cycling, can be more robust against inhibitors than polymerase chain reaction (PCR),9 and are compatible with paper-based microfluidics.10 Low-cost precision isothermal heaters have been demonstrated and could enable point-of-care application of this type of assay.11–13 Unfortunately, many low-cost point-of-care NAAT devices still rely on off-chip, instrumented sample preparation steps.4 Mechanical lysis methods, such as bead beating, are desirable in that one can avoid the need to purify the sample from a chemical lytic agent before the downstream bioassay, but these methods traditionally suffer from relatively complex, user- and power-intensive instruments and protocols.14
Here we demonstrate lysis of two bacteria—Staphylococcus epidermidis and a more difficult target Mycobacterium marinum—using a new form of highly portable, low-power, mechanical cell lysis. Like other mechanical approaches, it does not introduce reagents incompatible with nucleic acid amplification. We believe this new apparatus could enable point-of-care NAATs for a wide range of organisms. At least one other group has demonstrated a low-cost mechanical lysis method amenable to point-of-care diagnostics.15 What sets our device apart is that it is free from a device-specific power source, since adequate power can be provided by the readily-available audio signal provided by the portable device; one could integrate sample preparation with the end detection strategy, using smartphones. With the recent increase in the use of diagnostic interpretation aided by cell phones,16 which are fairly ubiquitous worldwide,7 this type of device may help close the loop in sensitive and specific pathogen detection in low resource settings. Here we show that the headphone jack of a low-cost mp3 player provides adequate power for this lysis methodology. The resulting DNA accessible for amplification after audio-powered lysis is compared to bead beating as a gold standard.
Material and methods
Bacterial culture
Staphylococcus epidermidis (strain Fussel ATCC 14990) was cultured in Tryptic Soy Broth (BD Bacto, Sparks, MD, USA) at 37 °C, shaking (250 rpm). Overnight cultures were diluted 1
:
100 in fresh medium and grown to mid-log phase (OD600 = ~2). Mycobacterium marinum (Aronson ATCC 927) was cultured in Middlebrook 7H9 broth with ADC enrichment (BD Difco, Sparks, MD, USA) at 30 °C, shaking (250 rpm) for 4–7 days. Cultures were diluted 1
:
100 in fresh medium and grown for 48 hours to mid-log phase (OD600 = ~1). After growth, cells were pelleted and resuspended in 1 volume Tris-EDTA (TE: 10 mM Tris-HCl, 1 mM EDTA, pH 8.0: Sigma-Aldrich, St Louis, MO, USA) buffer. Cell dilutions were also done in TE.
Audio-powered lysis
Coil bobbins were constructed from a hacksaw-excised well from a 24-well plate adhered to laser-cut acrylic upper and lower disks. The bobbin was wound with 490 turns of 32 AWG magnet wire, for a DC resistance of 11.4 ohms. The wound coil was soldered to a 1/8" stereo cable combining the left and right channels. The assembly was then wrapped with two turns of electrical tape around the circumference. Screw-top 2 mL O-ring tubes (02-682-558, Thermo Fisher Scientific, Waltham, MA, USA) were loaded with 100 μm glass beads (9830, Research Products International Corp., Mt. Prospect, IL, USA) and a 1/4 inch NdFeB magnet (S4, K&J Magnetics, Inc., Pipersville, PA, USA). Sample was then added to the tubes, and the tubes were loaded into the coils. To match the bead beater protocol 800 μL samples were tested, along with 500 and 300 μL sample sizes. A 30 Hz sine wave FLAC audio file was generated using Audacity 2.0.5,‡ and was played on the mp3 player (Sansa Clip+ 4 GB, SanDisk, Milpitas, CA, USA) at full volume with the equalizer set to maximize bass frequency response (Fig. 1). The video, included in the ESI,† was captured at 24 frames per second using an MU330 microscope camera (AMscope, Irvine, CA, USA) with a macro lens (Macro-Switar 75 mm F/1.9, Kern, Switzerland).
Bead beater
800 μL samples were added to 2 mL O-ring screw top tubes (Fisher 02-682-558) with 800 mg beads. Tubes were loaded into the bead beater (Mini-Beadbeater-8, Biospec Products, Inc., Bartlesville, OK, USA), set to “homogenize”, and run for (3) 1 minute cycles with a 1 minute pause between cycles.
qPCR
To quantify DNA recovery for S. epidermidis, qPCR was performed using primers designed against the htrA gene (FWD: 5′-GAG CGC ATA AGA CGT GAG AA-3′, REV: 5′-TCT TCT TGT GTC AGC TTC TCT ATT-3′), and using Bioline SensiFAST SYBR No-ROX qPCR kit (Bioline USA Inc., Taunton, MA, USA). Samples (1 μL) from the bead beater or audio-powered lysis were used in 20 μL qPCR reactions run on a real-time PCR instrument (Rotor-Gene Q, Qiagen, Valencia, CA, USA) using: 95 °C (3 min), 40 cycles of 95 °C (5 s), 54 °C (10 s), and 72 °C (15 s). Fluorescence data were collected during the 72 °C step using the green channel. To quantify DNA recovery for M. marinum, qPCR was performed using the assay described by Jacobs et al., substituting the SensiFAST Probe No-ROX qPCR kit (Bioline USA Inc., Taunton, MA, USA) for the master mix used previously.17 Samples were amplified as follows on a real-time PCR instrument (CFX96 Touch, Bio-Rad, Hercules, CA, USA): 95 °C (5 min), followed by 40 cycles of 90 °C (10 s) and 60 °C (20 s). Fluorescence data were collected during the 60 °C step using the green channel. Genomic DNA copy numbers were determined relative to standard curve analysis using purified DNA of known copy number using either the Rotor-Gene or Bio-Rad CFX software. Both assays are sensitive down to ~10 copies of the target sequence.
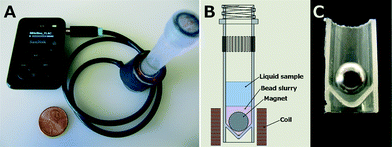 |
| Fig. 1 Overview of portable audio-powered electromagnetic cell lysis device. A: Sansa Clip mp3 player provides a 30 Hz sine wave signal to the coil using the headphone jack, US penny for scale. B: Schematic of tube showing user-added sample along with pre-loaded magnet and beads. C: Sectioned tube with magnet. The spherical magnet rides on the lower angled tube walls when the tube is vertically oriented. When provided an alternating magnetic field by the coil, the magnet rotates against the beads and the tube walls. | |
Statistics
A student t-test was performed on bead mass data (Fig. 2A) and M. marinum data (Fig. 3) in Excel (Microsoft, Redmond, WA, USA) for a single-tailed comparison assuming the data are homoscedastic. The 200 & 400 mg bead masses were grouped and compared separately to the 0 & 100 mg and 800 mg groups for both the 300 and 800 μL sample sizes.
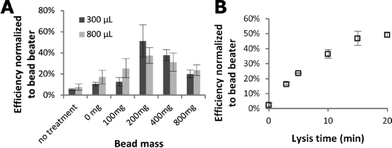 |
| Fig. 2 A: Dependence of portable audio-powered Staphylococcus epidermidis lysis efficiency on bead mass for 300 and 800 μL sample sizes. Amplifiable DNA quantified by qPCR, normalized to the bead beater computed copy number (7.1 × 104 copies per mL, 71 copies per PCR). Sample sizes of both 300 and 800 μL perform best with 200–400 mg beads. Data is mean ± 1 standard deviation, n = 3. B: Dependence of audio-powered lysis efficiency for S. epidermidus on total lysis time. Amplifiable DNA quantified by qPCR, normalized to the bead beater computed copy number (3.6 × 104 copies per mL, 36 copies per PCR) using a 800 μL sample size and 300 mg beads in the audio-powered device. Efficiency (as compared to bead-beater efficiency) plateaus near 50% at just over 10 minutes for the audio-powered device under these conditions. Data points are mean ± 1 standard deviation, n = 3. | |
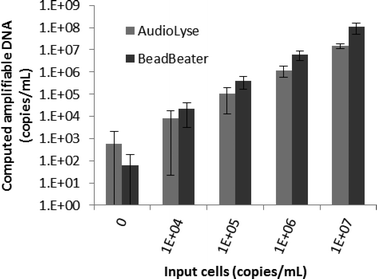 |
| Fig. 3 Amplifiable DNA quantified by PCR for varying input concentration of M. marinum cells. 500 μL sample size. 104 copies per mL corresponds to 10 copies per PCR quantification. Data points represent mean ± 1 standard deviation. Minimum n = 9 total spread across 3 different days. | |
Results
Fig. 2 shows the dependence of lysis of S. epidermidis cells by the portable audio-powered device on bead mass, sample size, and processing time. The majority of amplifiable DNA is released in less than 10 minutes. A mass of 200–400 mg of glass beads achieves higher efficiency than the other bead volume groups (p < 0.05) for both 300 and 800 μL samples. A thirty Hz excitation frequency was found to produce reliable magnet rotation (starting from rest) even in samples as viscous as 50% glycerol (6 centipoise at 20 °C, which was the highest viscosity tested).
Mycobacterium marinum, a more difficult target to lyse, was chosen for study due to its similarity to the major pathogen Mycobacterium tuberculosis. The performance of the audio-powered technique is compared to that of a bench-top bead beater for a range of M. marinum concentrations in Fig. 3. The audio-powered technique achieves appreciable lysis of M. marinum for all concentrations tested, with 104 cells per mL corresponding to an estimated maximum of 10 cells being transferred in the 1 μL sample used in the PCR. All non-zero input copy concentrations were significantly higher than the 0 copy experiment (p < 0.02), including the 104 copy per mL input experiments. Thus, we have demonstrated that the portable audio-powered technique can be used as a sample preparation tool for DNA amplification for both S. epidermidis and M. marinum.
Conclusions
The portable audio-powered device achieves 20–60% of the efficiency of the bench-top bead beater for various concentrations of M. marinum and S. epidermidis, while costing far less, consuming just a small fraction of the electrical power, emitting far less audible noise, and being highly portable. This decreased lysis efficiency, compared to the bead beater, is balanced by the expansion in applicable settings. Whether this level of performance is sufficient depends on infection levels, sampling technique, and the associated assay sensitivity. Cell phones have expanded communication and medical capabilities in remote settings.18 Now, in addition to using photo, voice, and text communication to transmit and interpret assay results, audio signals from a headphone jack can be used to retrieve amplifiable DNA from some of the hardest-to-lyse bacteria in virtually any setting. The power requirements of this method are quite low. The mp3 player output of 1.6 V peak-to-peak when powering a coil with a 12 ohm impedance (11.4 ohms DC, 0.6 ohms inductive at 30 Hz) results in a power draw of 107 mW; ten minutes of lysis with this coil would account for just 0.18% of the capacity of a 2700 mW h cell phone battery.
We expect further optimization of the sample volume and complexity, coil excitation frequency and duration, bead quantity and diameter, and other factors to lead to higher performance. Both S. epidermidis and M. marinum are difficult bacteria to lyse; performance with easier to lyse bacteria such as E. coli will likely be higher and testing is currently under way. Portable sample preparation could allow next generation point-of-care NAATs for bacteria such as Mycobacterium marinum and Staphylococcus epidermidis free from bench-top laboratory equipment.
What sets this device apart from other lysis techniques compatible with the point-of-care is freedom from additional power supplies (battery packs, etc.) and the potential of using the same peripheral equipment (cell phones) for sample preparation along with interpreting test results. Academic medical laboratories can implement the methodology shown here for very little cost. As shown here, less than $38 was spent for the mp3 player, magnet wire, cable, and bobbin materials per device, and less than $1 per assay was spent for the screw-top tube, magnet, and beads. Further reductions in cost would be realized if quantities were scaled up or the device were optimized to reduce magnet size, bead volume, tube cost, etc. While clinical laboratories may find the noise of bench top bead beaters acceptable, a quieter technique such as demonstrated here may be more amenable to the point-of-care. Also, the inexpensive nature of the hardware makes it nearly disposable.
We are currently broadening the sample types tested using this technique, along with other lysis methods, for a wide range of bacteria and clinical sample types including blood, sputum, and nasal matrix. It is our expectation that this work will contribute to the next generation of portable nucleic acid amplification tests, including the multiplexable, autonomous, disposable nucleic acid amplification test (MAD NAAT) project,11,19,20 a collaborative effort including the Yager group at the University of Washington, PATH, the ELITech Group, and GE Global Research.
Acknowledgements
Funding was provided by DARPA DSO HR0011-11-2-0007 and the UW Department of Bioengineering. Thank you to everyone in the Yager lab and collaborators at PATH, GE Global Research, and the ELITech Group for the support and feedback.
Notes and references
-
The value of diagnostics innovation, adoption and diffusion into health care, Advanced Medical Technology Association, 2005, http://www.lewin.com/publications/Publication/237/, (Accessed February 12, 2013) Search PubMed.
-
World Health Organization, Global Tuberculosis Report, 2013 Search PubMed.
-
UNITAID, Tuberculosis: Diagnostic Technology Landscape, World Health Organization, 2012 Search PubMed.
- R. Mariella, Biomed. Microdevices, 2008, 10(6), 777–784 CrossRef PubMed.
- C. C. Boehme, M. P. Nicol, P. Nabeta, J. S. Michael, E. Gotuzzo, R. Tahirli, M. T. Gler, R. Blakemore, W. Worodria, C. Gray, L. Huang, T. Caceres, R. Mehdiyev, L. Raymond, A. Whitelaw, K. Sagadevan, H. Alexander, H. Albert, F. Cobelens, H. Cox, D. Alland and M. D. Perkins, Lancet, 2011, 377, 1495–1505 CrossRef.
- A. A. Weaver, H. Reiser, T. Barstis, M. Benvenuti, D. Ghosh, M. Hunckler, B. Joy, L. Koenig, K. Raddell and M. Lieberman, Anal. Chem., 2013, 85(13), 6453–6460 CrossRef CAS PubMed.
- A. W. Martinez, S. T. Phillips, E. Carrilho, S. W. Thomas, H. Sindi and G. M. Whitesides, Anal. Chem., 2008, 80(10), 3699–3707 CrossRef CAS PubMed.
-
N. Dell, N. Breit, T. Chaluco, J. Crawford, and G. Borriello, Proc. 2nd ACM Symp. Comput. Dev. - ACM DEV '12, 2012 Search PubMed.
- H. Kaneko, T. Kawana, E. Fukushima and T. Suzutani, J. Biochem. Biophys. Methods, 2007, 70(3), 499–501 CrossRef CAS PubMed.
- B. A. Rohrman and R. Richards-Kortum, Lab Chip, 2012, 12(17), 3082–3088 RSC.
- J. Singleton, C. Zentner, J. Buser, P. Yager, P. LaBarre and B. H. Weigl, Proc. SPIE, 2013, 8615, 86150R CrossRef PubMed.
- P. LaBarre, K. R. Hawkins, J. Gerlach, J. Wilmoth, A. Beddoe, J. Singleton, D. Boyle and B. Weigl, PLoS One, 2011, 6(5), e19738 CAS.
- C. Liu, M. G. Mauk, R. Hart, X. Qiu and H. H. Bau, Lab Chip, 2011, 11(16), 2686–2692 RSC.
-
BioSpec Products - Mini-beadbeater-8, Available at: http://www.biospec.com/instructions/minibeadbeater_8/, Accessed January 10, 2015 Search PubMed.
- P. E. Vandeventer, K. M. Weigel, J. Salazar, B. Erwin, B. Irvine, R. Doebler, A. Nadim, G. A. Cangelosi and A. Niemz, J. Clin. Microbiol., 2011, 49(7), 2533–2539 CrossRef PubMed.
- V. Gubala, L. F. Harris, A. J. Ricco, M. X. Tan and D. E. Williams, Anal. Chem., 2012, 84(2), 487–515 CrossRef CAS PubMed.
- J. Jacobs, M. Rhodes, B. Sturgis and B. Wood, Appl. Environ. Microbiol., 2009, 75(23), 7378–7384 CrossRef CAS PubMed.
- A. K. Yetisen, M. S. Akram and C. R. Lowe, Lab Chip, 2013, 13(12), 2210–2251 RSC.
- N. Panpradist, B. J. Toley, X. Zhang, S. Byrnes, J. R. Buser, J. A. Englund and B. R. Lutz, PLoS One, 2014, 9(9), e105786 Search PubMed.
- B. J. Toley, B. McKenzie, T. Liang, J. R. Buser, P. Yager and E. Fu, Anal. Chem., 2013, 85(23), 11545–11552 CrossRef CAS PubMed.
Footnotes |
† Electronic Supplementary Information (ESI) available: Video of audio-powered lysis device. See DOI: 10.1039/c5lc00080g |
‡ Audacity(R) software is copyright (c) 1999–2012 Audacity Team. [Web site: http://audacity.sourceforge.net/. It is free software distributed under the terms of the GNU General Public License.] The name Audacity(R) is a registered trademark of Dominic Mazzoni. |
|
This journal is © The Royal Society of Chemistry 2015 |