DOI:
10.1039/C5FO00918A
(Review Article)
Food Funct., 2016,
7, 46-57
Carboxymethyl-lysine: thirty years of investigation in the field of AGE formation
Received
27th July 2015
, Accepted 22nd September 2015
First published on 25th September 2015
Abstract
In 1985 carboxymethyl-lysine (CML), the first glycoxidation product, was discovered by Dr Ahmed while trying to identify the major products formed in reactions of glucose with lysine under physiological conditions. From that moment, a significant number of researchers have joined efforts to study its formation routes both in foods and in living beings, and the possibility of the existence of an additive action between food-occurring and in vivo produced CML and to explore all the implications associated with its appearance in the biological systems, regardless of its origin. This review presents interesting information on the latest advances in the research on CML sources, mitigation strategies, intake, metabolism and body fluid and tissue delivery, its possible in vivo synergy with highly modified advanced glycation end products-protein, and the physio-pathological implications derived from the presence of this compound in body fluids and tissues.
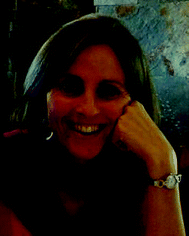 Delgado-Andrade | Dr Delgado-Andrade is a scientific researcher from the Spanish National Research Council working in the field of health–diet interactions since 1997. Her major interest is in understanding the connection of food processing contaminants and the progress and development of degenerative disorders such as inflammatory based pathologies, osteoporosis or musculoskeletal illness. Owing to a multidisciplinary training acquired in Spanish and European laboratories, she is currently involved in different scientific projects in collaboration with important international researchers and institutions. She also carries out advisory work for the food industry. |
Introduction
The Maillard reaction (MR) occurs as a consequence of the thermal treatment of foods and ingredients and is responsible for the production of a large group of heterogeneous substances that definitively influence the acceptance of the final food product.1 The Western diet, rich in thermally processed foods, provides an abundant daily MR product (MRP) intake,2 and bakery products are one of the major sources of amino acid derivatives of MR in conventional diets.3 Maillard like-reactions also occur in living organisms and the products generated in vivo are named advanced glycation end products (AGE) and advanced lipoxidation end products (ALE).4
Particularly, carboxymethyl-lysine (CML) is a well-characterized AGE or ALE owing to its double route of formation that can be produced in both foods and biological systems. It was the first glycoxidation product discovered, a task carried out in 1985 by Dr Ahmed while trying to identify the major products formed in reactions of glucose with lysine under physiological conditions.5 A few years later, the compound was also detected at high levels in thermally processed foods containing milk derivatives and ascorbate.6 After these findings, other researchers shortly demonstrated its presence in lens proteins7 and skin collagen.8 Among the multiple routes of CML formation well-described in the scientific literature (Fig. 1),9 the major ones are the oxidation of fructosyl-lysine (an Amadori product) producing CML by the AGE path; and the direct reaction of glyoxal, produced during lipid peroxidation, with the ε-amino group of lysine, leading to CML by the ALE path.10–12 Probably its multiple formation pathways make CML one of the MR compounds with greater occurrence in foods and in vivo, and hence its suitability as a marker of AGE accumulation in foods and in animal and clinical studies. Although there is still limited evidence on the pathogenic action of CML, its multiple roles and functions have been observed: it is considered a biomarker of aging and of glycoxidative, lipoxidative, oxidative and carbonyl stress; it has been identified as a major AGE-epitope and RAGE (specific receptor for AGE) ligand; and there are some pieces of evidence on its certain ability to behave as a metal chelator. The scientific interest in these compounds lies in the recent discovery of the involvement of highly modified AGE-proteins in an increasing number of degenerative disorders, such as cardiovascular or neurodegenerative pathologies, diabetes complications or bone and cartilage diseases.9,13,14
 |
| Fig. 1 Proposed routes for CML formation as described by Thorpe & Baynes.9 | |
On the other hand, in the last decade many researchers have pointed to the interesting matter of the possible bioactivity of dietary AGE, particularly CML. In this sense, it has been shown that an in vivo synergistic action of AGE from dietary and endogenous origin may occur that could promote a systemic glycoxidant burden, oxidant stress and cell activation, increasing the vulnerability of target tissues to injury and producing various harmful effects to health.15 However, it must be pointed out that some of those studies were performed using an immunochemical method for AGE determination and there is uncertainty about the specificity of the antibody used as well as certain doubts about the linearity of the response when a wide range of AGE concentrations are tested.16 For these reasons, currently many studies are focused on unravelling the possible bioactivity of dietary AGE and their involvement in the production of AGE-associated diseases.
This review shows interesting information on the latest advances in the research on CML sources, mitigation in foods, intake, metabolism and body fluid and tissue delivery, its possible in vivo synergy with highly modified AGE-proteins and the physio-pathological implications derived from the presence of this compound in body fluids and tissues.
Dietary sources and exposure to CML
Since foods are often exposed to cooking or other thermal processes in order to improve their safety and sensory profiles, MRP are part of our daily diet. Moreover, new food habits are introducing important amounts of these products, mainly derived from bakery products and coffee.17 In the particular case of CML occurrence in foods, beside the databases created by Goldberg et al.18 and Uribarri et al.19 one of the more modern studies has been developed by Hull et al.12 in 257 food items as part of the Western-style diet. Food categories considered were meat and fish, cereal-derived products, dairy products, coffee, soups and sauces, and the group of fruit and vegetables, cooked or thermally treated to some extent. As previously documented by other authors,10,20,21 dairy products contained the highest CML levels compared with other food categories, but it is worthy of mention that the CML amount detected in dairy products in the study by Hull et al. was surprisingly abundant reaching a mean value of 514.4 mg per 100 g protein. The huge variety of ingredients and processing conditions of the dairy products included in this study would justify the large variations in the CML level within this food category. Meanwhile, the cereal-derived group also exhibited important CML concentrations, with a value of 28.1 and 17.8 mg per 100 g protein for cereals and bread and savoury biscuits respectively; as well as the sweets and snacks category (34.0 mg per 100 g protein). In contrast, low levels were detected in the meat and fish group and in the fruit and vegetable group (4.5 and 2.7 mg per 100 g protein, respectively). Besides providing important data of CML content in foods, Hull et al.12 once again corroborated the incidence of high temperatures applied in processes such as sterilisation, evaporation, drying, roasting, and frying vs. the moderate temperatures used in pasteurisation or boiling in the production of CML in the food matrices.
Despite the growing information available on the CML levels in foods, not too many studies have explored the exposure to CML through the whole diet, probably due to the difficulty of providing and/or monitoring and controlling the diet of a representative group of individuals. In this sense the efforts made by the group of Drs Vlassara, Uribarri and co-workers must be especially mentioned.22–24 For the interest of this review, two additional studies in which the CML daily intake (mg per day) was evaluated, are described in more detail. They are characterized by the fact that the diets were prepared for all the participants within controlled conditions, under the supervision of researchers, and supplied to the participant's home by a catering service. CML daily intake was quantified taking into account food consumption and measuring the CML content of the culinary preparations. For that purpose the daily menus were also collected at the lab to perform the CML analysis using chromatographic methods. The first one is the ICARE study, where authors explored the effects of a high vs. low MRP diet consumed by a group of people aged 18–24 years during four weeks on different indexes of cardiovascular risk and exposure to different Maillard compounds.25 The authors established that the diet more similar to the French standard (high MRP diet), including culinary techniques such as grilling, frying, roasting, baking and containing commercial food preparations led to the consumption of 5.4 mg CML per day, while the diet avoiding these kinds of preparations and products (low MRP diet) supposed a daily CML intake of 2.2 mg. The second study worthy of mention is the one by Seiquer et al.26 who also designed high and low MRP diets based on the needs and preferences of Spanish adolescent boys aged 11–14 years. The MRP rich diet, which in fact was the daily diet of this population, supposed an intake of 11.3 mg CML per day whereas the MRP poor diet led to a consumption of 5.4 mg CML per day. Although data are in the same range of magnitude the differences found between the high MRP diets of both studies25,26 could be due to the food preferences of teenagers, which included more of highly processed foods (hamburgers, pizzas, soft drinks, etc.) despite the fact that they were modulated to adapt to their physiological needs and under health criteria. Due to the evident differences in food habits depending on the age, geographical location and food availability in the area, researchers are becoming aware of the necessity for investigating the specific exposure level of each population to such processing contaminants.
Mitigation of CML formation in foods
As any other food processing contaminant, CML formation in meals can be lessened by two different ways: modifying the food processing parameters with a major impact on the CML genesis (usually heating time, temperature and the type of cooking procedure) or using inhibitors during the culinary preparation, such as those who have demonstrated effectiveness to avoid in vivo CML formation.27
Regarding the cooking conditions, as logical, culinary techniques like frying, toasting or roasting favour CML formation, whereas boiling, steaming or stewing greatly moderate its production. Uribarri et al.19 demonstrated that poached or steamed chicken contained less than one fourth the AGE present in roasted or broiled chicken. In general, in all food categories tested in their study, culinary treatments applying higher temperatures and lower moisture contents led to increase of the AGE presence. In the particular case of cookies, some investigations have been conducted to set the more adequate temperature–time regime during baking in order to avoid, as much as possible, CML formation. It was established that a baking temperature of 180 °C applied for 16 min might be a compromised selection to reduce CML genesis maintaining suitable sensory properties.28
In relation to the use of inhibitors, the potential of some molecules used in medicine for the same aim has been explored. This is the case of some nucleophilic reagents such as pyridoxamine29 or aminoguanidine30 which can react with active dicarbonyls to inhibit CML formation. A recent study has stated that heat-induced AGE formation in olive oil is completely blocked in the presence of aminoguanidine and that the pre-treatment of beef with acidic solutions (marinades) of lemon or vinegar before roasting also decreases the CML formation rate.19 However, in the opinion of some researchers, the ideal candidates should have two important features. The first one refers to its distribution, which must be wide in nature to make the product economically accessible; the second is related to the fact that they might be present in raw foods to be used as preventives while thermal processing is applied.28 With this description it can be deducted that natural antioxidants such as flavonoids and phenolic compounds have good potential as inhibitors of CML production since they scavenge free radicals, one of the most important pathways that promote CML generation in foods. Thus, the flavonoid fraction isolated from the ethanolic extract of the berry of Vaccinium vitisidaea has been shown to inhibit CML formation.31 Mesías et al.32 have demonstrated the antiglycative and carbonyl trapping properties of the water soluble fraction of coffee silverskin. In this case, the compounds responsible for the action are suggested to be not only the phenolic compounds present in this product, especially chlorogenic acid, but also new antioxidants not naturally present in this food matrix, the high molecular weight polymers (melanoidins) formed through the Maillard reaction during the roasting of coffee.
Finally, we must be aware that any strategy undertaken to mitigate the formation of CML in food should always be oriented to satisfy consumer taste, so the addition of new compounds (inhibitors) must not modify the acceptability of the final product. On the other hand, the control of parameters in which traditional cooking takes place can alter the final result. Therefore, mitigating the formation of CML to retain the sensory characteristics of food and its safety is a challenge we have to face in the future.
Bioavailability of CML from the diet, metabolism data and tissue/fluid delivery
Once MRP are ingested in the diet, the gastrointestinal tract represents the first barrier to their penetration into the organism. When the Amadori compounds, the earliest MRP, arrive in the stomach the acidic environment can reverse a fraction of them; thus, it is known that about 50% of the fructoselysine reverts to lysine under acidic conditions.33 However, this is not the case of CML, which is a stable final product of the advanced glycation. After the gastric step, the intestinal barrier filters the compounds that will reach the internal media. Regardless of the exact mechanism, of course several factors will affect the absorption rate of CML. One of them is its solubility after the gastrointestinal digestion, since this is often the first factor determining the bioavailability of a compound.34 On the other hand, the molecular weight35 and the free or protein-bound form of CML in the food play a significant part, probably because the protein-bound form impairs the proteolytic breakdown, leading to a lower absorption rate. In this sense, our own research team recently established that the administration of diets containing complex protein-bound forms of CML coming from bread crust led to a higher rate of CML excretion in faeces than the administration of the simpler CML form present in the LMW fraction (<5 kDa) extracted from that bread crust (40.5 vs. 27.9%, respectively).36 In the same line but using the advanced fluorescent compound pentosidine Förster et al.37 demonstrated its better absorption when administered from coffee brew (free) than when ingested in bakery products (protein-bound). Regarding the absorption mechanism of dietary CML, the exact pathway remains unclear, but free CML is most probably absorbed by simple diffusion rather than transported across the epithelium.38 Nevertheless the absorption of CML as a dipeptide seems to use peptide transporters especially PEPT1.39
Not many clinical studies are available going deeply into absorption, biodistribution and elimination of CML; moreover, data remain inconclusive. In this respect, our investigations derived from the study of healthy adolescents aged 11–14 years fed a diet low or high in AGE, are in line with data of different authors and confirm that CML absorption and faecal excretion are strongly influenced by dietary CML levels; while urinary elimination, although also related to intake, appears to reach a limit or a saturation stage.40 The mentioned study evidenced a greater CML faecal excretion after the consumption of the AGE rich diet and a urinary elimination rate of 24% compared with the 15% of CML elimination when the low AGE diet was administered. In the case of animal studies the information is a bit more abundant. As referred to above, LMW AGE are easier and faster absorbed than protein-bound forms, consequently its metabolic transit and clearance is also favoured by glomerular filtration.4 Thus, the fast renal clearance of free CML after its intravenous perfusion to rats has been demonstrated, with ≈87% of CML detected in urine in 2 h.41 However focusing on the administration of protein-bound CML within the feeding, Somoza et al.42 gave rats doses of 110 of 310 mg per kg body weight of CML-fortified casein for 10 days to find that CML recovery in urinary excretion was only 26 and 29%, respectively. In that context, Fig. 2 shows the CML excretion rates and its total recovery pointed out in different trials developed in our research group. On the one hand, CML transit was investigated after feeding rat diets containing CML from bread crust (BC diet) or its LMW or HMW soluble compounds (LMW and HMW diets, respectively);36 on the other, in a parallel study, a diet containing CML from the glucose–lysine model system was used (GL diet).43 There were two important differences in the design of these assays: (i) the origin of the CML, which was produced within a complex food in the first (bread crust) and in a model system in the second (glucose–lysine), a fact that gives rise to different CML forms; (ii) the amount of CML administered to the animals, which was extreme in the GL diet aimed to force the conditions of the assay (175 vs. 57, 36 and 37 μg per day for GL, BC, LMW and HMW diets, respectively). As a summary of these studies, it can be concluded that more the CML intake, the more the faecal excretion of CML was, with especially high value after feeding the GL diet, presumably motivated by the CML excess offered. These data support the statement established by different authors who have documented the strong correlation between CML intake and its faecal excretion.44,45 The rate of urinary CML elimination was always lower after consumption of any of the experimental diets compared with the control diet (CML consumption: 30 μg per day). At this point our findings are not coincident with those from other studies who have reported good correlation between CML intake and its urinary elimination.25,44 Considering the total CML excretion rate, only in the case of GL group the ingested CML is recovered almost in total (92%), indicating the easier clearance of this form of CML. However, animal fed diets including CML from bread crust or its soluble fractions reached the same values of CML recovery found in the control group or even lower (≤55%). Similar to these results, Alamir et al.46 documented that 60% of the ingested CML from a diet based on extruded protein was eliminated in the faeces and urine of rats.
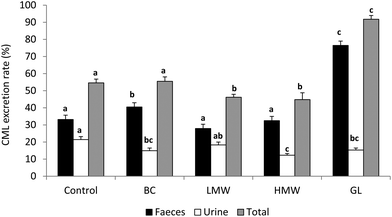 |
| Fig. 2 CML excretion rates (%) in rat fed diets containing CML from bread crust (BC diet), from its soluble LMW and HMW compounds (LWM and HMW diets) or from the glucose–lysine model system (GL diet). Different letters for a parameter (faecal, urine or total recovery) between diets indicate significant differences (one-way Anova followed by Duncan's test to compare means with significant variation, P < 0.05). | |
Different factors could be responsible for the incomplete retrieval of CML: (i) digestive degradation of ingested CML; (ii) biotransformation of the compound within the internal media; or (iii) exceeding the detoxification capacity of the organism, leading to accumulation in different target tissues.36 In this sense, after the intravenous injection of free CML to rats a temporary accumulation in the liver was shown, which for the authors suggested an important affinity of CML to specific hepatic proteins.41 He et al.47 using 14C labelled AGE, observed that after 72 h administration more than the half of the absorbed AGE were found attached to the liver and kidney, and minor amounts bound to the lung, heart and spleen. In the above mentioned trials using bread crust and a heated glucose–lysine model system as CML sources to elaborate animal diets, special accumulation of CML were detected in the cardiac tissue and the tail tendons of rats, but not in the pelvic bone36,43 (Fig. 3). Taking into account that tendons and bones are essentially constituted by collagen type I, this discordant result was a surprise. However, tendon collagen is more susceptible to glycation than bone collagen48 due to the protection provided by the mineral phase of bone against glycation.49 Another important factor to consider is the lower turnover of collagen type I in the tendons and other connective tissues such as cartilage and ligaments compared with bones,50 which could be significant in the accumulation of CML. In the same line, Verzijl et al.51 established that CML levels in human skin and articular collagen were sensibly different as a consequence of the different collagen turnovers in these tissues.
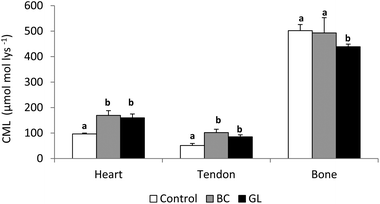 |
| Fig. 3 CML level (μmol per mol Lys) in different tissues of rat fed diets containing CML from bread crust (BC diet) or from the glucose–lysine model system (GL diet). Different letters within a tissue (heart, tendon and bone) indicate significant differences (one-way Anova followed by Duncan's test to compare means with significant variation, P < 0.05). | |
It is now well-established that the intake of dietary AGE provokes an increase in serum AGE. Uribarri et al.52 studied a population of 90 healthy subjects and stated a positive and significant correlation between the level of serum CML and the AGE intake. They concluded that dietary AGE, which are abundantly present in the Western-diet, contribute significantly to the body's pool of AGE. Accordingly, Somoza et al.42 evidenced that the dietary administration of casein-linked CML for 10 days to rats increased the endogenous CML load in plasma and several tissues. In the animal studies developed in our facilities working with bread crust33 and heated glucose–lysine model system43 as sources of AGE in the diets protein-bound CML in serum resulted unmodified. The trial developed by Alamir et al.,46 in which rats were fed a diet based on crushed extruded biscuits, the protein-bound CML in plasma was also stable, however the free CML increased with the consumption of this diet. Therefore, the measurement of that free fraction seems to be more informative aimed to investigate the effects of dietary AGE on circulating CML and it could be a potential index of the CML with dietary origin.
In the organism the total pool of circulating AGE is the sum of those with dietary origin, including AGE absorbed as such products as well as those formed in vivo from the absorbed dicarbonyl compounds which can act as seeds for AGE formation;53 and those endogenously formed. The endogenous fraction includes AGE formed on plasma protein, AGE bound to peptides released by extracellular proteolysis from tissue-immobilized AGE and free adducts formed by the proteolytic degradation of intracellular proteins.54 An interesting observation in recent years is the speculation that an in vivo synergistic action of AGE from different sources (dietary and endogenous) could occur,55 and then promote a systemic glycoxidant burden, oxidant stress and cell activation, increasing the vulnerability of target tissues to injury15 and producing various harmful effects on health. Throughout this review different arguments in support of this theory will be provided; although, in the opinion of some authors we still have patchy information on dietary AGE, knowledge gaps and questions to answer before a conclusion can be drawn.16
Evidence of the involvement of CML in physiological/physio-pathological events
In this section the contribution of CML to the development and/or progress of different physiological/physio-pathological events is considered whatever its origin in the organism, dietary or endogenous. In some studies the contribution of the dietary CML has been widely described due to the development of trials under AGE administration; whereas some others have been focused on detection of endogenously formed CML and its linkage with the pathogenesis of the disorder. Sometimes the synergic action of dietary and in vivo formed CML is suggested.
Several mechanisms have been described by which CML, as any other AGE, can cause tissue damage. These include: (i) modification of protein function due to alterations in its structure/conformation; (ii) changes in the tissue itself as a result of inter and intramolecular crosslinking: (iii) promoting the formation of free radicals; (iv) inducing inflammatory response after binding to specific receptors (RAGE) or other cell membrane receptors, the activity of which increases in proportion to the AGE concentration.56 The AGE–RAGE interaction modulates the pro-inflammatory pathways with transcriptional activation and alters the expression of several inflammatory mediators, such as cytokines, triggering inflammatory reactions.57 This is precisely the hypothesis on which it rests the adverse effect attributed to dietary CML, presumably a RAGE ligand that induces the increase in the inflammatory markers in plasma as well as the oxidative stress.16
Common biological processes with CML participation
The number of biological processes in which CML is known to be involved is increasing in parallel with our knowledge in biochemistry, and particularly with the understanding of some degenerative disorders. Currently it is well-known that this is a molecule, as many others in the biological systems, which is with us from the beginning to the end of our life. Interestingly, Dittrich et al.58 studied the influence of the delivery mode on the infant's CML levels in the first 3 days after birth, establishing that in vaginally delivered neonates urinary CML levels were 2-fold higher compared to neonates delivered by caesarean section (1306 vs. 601 ng mL−1) despite the formula feeding of the former ones. Authors concluded that during the first days after birth, urinary CML excretion rather mirrors endogenous oxidative stress than nutritional uptake and that oxidative stress must be necessarily higher during childbirth labour than during caesarean.
The physiological aging process implies the formation of CML, among other AGE, at the intra and extracellular level. In particular, non-enzymatic glycation of collagen is closely involved in the external image associated with aging, since it causes the tissue crosslinking by the formation of intermolecular bridges leading to hindered turnover and conducting to rigidity and lack of elasticity in the skin.59 The concentrations of CML and pentosidine have been found to increase significantly with age in skin collagen of rats when it was measured for 25 months.60 As another circumstance linked to aging it has also been described as the way in which the human lens accumulates a number of postsynthetic protein modifications during the lifetime, predisposing the crystalline toward glycation, aggregation and cataractogenesis.61
Apart from these normal biological events, for the moment, scientific evidence points to the statistical associations between CML accumulation in human tissues and multiorgan disorders such as cardiovascular and renal diseases or sarcopenia; but a proven causal relationship between CML and human disease has not been established despite extensive experimental evidence of such association. For example, Cai et al.,62 after feeding wild type mice methylglyoxal-modified BSA (MG-BSA) as a source of AGE precursor in the diet for 6 months, reported a causal relationship between the MG-BSA consumption and the presence of higher levels of oxidative stress markers. In parallel, insulin resistance was established in four generations of mice consuming the mentioned diet.63
The important association between diabetes and AGE concerns the fact that hyperglycaemia heightens the glycation process, in particular in the insulin-independent tissues, where there is no efficient restriction to the cellular entry of glucose, such as red blood cells, endothelial cells, peripheral nerve tissue cells, eye lens cells and kidney cells. Consequently these tissues are predisposed to raised levels of glycation of intracellular proteins.64 Moreover, in diabetes, the removal of aged or damaged proteins is impaired since the enzymes involved may also be glycated, reducing the efficiency of protein catabolism and producing a delayed or reduced protein turnover and eventually resulting in CML accumulation.65 Hofmann et al.,66 in a trial using insulin resistant db/db mice (model of type 2 diabetes) fed for 20 weeks with either a high or a low-AGE diet, demonstrated that diabetes progression is aggravated by the level of dietary AGE. The AGE-rich diet induced two-fold higher levels of serum CML and methylglyoxal concentrations and increased body weight gain. In parallel, diabetes pathology was accelerated in animals fed the AGE-rich diet, as demonstrated by a higher fasting insulin concentration, lower glucose and insulin tolerance and a trend to increased proteinuria. Similarly, Zheng et al.67 demonstrated that a high-AGE diet promoted the faster apparition of diabetes-associated complications such as the increased deposit of CML (or total AGE) in the kidney and the implementation of diabetic nephropathy, glomerular hypertrophy and/or mesangial damage. Moreover, Ghanem et al.68 have recently established the association between diabetic retinopathy and levels of pentosidine and CML in aqueous humour and serum of patients, concluding that both can be suitable as biochemical markers of glycoxidation and associated with onset or progression of diabetic retinopathy.
The involvement of AGE in cardiovascular diseases seems to be related to increased crosslinking in the collagen in blood vessels by the glycation of free amino acids. This phenomena, which may occur in diabetic patients and can also be exacerbated by a high-AGE diet, can provoke arterial stiffening, myocardial relaxation abnormalities, the formation of atherosclerotic plaque and endothelial dysfunction.13 The chemical change of low-density lipoproteins (LDL) is another mechanism by which in vivo and exogenous AGE may induce cardiovascular injury. Bucala et al.69 demonstrated that after glycation, LDL becomes susceptible to crosslinking with the collagen in blood vessels, so that LDL is not taken up by the cell and accumulates in the arterial wall. This is the first step for attracting macrophages, followed by the formation of foam cells and the subsequent appearance of the atheroma plaque. In accordance with this, the level of CML in morphologically normal as well as atherosclerotic vessel walls of foetal, juvenile and young adult origin was measured in the study by Nerlich and Schleicher.70 The data suggested that CML accumulated slowly in normal arterial walls while age advances, but this process was significantly accelerated in atherosclerosis. An additional factor to consider within the context of this pathology is the fact that some studies have pointed that CML exposure might deplete the activity of nitric oxide, an important vasodilator produced in the endothelium of vessels by affecting its normal synthesis.71 Despite the information relating to CML and cardiovascular disease, no clear evidence seems to exist that the AGE–RAGE axis has a major influence on essential hypertension.72
Finally, it is worthy of mention that CML has been proposed to act as a chelator and an activator of redox-active metal-ions, such as iron and copper, thus promoting the tissue damage not only in the atherosclerotic process, but also in diabetes, uraemia and other diseases. Due to the conformation of the CML-modified protein the multivalent chelation of metal ions is unlikely to occur; however, it can be hypothesised that a possible collaboration between CML and other amino acids in proteins exists to trap and activate metal ions.9,73 In this sense, Cameron and Cotter74 showed the efficiency of chelation therapy against the appearance of peripheral vascular disease in diabetic rats.
Among the scant studies reporting the effects of the consumption of an AGE-rich diet on cardiovascular risk, the ICARE project evidenced that the healthy volunteers consuming this diet during 4 weeks raised their values of HDL total cholesterol, triglycerides, fasting insulinaemia and HOMA index (Homeostasis Model Assessment), and a correlation between CML levels and the lipid fraction in plasma was established.25
More recent revelations on the involvement of CML in other disorders
As mentioned, in vivo formation of AGE/ALE and its precursors are augmented in processes developed under hyperglycemia, hyperlipidemia and enhanced oxidative stress, a typical metabolic profile in obesity and obesity-related complications. The glyoxalase defense system against AGE/ALE is suppressed in this pathology and this contributes to further increased AGE/ALE formation. Moreover, these products induce the dysregulation of adipokine secretion and can provoke insulin resistance in cultured adipocytes.75 A few years ago, Gaens et al.76 demonstrated the CML accumulation in fatty livers of obese patients, and that the concentration progressively increased with the steatosis grade. Likewise, bigger CML deposits were observed in skeletal muscles of obese patients, a fact which was associated with weight gain.77 Despite the higher CML concentration detected in different tissues in obesity, plasmatic CML seems to be decreased. Thus, Sebekova et al.78 found significantly lower CML plasma levels in obese children compared to lean controls. Curiously, it has been recently described that there is an inverse relationship between circulating CML and the fat mass,79 suggesting that widening of adipose tissue mass in obesity contributes to decreased CML plasma levels. The investigations in the field point that RAGE could play a role in this local accumulation of AGE in tissues in obesity and at the same time decrease AGE plasma levels.80 Conversely to these findings, recently Uribarri et al.81 have evidenced that serum CML increased in obese subjects with a metabolic syndrome profile.
Recent investigations have unraveled the contribution of AGE and oxidative stress in the development of nonalcoholic hepatopathies. To act intracellularly CML needs to bind several receptors and ligand proteins such as RAGE, among others. The role of the axis ligand/RAGE in the hepatic diseases was investigated by Brownlee82 both in in vitro and in vivo trials, reporting that this interaction is a key factor in the activation of intracellular signaling and the subsequent cytokine production associated with inflammation processes or tissue regeneration. In this sense, Gaens et al.,76 using in vitro cultured hepatocytes, demonstrated that the apparition of steatosis was linked to CML accumulation and formation within these cells, and attributed the compound a pro-inflammatory action through increased RAGE expression. On the other hand, the work by Patel et al.83 established that focal hepatic inflammation was induced in 26-week old rats fed for 13 weeks a high AGE-diet whereas a regular diet did not promote any hepatic change. This study is consistent with the hypothesis that dietary AGE can initiate oxidative stress through the activation of AGE receptors expressed in liver cells. However, some recent research developed in adult rats fed on bread crust or a heated glucose–lysine model system as the AGE source in the diet has evidenced that the status of the antioxidant defense in this organ improved84 or was unaffected.85
Thus, although there is still much effort required in this field, an important body of investigations highlight that CML might interact with specific hepatocyte receptors and lead to chain reactions that increase intracellular oxidative stress and induce liver inflammation. The accumulative effects of such events combined with genetic predisposition and environmental conditions can contribute to the progression of a stage of hepatic dysfunction, a possible situation in obesity and diabetes.86
Some neurodegenerative sicknesses, such as Parkinson's and Alzheimer's disease where neuronal loss can exceed 90% in the vulnerable neuronal population, seem to involve AGE in their development since they are produced in the oxidative stress associated with these chronic neurodegenerative processes. The brain has been shown to possess relatively low antioxidant mechanisms, which makes this tissue especially vulnerable to oxidative damage87 and then to AGE formation, with the singularity that neo-formed AGE can induce their own production in a positive feedback loop that intensify the oxidative damage.88 In the case of Alzheimer's disease, a recent study reported that AGE can induce the hyperphosphorylation of the tau protein, one of the major constituents of the neurofibrillary tangles (a common primary marker of Alzheimer's), impairing synapse and memory functions in rats.89 Moreira et al.90 reported that CML along with its glycation-specific precursor hexitol-lysine, was increased in neurons from subjects affected by Alzheimer's disease. On the other hand, consistent with the before mentioned hypothesis of CML as a chelator and an activator of redox-active metal-ions,9,73 significant rises in the chelatable iron in Alzheimer's plaque have been detected.91 The increase in the level of AGE and RAGE in the frontal cortex of Parkinson's disease patients compared to healthy population has been documented,92 as well as the fact that crosslinking phenomena leading to AGE formation contribute in the genesis of aggregates and deposits of certain proteins directly related to the pathogenesis of this disorder.93 On the other hand, Cai et al.94 have demonstrated that a dementia like syndrome can be induced in mice through a diet rich in methyl-glyoxal derivatives, compounds which are important AGE precursors.
Information on the relationship between circulating CML-AGE and cancer risk is emerging. As an example, recently Jiao et al.95 have evaluated the connection between CML consumption and pancreatic cancer establishing that dietary CML intake is associated with modestly increased risk of pancreatic cancer in men, which may partially explain the known positive association between red meat and pancreatic cancer.
In recent years there is a growing interest in the knowledge on the involvement of AGE in cartilage and bone pathologies such as osteoarthritis (OA) and rheumatoid arthritis (RA), where AGE represent new epitopes and contain new antigenic structures, possibly contributing to the generation of the typical autoimmune response of RA. The study of Drinda et al.96 showed that CML was raised in the synovial tissue of patients affected by RA as well as in the control subjects with OA, demonstrating that the presence of this AGE in higher concentration is not a specific phenomenon of RA and is not restricted to those diseases with autoimmune pathways.
Finally, several studies have suggested that AGE may affect the quality of bone via impaired bone remodelling, thus contributing to the development of osteoporosis. In this sense, significantly higher levels of pentosidine and CML in the serum of patients with this disorder has been reported.97 Furthermore, a recent investigation has stated a statistically significant prospective association of CML circulating levels with the incidence of hip fracture which was independent of the bone mass density.98 Meanwhile, AGE accumulation in the bone induces a negative effect on biomechanical properties, decreasing its toughness, increasing its stiffness and therefore contributing to skeletal fragility.99 Our own research group has carried out experiments to analyse the effect of three months’ consumption of AGE from the heated glucose–lysine model system or from bread crust on bone metabolism and mineralization, as well as on aspects of its mechanical behaviour.100,101 Physical parameters of femur weight, length and density were unaffected compared with the control group, but femur volume decreased after consumption of bread crust AGE. The mechanical tests assessed on the tibia bone showed a trend to reduce failure load after AGE consumption, as well as a 40% decrease in the energy to failure. The study of the mechanical properties of the tendon tail of these animals stated that diets rich in AGE led to stiffer tendons. When data were included in a finite element model to analyse stress–strain distribution in a muscle-tendon unit (tibialis anterior) higher stress and strain in the muscle were detected after AGE consumption, which could imply possible pain in joint mobility.102
In summary, our knowledge on CML formation in food and in vivo has largely increased since it was detected in 1985 for the first time, but the interaction of dietary AGE-biological effects, the importance of the AGE–RAGE axis or the role of AGE's pool in the common physiological processes during life is still far from being understood in all angles. The progress at the molecular level in understanding the physio-pathological processes accompanying the advance of AGE-related diseases is a key factor. From our present knowledge, what can be stated is that CML, regardless of its origin, is clearly involved in inflammation and oxidative stress mediated processes. Researchers dedicated to Nutrition and Food Science as well as pertinent Sanitary Authorities are required to continue urging the consumers about the benefits of maintaining a balanced and varied diet, not only in the food consumed but also in the way it is processed to avoid as much as possible the presumable harmful effects of AGE and to take positive actions associated with other Maillard reaction derivatives.
Acknowledgements
This work was supported by a “Ramón y Cajal” postdoctoral contract of the Spanish Ministry of Economy and Competitiveness.
References
- C. Delgado-Andrade, F. J. Morales, I. Seiquer and M. P. Navarro, Maillard reaction products profile and intake from Spanish typical dishes, Food Res. Int., 2010, 43, 1304–1311 CrossRef CAS PubMed
.
- H. Vlassara, Advanced glycosylation in nephropathy of diabetes and aging, Adv. Nephrol. Necker Hosp., 1996, 25, 303–307 CAS
.
- T. Henle, AGEs in foods: Do they play a role in uremia?, Kidney Int., 2003, 63, S145–SS47 CrossRef PubMed
.
- M. W. Poulsen, R. V. Hedegaard, J. M. Andersen, B. de Courten, S. Bügel, J. Nielsen, L. H. Skibsted and L. O. Dragsted, Advanced glycation endproducts in food and their effects on health, Food Chem. Toxicol., 2013, 60, 10–37 CrossRef CAS PubMed
.
- M. U. Ahmed, S. R. Thorpe and J. W. Baynes, Identification of Nε-(carboxymethyl)lysine as a degradation product of fructoselysine in glycated protein, J. Biol. Chem., 1986, 261, 4889–4894 CAS
.
- H. F. Erbersdobler, Protein reactions during food processing and storage-their relevance to human nutrition, Bibl. Nutr. Dieta, 1989, 43, 140–155 Search PubMed
.
- J. A. Dunn, J. S. Patrick, S. R. Thorpe and J. W. Baynes, Oxidation of glycated proteins: age-dependent accumulation of Nε-(carboxymethyl)lysine in lens proteins, Biochemistry, 1989, 28, 9464–9468 CrossRef CAS
.
- J. A. Dunn, D. R. McCance, S. R. Thorpe, T. J. Lyons and J. W. Baynes, Age-dependent accumulation of Nε-(carboxymethyl)lysine and Nε-(carboxymethyl)hydroxylysine in human skin collagen, Biochemistry, 1991, 30, 1205–1210 CrossRef CAS
.
- S. R. Thorpe and J. W. Baynes, CML: a brief history, Int. Congr. Ser., 2002, 1245, 91–99 CrossRef CAS
.
- S. H. Assar, C. Moloney, M. Lima, R. Magee and J. M. Ames, Determination of Nε–(carboxymethyl)lysine in food systems by ultra performance liquid chromatography-mass spectrometry, Amino Acids, 2009, 36, 317–326 CrossRef CAS PubMed
.
- C. Niquet-Leridon and F. J. Tessier, Quantification of Nε-carboxymethyl-lysine in selected chocolate-flavoured drink mixes using high-performance liquid chromatography-linear ion trap tandem mass spectrometry, Food Chem., 2011, 126, 655–663 CrossRef CAS PubMed
.
- G. L. J. Hull, J. V. Woodside, J. M. Ames and G. J. Cuskelly, Nε-(carboxymethyl)lysine content of foods commonly consumed in a western style diet, Food Chem., 2012, 131, 170–174 CrossRef CAS PubMed
.
- C. Luevano-Contreras and K. Chapman-Novakofski, Dietary advanced glycation end products and aging, Nutrients, 2010, 2, 1247–1265 CrossRef CAS PubMed
.
- C. Delgado-Andrade, Maillard reaction products: some considerations on their health effects, Clin. Chem. Lab. Med., 2014, 52, 53–60 CrossRef CAS PubMed
.
- W. Cai, J. C. He, L. Zhu, M. Peppa, C. Lu, J. Uribarri and H. Vlassara, High levels of dietary advanced glycation end products transform low-dersity lipoprotein into a potent redox-sensitive mitogen-activated protein kinase stimulant in diabetic patients, Circulation, 2004, 110, 285–291 CrossRef CAS PubMed
.
- J. M. Ames, Evidence against dietary advanced glycation endproducts being a risk to human health, Mol. Nutr. Food Res., 2007, 54, 1085–1090 Search PubMed
.
- F. J. Morales, V. Somoza and V. Fogliano, Physiological relevance of dietary melanoidins, Amino Acids, 2012, 42, 1097–1109 CrossRef CAS PubMed
.
- T. Goldberg, W. Cai, P. Melpomeni, V. Dardaine, B. S. Baliga, J. Uribarri and H. Vlassara, Advanced glycoxidation end products in commonly consumed foods, J. Am. Diet Assoc., 2004, 104, 1287–1291 CrossRef CAS PubMed
.
- J. Uribarri, S. Woodruff, S. Goodman, W. Cai, X. Chen, R. Pyzik, A. Yong, G. E. Striker and H. Vlassara, Advanced glycation end products in foods and a practical guide to their reduction in the diet, J. Am. Diet Assoc., 2010, 110, 911–916 CrossRef PubMed
.
- S. Drusch, V. Faist and H. F. Erbersdobler, Determination of Nε-carboxymethyllysine in milk products by a modified reversed-phase HPLC method, Food Chem., 1999, 65, 547–553 CrossRef CAS
.
- A. Charissou, L. Ait-Ameur and I. Birlouez-Aragon, Evaluation of a gas chromatography/mass spectrometry method for the quantification of carboxymethyllysine in food samples, J. Chromatogr., A, 2007, 1140, 189–194 CrossRef CAS PubMed
.
- H. Vlassara, W. Cai, J. Crandall, T. Goldberg, R. Oberstein, V. Dardaine, M. Peppa and E. J. Rayfield, Inflammatory mediators are induced by dietary glycotoxins, a major risk factor for diabetic angiopathy, Proc. Nat. Acad. Sci. U. S. A., 2002, 99, 15596–15601 CrossRef CAS PubMed
.
- J. Uribarri, M. Peppa, W. Cai, T. Goldberg, M. Lu, C. He and H. Vlassara, Restriction of dietary glycotoxins reduces excessive advanced glycation end products in renal failure patients, J. Am. Soc. Nephrol., 2003, 14, 728–731 CrossRef CAS
.
- J. Uribarri, W. Cai, M. Ramdas, S. Goodman, R. Pyzik, X. Chen, L. Zhu, G. E. Striker and H. Vlassara, Restriction of advanced glycation end products improves insulin resistance in human type 2 diabetes, Diabetes Care, 2011, 34, 1610–1616 CrossRef CAS PubMed
.
- I. Birlouez-Aragon, G. Saavedra, F. J. Tessier, A. Galinier, L. Ait-Ameur, F. Lacoste, C. N. Niamba, N. Alt, V. Somoza and J. M. Lecerf, A diet based on high-heat-treated foods promotes risk factors for diabetes mellitus and cardiovascular diseases, Am. J. Clin. Nutr., 2010, 91, 1220–1226 CrossRef CAS PubMed
.
- I. Seiquer, L. A. Rubio, M. J. Peinado, C. Delgado-Andrade and M. P. Navarro, Maillard reaction products modulate gut microbiota composition in adolescents, Mol. Nutr. Food Res., 2014, 58, 1552–1560 CAS
.
- L. Han, L. Li, B. Li, D. Zhao, Y. Li, Z. Xu and G. Liu, Review of the characteristica of food-derived and endogenous Nε-carboxymethyllysine, J. Food Prot., 2013, 76, 912–918 CrossRef CAS PubMed
.
- L. Cheng, C. Jin and Y. Zhang, Investigation of variations in the acrylamide and Nε-(carboxymethyl) lysinecontents in cookies during baking, J. Food Sci., 2014, 79, T1030–T1038 CrossRef CAS PubMed
.
- V. F. Carvalho, L. T. Florin, E. D. Barreto, R. C. Torres, M. M. Batista, F. C. Amendoeira, R. S. B. Cordeiro, M. A. Martins and P. M. R. E. Silva, Inhibition of advance glycation end products by aminoguanidine restores mast cell numbers and reactivity in alloxan-diabetic rats, Eur. J. Pharmacol., 2001, 669, 143–148 CrossRef PubMed
.
- D. L. Price, P. M. Rhett, S. R. Thorpe and J. W. Baynes, Chelatng activity of advanced end products inhibitors, J. Biol. Chem., 2001, 276, 48967–48972 CrossRef CAS PubMed
.
- L. P. Beaulieu, C. S. Harris, A. Saleem, A. Cuerrier, P. S. Haddad, L. C. Martineau, S. A. L. Bennett and J. T. Arnason, Inhibitory effect of the Cree traditional medicine wiishichimanaanh (Vaccinium vitis-idaea) on advanced glycation endproduct formation: identification of active principles, Phytother. Res., 2010, 24, 741–747 CAS
.
- M. Mesías, M. Navarro, N. Martínez-Saez, M. Ullate, M. D. del Castillo and F. J. Morales, Antiglycative and carbonyl trapping properties of the water soluble fraction of coffee silverskin, Food Res. Int., 2014, 62, 1120–1126 CrossRef PubMed
.
- M. Friedman, Browning ans its prevention, J. Agric. Food Chem., 1996, 44, 631–653 CrossRef CAS
.
-
C. Delgado-Andrade, A. Haro, R. Castellano and J. A. Rufián-Henares, in Assessing the generation and bioactivity of neo-formed compounds in thermally treated foods, ed. C. Delgado-Andrade and J. A. Rufián-Henares, Atrio, Granada, 2009, pp. 51–62 Search PubMed
.
- V. Faist and H. F. Erbersdobler, Metabolic transit and in vivo effects of melanoidins and precursor compounds deriving from the Maillard reaction, Ann. Nutr. Metab., 2001, 45, 1–12 CrossRef CAS PubMed
.
- I. Roncero-Ramos, C. Delgado-Andrade, F. J. Tessier, C. Niquet-Léridon, C. Strauch, V. M. Monnier and M. P. Navarro, Metabolic transit of N(ε)-carboxymethyl-lysine after consumption of AGEs from bread crust, Food Funct., 2013, 4, 1032–1039 CAS
.
- A. Förster, Y. Kühne and T. Henle, Studies on absorption and elimination of dietary Maillard reaction products, Ann. N. Y. Acad. Sci., 2005, 1043, 474–481 CrossRef PubMed
.
- S. Grunwald, R. Krause, M. Bruch, T. Henle and M. Brandsch, Transepithelial flux of early and advanced glycation compounds across Caco-2 cell monolayers and their interaction with intestinal amino acid and peptide transport systems, Br. J. Nutr., 2006, 95, 1221–1228 CrossRef CAS
.
- M. Hellwig, S. Geissler, R. Matthes, A. Peto, C. Silow, M. Brandsch and T. Henle, Transport of free and peptide-bound glycated amino acids: synthesis, transepithelial flux at Caco-2 cell monolayers, and interaction with apical membrane transport proteins, ChemBioChem, 2011, 12, 1270–1279 CrossRef CAS PubMed
.
- C. Delgado-Andrade, F. J. Tessier, C. Niquet-Léridon, I. Seiquer and M. P. Navarro, Study of the urinary and faecal excretion of Nε-carboxymethyllysine in young human volunteers, Amino Acids, 2012, 43, 595–602 CrossRef CAS PubMed
.
- R. Bergmann, R. Helling, C. Heichert, M. Scheunemann, P. Mading, H. Wittrisch, B. Johannsen and T. Henle, Radio fluorination and positron emission tomography (PET) as a new approach to study the in vivo distribution and elimination of the advanced glycation endproducts N-epsiloncarboxymethyllysine (CML) and N-epsilon-carboxyethyllysine (CEL), Nahrung, 2001, 45, 182–188 CrossRef CAS
.
- V. Somoza, E. Wenzel, C. Weiss, I. Clawin-Radecker, N. Grubel and H. F. Erbersdobler, Dose-dependent utilisation of casein-linked lysinoalanine, N(epsilon)-fructoselysine and N(epsilon)-carboxymethyllysine in rats, Mol. Nutr. Food Res., 2006, 50, 833–841 CAS
.
- I. Roncero-Ramos, C. Niquet-Léridon, C. Strauch, V. M. Monnier, F. J. Tessier, M. P. Navarro and C. Delgado-Andrade, An advanced glycation end product (AGE)-rich diet promotes Nε-carboxymethyl-lysine accumulation in the cardiac tissue and tendons of rats, J. Agric. Food Chem., 2014, 62, 6001–6006 CrossRef CAS PubMed
.
- R. Liardon, D. De Weck-Gaudard, G. Philippossian and P. A. Finot, Identification of N.epsilon.-carboxymethyllysine: a new Maillard reaction product in rat urine, J. Agric. Food Chem., 1987, 35, 427–431 CrossRef CAS
.
-
F. J. Tessier, C. Niquet-Léridon, L. Rhazi, K. Hedhili, I. Seiquer, M. P. Navarro and C. Delgado-Andrade, in Maillard Reaction Interface between Aging, Nutrition and Metabolism, ed. M. C. Thomas and J. M. Forbes, RSC Publishing, Cambridge, 2010, vol. 332, pp. 144–150 Search PubMed
.
- I. Alamir, C. Niquet-Leridon, P. Jacolot, C. Rodriguez, M. Orosco, P. M. Anton and F. J. Tessier, Digestibility of extruded proteins and metabolic transit of N (epsilon)-carboxymethyllysine in rats, Amino Acids, 2013, 44, 1441–1449 CrossRef CAS PubMed
.
- C. He, J. Sabol, T. Mitsuhashi and H. Vlassara, Dietary glycotoxins: inhibition of reactive products by aminoguanidine facilitates renal clearance and reduces tissue sequestration, Diabetes, 1999, 48, 1308–1315 CrossRef CAS
.
- D. Vashishth, G. J. Gibson, J. I. Khoury, M. B. Schaffler, J. Kimura and D. P. Fyhrie, Influence of nonenzymatic glycation on biomechanical properties of cortical bone, Bone, 2001, 28, 195–201 CrossRef CAS
.
- R. Eastell, A. Colwell, L. Hampton and J. Reeve, Biochemical markers of bone resorption compared with estimates of bone resorption from radiotracer kinetic studies in osteoporosis, J. Bone Miner. Res., 1997, 12, 59–65 CrossRef CAS PubMed
.
- M. J. Silva, M. D. Brodt, M. A. Lynch, J. A. McKenzie, K. M. Tanouye, J. S. Nyman and X. Wang, Type 1 Diabetes in Young Rats Leads to Progressive Trabecular Bone Loss, Cessation of Cortical Bone Growth, and Diminished Whole Bone Strength and Fatigue Life, J. Bone Miner. Res., 2009, 24, 1618–1627 CrossRef CAS PubMed
.
- N. Verzijl, J. DeGroot, S. R. Thorpe, R. A. Bank, J. N. Shaw, T. J. Lyons, J. W. Bijlsma, F. P. Lafeber, J. W. Baynes and J. M. TeKoppele, Effect of collagen turnover on the accumulation of advanced glycation end products, J. Biol. Chem., 2000, 275, 39027–39031 CrossRef CAS PubMed
.
- J. Uribarri, W. Cai, O. Sandu, M. Peppa, T. Goldberg and H. Vlassara, Diet-derived advanced glycation end products are major contributors to the body's AGE pool and induce inflammation in healthy subjects, Ann. N. Y. Acad. Sci., 2005, 1043, 461–466 CrossRef CAS PubMed
.
- J. Degen, M. Hellwig and T. Henle, 1,2-Dicarbonyl compounds in commonly consumed foods, J. Agric. Food Chem., 2012, 60, 7071–7079 CrossRef CAS PubMed
.
- K. Kaňková and K. Šebeková, Genetic variability in the RAGE gene: Possible implications for nutrigenetics, nutrigenomics, and understanding the susceptibility to diabetic complications, Mol. Nutr. Food Res., 2005, 49, 700–709 Search PubMed
.
- V. Somoza, Five years of research on health risks and benefits of Maillard reaction products: An update, Mol. Nutr. Food Res., 2005, 49, 663–672 CAS
.
- J. Shibao and D. H. M. Bastos, Produtos da reação de Maillard em alimentos: implicações para a saúde, Rev. Nutr., 2011, 24, 895–904 CAS
.
- R. Ramasamy, S. F. Yan and A. M. Schmidt, Receptor for AGE (RAGE): signaling mechanisms in the pathogenesis of diabetes and its complications, Ann. N. Y. Acad. Sci., 2011, 1243, 88–102 CrossRef CAS PubMed
.
- R. Dittrich, I. Hoffmann, P. Stahl, A. Müller, M. W. Beckmann and M. Pischetsrieder, Concentrations of Nepsilon-carboxymethyllysine in human breast milk, infant formulas, and urine of infants, J. Agric. Food Chem., 2006, 54, 6924–6928 CrossRef CAS PubMed
.
-
J. Rodríguez-García, Estudio de distintos marcadores de glicación en las complicaicones crónicas de la diabetes mellitus, PhD Thesis, University of Santiago de Compostela, Spain Search PubMed
.
- W. T. Cefalu, A. D. Bell-Farrow, Z. Q. Wang, W. E. Sonntag, M. X. MX, J. W. Baynes and S. R. Thorpe, Caloric restriction decreases age-dependent accumulation of the glycoxidation products, N epsilon-(carboxymethyl)lysine and pentosidine, in rat skin collagen, J. Gerontol., Ser. A, 1995, 50, B337–B341 CrossRef CAS PubMed
.
- R. J. Truscott, Age-related nuclear cataract-oxidation is the key, Exp. Eye Res., 2005, 80, 709–725 CrossRef CAS PubMed
.
- W. Cai, J. C. He, L. Zhu, X. Chen, F. Zheng, G. E. Striker and H. Vlassara, Oral glycotoxins determine the effects of calorie restriction on oxidant stress, agerelated diseases, and lifespan, Am. J. Pathol., 2008, 173, 327–336 CrossRef CAS PubMed
.
- W. Cai, M. Ramdas, L. Zhu, X. Chen, G. E. Striker and H. Vlassara, Oral advanced glycation endproducts (AGEs) promote insulin resistance and diabetes by depleting the antioxidant defenses AGE receptor-1 and sirtuin 1, Proc. Natl. Acad. Sci. U. S. A., 2012, 109, 15888–15893 CrossRef CAS PubMed
.
- F. J. Tessier, The Maillard reaction in the human body. The main discoveries and factors that affect glycation, Pathol. Biol., 2010, 58, 214–219 CrossRef CAS PubMed
.
- P. E. Morgan, R. T. Dean and M. J. Davies, Inactivation of cellular enzymes by carbonyls and protein-bound glycation/glycoxidation products, Arch. Biochem. Biophys., 2002, 403, 2592–2569 CrossRef
.
- S. M. Hofmann, H.-J. Dong, Z. Li, W. Cai, J. Altomonte, S. N. Thung, F. Zeng, E. A. Fisher and H. Vlassara, Improved insulin sensitivity is associated with restricted intake of dietary glycoxidation products in the db/db mouse, Diabetes, 2002, 51, 2082–2089 CrossRef CAS
.
- F. Zheng, C. He, W. Cai, M. Hattori, M. Steffes and H. Vlassara, Prevention of diabetic nephropathy in mice by a diet low in glycoxidation products, Diabetes/Metab. Res. Rev., 2002, 18, 224–237 CrossRef PubMed
.
- A. A. Ghanem, A. Elewa and L. F. Arafa, Pentosidine and N-carboxymethyl-lysine: biomarkers for type 2 diabetic retinopathy, Eur. J. Ophthalmol., 2011, 21, 48–54 CrossRef
.
- R. Bucala, Z. Makita, G. Vega, S. Grundy, T. Koschinsky, A. Cerami and H. Vlassara, Modification of low density lipoprotein by advanced glycation end products contributes to the dyslipidemia of diabetes and renal insufficiency, Proc. Natl. Acad. Sci. U. S. A., 1994, 91, 9441–9445 CrossRef CAS
.
- A. G. Nerlich and E. D. Schleicher, N(epsilon)-(carboxymethyl)lysine in atherosclerotic vascular lesions as a marker for local oxidative stress, Atherosclerosis, 1999, 144, 41–47 CrossRef CAS
.
- B. Xu, R. Chibber, D. Ruggiero, E. Kohner, J. Ritter and A. Ferro, Impairment of vascular endothelial nitric oxide synthase activity by advanced glycation end products, FASEB J., 2003, 17, 1289–1291 CrossRef CAS PubMed
.
- M. Baumann, Role of advanced glycation end products in hypertension and cardiovascular risk: human studies, J. Am. Soc. Hypertens., 2012, 6, 427–435 CrossRef CAS PubMed
.
- M. Qian and J. W. Eaton, Glycochelates and the etiology of diabetic peripheral neuropathy, Free Radical Biol. Med., 2000, 28, 652–656 CrossRef CAS
.
- N. E. Cameron and M. A. Cotter, Effects of an extracellular metal chelator on neurovascular function in diabetic rats, Diabetologia, 2001, 44, 621–628 CrossRef CAS PubMed
.
- K. H. J. Gaens, C. D. A. Stehouwera and C. G. Schalkwijk, Advanced glycation endproducts and its receptor for advanced glycation endproducts in obesity, Curr. Opin. Lipidol., 2013, 24, 4–11 CrossRef CAS PubMed
.
- K. H. J. Gaens, P. M. Niessen, S. S. Rensen, W. Buurman, J. W. Greve, A. Driessen, M. G. Wolfs, M. H. Hofker, J. G. Bloemen, C. H. Dejong, C. D. Stehouwer and C. G. Schalkwijk, Endogenous formation of Nepsilon-(carboxymethyl)lysine is increased in fatty livers and induces inflammatory markers in an in vitro model of hepatic steatosis, J. Hepatol., 2012, 56, 647–655 CrossRef CAS PubMed
.
- M. P. de la Maza, J. Uribarri, D. Olivares, S. Hirsch, L. Leiva, G. Barrera and D. Bunout, Weight increase is associated with skeletal muscle immunostaining for advanced glycation end products, receptor for advanced glycation end products, and oxidation injury, Rejuvenation Res., 2008, 11, 1041–1048 CrossRef CAS PubMed
.
- K. Sebekova, V. Somoza, M. Jarcuskova, A. Heidland and L. Podracká, Plasma advanced glycation end products are decreased in obese children compared with lean controls, Int. J. Pediatr. Obes., 2009, 4, 112–118 CrossRef PubMed
.
- R. D. Semba, L. Arab, K. Sun, E. J. Nicklett and L. Ferrucci, Fat mass is inversely associated with serum carboxymethyl-lysine, an advanced glycation end product, in adults, J. Nutr., 2011, 141, 1726–1730 CrossRef CAS PubMed
.
- K. M. Myint KM, Y. Yamamoto, T. Doi, I. Kato, A. Harashima, H. Yonekura, T. Watanabe, H. Shinohara, M. Takeuchi, K. Tsuneyama, N. Hashimoto, M. Asano, S. Takasawa, H. Okamoto and H. Yamamoto, RAGE control of diabetic nephropathy in a mouse model: effects of RAGE gene disruption and administration of lowmolecular weight heparin, Diabetes, 2006, 55, 2510–2522 CrossRef PubMed
.
- J. Uribarri, W. Cai, M. Woodward, E. Tripp, L. Goldberg, R. Pyzik, K. Yee, L. Tansman, X. Chen, V. Mani, Z. A. Fayad and H. Vlassara, Elevated serum advanced glycation endproducts in obese indicate risk for the metabolic syndrome: A link between healthy and unhealthy obesity?, J. Clin. Endocrinol. Metab., 2015, 100, 1957–1966 CrossRef CAS PubMed
.
- M. Brownlee, Biochemistry and molecular cell biology of diabetic complications, Nature, 2001, 414, 813–820 CrossRef CAS PubMed
.
- R. Patel, S. S. Baker, W. Liu, S. Desai, R. K. R. Alkhouri, L. Mastrandrea, A. Sarfraz, W. Cai, H. Vlassara, M. S. Patel, R. D. Baker and L. Zhu, Effect of dietary advanced glycation end products on mouse liver, PLoS One, 2012, 7, e35143 CAS
.
- S. Pastoriza, I. Roncero-Ramos, J. A. Rufián-Henares and C. Delgado-Andrade, Antioxidant balance after long-term consumption of standard diets including bread crust glycated compounds by adult rats, Food Res. Int., 2014, 64, 106–113 CrossRef CAS PubMed
.
- S. Pastoriza, J. A. Rufián-Henares and C. Delgado-Andrade, Effects of long-term consumption of standard diets including glucose-lysine model glycated compounds on the antioxidant status of adult rats, Food Chem., 2015, 183, 283–290 CrossRef CAS PubMed
.
- J. C. D. Santos, B. I. B. Valentim, O. R. P. de Araújo, T. R. Ataide and M. O. F. Goulart, Development of Nonalcoholic Hepatopathy: Contributions of Oxidative Stress and Advanced Glycation End Products, Int. J. Mol. Sci., 2013, 14, 19846–19866 CrossRef PubMed
.
- J. B. Schulz, J. Lindenau, J. Seyfried and J. Dichgans, Glutathione, oxidative stress and neurodegeneration, Eur. J. Biochem., 2000, 267, 4904–4911 CrossRef CAS
.
- H. Vicente Miranda and T. F. Outeiro, The sour side of neurodegenerative disorders: the effects of protein glycation, J. Pathol., 2010, 221, 13–25 CrossRef PubMed
.
- X. H. Li, B. L. Lv, J. Z. Xie, J. Liu, X. W. Zhou and J. Z. Wang, AGEs induce Alzheimer-like tau pathology and memory deficit via RAGE-mediated GSK-3 activation, Neurobiol. Aging, 2012, 33, 1400–1410 CrossRef PubMed
.
-
P. I. Moreira, M. A. Smith, X. Zhu, A. Nunomura, R. J. Castellani and G. Perry, in Maillard Reaction: Chemistry at the interface of Nutrition, Aging and Disease, ed. J. W. Baynes, V. M. Monnier, J. M. Ames and S. R. Thorpe, Ann. N. Y. Acad. Sci., 2005, vol. 1043, pp. 545–552 Search PubMed
.
- M. A. Smith, P. L. Harris, L. M. Sayre and G. Perry, Iron accumulation in Alzheimer disease is a source of redoxgenerated free radicals, Proc. Natl. Acad. Sci. U. S. A., 1997, 94, 9866–9868 CrossRef CAS
.
- E. Dalfo, M. Portero-Otin, V. Ayala, A. Martinez, R. Pamplona and I. Ferrer, Evidence of oxidative stress in the neocortex in incidental Lewy body disease, J. Neuropathol. Exp. Neurol., 2005, 64, 816–830 CrossRef CAS
.
- J. Li, D. Liu, L. Sun, Y. Lu and Z. Zhang, Advanced glycation end products and neurodegenerative diseases: Mechanisms and perspective, J. Neurol. Sci., 2012, 317, 1–5 CrossRef CAS PubMed
.
- W. Cai, J. Uribarri, L. Zhu, X. Chen, S. Swamy, Z. Zhao, F. Grosjean, C. Simonaro, G. A. Kuchel, M. Schnaider-Beeri, M. Woodward, G. E. Striker and H. Vlassara, Oral glycotoxins are a modifiable cause of dementia and the metabolic syndrome in mice and humans, Proc. Natl. Acad. Sci. U. S. A., 2014, 111, 4940–4945 CrossRef CAS PubMed
.
- L. Jiao, R. Stolzenberg-Solomon, T. P. Zimmerman, Z. Duan, L. Chen, L. Kahle, A. Risch, A. F. Subar, A. J. Cross, A. Hollenbeck, H. Vlassara, G. Striker and R. Sinha, Dietary consumption of advanced glycation end products and pancreatic cancer in the prospective NIH-AARP Diet and Health Study, Am. J. Clin. Nutr., 2015, 101, 126–134 CrossRef CAS PubMed
.
- S. Drinda, S. Franke, C. C. Canet, P. Petrow, R. Bräuer, C. Hüttich, G. Stein and G. Hein, Identification of the advanced glycation end products N(epsilon)-carboxymethyllysine in the synovial tissue of patients with rheumatoid arthritis, Ann. Rheum. Dis., 2002, 61, 488–492 CrossRef CAS
.
- G. Hein, R. Wiegand, G. Lehmann, G. Stein and S. Franke, Advanced glycation end-products pentosidine and N epsilon-carboxymethyllysine are elevated in serum of patients with osteoporosis, Rheumatology, 2003, 42, 1242–1246 CrossRef CAS PubMed
.
- J. I. Barzilay, P. Bůžková, S. J. Zieman, J. R. Kizer, L. Djoussé, J. H. Ix, R. P. Tracy, D. S. Siscovick, J. A. Cauley and K. J. Mukamal, Circulating levels of carboxy-methyl-lysine (CML) are associated with hip fracture risk: the Cardiovascular Health Study, J. Bone Miner. Res., 2014, 29, 1061–1066 CrossRef CAS PubMed
.
- X. Wang, X. Shen, X. Li and C. M. Agrawal, Age-related changes in the collagen network and toughness of bone, Bone, 2002, 31, 1–7 CrossRef
.
- I. Roncero-Ramos, C. Delgado-Andrade, J. A. Rufián-Henares, J. Carballo and M. P. Navarro, Effects of model Maillard compounds on bone characteristics and functionality, J. Sci. Food Agric., 2013, 93, 2816–2821 CrossRef CAS PubMed
.
- C. Delgado-Andrade, I. Roncero-Ramos, J. Carballo, J. A. Rufián-Henares, I. Seiquer and M. P. Navarro, Composition and functionality of bone affected by dietary glycated compounds, Food Funct., 2013, 4, 549–556 CAS
.
- J. Grasa, B. Calvo, C. Delgado-Andrade and M. P. Navarro, Variations in tendon stiffness due to diets with different glycotoxins affect mechanical properties in the muscle-tendon unit, Ann. Biomed. Eng., 2013, 41, 488–496 CrossRef CAS PubMed
.
|
This journal is © The Royal Society of Chemistry 2016 |
Click here to see how this site uses Cookies. View our privacy policy here.