DOI:
10.1039/C5FO01086A
(Paper)
Food Funct., 2016,
7, 140-150
Anti-steatotic effects of an n-3 LCPUFA and extra virgin olive oil mixture in the liver of mice subjected to high-fat diet
Received
8th September 2015
, Accepted 30th September 2015
First published on 5th October 2015
Abstract
Non-alcoholic fatty liver disease (NAFLD) is characterized by liver steatosis, oxidative stress, and drastic depletion of n-3 long-chain polyunsaturated fatty acids (n-3 LCPUFA), namely, eicosapentaenoic acid (C20:5 n-3, EPA) and docosahexaenoic acid (C22:6 n-3, DHA), which trigger lipolysis stimulation and lipogenesis inhibition. Extra virgin olive oil (EVOO) has important antioxidant effects. This study evaluated the anti-steatotic effects of n-3 LCPUFA plus EVOO in the liver of male C57BL/6J mice subjected to a control diet (CD) (10% fat, 20% protein, 70% carbohydrate) or high fat diet (HFD) (60% fat, 20% protein, 20% carbohydrate), without and with supplementation with n-3 LCPUFA (100 mg per kg per day) plus EVOO (100 mg per kg per day) for 12 weeks. HFD induced (i) liver steatosis (increased total fat, triacylglycerols, and free fatty acid total contents), (ii) higher fasting serum glucose and insulin levels and HOMA index, total cholesterol, triacylglycerols and TNF-α and IL-6, (iii) liver and plasma oxidative stress enhancement, (iv) depletion of the n-3 LCPUFA hepatic content, and (v) increment in lipogenic enzyme activity and reduction in lipolytic enzyme activity. These changes were either reduced (p < 0.05) or normalized to control the values in animals subjected to HFD supplemented with n-3 LCPUFA plus EVOO. In conclusion, n-3 LCPUFA plus EVOO intervention exerts anti-steatotic effects underlying antioxidant and anti-inflammatory responses, improved insulin sensitivity, and recovery of the lipolytic/lipogenic status of the liver altered by HFD, and supports the potential therapeutic use of n-3 LCPUFA plus EVOO supplementation in the treatment of human liver steatosis induced by nutritional factors or other etiologies.
Introduction
Hepatic steatosis corresponds to an abnormal intracellular accumulation of triacylglycerols in the cytoplasm of hepatocytes, a condition known as non-alcoholic fatty liver disease (NAFLD).1 NAFLD is frequently associated with obesity and insulin resistance in patients with negligible alcohol consumption, represents the most common chronic liver disease worldwide,2 and exhibits a pathogenic overlapping with diabetes and cardiovascular disease.3 The development of NAFLD is directly associated with an enhancement in the pro-oxidant status of the liver, a feature reported in obese patients4 and in mice subjected to high-fat diet (HFD).5 Liver oxidative stress in NAFLD is associated with depletion of hepatic n-3 long-chain polyunsaturated fatty acids (n-3 LCPUFAs), a change that has been ascribed to loss by lipid peroxidation and reduction in the biosynthetic capacity of the liver.6 The latter alteration is related to low intake of the n-3 LCPUFA precursor α-linolenic acid (C18:3 n-3, ALA) and high intake of trans isomers (elaidic acid; c18:1 n-9 trans) as desaturase inhibitors,6 thus determining a drastic diminution in the Δ-5 and Δ-6 desaturase enzymatic activity of the liver,7 a finding also observed in HFD-induced liver steatosis in mice.8 In addition, the development of a pro-inflammatory status may promote NAFLD progression from steatosis to steatohepatitis, and then cirrhosis.9,10
For more than four decades, epidemiological, clinical, biochemical, and physiological studies have established the importance and benefits of n-3 LCPUFA, particularly eicosapentaenoic acid (C20:5 n-3, EPA) and docosahexaenoic acid (C22:6 n-3, DHA). These fatty acids have been associated with key roles in numerous physiological functions, suggesting that their administration may prevent several non-transmissible chronic diseases.11,12 EPA and DHA are also important regulators of lipid metabolism, having key cytoprotective properties as anti-inflammatory and neuroprotective actions.13 In fact, recent studies have established beneficial effects on prevention of liver steatosis.5 Furthermore, anti-steatotic effects of n-3 LCPUFA in the liver include directing fatty acids away from triglyceride storage promoting their oxidation.14
Extra virgin olive oil (EVOO) is a dietary component representing a characteristic food of the Mediterranean diet, which is considered as healthy for its antioxidant, anti-inflammatory, and cardiovascular protective actions.15 Nutritionally, EVOO is a good source of oleic acid (C18:1, n-9, OA), a fatty acid (FA) that is regarded as one of the factors explaining the health effects of the Mediterranean diet.16 Furthermore, EVOO is also characterized by its high content of tocopherols (particularly alpha-tocopherol) and different polyphenols, hydroxytyrosol being the most relevant antioxidant with healthy properties present in EVOO.17 The antioxidant and anti-inflammatory effects of EVOO and the favorable effects described for n-3 LCPUFA may have strong synergistic healthy benefits for consumers. Actually, ingestion of n-3 LCPUFA and EVOO constitutes a nutritional recommendation.18 In view of these considerations, the present study was aimed to test the hypothesis that dietary n-3 LCPUFA mixed EVOO supplementation triggers antioxidant and anti-inflammatory responses that prevent liver steatosis induced by HFD with less proportion of n-3 LCPUFA feeding in mice.
Material and methods
Ethics statement
Experimental animal protocols and animal procedures complied with the Guide for the Care and Use of Laboratory Animals (National Academy of Sciences, NIH Publication 6-23, revised 1985) were approved by the Bioethics Committee for Research in Animals, Faculty of Medicine, University of Chile (CBA #0630 FMUCH).
Animal preparation and supplementation with n-3 LCPUFA and/or EVOO
Weaning male C57BL/6J mice weighing 12–14 g (Bioterio Central, ICBM, Faculty of Medicine, University of Chile) were randomly assigned to each experimental group (n = 10–12 per experimental group) and were allowed free access to specially formulated control or high fat diets. The composition of the control diet (CD) (expressed as % total calories) was 10% fat, 20% protein, and 70% carbohydrate, with a caloric value of 3.85 kcal g−1, free of EPA and DHA, and contained 0.7 g of α-linolenic acid (ALA) per 100 g of diet. The composition of the HFD was 60% fat, 20% protein, and 20% carbohydrate, with a caloric value of 5.24 kcal g−1, free of EPA and DHA, and contained 0.7 g of ALA per 100 g of diet (Research Diet INC, Rodent Diet, Product data D12450B and D12492, USA). The animals received water ad libitum and were housed on a 12-hour light/dark cycle from days 1 to 84 (12 weeks). During this period the n-3 LCPUFA supplemented groups received fish oil (encapsulated fish oil containing 600 mg [400 mg EPA + 200 mg DHA] g−1; UP UltraOmega3, New Science, Chile) or EVOO (Huasco Valley, Atacama, Chile), supplemented groups received 100 mg per day, through oral administration and the control groups received isovolumetric amounts of saline, thus comprising eight experimental groups: (a) CD (control), (b) CD plus n-3 LCPUFA, (c) CD plus EVOO, (d) CD plus n-3 LCPUFA + EVOO, (e) HFD, (f) HFD n-3 LCPUFA, (g) HFD + plus EVOO and (h) HFD plus n-3 LCPUFA + EVOO. Under these conditions the n-3 LCPUFA groups received daily doses of 67 mg kg−1 of EPA and 33 mg kg−1 of DHA. EVOO presents 71% of oleic acid with respect to the total fatty acid content, 860 mg of total polyphenols per L of EVOO and 250 mg of alpha-tocopherol per L of EVOO. Weekly controls of the body weight and diet intake were performed through the whole period. At the end of the 12th week, the animals were fasted (6–8 h), anesthetized with ketamine and xylazine (150 and 10 mg kg−1, respectively), and blood samples were obtained by cardiac puncture for serum AST, ALT, glucose, insulin, triacylglycerols, total cholesterol, LDL-cholesterol, and HDL-cholesterol assessments. Liver samples were frozen in liquid nitrogen for determination of the fatty acid composition; in addition, the liver samples were fixed in phosphate-buffered formalin, embedded in paraffin, stained with hematoxylin-eosin and analysed by optical microscopy in a blind fashion describing the presence of steatosis and inflammation, both graded as absent, mild, moderated and severe.19 In this case visceral adipose tissue only included two adipose tissues associated with epididymis of mice, and these tissues were totally removed and weighed, according Tran et al.20
Measurements of serum parameters and fat liver content
Serum glucose (mM), cholesterol (mg per 100 mL), LDL cholesterol (mg per 100 mL), HDL cholesterol (mg per 100 mL) and triacylglycerol levels (mg dL−1) were measured using specific diagnostic kits (Wiener Lab, Argentina). A commercial immunoassay kit for mice serum insulin assessment (μU mL−1) was used, according to the manufacturer's instructions (Mercodia, Uppsala, Sweden). Insulin resistance was estimated by the homeostasis model assessment method (HOMA) [fasting insulin (μU mL−1) × fasting glucose (mM)/22.5].21 Serum aspartate transaminase (AST) and alanine transaminase (ALT) activities (U L−1) were measured using specific diagnostic kits (Biomerieux SA, Marcy l'Etoile, France). ELISA kits were used for assessment of serum levels (pg mL−1) of TNF-α and IL-6 (Cayman Chemical Company, Ann Arbor, MI, USA). The liver total fat content (mg g−1) was evaluated according to Bligh and Dyer,22 and triacylglycerols (mg g−1) and free fatty acid (μM g−1) levels in the liver were measured using specific kits, according to the manufacturer's instructions (Cayman Chemical Company, Ann Arbor, MI, USA).
Assays for oxidative stress-related parameters in the liver and plasma
In anesthetized animals, livers were perfused in situ with a cold solution containing 150 mM KCl and 5 mM Tris (pH 7.4) to remove the blood for glutathione and protein carbonylation assessments. The reduced glutathione (GSH) and glutathione disulfide (GSSG) contents were assessed with an enzymatic recycling method.23 The contents of protein carbonyls, F2-isoprostanes, and thiobarbituric acid reactants (TBARs) in the liver and the plasma levels of TBARs and the antioxidant capacity of plasma were measured using specific kits, according to the manufacturer's instructions (Cayman Chemical Company, Ann Arbor, MI, USA).
Enzymatic activity assay in liver tissue
Acetyl CoA carboxylase (ACC) activity was determined using the method of Zimmermann et al.24 Briefly, 1 g frozen liver was homogenized with 3 volumes of phosphate bicarbonate buffer (composition in mmol L−1: KHCO3 70; K2HPO4 85; KH2PO4 9; dithiothreitol 1, pH 7.0). The cytosolic fraction was obtained after centrifuging the supernatant at 100
000g for 1 h at 4 °C. The ACC activity was measured using an NADH-linked assay.24 The assay medium (56 mmol L−1 Tris-HCl, pH 8.0, 10 mmol L−1 MgCl2, 11 mmol L−1 EDTA, 4 mmol L−1 ATP, 52 mmol L−1 KHCO3, 0.75 mg mL−1 bovine serum albumin (BSA), 0.5 mmol L−1 NADH and 1.4 mmol L−1 phosphoenolpyruvate) was mixed with 5.6 U mL−1 pyruvate kinase and 5.6 U mL−1 lactate dehydrogenase. The baseline was followed at 30 °C until a constant slope was reached. For every 2.3 volumes of the medium, 1 volume of the activated homogenate was added and the reaction was started with acetyl-CoA (0.125 mmol L−1 final concentration). For enzymatic activation, 1 volume of the homogenate was incubated with 1 volume of activation buffer (20 mmol L−1 citrate, 100 mmol L−1 Tris-HCl, pH 8.0, 1.5 mg mL−1 BSA, 20 mmol L−1 MgCl2 and 20 mmol L−1 reduced glutathione (GSH, pH 7.5) for 15 min at 37 °C. The fatty acid synthase (FAS) activity was assessed in cytosolic liver tissue fractions by measuring malonyl CoA-dependent NADPH oxidation at 37 °C as described by Halestrap et al.25 The activity of carnitine-palmitoyl transferase-1 (CPT-1) was determined spectrophotometrically using the method described by Karlic et al.26
Fatty acid profile
Quantitative extraction and separation of total lipids from the liver were carried out according to Bligh and Dyer,22 containing butylated hydroxytoluene (BHT) as an antioxidant. Erythrocytes and tissue samples were homogenized in ice-cold chloroform/methanol (2
:
1 v/v) containing 0.01% BHT in an UltraTurrax homogenizer (Janke & Kunkel, Stufen, Germany). Total lipids from the liver samples were extracted with chloroform/isopropanol (2
:
1 v/v).
Preparation and gas chromatographic analysis of fatty acid methyl esters (FAME)
FAME from the total lipid liver samples were prepared with boron trifluoride (12% methanolic solution) according to Morrison and Smith,27 and followed by methanolic sodium hydroxide (0.5 N) solution. Phospholipids for FAME synthesis were extracted from the silica gel spots with 15 mL of chloroform/methanol/water (10
:
10
:
1) and evaporated under a nitrogen stream. FAME samples were cooled and extracted with 0.5 mL of hexane. FAME were separated and quantified by gas-liquid chromatography in an Agilent Hewlett-Packard equipment (model 7890A, CA, USA) using a capillary column (Agilent HP-88, 100 m × 0.250 mm; I.D. 0.25 μm) and a flame ionization detector (FID). The injector temperature was set at 250 °C and the FID temperature at 300 °C. The oven temperature at injection was initially set at 140 °C and was programmed to increase to 220 °C at a rate of 5 °C per min. Hydrogen was utilized as the carrier gas (35 cm per second flow rate) in the column and the inlet split ratio was set at 20
:
1. The identification and quantification of FAME were achieved by comparing the retention times and the peak area values (%) of the unknown samples with those of a commercial lipid standard (Nu-Chek Prep Inc.). C23:0 was used as an internal standard (Nu-Chek Prep Inc., Elysian MN, USA) and a Hewlett-Packard Chemstation (Palo Alto, CA, USA) data system was used for peak analysis.
Statistical analysis
Statistical analysis was performed with GraphPad Prism 6.0 software (GraphPad Prism Software, Inc., San Diego, USA). The values shown represent the mean ± SEM for each experimental group. Evaluations of normality data distribution were performed using the Shapiro–Wilk test. Assessment of the statistical significance of differences between the mean values was performed by two-way-ANOVA and the Bonferroni post-test. A P < 0.05 was considered significant.
Results
n-3 LCPUFA + EVOO supplementation reduces the HFD-induced increase in visceral adipose and hepatic parameters
Mice subjected to the indicated dietary protocols and exhibiting comparable initial body weights showed similar increases in the final body weights in the CD fed groups given saline, n-3 LCPUFA, EVOO, and n-3 LCPUFA + EVOO for 12 weeks, which were significantly enhanced by HFD feeding (Table 1A). Under these conditions, the liver weight was not modified, but the liver weight/final body weight ratio showed significant reduction in HFD fed mice compared to CD fed groups. However, n-3 LCPUFA + EVOO supplementation prevents this effect in the mice fed HFD (Table 1A). The visceral adipose tissue weight in HFD groups was 267%, 182%, 245%, and 106% higher than those given CD and subjected to saline, n-3 LCPUFA, EVOO, and n-2 LCPUFA + EVOO, respectively (Table 1A). The content of hepatic total fat, triacylglycerol, and free fatty acids in the control (CD) animals was unchanged by the different supplementations, however, these parameters were significantly elevated by HFD, with the values found in the mice given HFD and supplemented with n-3 LCPUFA + EVOO being significantly lower than those subjected to saline, n-3 LCPUFA, or EVOO alone (Table 1B). Interestingly, n-3 LCPUFA + EVOO generated a normalization in these parameters compared with the CD group (Table 1B).
Table 1 General and biochemical parameters in control mice and high fat diet fed mice subjected to n-3 LCPUFA, EVOO, and n-3 LCPUFA plus EVOO supplementation
|
Groups |
Control diet (CD) |
High fat diet (HFD) |
Saline |
n-3 LCPUFA |
EVOO |
n-3 LCPUFA + EVOO |
Saline |
n-3 LCPUFA |
EVOO |
n-3 LCPUFA + EVOO |
Values represent means ± SEM for 7–10 mice per experimental group. Significant differences between the groups are indicated by the letter identifying each group (p < 0.05; by two-way ANOVA and Bonferroni post-test). |
A. General parameters |
(a) |
(b) |
(c) |
(d) |
(e) |
(f) |
(g) |
(h) |
Initial body weight (g) |
13.8 ± 0.7 |
13.7 ± 0.7 |
13.8 ± 0.6 |
13.7 ± 0.7 |
13.9 ± 0.8 |
14.1 ± 0.8 |
14.3 ± 0.8 |
13.5 ± 0.6 |
Final body weight (g) |
27.6 ± 1.3e,f,g,h |
27.5 ± 1.5e,f,g,h |
27.0 ± 2.0e,f,g,h |
26.6 ± 1.4e,f,g,h |
38.9 ± 0.9a,b,c,d |
38.3 ± 1.1a,b,c,d |
38.1 ± 0.9a,b,c,d |
37.9 ± 0.9a,b,c,d |
Liver weight (g) |
0.96 ± 0.1 |
0.99 ± 0.1 |
1.00 ± 0.2 |
0.96 ± 0.2 |
1.07 ± 0.2 |
1.14 ± 0.2 |
1.05 ± 0.2 |
1.09 ± 0.2 |
Liver weight (g)/final body weight (g) ratio |
0.035 ± 0.002e,g |
0.036 ± 0.003e,g |
0.037 ± 0.004e,g |
0.036 ± 0.004e,g |
0.028 ± 0.003a,b,c,d |
0.030 ± 0.003c |
0.028 ± 0.002a,b,c,d |
0.029 ± 0.004 |
Visceral adipose tissue (g) |
1.06 ± 0.3e,f,g,h |
1.03 ± 0.2e,f,g,h |
1.07 ± 0.2e,f,g,h |
1.07 ± 0.3e,f,g,h |
3.89 ± 0.5a,b,c,d,f,h |
2.90 ± 0.4a,b,c,d,e,g |
3.70 ± 0.3a,b,c,d,f,h |
2.20 ± 0.5a,b,c,d,e,g |
|
B. Liver parameters |
Total fat (mg per g liver) |
33.4 ± 5.8e,f,g |
29.1 ± 2.5e,f,g,h |
30.4 ± 2.7e,f,g,h |
28.5 ± 4.2e,f,g,h |
102.4 ± 4.6a,b,c,d,f,g,h |
84.6 ± 6.4a,b,c,d,e,h |
81.4 ± 10.1a,b,c,d,e,h |
42.90 ± 5.0b,c,d,e,f,g |
Triacylglycerols (mg per g liver) |
31.3 ± 2.7e,f,g |
26.3 ± 2.3e,f,g,h |
26.0 ± 2.8e,f,g,h |
27.5 ± 2.1e,f,g,h |
94.7 ± 7.8a,b,c,d,f,g,h |
75.7 ± 11.9a,b,c,d,e,h |
79.2 ± 9.0a,b,c,d,e,h |
37.2 ± 3.9b,c,d,e,f,g |
Free fatty acid (μM per g liver) |
280.9 ± 26.7e,f,g |
244.7 ± 10.7e,f,g |
253.5 ± 12.5e,f,g |
244.0 ± 11.7e,f,g |
749.0 ± 26.1a,b,c,d,f,g,h |
566.8 ± 38.9a,b,c,d,e,h |
641.6 ± 63.7a,b,c,d,e,h |
303.9 ± 36.0b,c,d,e,f,g |
|
C. Serum parameters |
Triacylglycerols (mg dL−1) |
127.0 ± 9.3e,f,g,h |
120.6 ± 9.9e,f,g,h |
123.7 ± 5.8e,f,g,h |
123.0 ± 4.0e,f,g,h |
172.8 ± 12.4a,b,c,d,h |
163.2 ± 6.8a,b,c,d,h |
154.4 ± 8.7a,b,c,d,h |
143.5 ± 5.6a,b,c,d,e,f,g |
Total cholesterol (mg dL−1) |
75.9 ± 10.0e,f,g,h |
73.9 ± 7.9e,f,g,h |
69.9 ± 7.7e,f,g,h |
74.4 ± 8.3e,f,g,h |
136.9 ± 10.1a,b,c,d,h |
132.1 ± 8.1a,b,c,d,h |
124.5 ± 4.1a,b,c,d,e |
117.7 ± 7.2a,b,c,d,e,f,g |
LDL-cholesterol (mg dL−1) |
49.1 ± 5.9e,f,g |
49.6 ± 4.4e,f,g |
49.8 ± 3.2e,f,g |
47.8 ± 5.8e,f,g |
83.9 ± 3.3a,b,c,d,h |
75.4 ± 5.5a,b,c,d,h |
73.8 ± 4.0a,b,c,d,e,h |
50.3 ± 5.3e,f,g |
HDL-cholesterol (mg dL−1) |
32.9 ± 7.4e,f,g,h |
30.5 ± 12.9e,f,g,h |
26.4 ± 15.8e,f,g,h |
32.6 ± 13.1e,f,g,h |
63.5 ± 27.2a,b,c,d |
66.2 ± 27.3a,b,c,d |
59.8 ± 29.1a,b,c,d |
73.7 ± 24.4a,b,c,d |
|
D. Insulin resistance |
Fasting glucose (mg dL−1) |
126.4 ± 14.6e,f,g |
101.3 ± 11.9e,f,g,h |
108.5 ± 13.6e,f,g,h |
108.4 ± 16.5e,f,g,h |
237.1 ± 33.9a,b,c,d |
181.9 ± 22.9a,b,c,d |
224.7 ± 23.8a,b,c,d |
147.9 ± 11.2b,c,d,e,f,g |
Fasting insulin (units per mL) |
5.60 ± 0.93e,f,g |
5.16 ± 0.67e,f,g |
5.13 ± 0.82e,f,g |
5.24 ± 0.64e,f,g |
15.59 ± 2.15a,b,c,d,f,g,h |
10.63 ± 1.39a,b,c,d,e,h |
12.79 ± 1.73a,b,c,d,e,h |
5.58 ± 0.83e,f,g |
HOMA |
1.20 ± 0.1e,f,g |
1.20 ± 0.05e,f,g |
1.15 ± 0.06e,f,g |
1.17 ± 0.07e,f,g |
8.69 ± 0.77a,b,c,d,h |
6.08 ± 0.54a,b,c,d,h |
7.44 ± 0.52a,b,c,d,h |
1.22 ± 0.1e,f,g |
|
E. Serum transaminases |
AST (U L−1) |
139.5 ± 11.0 |
143.4 ± 14.0 |
145.4 ± 10.1 |
140.3 ± 6.8 |
145.7 ± 10.1 |
142.5 ± 8.6 |
141.2 ± 10.6 |
140.0 ± 12.3 |
ALT (U L−1) |
72.4 ± 8.1 |
69.0 ± 6.8 |
64.6 ± 6.2 |
69.0 ± 3.6 |
68.5 ± 7.6 |
67.1 ± 5.0 |
64.2 ± 7.7 |
65.2 ± 5.4 |
|
F. Serum cytokines |
IL-6 (pg mL−1) |
29.6 ± 7.8e,f,g |
29.5 ± 2.9e,f,g |
32.1 ± 6.6e,f,g |
28.6 ± 6.1e,f,g |
62.7 ± 12.0a,b,c,d,h |
50.8 ± 8.1a,b,c,d,h |
59.3 ± 10.0a,b,c,d,h |
31.5 ± 8.2e,f,g |
TNF-α (pg mL−1) |
23.8 ± 4.6e,f,g |
22.0 ± 2.9e,f,g |
24.5 ± 3.5e,f,g |
23.0 ± 3.70e,f,g |
51.7 ± 9.8a,b,c,d,h |
38.6 ± 6.3a,b,c,d,h |
43.7 ± 7.0a,b,c,d,h |
24.9 ± 4.3e,f,g |
n-3 LCPUFA + EVOO supplementation diminishes HFD-induced enhancements in serum lipid levels without changing those of HDL-cholesterol
The levels of serum triacylglycerols, total cholesterol, LDL-cholesterol, and HDL-cholesterol were (i) comparable to those in the mice given CD and supplemented with either saline, n-3 LCPUFA, EVOO, or n-3 LCPUFA + EVOO; (ii) significantly elevated by HFD over CD values under the different supplementations; and (iii) reduced (P < 0.05) in the mice given HFD and n-3 LCPUFA + EVOO supplementation compared to those treated with individual saline, n-3 LCPUFA, or EVOO, with the exception of HDL-cholesterol values that remained constant in this group (Table 1C).
n-3 LCPUFA + EVOO supplementation normalizes HFD-induced enhancements in serum levels of glucose, insulin, and HOMA values
The mice subjected to HFD exhibited 88% and 178% increases in the serum levels of fasting glucose and insulin, respectively, with a consequent 7.2-fold enhancement in the HOMA index over those given CD, changes that were not modified by individual n-3 LCPUFA or EVOO supplementation (Table 1D). When compared to control values, HFD-induced insulin resistance was abolished in the animals receiving HFD + n-3 LCPUFA + EVOO supplementation, however no significant alterations were achieved in HOMA values by individual n-3 LCPUFA or EVOO supplementation in the animals fed with CD or HFD (Table 1D).
n-3 LCPUFA + EVOO supplementation suppresses HFD-induced higher serum IL-6 and TNF-α levels, liver steatosis, and liver morphological alterations
Experimental groups subjected to CD and HFD protocols exhibited no significant changes in serum AST and ALT activities (Table 1E). In relation to CD, the HFD group exhibited significantly enhanced serum levels of IL-6 and TNF-α, an effect that was suppressed by n-3 LCPUFA + EVOO supplementation in the HFD group (Table 1F). The mice given CD and subjected to saline (Fig. 1A), n-3 LCPUFA (Fig. 1B), EVOO (Fig. 1C), or n-3 LCPUFA + EVOO (Fig. 1D) exhibited normal histology. HFD induced macrovesicular and microvesicular hepatic steatosis (Fig. 1E), a feature that did not achieve significant reduction upon supplementation with n-3 LCPUFA (Fig. 1F) or EVOO (Fig. 1G), whereas it was reverted by n-3 LCPUFA + EVOO supplementation, with the persistence of few steatosis foci (Fig. 1H).
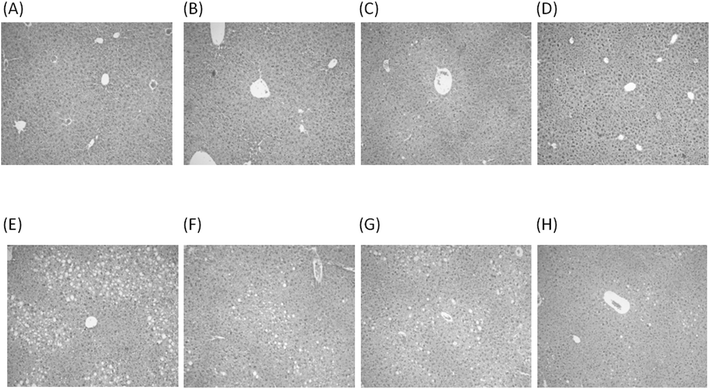 |
| Fig. 1 Liver histology in control mice and high fat diet (HFD) fed animals subjected to n-3 LCPUFA, EVOO, and n-3 LCPUFA plus EVOO supplementation. Representative liver sections from animals given (A) control diet (CD), (B) CD plus n-3 LCPUFA, (C) CD plus EVOO, (D) CD plus n-3 LCPUFA mixed with EVOO, (E) HFD, (F) HFD plus n-3 LCPUFA, (G) HFD plus EVOO and (H) HFD plus n-3 LCPUFA mixed with EVOO (hematoxylin–eosin liver sections from a total of 9 animals per experimental group; original magnification ×10). | |
HFD-induced changes in plasma and liver oxidative stress-related parameters are abolished by n-3 LCPUFA + EVOO supplementation
HFD-induced increases (P < 0.05) in the plasma levels of TBARs were normalized after HFD plus n-3 LCPUFA + EVOO supplementation (Fig. 2A), a protocol that also returned to normal the decline in the antioxidant capacity of plasma, as compared to the control values (Fig. 2B). The animals subjected to HFD with saline, n-3 LCPUFA, or EVOO supplementation exhibited decreased liver GSH contents compared to the respective CD groups, whereas those in the HFD group supplemented with n-3 LCPUFA + EVOO were comparable to the CD mice subjected to saline (Fig. 2C). Under these conditions, the liver GSSG levels were not modified in all studied groups (Fig. 2D), however, total GSH equivalent depletion was normalized in the HFD + n-3 LCPUFA + EVOO supplementation group compared to the HFD group, reaching the values comparable to the CD group (Fig. 2E). Consistent with these results, the liver GSH/GSSS ratios in the mice subjected to HFD + n-3 LCPUFA + EVOO were comparable to the values observed in the CD group (Fig. 2F). HFD-induced increases (P < 0.05) in the hepatic content of protein carbonyls (Fig. 2G), F2-isoprostanes (Fig. 2H), and TBARs (Fig. 2I) over the CD values were abolished by the combined HFD plus n-3 LCPUFA + EVOO supplementation.
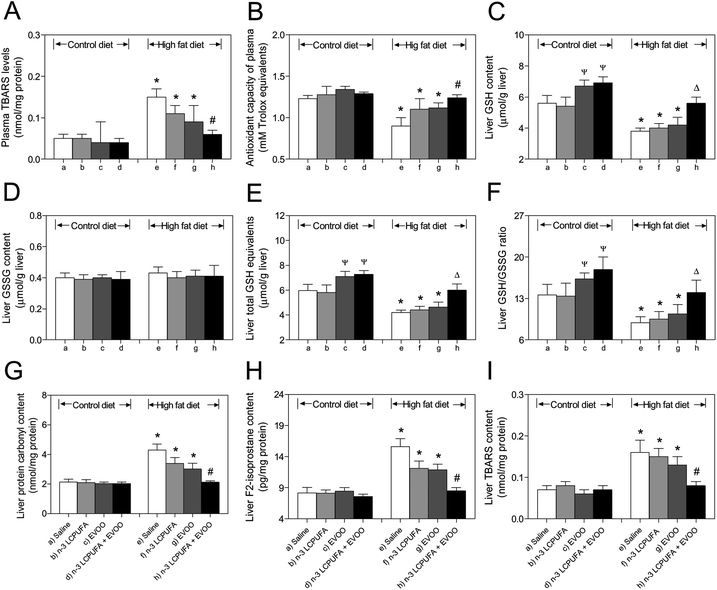 |
| Fig. 2 Oxidative stress-related parameters in the liver of control mice and high fat diet (HFD) fed mice subjected to n-3 LCPUFA, EVOO, and n-3 LCPUFA plus EVOO supplementation. Values represent means ± SEM for 7–10 mice per experimental group. Significant differences between the groups (two-way ANOVA and Bonferroni post-test): *p < 0.05 versus a,b,c,d,h; #p < 0.05 versus e,f,g; Ψp < 0.05 versus a,b; Δp < 0.05 versus c,d,e,f,g. Total GSH equivalents = GSH + 2GSSG. | |
n-3 LCPUFA + EVOO supplementation normalizes the changes in lipogenic and lipolytic hepatic enzyme activities induced by HFD
Liver ACC, FAS, and CTP-1 activities in CD animals were comparable among the groups supplemented with saline, n-3 LCPUFA, EVOO, or n-3 LCPUFA + EVOO (Fig. 3A–C). HFD induced 96% and 167% increases in the activity of the ACC (Fig. 3A) and FAS (Fig. 3B) over basal values, respectively, with concomitant reduction of 49% to 59% in the activity of CPT-1 (Fig. 3C), when compared to the respective control values, effects that were normalized in the mice receiving HFD plus n-3 LCPUFA + EVOO supplementation (Fig. 3A–C). Under these conditions, HFD plus n-3 LCPUFA alone or EVOO alone did not achieve the recovery in these parameters compared to the control group fed with HFD (Fig. 3A–C).
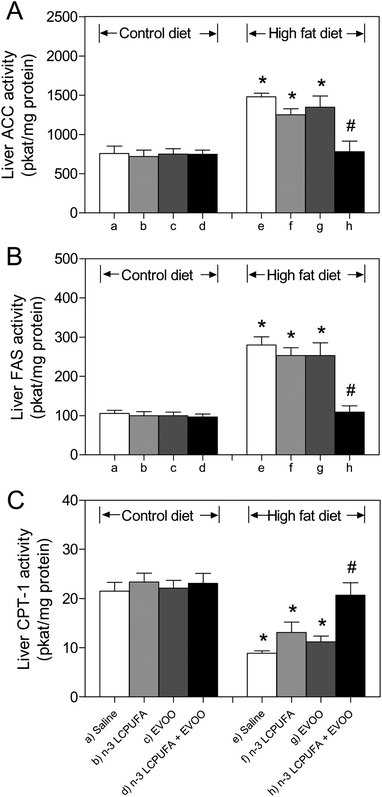 |
| Fig. 3 Changes in lipogenic and lipolytic enzyme activity in control mice and high fat diet (HFD) fed mice subjected to n-3 LCPUFA, EVOO, and n-3 LCPUFA plus EVOO supplementation. Values represent means ± SEM for 7–10 mice per experimental group. Significant differences between the groups (two-way ANOVA and Bonferroni post-test): *p < 0.05 versus a,b,c,d,h; #p < 0.05 versus e,f,g. | |
Effects of n-3 LCPUFA, EVOO, and n-3 LCPUFA + EVOO supplementation on the liver fatty acid composition in CD and HFD fed mice
The HFD fed mice exhibited an increment (P < 0.05) in the hepatic content of total SFA (24%), which is mainly due to palmitic acid (C16:0) enhancement (30%), without significant changes in that of total MUFA, compared to the control values (Table 2). In parallel, significant reductions in the content of PUFA (35%), LCPUFA (44%), n-6 and n-3 LCPUFA (41% and 52% respectively) were observed after HFD feeding over the CD values, regardless of n-3 LCPUFA, EVOO, or n-3 LCPUFA supplementation (Table 2). Under these conditions, diminutions (P < 0.05) in the hepatic content of LA (25%), ALA (39%), AA (65%), EPA (140%) and DHA (102%) were found in the mice given HFD, besides an increment in the n-6 LCPUFA/n-3 LCPUFA ratio (18%), compared to CD (Table 2). Supplementation with n-3 LCPUFA + EVOO in the HFD fed mice achieved normalization of the hepatic content of the total SFA, palmitic acid, ALA, DHA and n-6 LCPUFA/n-3 LCPUFA ratios compared with the CD group, without normalization of the LA, AA, EPA, PUFA, LCPUFA, n-6 LCPUFA and n-3 LCPUFA levels (Table 2). Furthermore, n-3 LCPUFA supplementation increased the liver total n-3 LCPUFA content in 3.19 g per 100 g FAME in the mice subjected to CD (Table 2; b–a) and in 1.25 g per 100 g FAME in those given HFD (Table 2; f–e), thus representing 61% reduction in the hepatic n-3 LCPUFA levels by HFD.
Table 2 Fatty acid composition of total liver lipids obtained from control mice and high fat diet (HFD) fed mice subjected to n-3 LCPUFA, EVOO and n-3 LCPUFA plus EVOO supplementation
|
Fatty acid composition (g per 100 g FAME) |
Groups |
Control diet (CD) |
High fat diet (HFD) |
Saline |
n-3 LCPUFA |
EVOO |
n-3 LCPUFA + EVOO |
Saline |
n-3 LCPUFA |
EVOO |
n-3 LCPUFA + EVOO |
Values are expressed as g fatty acid per 100 g FAME and represent the mean ± SEM for n = 8 mice per experimental group. The groups were compared by two-way ANOVA and Bonferroni post-test. (p < 0.05), with significant differences being indicated by the letter identifying each group. Saturated fatty acids (SFA) correspond to 14:0, 16:0 and 18:0. Monounsaturated fatty acids (MUFA) correspond to 14:1, n-7, 16:1, n-7 and 18:1, n-9. Polyunsaturated fatty acids (PUFA) correspond to 18:2, n-6, 18:3, n-3, 20:4, n-6, 20:5, n-3, 22:5, n-3, and 22:6, n-3; n-6 long-chain polyunsaturated fatty acids (LCPUFA) are 20:4, n-6; n-3 LCPUFA are 20:5, n-3, 22:5, n-3, and 22:6, n-3; n-6/n-3 ratio: 20:4, n-6/(20:5, n-3 + 22:5, n-3 + 22:6, n-3). |
Fatty acid |
(a) |
(b) |
(c) |
(d) |
(e) |
(f) |
(g) |
(h) |
16:0 |
35.1 ± 3.5e |
33.2 ± 3.1e |
33.0 ± 5.0e |
30.1 ± 3.8e,f,g |
45.8 ± 3.2a,b,c,d,h |
41.4 ± 3.8d |
42.6 ± 3.7b,c,d |
37. 9 ± 3.9e |
18:1, n-9 |
21.7 ± 2.8c,d,g,h |
20.9 ± 1.9c,d,g,h |
28.9 ± 3.1a,b,e |
26.6 ± 3.2b |
23.6 ± 3.6g |
24.9 ± 2.9g |
31.2 ± 2.7b |
29.1 ± 2.7a,b |
18:2, n-6 (LA) |
15.3 ± 1.9b,c,d,e,f,g,h |
10.8 ± 1.3a |
12.3 ± 0.9a |
11.7 ± 1.3a |
11.5 ± 1.4a |
10.9 ± 0.8a |
12.4 ± 1.2a |
12.0 ± 1.4a |
18:3, n-3 (ALA) |
1.10 ± 0.2e |
1.07 ± 0.1e |
0.95 ± 0.1e |
0.99 ± 0.1e |
0.79 ± 0.04a,b,c,d,f,g,h |
1.01 ± 0.7e |
1.03 ± 0.5e |
1.05 ± 0.6e |
20:4, n-6 (AA) |
12.4 ± 1.0b,d,e,f,g,h |
9.02 ± 0.9a |
10.2 ± 1.2a,e,f |
8.14 ± 0.8a |
7.51 ± 0.7a,c |
7.95 ± 0.7a,c |
8.01 ± 0.9a |
9.03 ± 0.8a |
20:5, n-3 (EPA) |
1.03 ± 0.05b,c,e,g |
2.82 ± 0.4a,c,e,f,g,h |
0.78 ± 0.04a,b,d,e,f,g,h |
2.16 ± 0.5a,c,e,f,g,h |
0.43 ± 0.06a,b,c,d,f,g,h |
0.89 ± 0.1a,b,d,h |
0.65 ± 0.05a,b,d,e,f,h |
0.95 ± 0.06a,b,c,d,e,f,g |
22:6, n-3 (DHA) |
4.06 ± 0.3b,c,d,e,f,g,h |
5.67 ± 0.7a,c,b,e,f,g,h |
3.69 ± 0.4a,b,d,e,f,g |
4.25 ± 0.6b,c,f,g |
2.01 ± 0.4a,b,e,f,g |
2.85 ± 0.7a,b,d |
2.50 ± 0.6a,b,c,d |
3.11 ± 0.8b |
Total SFA |
37.9 ± 3.2e |
35.4 ± 3.0e,f,g |
33.2 ± 3.4e,f,g |
32.9 ± 2.8e,f,g |
47.1 ± 3.7a,b,c |
42.1 ± 2.8b,c,d |
43.2 ± 3.5c,d |
38.0 ± 3.6d |
Total MUFA |
25.7 ± 2.6c,g |
25.1 ± 2.9c,g |
30.8 ± 2.9 |
28.9 ± 2.7 |
29.1 ± 2.9 |
26.5 ± 2.5 |
33.5 ± 3.0 |
30.5 ± 2.9 |
Total PUFA |
36.4 ± 3.5e,g |
39.5 ± 3.7e,f,g,h |
36.0 ± 3.8e,g |
38.2 ± 3.5e,g |
23.8 ± 2.4a,b,c,d,f,h |
31.4 ± 3.0b,e,g |
23.3 ± 2.6a,b,c,d,f,h |
31.5 ± 3.0a,b,d,e,g |
Total LCPUFA |
18.1 ± 1.9d,e,f,g,h |
17.9 ± 1.6d,e,f,g,h |
15.2 ± 1.4e,f,g |
14.7 ± 1.2a,e,f,g |
10.1 ± 1.3a,b,c,d,h |
11.8 ± 1.1a,b,c,d |
11.3 ± 0.9a,b,c,d,h |
13.2 ± 1.1a,b,c,d,e |
Total n-6 LCPUFA |
12.9 ± 1.3b,d,e,f,g,h |
9.51 ± 0.9a,c,d,e,b |
10.4 ± 1.0e |
8.25 ± 0.7a,b,c,e |
7.58 ± 0.8a,b,c,h |
8.03 ± 0.8a,b |
8.11 ± 0.8a,b |
9.05 ± 0.7a,e |
Total n-3 LCPUFA |
5.20 ± 0.3b,c,d,e,f,g,h |
8.39 ± 0.8a,c,d,e,f,g,h |
4.80 ± 0.7b,d,e,f,g,h |
6.45 ± 0.5a,b,c,e,f,g,h |
2.52 ± 0.3a,b,c,d,f,g,h |
3.77 ± 0.5a,b,c,d,e,h |
3.19 ± 0.3a,b,c,d,e,h |
4.15 ± 0.4a,b,d,e,f,g |
n-6 LCPUFA/n-3 LCPUFA ratio |
2.48 ± 0.5b,d,e |
1.13 ± 0.3a,c,d,e,f,g |
2.17 ± 0.4b,d,e |
1.28 ± 0.2a,c,e,f,g,h |
3.01 ± 0.4a,b,c,d,e,f,h |
2.13 ± 0.6b,d,e |
2.54 ± 0.3b,d,e |
2.18 ± 0.4b,d,e |
Discussion
The mice subjected to HFD developed liver steatosis in relation to insulin resistance, oxidative stress, pro-inflammatory and pro-lipogenic responses, with depletion of hepatic PUFA and increment in visceral adipose tissue, most of which also occurred in rats fed a high fat diet or sucrose-rich diet leading to dysfunctional adipose tissue,28,29 and that are prevented by supplementation with n-3 LCPUFA plus EVOO. The combined protocol may be exerting these beneficial effects due to the activation of the transcription factor peroxisome proliferator-activated receptor-α (PPAR-α), promoting fatty acid FA oxidation, concomitantly with downregulation of lipogenic sterol regulatory element binding protein-1c (SREBP-1c) by the n-3 LCPUFA component (EPA + DHA), thus decreasing the pro-lipogenic status of the liver set in by HFD.30,31 This contention is supported by a significant increase in the activity of CPT-1 by n-3 LCPUFA plus EVOO administration toward the control values, which was reduced by HFD, in parallel with a decrease in that of ACC and FAS showing enhancement by HFD. n-3 LCPUFA (EPA + DHA) combined with EVOO normalized the insulin resistance status induced by HFD in mice. In this respect, the main mechanisms involved in the n-3 LCPUFA enhanced insulin sensitivity are (i) GLUT4 upregulation, (ii) inhibition of PPAR-γ, and (iii) downregulation of SREBP-1c,32 with EVOO improving the blood glucose and postprandial insulin response.33,34 Previous studies on mice revealed the hepatoprotective effects of n-3 LCPUFA on different disturbances induced by 12 weeks of HFD, however, these beneficial effects were achieved using 200 mg n-3 LCPUFA (108 mg EPA + 96 mg DHA) per kg per day.5,14 In the present study, the n-3 LCPUFA plus EVOO protocol used prevented all metabolic alterations induced by HFD, effects that were obtained with a lower dose of 100 mg n-3 LCPUFA (67 mg EPA + 33 mg DHA) per kg per day involving a different EPA
:
DHA proportion, which may establish the potentiating effects of n-3 LCPUFA with a low dose of EVOO.
In addition to the anti-steatotic effect of n-3 LCPUFA plus EVOO, the combined protocol elicited reduction in the pro-inflammatory responses induced by HFD, which can be explained by at least three mechanisms. First, n-3 LCPUFA-activated PPAR-α may interact with pro-inflammatory factor nuclear factor-κB (NF-κB)p65 with the formation of inactive PPAR-α/NF-κBp65 complexes.35 Second, EPA and DHA are biotransformed into different derivatives such as resolvins, protectins, and maresines,36,37 or epoxygeneted FAs,38 which are mediators of the resolution of acute and chronic inflammatory states in tissues. Third, the spontaneous lipid peroxidation of EPA and DHA leads to the formation of J3-isoprostanes that activate nuclear factor-erythroid 2 related factor 2 (Nrf2), thus promoting the expression of antioxidant enzymes, with reduction of oxidative stress that deactivates NF-κB, otherwise favoring pro-inflammatory cytokine formation.39,40 In agreement with an increased n-3 LCPUFA utilization for derivative formation, the hepatic content of total n-3 LCPUFA exhibited 61% reduction by HFD compared to CD. Accordingly, the lipid peroxidation-related parameters F2-isoprostanes and TBARs or protein oxidation enhanced by HFD in the liver are normalized by n-3 LCPUFA plus EVOO administration, with the recovery of the antioxidant capacity of plasma. Interestingly, the glutathione status of the liver affected by HFD is also recovered by n-3 LCPUFA plus EVOO, a feature that may involve Nrf2-dependent induction of the enzymes synthesizing hepatic glutathione,39,40 a feature that deserves to be studied under the impact of combined n-3 LCPUFA and oleic acid supplementation.
EVOO has also been postulated as a hepatoprotective product against the development of NAFLD (prevention and/or treatment),41 which was shown to decrease the accumulation of triacylglycerols in the liver of rats subjected to a methionine choline-deficient diet.41 EVOO is characterized by the substantial content of OA and antioxidants, including α-tocopherol, hydroxytyrosol, and oleuropein,16,17 components that may underlie its anti-steatotic effects. Administration of the polyphenols, oleuropein and hydroxytyrosol, significantly reduced the serum glucose and cholesterol levels in diabetic rats,42 oleuropein being able to attenuate liver steatosis in the HFD fed mice by downregulating the Wnt10b- and fibroblast growth factor receptor 1-mediated signaling cascades involved in hepatic lipogenesis.43 Furthermore, in hepatocytes treated with free FA, the addition of oleuropein reduced intracellular triacylglyceride accumulation through inhibition of extracellular signal-regulated kinase.44 Although a clinical controlled trial established that n-3 LCPUFA is efficient in attenuating liver steatosis,45 excess of these FAs may increment oxidative stress and the risk of cardiovascular disease.46 Considering the antioxidant action of the components of EVOO and the prevention of oxidative stress in obesity and metabolic syndrome patients,47 a combination of low doses of n-3 LCPUFA and EVOO may be successful in the prevention or treatment of NAFLD, due to possible synergistic effects. Supporting this proposal is the one-year dietary intervention with n-3 LCPUFA enriched with olive oil, which significantly diminished liver steatosis and improved the adiponectin levels in humans.48 In addition to the antioxidant components of EVOO, OA may also be involved in the beneficial effects of the combined n-3 LCPUFA plus EVOO protocol against HFD-induced lipotoxicity in the liver. This can be visualized in terms of (i) OA-induced promotion of cell tolerance enhancement through increasing cellular antioxidant capacity via development of a mild lipid peroxidation response (lipohormesis);49 and (ii) OA-dependent stimulation of the transcription of genes for PPAR-α, FA translocase (CD36), and mitochondrial β-oxidation enzymes by activation of peroxisome proliferator-activated receptor-γ coactivator 1α (PGC1α) signaling, leading to increased rates of FA oxidation.50
In conclusion, n-3 LCPUFA plus EVOO supplementation prevents HFD-induced liver steatosis, concomitantly with suppression of dyslipidemia, insulin resistance, oxidative stress and pro-inflammatory responses, normalization in the activity of lipogenic and lipolytic hepatic enzymes, and important modifications in the FA liver profile. n-3 LCPUFA and EVOO may act in synergy to achieve these changes, which might be associated with PPAR-α and Nrf-2 activation and SREBP-1c and NF-κB downregulation.
Acknowledgements
The authors are grateful to the Chilean Endocrinology and Diabetes Society (SOCHED 2013-04 PROJECT to R. V.) and grant (11140174) from Initiation FONDECYT (National Fund for Scientific and Technological Development) to R. V. for supporting this study. Finally, the Enlaza-Mundos Program of the Mayor of Medellin (Colombia) – Agency for Higher Education of Medellin-SAPIENCIA, for the support to co-finance postgraduate study abroad (Maria Catalina Hernandez-Rodas).
References
- L. Abenavoli, N. Milic, V. Peta, F. Alfieri, A. De Lorenzo and S. Bellentani, World J. Gastroenterol., 2014, 20, 16831–16840 CrossRef CAS PubMed.
- P. Almeda-Valdes, N. Aguilar-Olivos, M. Uribe and N. Mendez-Sanchez, Rev. Recent Clin. Trials, 2014, 3, 148–158 Search PubMed.
- R. Karbasi-Afshar, A. Saburi and H. Khedmat, J. Tehran Univ. Heart Cent., 2014, 12, 1–8 Search PubMed.
- L. A. Videla, R. Rodrigo, M. Orellana, V. Fernández, G. Tapia, L. Quiñones, N. Varela, J. Contreras, R. Lazarte, A. Csendes, J. Rojas, F. Maluenda, P. Burdiles, J. C. Díaz, G. Smok, L. Thielemann and J. Poniachik, Clin. Sci., 2004, 106, 261–268 CrossRef CAS PubMed.
- R. Valenzuela, A. Espinosa, D. González-Mañán, A. D'Espessailles, V. Fernández, L. A. Videla and G. Tapia, PLoS One, 2012, 7, e46400 CAS.
- J. Araya, R. Rodrigo, L. A. Videla, L. Thielemann, M. Orellana, P. Pettinelli and J. Poniachik, Clin. Sci., 2004, 106, 635–643 CrossRef CAS PubMed.
- J. Araya, R. Rodrigo, P. Pettinelli, A. V. Araya, J. Poniachik and L. A. Videla, Obesity, 2010, 18, 1460–1463 CrossRef CAS PubMed.
- R. Valenzuela, C. Barrera, A. Espinoza, P. Llanos, P. Orellana and L. A. Videla, Prostaglandins Leukotrienes Essent. Fatty Acids, 2015, 98, 7–14 CrossRef CAS PubMed.
- K. L. Milner, D. van der Poorten, A. Xu, E. Bugianesi, J. G. Kench, K. S. Lam, D. J. Chisholm and J. George, Hepatology, 2009, 49, 1926–1934 CrossRef CAS PubMed.
- Y. Kamari, A. Shaish, E. Vax, S. Shemesh, M. Kandel-Kfir, Y. Arbel, S. Olteanu, I. Barshack, S. Dotan, E. Voronov, C. A. Dinarello, R. N. Apte and D. Harats, J. Hepatol., 2011, 55, 1086–1094 CrossRef CAS PubMed.
- T. C. Lee, P. Ivester, A. G. Hester, S. Sergeant, L. D. Case, T. Morgan, E. O. Kouba and F. H. Chilton, Lipids Health Dis., 2014, 13, 196 CrossRef PubMed.
- S. Lorente-Cebrian, A. G. Costa, S. Navas-Carretero, M. Zabala, J. A. Martinez and M. J. Moreno-Aliaga, J. Physiol. Biochem., 2013, 69, 633–651 CrossRef CAS PubMed.
- Q. Liu, D. Wu, N. Ni, H. Ren, C. Luo, C. He, J. X. Kang, J. B. Wan and H. Su, Mar. Drugs., 2014, 12, 2341–2356 CrossRef PubMed.
- G. Tapia, R. Valenzuela, A. Espinosa, P. Romanque, C. Dossi, D. Gonzalez-Mañán, L. A. Videla and A. D'Espessailles, Mol. Nutr. Food Res., 2014, 58, 1333–1341 CAS.
- S. Cicerale, L. J. Lucas and R. S. Keast, Curr. Opin. Biotechnol., 2012, 23, 129–135 CrossRef CAS PubMed.
- S. Cicerale, X. A. Conlan, A. J. Sinclair and R. S. Keast, Crit. Rev. Food Sci. Nutr., 2009, 49, 218–236 CrossRef CAS PubMed.
- M. I. Covas, K. Nyyssönen, H. E. Poulsen, J. Kaikkonen, H. J. Zunft, H. Kiesewetter, A. Gaddi, R. de la Torre, J. Mursu, H. Bäumler, S. Nascetti, J. T. Salonen, M. Fitó, J. Virtanen, J. Marrug; EUROLIVE and S. Group, Ann. Intern. Med., 2006, 145, 333–341 CrossRef CAS.
- E. Scoditti, C. Capurso, A. Capurso and M. Massaro, Vasc. Pharmacol., 2014, 63, 127–134 CrossRef CAS PubMed.
- S. Piro, L. Spadaro, M. Russello, D. Spampinato, C. E. Oliveri, E. Vasquez, R. Benigno, F. Brancato, F. Purrello and A. M. Rabuazzo, Nutr., Metab. Cardiovasc. Dis., 2008, 18, 545–552 CrossRef CAS PubMed.
- T. T. Tran, Y. Yamamoto, S. Gesta and C. R. Kahn, Cell Metab., 2008, 7, 410–420 CrossRef CAS PubMed.
- D. R. Matthews, J. P. Hosker, A. S. Rudenski, B. A. Naylor, D. F. Treacher and R. C. Turner, Diabetologia, 1985, 28, 412–419 CrossRef CAS.
- E. G. Bligh and W. J. Dyer, Can. J. Biochem. Physiol., 1959, 37, 911–917 CrossRef CAS.
- I. Rahman, A. Kode and S. K. Biswas, Nat. Protoc., 2006, 1, 3159–3165 CrossRef CAS PubMed.
- R. Zimmermann, G. Haemmerle, E. M. Wagner, J. G. Strauss, D. Kratkya and R. Zechner, J. Lipid Res., 2003, 44, 2089–2099 CrossRef CAS PubMed.
- A. P. Halestrap and R. M. Denton, Biochem. J., 1973, 132, 509–517 CrossRef CAS.
- H. Karlic, S. Lohninger, T. Koeck and A. Lohninger, J. Histochem. Cytochem., 2002, 50, 205–212 CrossRef CAS PubMed.
- W. R. Morrison and L. M. Smith, J. Lipid Res., 1964, 5, 600–608 CAS.
- M. E. D'Alessandro, D. Selenscig, P. Illesca, A. Chicco and Y. B. Lombardo, Food Funct., 2015, 6, 1299–1309 Search PubMed.
- M. S. Wiedemann, S. Wueest, F. Item, E. J. Schoenle and D. Konrad, Am. J. Physiol.: Endocrinol. Metab., 2013, 305, E388–E395 CrossRef CAS PubMed.
- H. Poudyal, S. K. Panchal, V. Diwan and L. Brown, Prog. Lipid Res., 2011, 50, 372–387 CrossRef CAS PubMed.
- J. Zúñiga, M. Cancino, F. Medina, P. Varela, R. Vargas, G. Tapia, L. A. Videla and V. Fernández, PLoS One, 2011, 6, e28502 Search PubMed.
- M. Bhaswant, H. Poudyal and L. Brown, J. Nutr. Biochem., 2015, 26, 571–584 CrossRef CAS PubMed.
- F. Violi, L. Loffredo, P. Pignatelli, F. Angelico, S. Bartimoccia, C. Nocella, R. Cangemi, A. Petruccioli, R. Monticolo, D. Pastori and R. Carnevale, Nutr. Diabetes, 2015, 5, e172 CrossRef CAS PubMed.
- S. Farnetti, N. Malandrino, D. Luciani, G. Gasbarrini and E. Capristo, J. Med. Food, 2011, 14, 316–321 CrossRef CAS PubMed.
- C. N. Serhan, J. Dalli, R. A. Colas, J. W. Winkler and N. Chiang, Biochim. Biophys. Acta, 2015, 1851, 397–413 CrossRef CAS PubMed.
- I. Tatsuno, Y. Saito, K. Kudou and J. Ootake, J. Clin. Lipidol., 2013, 7, 199–207 CrossRef PubMed.
- B. De Roos, Y. Mavrommatis and I. A. Brouwer, Br. J. Pharmacol., 2009, 158, 413–428 CrossRef CAS PubMed.
- M. L. Pall and S. Levine, Sheng Li Xue Bao, 2015, 25, 1–18 Search PubMed.
- L. Gao, J. Wang, K. R. Sekhar, H. Yin, N. F. Yared, S. N. Schneider, S. Sasi, T. P. Dalton, M. E. Anderson, J. Y. Chan, J. D. Morrow and M. L. Freeman, J. Biol. Chem., 2007, 282, 2529–2537 CrossRef CAS PubMed.
- P. Priore, A. Cavallo, A. Gnoni, F. Damiano, G. V. Gnoni and L. Siculella, IUBMB Life, 2015, 67, 9–17 CrossRef CAS PubMed.
- O. Hussein, M. Grosovski, E. Lasri, S. Svalb, U. Ravid and N. Assy, World J. Gastroenterol., 2007, 13, 361–368 CrossRef CAS.
- H. Jemai, A. El Feki and S. Sayadi, J. Agric. Food Chem., 2009, 57, 8798–8804 CrossRef CAS PubMed.
- S. Park, Y. Choi, S. J. Um, S. K. Yoon and T. Park, J. Hepatol., 2011, 54, 984–993 CrossRef CAS PubMed.
- W. Hur, S. W. Kim, Y. K. Lee, J. E. Choi, S. W. Hong, M. J. Song, S. H. Bae, T. Park, S. J. Um and S. K. Yoon, Nutr. Res., 2012, 32, 778–786 CrossRef CAS PubMed.
- E. Scorletti, L. Bhatia, K. G. McCormick, G. F. Clough, K. Nash, P. C. Calder, C. D. Byrne and WELCOME Trial Investigators, Contemp. Clin. Trials, 2014, 37, 301–311 CrossRef CAS PubMed.
- M. L. Burr, F. D. Dunstan and C. H. George, J. Membr. Biol., 2005, 206, 155–163 CrossRef CAS PubMed.
- C. Razquin, J. A. Martinez, M. A. Martinez-Gonzalez, M. T. Mitjavila, R. Estruch and A. Marti, Eur. J. Clin. Nutr., 2009, 63, 1387–1393 CrossRef CAS PubMed.
- F. Sofi, I. Giangrandi, F. Cesari, I. Corsani, R. Abbate, G. F. Gensini and A. Casini, Int. J. Food Sci. Nutr., 2010, 61, 792–802 CrossRef CAS PubMed.
- H. Haeiwa, T. Fujita, Y. Saitoh and N. Miwa, Mol. Cell. Biochem., 2014, 386, 73–83 CrossRef CAS PubMed.
- J. H. Lim, Z. Gerhart-Hines, J. E. Dominy, Y. Lee, S. Kim, M. Tabata, Y. K. Xiang and P. Puigserver, J. Biol. Chem., 2013, 288, 7117–7126 CrossRef CAS PubMed.
|
This journal is © The Royal Society of Chemistry 2016 |