DOI:
10.1039/C4FO00749B
(Paper)
Food Funct., 2015,
6, 275-285
Enhanced bioavailability and safety of curcumagalactomannosides as a dietary ingredient
Received
19th August 2014
, Accepted 9th November 2014
First published on 10th November 2014
Abstract
In spite of the various bioavailable formulations of curcumin for pharma and dietary supplement applications, food grade formulations suitable as a dietary ingredient, and capable of providing significant levels of plasma curcumin, are limited. The present contribution describes the safety and oral bioavailability of a novel water soluble formulation of curcumin, curcumagalactomannosides (CGM), when used as a dietary ingredient in selected food items. CGM was prepared using a food grade hydrocolloid (galactomannans) derived from the kitchen spice fenugreek (Trigonella foenum graccum), without using any synthetic excipients. The safety of the formulation was assessed through acute and subchronic toxicity studies on Wistar rats and genotoxicity studies. The efficacy of CGM as a bioavailable dietary ingredient was assessed by successfully preparing various food items and by measuring the post-blood plasma curcumin levels at various time intervals after the consumption of food items. High performance liquid chromatography coupled with a photodiode array detector (HPLC-PDA) and electrospray ionization tandem mass spectrometer (ESI-MS/MS) was employed for the quantification of plasma curcuminoids. It was observed that CGM is safe and suitable for further development and clinical studies, with a no observable adverse effect level (NOAEL) up to 2.0 g kg−1 per day b.wt. CGM was found to offer seven to ten times higher bioavailability of curcumin in humans, when incorporated into various food/beverage items at 100 mg CGM per serving size, as compared to the standard unformulated curcumin.
1. Introduction
Curcuma longa L. (Turmeric) is a popular kitchen spice that has been widely used in Indian curries and medicinal preparations for thousands of years. Modern scientific research have identified many bioactive compounds in turmeric rhizomes, in which curcumin or diferuloylmethane, [1,7-bis[4-hydroxy-3-methoxyphenyl]-1,6-heptadiene-3,5-dione], a hydrophobic polyphenolic compound, has been characterized as the most active component responsible for numerous health beneficial pharmacological effects.1,2 Observational studies have already delineated the dietary intake of turmeric with a reduced incidence of chronic diseases such as Cancer and Alzheimer's in the subcontinent of India.3,4 With more than 3000 preclinical investigations on various aspects of cancer, curcumin stands as one of the best studied natural products to date; a significant promising candidate capable of selectively modulating multiple cell signaling pathways.1–4 Considering the multi-targeted mechanism of action of this promiscuous natural agent of immense therapeutic value, curcumin has emerged as an ‘yellow gold’.5 Though some food/beverage products containing curcumin are available today, the number of branded food products containing physiologically relevant amounts of curcumin per serving is limited due to the very low solubility and stability of curcuminoids in water and oil.2,6 The solubility of curcumin in aqueous buffer at pH 5.0 has been reported to be as low as 11 ng mL−1.6 In addition, the poor systemic oral bioavailability of curcumin (due to its extremely low aqueous solubility, high hydrolytic instability and rapid enzymatic in vivo degradation) has also been a major limitation against the development of curcumin as a functional food ingredient or therapeutic agent.2,6,7
While the uptake and distribution of curcumin in the body is essential for its biological activity, curcumin offers only negligible quantities in plasma due to the poor absorption, permeability and rapid metabolism.2,7 The consumption of even 10 to 12 g of curcumin as capsules was reported to provide only less than 50 ng mL−1 of curcumin in human plasma.8 Several attempts to overcome the problem of poor bioavailability of curcuminoids, comprising the use of adjuvants to inhibit in vivo enzymatic degradation, the formation of nanoparticles, and the formulation of phospholipid complexes and liposomes, have been reported.2,7,9 However, enhanced bioavailable formulations suitable for development as food ingredients are limited due to the presence of non-food grade excipients, extreme insolubility/hydrophobicity, nano-size, regulatory noncompliance and high cost. Thus, there exists a huge demand for novel technologies and formulations to produce cost-effective food-grade bioavailable formulations for stable and water soluble curcuminoids employing GRAS (Generally Regarded as Safe)-listed natural ingredients.
Recently, we reported an enhanced bioavailable form of curcumin, namely, curcumagalactomannosides (hereafter named as ‘CGM’), formulated using fenugreek (Trigonella foenum-graecum)-derived soluble dietary fiber composed of galactose and mannose units (galactomannan).10 Fenugreek galactomannans have already been established as a versatile hydrocolloid possessing gelling, thickening, emulsifying, stabilizing and encapsulating properties,11 with functional benefits such as hypolipidemic, hypoglycaemic, appetite reducing and gastroprotective effects.12 CGM was found to deliver significantly high levels of plasma curcumin (1 to 1.5 μM for 6 to 8 h post-administration) as compared to the standard unformulated curcumin (0.04 to 0.08 μM for less than an hour) when supplemented at 250 mg kg−1 b.wt in Wistar rats and 250 mg in humans.10 Thus, CGM is a highly economical, hydrocolloid-based, regulatory compliant, enhanced bioavailable form of curcumin, having the potential for further development as a novel functional food ingredient, if it can further satisfy the other requirements on taste/flavor, solubility, stability, microbial status, and safety. In the present contribution, solubility, particle size, stability (storage, pH and heat), nutritional composition and safety (as studied by acute, subchronic and genotoxicity studies), have been investigated to check its possibility of development as a food ingredient capable of delivering significant amounts of curcumin into the blood stream. Optimized dosages of CGM for incorporation in selected food/beverage items, without compromising the taste/appearance and bioavailability, has also been investigated in the present study. HPLC-PDA and tandem mass spectrometry (ESI-MS/MS) were employed for the quantification of free curcuminoids in plasma, and the area under curve (AUC) calculations of total free curcuminoids concentration-time plots was considered for bioavailability estimations. Unformulated curcumin standardized to 95% purity was supplemented as (500 mg × 2) hard shell gelatin capsules for the purpose of comparing the bioavailability, since it was not possible to incorporate the unformulated curcumin into the food and beverage recipes investigated in the present study.
2. Materials and methods
2.1. General
Curcumagalactomannosides (CGM) exhibiting enhanced bioavailability (prepared as per the method of Krishnakumar et al., 2012; see ref. 10) and unformulated curcumin standardized to 95% purity were provided by Akay Flavours & Aromatics Pvt Ltd, Kerala, India. Standard curcumin (CAS Registry no. 458-37-7) was purchased from Sigma-Aldrich, Bangalore, India. Curcumin content was estimated by a reverse phase high pressure liquid chromatography (HPLC) procedure, employing a Phenomenex column (250 × 4.6 mm, 3 μm) (Shimadzu Analytical Pvt. Ltd, Mumbai, India).13 An Agilent 6460 triple quadrupole tandem mass spectrometer (MS/MS) fitted with an electrospray ionization (ESI) probe (Agilent India Pvt. Ltd, Bangalore, India) was employed for the identification of curcuminoids in plasma using multiple reaction monitoring mode (MRM). An Agilent JetStream source operated with a capillary voltage of 3500 V, 375 °C sheath gas temperature and 325 °C drying gas temperature was employed in positive ionization mode. MRM for each of the curcuminoids were recorded at collision energies of 48, 24 and 20 V respectively for curcumin, DMC and BDMC, with the corresponding fragmentor energies of 113, 103 and 94 V. Analytical reference standards of curcumin (CAS# 458-37-7; purity >98%), DMC (CAS# 22608-11-3; purity >98%) and BDMC (CAS# 33171-05-0; purity >95%) were obtained from Sigma-Aldrich, Bangalore, India. Nutritional composition was analysed by a standardised procedure from the Association of Analytical Chemists.14 Particle size was measured using a Melvern Zetasizer Nano ZS90 particle size analyzer (Malvern Instruments Ltd, Worcestershire, UK).
2.2. Stability studies of CGM
Storage stability studies were carried out using a protocol prepared by following the International Conference on Harmonization (ICH) guidelines.15 Briefly, the sample packets (10 g) of CGM were incubated at 40 °C ± 2 °C and 75 ± 5% relative humidity for a period of 6 months in a stability chamber (Remi, Mumbai, India). The samples were withdrawn at 0, 1, 2, 3, and 6 months and analyzed for various physicochemical parameters, as shown in Table 1. The pH stability of the aqueous solution was checked by preparing 5% (w/w) solutions at pH 2.0, 5.0, and 6.8 using hydrochloric acid and phosphate buffers. 5 mL volumes of the solutions were withdrawn at regular intervals of 2 h for a period of 24 h, and the curcumin content was checked by HPLC. Temperature stability was verified by maintaining the solution at 90 ± 2 °C for 30 min followed by HPLC analysis.
Table 1 Stability study of CGM performed as per an in-house protocol developed on the basis of ICH guidelines. The samples were incubated at 40 ± 2 °C and 75% ± 5% RH for a period of 6 months
Parameter |
0 month |
1 month |
2 months |
3 months |
6 months |
Appearance |
Golden yellow to orange colour granules |
Golden yellow to orange colour granules |
Golden yellow to orange colour granules |
Golden yellow to orange colour granules |
Golden yellow to orange colour granules |
Odour |
Characteristic |
Characteristic |
Characteristic |
Characteristic |
Characteristic |
Curcumin content (%) |
40.8 |
40.6 |
39.9 |
40.5 |
40.2 |
Moisture (%) |
2.3 |
2.3 |
2.3 |
2.3 |
2.2 |
Bulk density (g mL−1) |
0.51 |
0.51 |
0.51 |
0.51 |
0.51 |
Carbohydrates (g per 100 g) |
80.04 |
80.14 |
79.26 |
80.71 |
80.16 |
Protein (g per 100 g) |
6.0 |
6.1 |
5.8 |
6.0 |
6.0 |
|
Microbiology
|
Total plate count |
<1000 cfu g−1 |
300 cfu g−1 |
400 cfu g−1 |
350 cfu g−1 |
350 cfu g−1 |
Yeast & Mould |
<100 cfu g−1 |
30 cfu g−1 |
30 cfu g−1 |
30 cfu g−1 |
30 cfu g−1 |
Coliforms |
<3 MPN g−1 |
<3 MPN g−1 |
<3 MPN g−1 |
<3 MPN g−1 |
<3 MPN g−1 |
E. coli |
Absent/g |
Absent/g |
Absent/g |
Absent/g |
Absent/g |
Salmonella |
Absent/25 g |
Absent/25 g |
Absent/25 g |
Absent/25 g |
Absent/25 g |
2.3. Animals
All animal experiments were conducted after obtaining prior permission from the Institutional Animal Ethics Committee and were performed as per the instructions prescribed by the Committee for the Purpose of Control and Supervision of Experiments on Animals (CPCSEA), Government of India. Young adult male (average b.wt 200 g) and female (average b.wt 150 g) Wistar rats, 7–8 weeks old, were purchased from the Animal Breeding Station, Kerala Agricultural University, India. They were housed in the animal house facility of the Amala Cancer Research Centre, in well ventilated sterile polypropylene cages. Rats were maintained at a controlled temperature of 22 ± 2 °C and a relative humidity of 60 ± 5% and provided with 12 h light/dark cycles. They were fed with normal pelleted rat chow (M/s Sai Durga Feeds and Foods, Bangalore, India) and water ad libitum.
2.4. Toxicity studies of CGM
2.4.1. Acute toxicity studies.
Acute toxicity studies were performed initially to verify whether CGM produced any toxic effects when supplemented at the maximum recommended concentration. CGM was suspended in water and orally administered as a single dose at the limit dose of 5 g kg−1 b.wt in a sequential manner. Forty rats were divided into four groups, with each group containing five animals per sex with similar weights. Group I was the control (untreated), and Groups II, III and IV were administered with CGM at 1.0, 2.0 and 5.0 g kg−1 b.wt respectively. All the animals were observed for mortality, clinical and behavioral signs for the first 10, 30, 60, 120, 240 and 360 min post dose, and thereafter were observed twice daily for mortality and once daily for clinical signs during the study period of 14 days.
2.4.2. Oral subchronic toxicity study (90 days).
Fifty healthy Wistar rats, after acclimatization to laboratory conditions, were divided into five groups as above. Group I was kept untreated normal, group II as vehicle (1 mL water) treated control and groups III, IV and V treated with CGM at doses of 0.5, 1.0 and 2.0 g kg−1 b.wt respectively, by oral gavage for 90 days. Observations of pharmacotoxic signs were made at 10, 30, 60 and 120 min post-dose during the first day, and continued twice daily for 90 days. The animals were monitored for clinical and behavioral symptoms such as diarrhea, immobility, neuromuscular problems, mortality and any other adverse reactions. Body weight and food and water consumption were determined every week and expressed for a single cage of five animals. After 90 days, the animals were sacrificed by cervical dislocation under light ether anesthesia. Necropsy of the animals was performed in the presence of a veterinary doctor, and observations were recorded. The weight of the brain, liver, stomach, kidney and spleen were recorded and expressed in relation to the final body weight. The tissue samples were fixed in 10% formalin, and embedded tissues were cut into slices of 2 to 4 μm and stained with hematoxylin-eosin for histopathological examinations with an optical microscope of 100× magnification (Olympus-Magnus trinocular microscope, Tokyo, Japan).
Blood was collected by direct heart puncture and taken into EDTA vials for assaying haematological parameters. The total white blood cells were measured after diluting the blood in Turk's fluid and counting them using a hemocytometer.15 For the differential leukocyte count, a blood smear was prepared on a clean glass slide and stained with Leishman's stain, and various types of cells were counted manually using a microscope.16 The platelet count was determined by diluting the blood with Rees and Ecker diluting fluid and counting the platelets using a haemocytometer.16 The total RBC count was measured by diluting the blood with Dacie's fluid and counting the red blood cells using a counting chamber.16 The haemoglobin content (Hb) was measured by cyanomethaemoglobin (Drabkin's) method using a kit purchased from M/s Agappe Diagnostics, Mumbai, India.
A portion of blood was also collected in non-EDTA tubes, and the serum was separated by centrifugation at 5000 rpm for 10 min and was used for the following investigations. The total bilirubin was determined as detailed by the Pearlman method;17 alkaline phosphatase (ALP) was estimated by p-nitrophenyl picolinate (PNNP) hydrolysis; alanine amino transferase (ALT) and aspartate aminotransferase (AST) were estimated using kinetic method kits supplied by M/s Raichem, India, using a Microlab 300 auto analyzer (Merck, Mumbai, India); albumin was determined by its reaction with bromocresol green, and the total protein concentration was determined by the Biuret method.18 Kidney function markers, such as creatinine and blood urea nitrogen, were estimated by Jaffe's kinetic and urease methods respectively.19 The total cholesterol was estimated by the CHOD–PAP (cholesterol oxidase − phenol + aminophenazone) enzymatic method,20 triglycerides by the GPO–PAP (glycerol-3-phosphate oxidase − phenol + aminophenazone) method21 and HDL cholesterol by precipitation with phosphotungistic acid. VLDL cholesterol was estimated by the Friedewald equation (VLDL = triglyceride/5) and LDL cholesterol by the equation LDL = total cholesterol – (HDL + VLDL). Serum sodium, potassium and bicarbonate were estimated using a Flame photometer with an ion selective electrolyte analyzer. Chloride was estimated by the mercurous thiocyanate method using a kit from M/s Raichem, India.
2.4.3. Mutagenicity studies.
Evaluation of the ability of CGM to induce reverse mutation at the histidine loci in three S. typhimurium strains, TA 98, TA 100 and TA 102 (Ames test), was conducted according to standard procedures.22 Mutagenicity of CGM was done by plate incorporation method in the presence and absence of an exogenous metabolic activation system at five doses (0.1, 0.5, 1, 3, 5 mg per plate) in triplicates for each dose. 5 μg sodium azide/plate dissolved in distilled sterile water was used as positive control. A plate without drug and mutagens was used as a negative control, and 200 μl DMSO was used as a vehicle control. 2 mL top agar layer (0.6% agar and 0.5% NaCl) containing S. typhimurium strains, 0.5 mM histidine–biotin solution and different concentrations of CGM were shaken well and poured onto 25 mL of agar. The plates (triplicate) were incubated for 48 h at 37 °C, and revertant colonies were counted using a colony counter.
Rat liver microsomal enzyme was used for metabolic activation of the mutagen in vitro.23 Microsome P450 enzyme was induced in the rat liver by the administration of 0.1% phenobarbital; the liver was excised aseptically, and microsomal S9 fractions were prepared by mixing S9 mix (500 μL) with sodium phosphate buffer (0.2 M, pH 7.4), NADP (0.1 M), glucose 6-phosphate (1 M, pH 7.4), MgCl2–KCl (10 μL) in the presence of mutagen, 2-acetamidoflourene (20 μg per plate), different concentrations of CGM and bacterial strains TA 98, TA 100, TA 102. The fractions were incubated at 37 °C for 45 min. They were then mixed with 2 mL of molten top agar, supplemented with histidine and biotin (0.05 mM). The mixture was shaken well and poured onto the surface of 25 mL of minimal agar. After 48 h incubation, the mutagenic response was evaluated by counting the revertant colonies per plate and comparing with the control groups. The test substance was considered to be mutagenic if there was a three-fold increase in the tester strains when compared to the negative control.
2.5. Preparation of food containing CGM and its oral bioavailability studies
Food items containing 100 mg per serving size of CGM were successfully prepared, and their organoleptic properties were assessed by a panel of seven experts. On the basis of sensory results, the following food items were used for consumption and subsequent estimation of the bioavailability of curcumin from the foodstuffs. Vegetable soup premix powder containing 100 mg of CGM per 10 g was prepared and made into soup with 100 mL of 60 to 65 °C hot water; 20 g of mango juice pulp was homogenized with 100 mg of CGM and further diluted to 100 mL with cold water; 100 mg of CGM containing 30 g yogurt, honey containing 100 mg of CGM per 10 g, chocolate containing 100 mg of CGM per 20 g and 20 g of cookies containing 100 mg of CGM were selected for studies.
Eight human volunteers (four male and four female) aged between 25 and 40 years, who were healthy and not involved in any medication or health supplementation was selected for bioavailability studies. Institutional ethical committee clearance (IEC) and written consent from all individuals were obtained before the study, as per the protocol suggested by the Government of India. The volunteers were not allowed to ingest turmeric-containing food for three days prior to the test. Each volunteer was first given 1000 mg (500 mg × 2 hard gelatin capsules) of unformulated standard curcumin as the placebo, since it could not be incorporated in the above mentioned food items. 2 mL blood samples were withdrawn at 0, 0.5, 1, 3 and 5 h post-dose; the plasma was separated by centrifugation and frozen at −20 °C until analysis. After 1 week of the curcumin study, the subjects were given one serving of a food item containing 100 mg CGM, and the protocol was repeated exactly the same as above for the collection of plasma samples at various times after food consumption. Volunteers were asked to complete the food consumption within 3 min. The same procedure was repeated for each food item at an interval of one week. The total curcuminoids in plasma was measured by a previously reported reverse phase HPLC-PDA-ESI-MS/MS procedure employing PDA detection at 420 nm and methanol as the mobile phase (Phenomenex column 250 × 4.6 mm, 3 μm).10 The mass spectrometer was operated in positive ionization mode in multiple reaction monitoring (MRM) mode, which could detect m/z 369 (369 → 116.9) for curcumin, m/z 339 (339 → 146.9) for DMC and m/z 309 (309 → 146.9) for BDMC.
2.6. Statistical analysis
The values are expressed as mean ± SD. The statistical significance was compared between untreated and experimental groups by one way analysis of variance (ANOVA) followed by an appropriate post hoc test (Dunnet multiple comparison test) using Graphpad InStat software (version 3.05). The data for CGM treated animals were compared with those for untreated animals. The pharmacokinetic parameters were calculated by linear regression using Microsoft Excel (Microsoft Corporation, Richmond, VA, USA).
3. Results and discussion
Dietary intake of curcumin, the yellow pigment in turmeric rhizomes, has witnessed a tremendous growth during the last decade, owing to the increasing amount of research based evidences demonstrating its safety and pharmacological benefits as an efficient natural agent having a multi-targeted mechanism of action against various types of disease states, including cancer and Alzheimer's.1–4 However, the poor oral bioavailability of curcuminoids remains a limiting factor in attempts to translate most of these preclinical results to the clinic.2 The new formulation of curcumagalactomannosides (CGM) has already been shown to exhibit a more than 20-fold enhancement in bioavailability compared to standard curcumin when supplemented as capsules.10 CGM is a combination of bioactive extracts of two popular kitchen spices (curcumin and fenugreek) without any organic solvents or additives. The present contribution reports other properties of CGM, such as solubility, stability, particle size and safety, to exploit the possibility of its use as a food ingredient capable of providing significant levels of plasma curcuminoids. Various food/beverage items impregnated with CGM at various dosages per serving size were prepared and analyzed for sensory perception. The efficacy of such food items to provide high plasma curcumin levels (bioavailability) upon the consumption of a serving size of these foods was also investigated as proof of the concept.
A formulation of curcumin with enhanced bioavailability (CGM) was prepared from 95% pure commercially available curcumin and consisted of 78% curcumin, 16% demethoxycurcumin, 6% bis-demethoxycurcumin (commonly referred to as ‘curcuminoids’) and fenugreek derived soluble dietary fiber containing 76% galactomannans and 16% proteins. An earlier reported procedure using a gel-phase dispersion technique was employed for the preparation of CGM.10 CGM was obtained as a free flowing powder with 100 to 200 μm particles, which are capable of swelling and dissolving in water to produce a homogeneous and viscous yellow solution consisting of colloidal particles of 1.2 ± 0.2 μm. The swelling capacity of CGM was measured as 20.2 mL g−1 in water at pH 7.0, indicating its high water holding capacity. It was found to have a nutritional composition of 46.9% dietary fiber, 6.0% protein, 2.8% fat, 2.3% moisture and 40.2% curcumin with an energy content of 64.4 calorie per 100 g.
3.1. Stability of CGM
When tested for storage stability under accelerated conditions as specified in ICH guidelines, CGM was found to undergo only a 2.1% loss in curcumin content, with no significant change in other parameters such as colour, appearance, odour, taste, moisture content, bulk density, carbohydrates, proteins, and microbial status (Table 1), indicating the possibility of convenient storage for two years at ambient conditions of less than 25 °C in closed containers, away from direct light and moisture. An aqueous solution of CGM at pH 7.0 showed only 3% curcumin degradation when stored for 21 days under room conditions of 28 ± 2 °C. On heating to 90 ± 2 °C for 30 min, the solution showed only 0.1% curcumin degradation. Thus, the observed solubility, stability and enhanced bioavailability of CGM, along with the possibility of its economic production on a commercial scale and regulatory compliance, is the basis of the present study to further evaluate its potential as a novel functional food ingredient capable of delivering significant levels of curcuminoids into the bloodstream.
3.2. Toxicity studies of CGM
A systematic investigation of the safety of CGM as studied by acute, subchronic and genotoxicity was carried out, as these studies are generally used to satisfy hazard classification and labelling requirements for risk assessment in human health and the environment.24 The application of ultrasound as a novel method for the preparation of curcumagalactomannosides (CGM) particles, and its subsequent oral delivery to produce significantly high levels of colloidal curcumin in the intestine for better absorption, leading to a longer duration of significantly high levels of curcumin in the blood, demanded the pharmacological safety evaluation of CGM for human use. Moreover, the systematic evaluation of the toxicity of fenugreek galactomannans as employed in the preparation of CGM has not been reported, though toxicity studies of debitterized fenugreek powder containing more than 60% fiber content have been reported.25 However, curcumin has been evaluated in detail and reported as safe even at high doses.8 Though an initial investigation of 28 days of repeated dose toxicity studies of CGM on Wistar rats has shown no adverse effects,10 the present study evaluated the oral acute (14 days), subchronic (90 days) and genotoxic effects of CGM.
3.2.1. Acute toxicity studies.
The administration of a single high dose of CGM up to a concentration of 5 g kg−1 b.wt did not produce any mortality, behavioral changes or clinical signs of toxicity either immediately or during the post-treatment period of 14 days, irrespective of the sex of the animals. Food and water intake and body weight changes were similar to those of the normal group of animals, indicating that CGM has no detrimental effect upon growth patterns, since these indicators can show the adverse effects of drugs and chemicals.26 Necropsy of the animals showed neither any morphological changes in the tissues nor any gross pathological abnormalities, with no significant change in the weight of organs as compared to the untreated group of animals of both sexes after 14 days of administration. Hepatic function parameters (ALT, AST, ALP, total bilirubin, total albumin & globulin and A/G ratio), renal function parameters (blood urea, serum creatinine, serum electrolytes), and haematological parameters (WBC, RBC, platelet, haemoglobin, HDL, LDL, VLDL, triglycerides) were also comparable to those of the normal animals, indicating the safety of CGM administration at 5 g kg−1 b.wt.
3.2.2. Subchronic toxicity studies.
Oral administration of CGM for 90 days at doses of 0.5, 1.0 and 2.0 g kg−1 b.wt did not produce any obvious signs of toxicity or mortality. The body weight and relative food and water intake of the animals treated with CGM were similar to those of the untreated groups of animals of both sexes (Fig. 1). The average food intake of the male rats was nearly 15 g per day per animal, and that of the female rats was 10 g per day per animal. Data on the various haematological parameters measured in rats fed with CGM and the normal groups of animals are given in Table 2, and showed no significant alterations in Hb, RBC, WBC, and differential counts. The haematopoietic system is often considered one of the most sensitive parameters to assess the toxicity of drugs in animals and humans.26 Similarly, the data on the biochemical parameters of animals treated with CGM and those of the untreated normal group (Table 2) also showed no significant alterations in any of the specific enzyme activities (SGPT, SGOT, ALP) or in bilirubin, total protein and A/G ratio. Since liver damage is usually accompanied by an elevation of serum SGPT and SGOT, the consumption of CGM was found to have no toxic effects on the liver.27,28 A similar trend was observed in renal function markers (blood urea, serum creatinine, and serum electrolytes) even though higher levels of curcuminoids were being provided to the bloodstream continuously for 90 days by the administration of CGM at 2.0 g kg−1 body weight (Table 2). The data indicate no adverse effects on nephron cell function, since any damage to nephron cells will be accompanied by an elevation of serum creatinine and urea.26,28 Similarly, increases in creatinine and urea levels may be attributed either to damage to nephron cell function29 or to the depletion of extracellular fluid volume, which usually occurs during hyperglycemia.30 Cholesterol, LDL, HDL, triglyceride and VLDL cholesterol levels also showed no significant changes after thirteen weeks of oral administration of CGM, when compared to the untreated rats of both sexes (Table 2). Since cholesterol degradation, glucose synthesis and generation of free glucose from hepatic glycogen take place in the liver, this result also indicated the normal functioning of the liver, without any adverse effect on lipid or carbohydrate metabolism.
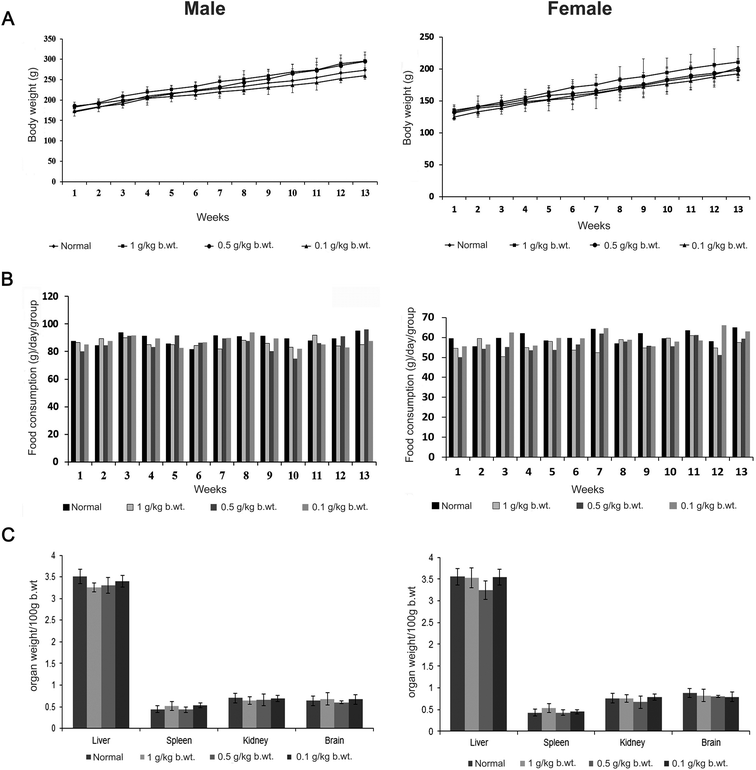 |
| Fig. 1 Effect of subchronic administration of CGM on (A) body weight, (B) food consumption, (C) weight of organs of both male and female rats. | |
Table 2 Hematological and biochemical parameters of rats treated with CGM at 2.0 g kg−1 b.wt for 90 daysa
Parameters |
Control |
2.0 g kg−1 |
1.0 g kg−1 |
0.5 g kg−1 |
Male |
Female |
Male |
Female |
Male |
Female |
Male |
Female |
Values are expressed as the mean ± SD, n = 5 animals of each sex per group.
|
Biochemical parameters
|
ALT (U L−1) |
79.8 ± 8.70 |
58.20 ± 11.17 |
70.8 ± 16.15 |
61.4 ± 14.54 |
64.4 ± 8.29 |
42.2 ± 3.70 |
80.8 ± 13.92 |
57.4 ± 14.54 |
AST (U L−1) |
178.4 ± 29.11 |
164.6 ± 19.46 |
207 ± 42.64 |
157.2 ± 33.10 |
186.6 ± 29.24 |
161.8 ± 20.07 |
189.2 ± 18.73 |
185.8 ± 33.10 |
ALP (U L−1) |
351.4 ± 27.22 |
325.0 ± 42.52 |
376.4 ± 26.81 |
266.2 ± 52.49 |
327.6 ± 51.26 |
231.2 ± 36.77 |
360 ± 34.32 |
262.2 ± 52.49 |
Bilirubin (mg dL−1) |
0.5 ± 0.07 |
0.40 ± 0.07 |
0.54 ± 0.05 |
0.54 ± 0.09 |
0.5 ± 0.07 |
0.5 ± 0.07 |
0.5 ± 0.10 |
0.48 ± 0.09 |
Urea (mg dL−1) |
66.6 ± 8.26 |
34.8 ± 3.11 |
43.2 ± 3.90 |
40.6 ± 10.45 |
59.8 ± 11.69 |
44.0 ± 7.65 |
45.4 ± 7.65 |
38.8 ± 11.1 |
Creatinine (mg dL−1) |
0.70 ± 0.07 |
0.50 ± 0.23 |
0.72 ± 0.11 |
0.72 ± 0.08 |
0.70 ± 0.16 |
0.68 ± 0.19 |
0.60 ± 0.10 |
0.46 ± 0.13 |
Cholesterol (mg dL−1) |
73.4 ± 7.44 |
77 ± 17.39 |
65.2 ± 3.56 |
84.4 ± 6.47 |
63.2 ± 6.38 |
82.8 ± 5.26 |
78.2 ± 12.79 |
69.0 ± 2.83 |
Triglycerides (mg dL−1) |
97.8 ± 12.5 |
127.8 ± 11.92 |
88.2 ± 5.17 |
107.4 ± 12.10 |
86.0 ± 5.79 |
98.8 ± 18.24 |
109 ± 11.11 |
118.4 ± 11.55 |
HDL (mg dL−1) |
32.6 ± 2.97 |
33.6 ± 3.85 |
29.8 ± 2.59 |
33.0 ± 3.16 |
29.4 ± 1.34 |
31.6 ± 2.07 |
31.8 ± 1.92 |
32.2 ± 1.10 |
LDL (mg dL−1) |
21.2 ± 8.98 |
18.2 ± 11.67 |
17.8 ± 3.35 |
30.0 ± 6.89 |
16.6 ± 4.56 |
31.4 ± 8.20 |
24.4 ± 10.74 |
13.2 ± 3.11 |
VLDL (mg dL−1) |
19.6 ± 2.61 |
25.6 ± 2.51 |
17.6 ± 1.14 |
21.4 ± 2.41 |
16.8 ± 1.79 |
19.8 ± 3.63 |
20.0 ± 3.16 |
24.0 ± 2.45 |
Sodium (mEq L−1) |
145.36 ± 3.95 |
144.70 ± 2.72 |
143.12 ± 0.87 |
134.38 ± 2.17 |
141.98 ± 1.02 |
142.28 ± 4.03 |
146.26 ± 3.95 |
134.38 ± 1.09 |
Potassium (mEq L−1) |
7.27 ± 1.63 |
6.08 ± 1.27 |
5.89 ± 1.31 |
6.15 ± 1.30 |
4.68 ± 1.29 |
5.09 ± 1.13 |
5.08 ± 0.44 |
6.15 ± 1.44 |
Chloride (mEq L−1) |
103.86 ± 0.96 |
103.94 ± 1.22 |
104.50 ± 0.67 |
102.70 ± 2.48 |
103.38 ± 0.97 |
101.56 ± 2.87 |
104.24 ± 1.5 |
102.70 ± 2.54 |
|
Hematological parameters
|
Hb (g dL−1) |
14.48 ± 0.94 |
13.16 ± 1.04 |
13.84 ± 1.22 |
13.62 ± 1.97 |
14.82 ± 1.35 |
14.52 ± 0.5 |
15.02 ± 0.58 |
14.26 ± 0.89 |
WBC (×103 mm−3) |
9.5 ± 2.18 |
5.58 ± 1.54 |
7.84 ± 2.12 |
11.7 ± 4.23 |
12.28 ± 2.32 |
05.48 ± 1.96 |
10.26 ± 0.48 |
8.12 ± 3.21 |
Platelet (×106 mm−3) |
514.2 ± 50 |
513.5 ± 69 |
579.6 ± 73 |
558.4 ± 91 |
468.4 ± 90 |
557.1 ± 69 |
502.3 ± 49 |
420.9 ± 87 |
RBC (×106 mm−3) |
7.48 ± 0.40 |
6.48 ± 0.69 |
6.82 ± 0.47 |
6.96 ± 1.12 |
7.61 ± 0.67 |
7.07 ± 0.43 |
7.71 ± 0.18 |
6.98 ± 0.23 |
Neutrophils (%) |
25.8 ± 5.12 |
24.8 ± 4.76 |
23.0 ± 5.24 |
25.2 ± 7.46 |
23.6 ± 4.04 |
23.6 ± 5.03 |
22.4 ± 3.65 |
24.5 ± 3.79 |
Lymphocytes (%) |
64.6 ± 4.83 |
65.8 ± 5.76 |
68.4 ± 4.93 |
65.6 ± 8.38 |
67.0 ± 3.54 |
66.8 ± 5.72 |
69.6 ± 4.77 |
68.3 ± 2.99 |
Necropsy of the CGM-treated group of animals, immediately after dissection, showed normal appearances of various organs and tissues. There were no significant differences in the group mean absolute or relative weights of various vital organs among CGM-treated rats when compared with untreated animals (Table 1). Histopathology of the brain, kidney, spleen and liver did not show any abnormalities among CGM treated animals, indicating the absence of any adverse toxicological effects from CGM administration at a dose of 2.0 g kg−1 b.wt for 13 weeks (Fig. 2).
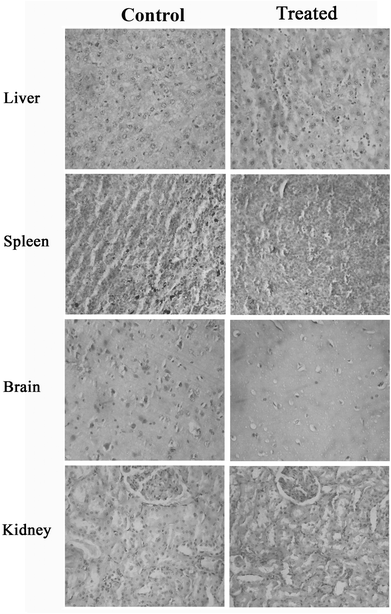 |
| Fig. 2 Histopathological analysis of rat organs treated with 2 g kg−1 b.wt of CGM for 90 days. | |
3.2.3. Mutagenicity of CGM.
Mutagenic substances can alter genetic material, and may act as carcinogens. Some act as direct mutagens, and some are indirect mutagens needing S9 microsomal fractions for activation. The Ames test is a quick and convenient assay to assess the mutagenic potential of a compound, and 90% of known carcinogens may be identified via this test.21 Though curcumin is known to be non-mutagenic and has been reported to inhibit the mutagenicity of several environmental mutagens and carcinogens,31 the unique composition of soluble dietary fiber and fenugreek proteins used in the preparation of CGM has not yet been tested for mutagenic effects. It was found that curcumagalactomannosides (CGM) did not produce any revertants in the absence and presence of metabolic activation (S9) mix when tested up to a concentration of 5 mg per plate, as compared to the negative control, in S. typhimurium strains. The positive control containing known mutagens induced an increase in the mean number of revertant colonies in each strain, while the vehicle control did not show any increase in revertant colonies (Table 3), indicating that there was no dose related mutagenicity for CGM, either with or without metabolic activation.
Table 3 Mutagenic study of CGM with and without S9-mix in Salmonella typhimurium using a reverse mutation assaya
|
Doses of CGM (μg per plate) |
TA 98 |
TA 100 |
TA102 |
The values are mean ± standard deviation of 3 different determinations.
Sodium azide (5 μg per plate) was used as the mutagen.
Spontaneous reversion (without mutagen and drug).
2-Acetamidofluorene (20 μg per plate) was used as the mutagen.
|
(Without S9-mix) |
Positive controlb |
861 ± 16 |
662 ± 16 |
860 ± 28 |
Negative controlc |
33 ± 3 |
118 ± 6 |
121 ± 4 |
5000 μg per plate |
25 ± 3 |
96 ± 5 |
91 ± 12 |
3000 μg per plate |
28 ± 2 |
102 ± 4 |
103 ± 3 |
1000 μg per plate |
34 ± 1 |
110 ± 6 |
104 ± 4 |
500 μg per plate |
34 ± 2 |
125 ± 6 |
100 ± 4 |
100 μg per plate |
34 ± 1 |
124 ± 4 |
104 ± 5 |
(With\ S9-mix) |
Positive controld |
683 ± 16 |
884 ± 17 |
875 ± 25 |
Negative controlc |
39 ± 5 |
129 ± 3 |
128 ± 4 |
5000 μg per plate |
35 ± 6 |
129 ± 9 |
104 ± 6 |
3000 μg per plate |
40 ± 6 |
146 ± 6 |
118 ± 4 |
1000 μg per plate |
44 ± 8 |
148 ± 10 |
109 ± 3 |
500 μg per plate |
36 ± 5 |
158 ± 8 |
124 ± 4 |
100 μg per plate |
38 ± 4 |
158 ± 13 |
127 ± 6 |
3.3. Preparation of food/beverage items containing CGM
Selected food items were prepared by incorporating the new formulation of curcumin, CGM, at levels of 100, 200 and 300 mg per serving, and subjected to sensory analysis by a panel of experts to select the optimum dosage which can be conveniently incorporated into the food matrix without compromising taste and appeal. Although these dosages of CGM could be incorporated without issues, the bioavailability study was carried out at a dosage of 100 mg per serving to verify the efficacy of the lowest possible dose. 100 mg of CGM-incorporated soup premix, with an attractive pale yellow colour, gave a tasty soup when 10 g of the premix was diluted with 100 mL of water at 60 to 65 °C. Fortification of fruit/vegetable juices, juice powders and fruit pulp also gave acceptable organoleptic properties, with an enhancement of colour to juices such as mango, pineapple, lemon and orange. The concentration of CGM may be adjusted to obtain the desired colour hue. A commercially available mango pulp (20 g) was fortified with 100 mg of CGM by homogenization and further diluted to obtain 100 mL mango juice. Homogenization was found to be a suitable method for impregnating honey with CGM to produce a desired concentration of 100 mg per 10 g of honey. Yet another matrix investigated for impregnation with CGM was chocolate. CGM was added along with cocoa solids and further ground to produce chocolates containing 100 mg CGM per 20 g, with an attractive dark brown characteristic chocolate colour. It was also added to milk chocolates to produce natural yellow coloured chocolate of lemon shade. When attempts were made to incorporate CGM in yogurt and milk, a slight settling of curcuminoids was observed. However, this problem was solved by high pressure homogenization in the presence of a thickening agent, pectin. Finally, cookies were also prepared with 100 mg CGM per 20 g, to obtain brownish yellow cookies. Though there were no taste or aroma issues, the baking process for the cookies, at 175 °C for 20 min, was found to degrade the curcuminoid levels by 33%.
3.4. Bioavailability studies
The relative absorption of curcuminoids upon consumption of CGM-incorporated food was tested on human volunteers, by quantifying the curcuminoid levels in blood plasma at various intervals of time after the ingestion of one serving size of the food item containing 100 mg of CGM. The identity of curcuminoids in plasma was confirmed by spiking standard curcumin in animal plasma at 1.0 μg mL−1, followed by extraction of the curcuminoids and positive ion electrospray tandem mass spectrometric analysis in MRM mode. Consumption of food/beverages containing CGM was found to significantly (p < 0.05) enhance the plasma curcuminoid levels, as compared to standard curcumin with 95% purity, when administered as 1000 mg (500 mg × 2) hard shell gelatin capsules (Fig. 3). The area under curve (AUC) calculations of the plot of plasma curcumin concentration vs. time revealed a relative enhancement of 7.2 times for soup, 5.8 times for yogurt, 6.8 times for mango juice, 8.7 times for honey and 7.4 times for chocolate when consumed as a single serving containing 100 mg of CGM. AUC is regarded as a robust method of measurement of bioavailability, since it takes into consideration the entire response over time, rather than the maximum concentration of curcumin observed in the blood at a particular time point, Cmax.32 However, it is important to note that no curcumin could be detected upon consumption of 20 g of cookies containing 100 mg of CGM, which were made via a high temperature baking process. The time taken to reach the maximum curcumin concentration in plasma was 30 min after the food ingestion, and no curcuminoids detected after 4 h. Unformulated curcumin, on the other hand, was never absorbed when used in the food items investigated in the present paper, though uniform impregnation was not achieved due to insolubility and crystallinity. The solubility of standard curcumin in aqueous solutions is very low, and the hydrolytic instability is very high.6 It is therefore poorly absorbed even with high dosages, as high as 12 g per day.8 The pharmacokinetic parameters, including the maximum concentration of curcuminoids found in plasma (Cmax), the time at which the maximum curcumin concentration was found in plasma (Tmax), and the time taken for 50% of absorbed curcumin to degrade (T1/2 max), were also estimated (Table 4). It was observed that Cmax and AUC0–6 h were significantly higher after the consumption of CGM-containing food, but the Cmax and AUC0–6 h values were different for different food items (Fig. 3). However, all the parameters were significantly high for CGM-impregnated foods as compared to standard curcumin, indicating the increased bioavailability of curcumin upon the consumption of food containing CGM. Tmax for CGM-containing food was 30 to 60 min as compared to 30 min for standard curcumin. Moreover, the absorbed curcumin was found to stay in the blood for a longer duration, as evident from the T1/2max values, ranging from 2 to 2.7 h in various food matrices, as compared to standard curcumin (Table 4). While various bioavailable formulations of curcumin have been reported, this is the first report, to the best of our knowledge, of the use of a bioavailable formulation of curcumin as a dietary ingredient capable of furnishing curcumin in plasma upon consumption of the respective food.
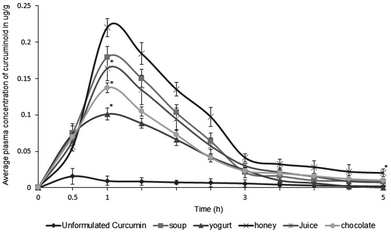 |
| Fig. 3 Average concentration of curcumin observed in human plasma after consumption of various food items containing 100 mg of CGM, per serving. Serving sizes were 10 g soup premix powder in 100 mL hot water, 20 g mango pulp in 100 mL water, 10 g honey, 20 g chocolate and 30 g of yogurt. Standard curcumin was administered at a 1000 mg dose as hard shell gelatin capsules (500 mg × 2). The data is expressed as the mean ± SD (n = 8); *p < 0.05; (100 mg CGM containing food versus 1000 mg standard curcumin). | |
Table 4 Pharmacokinetic parameters calculated from the plasma curcumin concentration – time plot for humans after consumption of food containing CGM
Sample administered |
Subjects (n) |
Dose |
C
max (μg g−1) |
T
max (h) |
T
1/2 max (h) |
AUC0–6 h (μg g−1) |
100 mg per serving size of CGM was impregnated in each of the food items; 100 mg CGM contains 40 mg of curcumin.
ND denotes ‘Not detected’. Cmax – maximum concentration of curcumin found in plasma, Tmax – time at which maximum curcumin concentration was found in plasma, T1/2 max – time taken for 50% of the absorbed curcumin to degrade.
|
Standard- curcumin |
8 |
1000 mg |
0.015 ± 0.005 |
0.5 |
0.7 |
205 ± 34 |
Soup |
8 |
100 mga |
0.179 ± 0.03 |
1 |
2.4 |
2460 ± 78 |
Yogurt |
8 |
100 mga |
0.101 ± 0.05 |
1 |
1.6 |
1435 ± 96 |
Honey |
8 |
100 mga |
0.219 ± 0.03 |
1 |
2.7 |
3075 ± 61 |
Juice |
8 |
100 mga |
0.163 ± 0.07 |
1 |
2.4 |
2255 ± 134 |
Chocolate |
8 |
100 mga |
0.137 ± 0.04 |
1 |
2.0 |
1845 ± 59 |
Cookies |
8 |
100 mga |
NDb |
NDb |
NDb |
NDb |
It was observed from the above results that the functionalities of fenugreek soluble fiber/protein composite, such as high swelling index in water, gel-like character and gummy nature, helped to establish a strong binding of colloidal curcumin in the fiber network, providing a microencapsulation effect even when present in various food/beverage matrices, which provided protection against rapid metabolism during gastrointestinal transit. Moreover, the thickening property of the fiber and the effect of ultrasonication aided in changing the character of the leached curcuminoids into amorphous, water miscible and colloidal forms, for better absorption.
4. Conclusions
The present study demonstrated the safety, stability, solubility and enhanced bioavailability of a novel food grade formulation of curcumin, CGM, when used as a dietary ingredient. The formulation was prepared by the ultrasound mediated gel-phase impregnation of curcumin into a non-digestible, but fermentable prebiotic soluble fiber – protein composite isolated from the popular kitchen spice fenugreek, without any excipients/additives. The present toxicity study, carried out in our laboratory, revealed the safety of the acute dosage at 5 g kg−1 b.wt and subchronic oral administration at 2.0 g kg−1 b.wt of CGM in Wistar rats with a NOAEL of 2.0 g kg−1 per day. The Ames test demonstrated no mutagenic character to CGM when tested up to a concentration of 5 mg per plate. Further investigations on solubility, particle size, stability and organoleptic properties indicated the possibility of the usage of CGM as a functional hydrocolloid-based food ingredient capable of providing enhanced bioavailability for curcumin when incorporated into various food and beverage items at 100 to 300 mg per serving size, without compromising the taste/appeal. Considering the encouraging results, further optimization of the conditions of impregnation of CGM in various food matrices are warranted for future development of this novel curcumin ingredient.
Conflict of interest
The authors declare that there are no conflicts of interest.
Acknowledgements
The authors are grateful to M/s Spices Board of India for the financial support, and the research grant MKT/R&D/04/10-12 for the value addition of spices. The authors also sincerely thank the Food Application Laboratory of M/s Akay Flavours & Aromatics Pvt. Ltd for the preparation of CGM incorporated food/beverages.
References
- I. Shureiqi and J. A. Baron, Cancer Prev. Res., 2011, 4, 296–298 CrossRef CAS PubMed.
- P. Sahdeo, K. T. Amit and B. A. Bharat, Cancer Res. Treat., 2014, 46, 2–18 CrossRef PubMed.
- C. M. Kaefer and J. A. Minler, J. Nutr. Biochem., 2008, 19, 347–361 CrossRef CAS PubMed.
- K. Ramaswamy, C. G. Subash, J. H. Kim, R. Simone and B. B. Aggarwal, Mol. Neurobiol., 2011, 44, 142–159 CrossRef PubMed.
- C. T. Keith, A. A. Borisy and B. R. Stockwell, Nat. Rev. Drug Discovery, 2005, 4, 71–78 CrossRef CAS PubMed.
- H. H. Tonnesen, M. Masson and T. Loftsson, Int. J. Pharm., 2002, 244, 127–135 CrossRef CAS.
- M. M. Yallapu, M. Jaggi and S. C. Chauhan, Drug Discovery Today, 2012, 17, 71–80 CrossRef CAS PubMed.
- C. D. Lao, M. T. Ruffin, D. Normolle, D. D. Heath, S. I. Murray, J. M. Bailey, M. E. Boggs, J. Crowell, C. L. Rock and D. E. Brenner, BMC Complement. Altern. Med., 2006, 7, 6–10 Search PubMed.
- H. Sasaki, Y. Sunagawa, K. Takahashi, A. Imaizumi, H. Fukuda, T. Hashimoto, H. Wada, Y. Katanasaka, H. Kakeya, M. Fujita, K. Hasegawa and T. Morimoto, Biol. Pharm. Bull., 2011, 34, 660–665 CAS.
- I. M. Krishnakumar, A. Ravi, D. Kumar, R. Kuttan and B. Maliakel, J. Funct. Foods, 2012, 4, 348–357 CrossRef PubMed.
- M. Srivastava and V. P. Kapoor, Chem. Biodiversity, 2005, 2, 295–317 CAS.
- J. R. Mathern, S. K. Raatz, W. Thomas and J. L. Slavin, Phytother. Res., 2009, 23, 1543–1548 CrossRef CAS PubMed.
- B. K. Jadhav, K. R. Mahadik and A. R. Paradkar, Chromatographia, 2007, 65, 483–488 CAS.
-
W. Horwits and G. W. Latimer Jr., Official Methods of Analysis of AOAC International, Maryland, USA, 18th edn, 2002 Search PubMed.
-
Guidance for Industry Q1A (R2), Stability Testing of New Drug Substances and Products, 2003. http://www.fda.gov/downloads/drugs/guidancecomplianceregulatoryinformation/guidances/ucm073369.pdf Search PubMed.
-
D. A. Nelson and M. W. Morrie, in Basic methodology in clinical diagnosis and management by laboratory methods, ed. J. B. Henry, Philadelphia, 1984, p. 589 Search PubMed.
-
G. K. Pal and P. Parvati, Textbook of practical physiology, Orient Longman Private Limited, India, 2003 Search PubMed.
- F. C. Pearlman and R. T. Lee, Clin. Chem., 1974, 20, 447–453 CAS.
- O. H. Lowry, N. J. Rosebrough, A. L. Farr and R. J. Randall, J. Biol. Chem., 1951, 193, 265–275 CAS.
- R. Deeg and J. Ziegenhorn, Clin. Chem., 1983, 29, 1798–1802 CAS.
-
T. G. Cole, S. G. Klotzsch and J. McNamara, in Handbook of lipoprotein testing, ed. N. Riafi, G. R. Warnick and M. H. Dominiczak, AACC Press, Washington, 1997, pp. 115–126 Search PubMed.
- B. N. Ames, J. Mccann and E. Yamasaki, Mutat. Res., 1975, 31, 347–364 CAS.
- G. K. Jayaprakasha, B. S. Jena, P. S. Negi and K. K. Sakariah, Z. Naturforsch., 2002, C57, 828–835 Search PubMed.
-
OECD Guidance on acute oral toxicity testing, in OECD Environment Health and Safety
publications series on testing and assessment, No.24, Organization for Economic Co-operation and Development, Paris, 2001 Search PubMed.
- Muralidhara, K. Narasimhamurthy, S. Viswanatha and B. S. Ramesh, Food Chem. Toxicol., 1999, 37, 831–838 CrossRef CAS.
- S. P. Tofovic and E. K. Jackson, J. Cardiovasc. Pharmacol., 1999, 33, 360–366 CrossRef CAS PubMed.
- M. F. Rahman, M. K. Siddiqui and K. Jamil, Hum. Exp. Toxicol., 2001, 20, 243–249 CAS.
- J. E. Hilaly, Z. H. Israili and B. Lyouss, J. Ethnopharmacol., 2004, 91, 43–50 CrossRef PubMed.
- N. Lameire, W. Van Biesen and R. Vanholder, Lancet, 2005, 365, 417–430 CrossRef CAS.
- C. E. Mogenson and C. K. Christensen, Hypertension, 1985, 7, 1164–1168 Search PubMed.
- M. Nagabhushan and S. V. Bhide, Nutr. Cancer, 1986, 8, 201–210 CrossRef CAS PubMed.
- W. Stahl, H. van den Berg, J. Aruthur and A. Bastl, Mol. Aspects Med., 2002, 23, 39–100 CrossRef CAS.
|
This journal is © The Royal Society of Chemistry 2015 |