DOI:
10.1039/C4FO00697F
(Paper)
Food Funct., 2015,
6, 203-209
Oleic acid content of a meal promotes oleoylethanolamide response and reduces subsequent energy intake in humans
Received
4th August 2014
, Accepted 30th September 2014
First published on 1st October 2014
Abstract
Animal data suggest that dietary fat composition may influence endocannabinoid (EC) response and dietary behavior. This study tested the hypothesis that fatty acid composition of a meal can influence the short-term response of ECs and subsequent energy intake in humans. Fifteen volunteers on three occasions were randomly offered a meal containing 30 g of bread and 30 mL of one of three selected oils: sunflower oil (SO), high oleic sunflower oil (HOSO) and virgin olive oil (VOO). Plasma EC concentrations and appetite ratings over 2 h and energy intake over 24 h following the experimental meal were measured. Results showed that after HOSO and VOO consumption the circulating oleoylethanolamide (OEA) was significantly higher than after SO consumption; a concomitantly significant reduction of energy intake was found. For the first time the oleic acid content of a meal was demonstrated to increase the post-prandial response of circulating OEA and to reduce energy intake at subsequent meals in humans.
Introduction
Endocannabinoids (ECs) are a class of lipid mediators which act as endogenous ligands of the G protein-coupled cannabinoid receptors. In the early nineties, the two primary ECs were discovered: the arachidonoylethanolamide (AEA) and the 2-arachidonoylglycerol (2-AG).1,2 AEA together with palmitoylethanolamide (PEA), oleoylethanolamide (OEA), and linoleoylethanolamide (LEA) belongs to the chemical group of N-acylethanolamines (NAEs).3–5 All these compounds take part in a wide range of biological processes: pain, anxiety and depression, nausea, addiction and withdrawal,6 and innate immunity.7 Moreover, they are involved in feeding regulation by influencing metabolic and reward systems.8 In particular, AEA and 2-AG showed orexigenic properties in rodents as they dose-dependently increased food intake by central and peripheral administration9,10 and were shown to be modulated by fasting and feeding states in the brain.11 In humans, the role of 2-AG in hedonic eating was demonstrated by Monteleone et al.12 who found a significant increase of the 2-AG concentration in plasma 2 h after consumption of a highly palatable meal but not after consumption of a non-palatable meal.
In contrast oral or intraperitoneal administration of OEA, as well as its duodenal increase, determined a decrease of food intake in mice and rats13–20 (for a review of the literature see Piomelli21); the mechanism underlying such an effect being recently demonstrated to involve the histaminergic system.22
The chemical composition of the ingested food plays a primary role in the OEA formation: infusion into the duodenum of glucose or proteins did not show any effect, whereas among several fats, only oleic acid elicited OEA production in animals.23
Interestingly, in humans Joosten and co-workers24 found that fasting and non-fasting plasma concentrations of AEA, OEA, PEA and stearoylethanolamide (SEA) were positively associated with both serum total free fatty acids and their specific fatty acid precursors namely arachidonic, oleic, palmitic and stearic acids, respectively.
However, in humans the evidence of diet influence on the EC system is still scarce and limited on macronutrient ratios.25 Moreover, to the best of our knowledge the post-prandial EC response was never associated with appetite cues and following energy intake in humans.
The objective of this study was to test the hypothesis that fatty acid composition of a meal, and mainly its oleic acid content, can influence the short-term response of ECs and subsequent energy intake in humans. To this purpose three equicaloric meals with the same macronutrient composition but containing oils providing different amounts of oleic acid were offered to healthy and fasted volunteers. Blood drawings were performed over the following two hours and energy intake at subsequent meals and over the following 24 h were measured by self-recorded food diaries.
Materials and methods
Materials
AEA, LEA, OEA, PEA, 2-AG and d8-AEA were purchased from Cayman (Cayman Chemical, Ann Arbor, MI). Ethanol (EtOH), methanol (MeOH), chloroform, acetone and water were purchased from Merck (Darmstadt, Germany). Plastic Vacutainer® serum tubes (16 × 100 mm, 10 mL) were purchased from Becton & Dickinson (1 Becton Drive, Franklin Lakes, NJ, USA). Polypropylene 1.5 mL tubes were purchased from Eppendorf (Hamburg, Germany), 12 × 75 mm glass tubes were purchased from Corning (Corning S.r.l., Via Mercantini 5, Turin, Italy). Verex™ Vial, 9 mm, screw top, μVial i3 (Qsert) and PTFE/silicone cap were purchased from Phenomenex (Torrance, CA, USA). Sunflower seed oil, high oleic sunflower oil and virgin olive oil were provided by the Oleifici Mataluni (Montesarchio, Benevento, Italy).
Subjects
Healthy subjects were selected from among students and staff of the Department of Agriculture of “Federico II” University of Naples. Thirty-five subjects were screened. Subjects taking any kind of drug, or presenting endocrine, hepatic, renal, tumoral, autoimmune, cardiovascular, hematological, neurological or psychiatric diseases, sleep disorders, or allergies requiring treatment, as well as those who experimented variation of their body weight over the previous three months or who were on a restrictive diet were excluded. The 51-item Three Factor Eating Questionnaire (TFEQ) was used to exclude restraint subjects (score in the restraint subscale F1 > 8).26 Fifteen subjects were eligible and they were enrolled to participate after signing an informed written consent. They were 7 male and 8 female, between 22 and 40 years old with a BMI between 18.1 and 25.0 kg m−2. All experimental procedures were approved by the Ethics Committee of the University of Naples.
Meals
Three oils differing in their fatty acid compositions were used in this study (Table 1). They were sunflower seed oil (SO), high oleic sunflower oil (HOSO) and virgin olive oil (VOO).
Table 1 Fatty acid composition and differences among sunflower seed oil (SO), high oleic sunflower oil (HOSO) and virgin olive oil (VOO) used in this study
Fatty acid |
Composition (%) |
Difference (%) |
SO |
HOSO |
VOO |
HOSO − SO |
VOO − SO |
Myristic acid |
0.07 |
0.05 |
0.01 |
−0.02 |
−0.06 |
Palmitic acid |
6.42 |
4.42 |
12.08 |
−2.00 |
5.66 |
Palmitoleic acid |
0.14 |
0.16 |
0.69 |
0.02 |
0.55 |
Heptadecanoic acid |
0.04 |
0.03 |
0.04 |
−0.01 |
0.00 |
Heptadecenoic acid |
0.02 |
0.04 |
0.06 |
0.02 |
0.04 |
Stearic acid |
3.29 |
2.67 |
2.34 |
−0.62 |
−0.95 |
Oleic acid trans |
0.04 |
0.04 |
0.00 |
0.00 |
−0.04 |
Oleic acid |
33.20 |
79.04 |
75.26 |
45.84 |
42.06 |
Linoleic acid trans |
0.45 |
0.09 |
0.00 |
−0.36 |
−0.45 |
Linoleic acid |
55.02 |
11.99 |
8.25 |
−43.03 |
−46.77 |
Arachidic acid |
0.24 |
0.24 |
0.28 |
0.00 |
0.04 |
Linolenic acid |
0.07 |
0.04 |
0.67 |
−0.03 |
0.60 |
Eicosenoic acid |
0.17 |
0.24 |
0.25 |
0.07 |
0.08 |
Behenic acid |
0.64 |
0.73 |
0.04 |
0.09 |
−0.60 |
Lignoceric acid |
0.21 |
0.23 |
0.01 |
0.02 |
−0.20 |
Thirty milliliters of each oil together with 30 g white bread were offered to the fasting subjects on 3 different occasions. Each meal provided an energy intake of 357 kcal, of which 75.9% came from lipids, 2.9% from proteins and 21.2% from carbohydrates. A higher content of lipids than in a nutritionally balanced meal was used in order to exclude the potential confounding factors from other meal components on both the short-term physiological response of ECs and the appetite cues.
Study protocol
The study was conducted at the Department of Agriculture of the University of Naples. It was a randomized intervention trial with a cross-over design. Volunteers were invited to reach the nutrition laboratory at 8:00 a.m. in a 10 h fasting condition on three occasions with a 1-week wash-out period from each other. On the evening before each test volunteers were instructed to consume a standardized dinner and to refrain from eating and drinking alcoholic or energy-drinks from 22:00 h. Once they arrived at the laboratory the participants had a 10 min rest and then they were instructed to rate their hunger, fullness, satiety, thirst and desire to eat on 100 mm visual analogue scales (VAS)27 anchored on the left as “not at all” and on the right as “extremely”. The questionnaire comprised 3 main questions (How great is your desire to eat?, How full do you feel?, and How satiated do you feel?), and subjects were asked to answer indicating on the scale the point corresponding to their sensations. After the first blood drawing (baseline) each subject was asked to seat in a specific position isolated from the others, and was presented a tray containing the experimental meal including the type of oil he/she was randomized to consume in that occasion. Subjects were asked to consume the meal within 15 minutes and the compliance was evaluated by controlling that the glass and the plate containing the foods were empty at the end of breakfast. At the following 30, 60, 120 minutes the subjects rated their appetite sensations on VAS and underwent blood drawings. After the last blood drawing, before participants left the laboratory, they were instructed to fill a 24 h food diary by recording the exact time, the types and amount (weight) of foods and beverages consumed from the moment they left the laboratory until the day after. On the next day volunteers had to return their 24 h food diary to the expert nutritionist of the research group and were submitted to a 24 h diet recall interview in order to assess the compliance and to validate the 24 h food diary.
Biochemical analysis
Blood was collected in Vacutainer® serum tubes and centrifuged at 2400g for 10 min at 4 °C. Serum was aliquoted (by 500 μL) and kept frozen at −80 °C until analysis.28 Concentrations of AEA, LEA, OEA, PEA and 2-AG were determined by isotopic dilution liquid chromatography-mass spectrometry as described previously by Côté and co-workers.29 Five hundred microliters of each sample were added in 1.5 mL polypropylene tubes and protein precipitation was obtained by adding 3 volumes of acetone and centrifuging at 14
000g for 10 min at 4 °C. The supernatants were collected, transferred to 12 × 75 mm glass tubes and subjected to lipid extraction by adding 1.5 mL of methanol–chloroform (1
:
2) containing 5 pmol of d8-anandamide as an internal standard. The organic phase was then dried under nitrogen, the resulting residue re-suspended in 100 μL of acetonitrile–water (1
:
1) and centrifuged (4 °C; 2400g; 10 min).
Chromatographic separation was performed using an HPLC apparatus equipped with two micropumps, Perkin-Elmer Series 200 (Norwalk, CT, USA). A Synergi Max RP 80 column, 50 × 2.1 mm (Phenomenex, USA) was used and the flow rate was set to 0.2 mL min−1. The injection volume was 10 μL. The mobile phase A consisted of H2O and 0.2% formic acid, while the mobile phase B was CH3CN. The gradient program was as follows: 50–79% B (10 min), 79–95% B (1 min), constant at 95% B (2 min), and finally returning to the initial conditions in 2 min. MS/MS analyses were performed on an API 3000 triple quadrupole mass spectrometer (Applied Biosystems, Canada). All the analyses were performed with a TurboIonSpray source with the following settings: drying gas (air) heated to 300 °C and a capillary voltage (IS) of 5000 V. The declustering potential (DP) and the collision energy (CE) were optimized for each compound by direct infusion of standard solutions (10 μg mL−1) into the mass spectrometer at a flow rate of 6 μL min−1, using a Model 11 syringe pump (Harvard, Apparatus, Holliston, MA, USA). The acquisition was carried out in MRM (Multiple Reaction Monitoring) in a positive ion mode for each compound.
Data acquisition and processing were performed using the Analyst software v. 1.4. Acquisition parameters are summarized in Table 2.
Table 2 Acquisition parameters used for the LC/MS/MS analysis
|
Precursor ion [M + H]+ |
Product ion [M + H]+ |
DP |
CE |
AEA |
348.0 |
62 |
40 |
35 |
OEA |
326.0 |
62 |
40 |
35 |
LEA |
324.0 |
62 |
60 |
30 |
PEA |
300.0 |
62 |
60 |
30 |
2-AG |
396.5 |
379.5 |
35 |
11 |
287.3 |
14 |
268.9 |
18 |
AEA-d8 |
356.5 |
63.2 |
50 |
31 |
209.3 |
18 |
Data analysis and statistics
The sample size needed to detect an effect of meal composition on the primary outcome (post-prandial response of ECs) and secondary outcome (the effect of meal composition on subsequent energy intake) was estimated on the basis of previous studies. A sample size of 13 participants was calculated to be adequate to find changes in the EC response significantly different using variation in accordance with Monteleone et al.12 and Gatta-Cherifi et al.,30 with an 80% power and an α = 0.05. A sample size of 12 subjects was adequate to detect a 19% difference in energy intake with a power of 80% and an α = 0.05 using variation in accordance with the findings of our previous studies.31,32
Statistical analyses were performed using the statistical package SPSS for Windows (version 13).
The results of both the appetite scores and of biochemical analyses were analyzed and expressed as the absolute changes from the baseline to reduce possible effects of inter-subject fasting variability. The subjective appetite sensations recorded after the consumption of the three types of oils and the EC curves were compared and tested for the effect of treatment and of time as factors using ANOVA for repeated measures. The total area under the curves (AUC) for hunger, fullness and satiety ratings (from baseline over 2 h from breakfast consumption) as well as for EC blood concentrations were also estimated using the linear trapezoidal rule. Differences in the AUC values were analyzed by one-way ANOVA and by Newman–Keuls multiple comparison test as post hoc. Differences were considered significant at p < 0.05.
Results
Biochemical analysis
No significant difference of individual plasma concentrations of ECs at baseline among experimental meals was found. Fig. 1 (panel A) shows the variations of plasma concentrations of AEA, 2-AG, LEA and PEA over 2 hours following the three meals. A tendency for reduced concentrations of AEA, 2-AG and PEA irrespective of the type of breakfast consumed was found. However, the OEA concentrations following HOSO and VOO consumption were 23.7% and 20.5% significantly higher than that following SO consumption, with AUC0–120 being 858 ± 54 pmol min mL−1, and 823 ± 28 pmol min mL−1vs. 654 ± 70 pmol min mL−1, respectively (Fig. 1, panel B). LEA concentrations did not change over time upon the three meals.
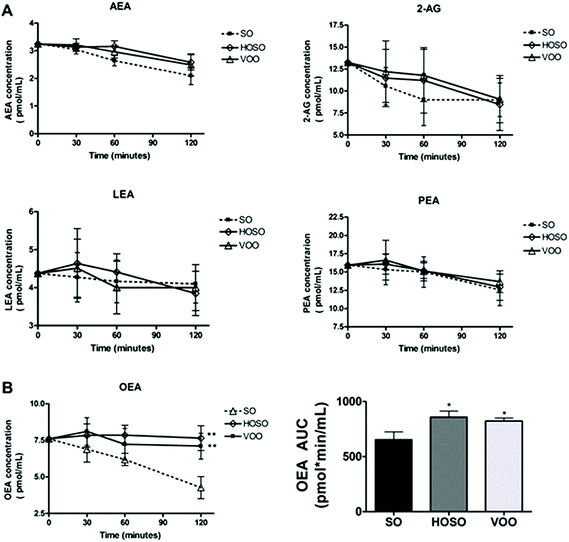 |
| Fig. 1 Post-prandial response of endocannabinoids. (A) concentration–time curves of AEA, 2-AG, LEA and PEA over 120 min following experimental meals; no significant difference of concentrations at baseline and following time points among experimental meals was found; (B) concentration–time curve and AUC of OEA over 120 min following experimental meals; no significant difference of baseline concentrations among experimental meals was found. Values are expressed as means ± SEM. *p < 0.05 vs. SO; **p < 0.001 vs. SO. | |
Energy intake at subsequent lunch and over 24 h
All participants returned a well done 24 h food diary and were submitted to 24 h diet recall interview. Data indicated that no difference in timing of subsequent lunch was found among participants following the three experimental meals. All subjects had their lunch always 3 h after the experimental meal. However, subjects had a significant 261 kcal and 250 kcal energy reduced lunch after HOSO and VOO consumption compared to SO consumption, respectively (Fig. 2).
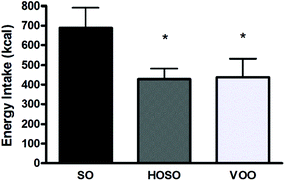 |
| Fig. 2 Energy intake. Energy intake (kcal) consumed during the lunch subsequent each experimental meal expressed as means ± standard deviation. *p < 0.05 for VOO and HOSO vs. SO. | |
No significant difference of energy intake over the 24 h was found (1787 ± 602 kcal, 1803 ± 542 kcal and 1646 ± 430 kcal following HOSO, VOO and SO consumption, respectively).
Appetite ratings
No significant difference of sensations of hunger, fullness and satiety at baseline among experimental meals was found. Fig. 3 shows appetite ratings and AUC over the 2 h following the consumption of breakfasts containing VOO, HOSO or SO. A trend of hunger reduction at 30 min and return to the baseline value over the following 60 min after the three meals were recorded. Only after 120 min of SO consumption subjects perceived a hunger sensation higher than the baseline and that perceived after HOSO and VOO consumption. Interestingly, increased fullness and satiety compared to baseline were found between 30 min and 60 min after meals containing HOSO and VOO, respectively, but not after SO. These perceptions were prolonged at 120 min only following VOO consumption. Looking at the appetite sensations over the 2 h after the breakfasts (AUC0–120), significant reductions of hunger and increase of fullness and satiety were found after VOO consumption compared to SO consumption.
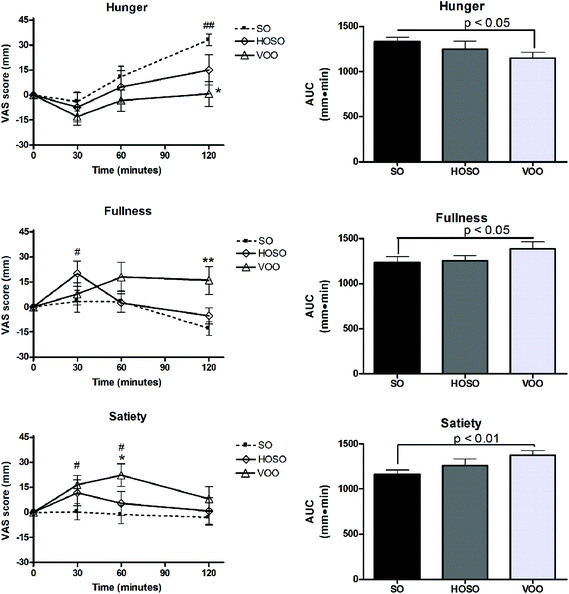 |
| Fig. 3 Appetite. Appetite rating–time curves and AUC of appetite sensations over 120 min following experimental meals. Values are expressed as means ± SEM. No significant difference of appetite sensations at baseline among experimental meals was found. At 120 min: *p < 0.01 for hunger following VOO vs. SO; **p < 0.001 for fullness following VOO vs. SO; #p < 0.01 from baseline; ##p < 0.001 from the baseline. AUCs of hunger, fullness and satiety after VOO are significantly different from SO. | |
Discussion
The main finding of this study is that the content of oleic acid ingested at a meal influences the post-prandial EC response, appetite sensations and energy intake at subsequent meals in humans.
Few human studies investigated the response of ECs to meals with specific chemical composition. In this study a trend to reduced postprandial concentrations of all ECs except that of OEA after HOSO and VOO consumption and of LEA after all experimental conditions were found.
The reduced post-prandial concentrations of ECs were in accordance with findings of previous studies.24,30,33 A physiological reason to this response might be linked to the peripheral action of post-prandial insulin and to the direct influence of meal lipids on EC biosynthesis/hydrolysis route in the upper intestine. In fact, Di Marzo and co-workers28 suggested that insulin reduces EC levels in a way inversely related to insulin resistance and it is known that dietary monounsaturated or polyunsaturated fatty acids can increase post-prandial insulin sensitivity in healthy subjects.34 Thus, it is likely that the consumption of a meal rich in unsaturated fatty acids might generally have reduced EC response through insulin.
On the other hand, the consumption of meals providing higher amounts of oleic acid (such as that including HOSO and VOO vs. SO) might sustain the post-prandial concentration of OEA independently from insulin action. In fact, oleic acid may act as precursor of OEA formation in the intestine as previously demonstrated in animals35–37 and/or trigger some physiological mechanisms modulating its selective spillover from the intestinal membrane phospholipids. This hypothesis is consistent with a previous study demonstrating that the consumption of virgin olive oil and high-oleic sunflower oil determined, over the following 2 hours, a significant increase of circulating oleic acid-rich phospholipids,38 which are known to be the precursors of intestinal biosynthesis of ECs at the mucosa level, epithelial cells and serosa.39 In addition, a strict connection between circulating ECs and free fatty acids was recently suggested in humans by Joosten and co-workers.24
Factors other than fatty acid composition of oils might have influenced the post-prandial response of LEA whose concentration did not change vs. baseline after the three meals.
It could not be excluded in the present study that different cephalic responses triggered by oral taste and/or different preference for the oils might contribute to influence the circulating pattern of ECs, through their well-known interaction with the gut metabolism. That dietary fat (but not other nutrients) can modify gut metabolism of ECs through oral sensing and selectively mobilize ECs in the upper gut, also influencing dietary behavior, was demonstrated in rats.40 On the other hand a link between circulating 2-AG and food preference was found by Monteleone and co-workers12 who showed increased plasma 2-AG in humans after consumption of their preferred food but not after the non-preferred one.12
Further human studies should clarify the role of meal lipid composition on the formation of different ECs induced by cephalic response.
Strikingly, both the meals eliciting the highest post-prandial OEA response (VOO and HOSO vs. SO) were associated with the highest reductions of energy intake at subsequent meals. These findings are in disagreement with the animal study conducted by Gaetani and co-workers,15 where OEA administration in free-feeding rats reduced only the meal frequency without altering the meal size, whereas they were perfectly in line with Provensi and co-workers.22
Social and cognitive cues could greatly influence the timing of eating in humans compared to animals, thus rendering insignificant the effect of OEA response on the time of eating while evidencing OEA effect on food intake at subsequent meals in our free-living participants. Several researchers aimed at ranking the effect of lipid composition on satiety.41–43 Alfenas and co-workers44 proposed that the satiety effect of fatty acids was linked to their oxidation rate: the higher is the number of double bonds, the faster is the rate of oxidation, the higher is the satiety. However, other studies did not confirm this suggestion.45,46 Several differences among the studies, including the amount and source of fats provided to the volunteers might cause such discrepancies rendering the debate still open.
Appetite ratings after consumption of the meal containing VOO were coherent with the reduced energy intake compared to the meal with SO. This might be a matter of dietary habits and cognitive factors on appetite sensations. In fact, data from food frequencies questionnaire (not shown) indicated that all study participants were used to consume virgin olive oil as conditioning fat, while the consumption of seed oils was sporadic. Familiarity with a food and expected satiation are interrelated. More familiar foods are expected to be more filling47 and measures of expected satiety are highly correlated with actual satiety.48 From a mechanistic point of view it could not be excluded that non-fat components present in VOO (but not in SO or HOSO) such as several volatile compounds (attributing to VOO the characteristic aroma) and phenolic compounds might contribute to the effect of VOO consumption on energy intake and appetite regulation as recently suggested in the elegant study by Frank and co-workers49 or reviewed by Panickar,50 respectively.
Conclusion
In conclusion, in this study for the first time it was demonstrated that the oleic acid content of a meal can increase the post-prandial response of circulating OEA and it may reduce energy intake at subsequent meals in humans. The present data offer a concept to design new food ingredients for energy intake control using edible oils rich in oleic acid. Further studies should evaluate whether these findings can be also reproduced in overweight/obese subjects and/or in the context of meals nutritionally balanced for macronutrient ratio.
Acknowledgements
The authors declare no competing financial interest.
References
- W. A. Devane, L. Hanus, A. Breuer, R. G. Pertwee, L. A. Stevenson, G. Griffin, D. Gibson, A. Mandelbaum, A. Etinger and R. Mechoulam, Science, 1992, 258, 1946–1949 CAS
.
- T. Sugiura, S. Kondo, A. Sukagawa, S. Nakane, A. Shinoda, K. Itoh, A. Yamashita and K. Waku, Biochem. Biophys. Res. Commun., 1995, 215, 89–97 CrossRef CAS
.
- S. Kondo, T. Sugiura, T. Kodaka, N. Kudo, K. Waku and A. Tokumura, Arch. Biochem. Biophys., 1998, 354, 303–310 CrossRef CAS PubMed
.
- H. H. Hansen, C. Ikonomidou, P. Bittigau, S. H. Hansen and H. S. Hansen, J. Neurochem., 2001, 76, 39–46 CrossRef CAS
.
- H. H. Schmid, P. C. Schmid and V. Natarajan, Prog. Lipid Res., 1990, 29, 1–43 CrossRef CAS
.
- J. F. Blankman and F. C. Benjamin, Pharmacol. Rev., 2013, 65, 849–871 CrossRef PubMed
.
- A. C. Kendall and A. Nicolaou, Prog. Lipid Res., 2013, 52, 141–164 CrossRef CAS PubMed
.
- V. Di Marzo, A. Ligresti and L. Cristino, Int. J. Obes., 2009, 33, S18–S24 CrossRef CAS PubMed
.
- C. M. Williams and T. C. Kirkham, Psychopharmacology, 1999, 143, 315–317 CrossRef CAS
.
- T. C. Kirkham, C. M. Williams, F. Fezza and V. Di Marzo, Br. J. Pharmacol., 2002, 136, 550–557 CrossRef CAS PubMed
.
- T. F. Gamage and A. H. Lichtman, Pediatr. Blood Cancer, 2012, 58, 144–148 Search PubMed
.
- P. Monteleone, F. Piscitelli, P. Scognamiglio, A. M. Monteleone, B. Canestrelli, V. Di Marzo and M. Mai, J. Clin. Endocrinol. Metab., 2012, 97, E917–E924 CrossRef CAS PubMed
.
- F. Rodríguez de Fonseca, M. Navarro, R. Gómez, L. Escuredo, F. Nava, J. Fu, E. Murillo-Rodríguez, A. Giuffrida, J. LoVerme, S. Gaetani, S. Kathuria, C. Gall and D. Piomelli, Nature, 2001, 414, 209–212 CrossRef PubMed
.
- J. Fu, S. Gaetani, F. Oveisi, J. Lo Verme, A. Serrano, F. Rodríguez De Fonseca, A. Rosengarth, H. Luecke, B. Di Giacomo, G. Tarzia and D. Piomelli, Nature, 2004, 425, 90–93 CrossRef PubMed
.
- S. Gaetani, F. Oveisi and D. Piomelli, Neuropsychopharmacology, 2003, 28, 1311–1316 CrossRef CAS PubMed
.
- F. Oveisi, S. Gaetani, K. T. Eng and D. Piomelli, Pharmacol. Res., 2004, 49, 461–466 CrossRef CAS PubMed
.
- M. J. Nielsen, G. Petersen, A. Astrup and H. S. Hansen, J. Lipid Res., 2004, 45, 1027–1029 CrossRef CAS PubMed
.
- K. Proulx, D. Cota, T. R. Castañeda, M. H. Tschöp, D. A. D'Alessio, P. Tso, S. C. Woods and R. J. Seeley, Am. J. Physiol. Regul. Integr. Comp. Physiol., 2005, 289, R729–R737 CrossRef CAS PubMed
.
- G. Astarita, B. Di Giacomo, S. Gaetani, F. Oveisi, T. R. Compton, S. Rivara, G. Tarzia, M. Mor and D. Piomelli, J. Pharmacol. Exp. Ther., 2006, 318, 563–570 CrossRef CAS PubMed
.
- J. Fu, J. Kim, F. Oveisi, G. Astarita and D. Piomelli, Am. J. Physiol. Regul. Integr. Comp. Physiol., 2008, 295, R45–R50 CrossRef CAS PubMed
.
- D. Piomelli, Trends Endocrinol. Metab., 2013, 24, 332–341 CrossRef CAS PubMed
.
- G. Provensi, R. Coccurello, H. Umehara, L. Munari, G. Giacovazzo, N. Galeotti, D. Nosi, S. Gaetani, A. Romano, A. Moles, R. Blandina and M. B. Passani, Proc. Natl. Acad. Sci. U.S.A., 2014, 111, 11527–11532 CrossRef CAS PubMed
.
- G. J. Schwartz, J. Fu, G. Astarita, X. Li, S. Gaetani, P. Campolongo, V. Cuomo and D. Piomelli, Cell Metab., 2008, 8, 281–288 CrossRef CAS PubMed
.
- M. Joosten, M. G. Balvers, K. C. Verhoeckx, H. F. Hendriks and R. F. Witkamp, Nutr. Metab., 2010, 14(7), 49 Search PubMed
.
- S. S. Naughton, M. L. Mathai, D. H. Hryciw and A. J. McAinch, Int. J. Endocrinol., 2013, 361895 Search PubMed
.
- A. J. Stunkard and S. Messick, J. Psychosom. Res., 1985, 29, 71–83 CrossRef CAS
.
- S. M. Green, H. J. Delargy, D. Joanes and J. E. Blundell, Appetite, 1997, 29, 291–304 CrossRef CAS
.
- V. Di Marzo, A. Verrijken, A. Hakkarainen, S. Petrosino, I. Mertens, N. Lundbom, F. Piscitelli, J. Westerbacka, A. Soro-Paavonen, I. Matias, L. Van Gaal and M. R. Taskinen, Eur. J. Endocrinol., 2009, 161, 715–722 CrossRef CAS PubMed
.
- M. Côté, I. Matias, I. Lemieux, S. Petrosino, N. Alméras, J. P. Després and V. Di Marzo, Int. J. Obes., 2007, 31, 692–699 Search PubMed
.
- B. Gatta-Cherifi, I. Matias, M. Vallée, A. Tabarin, G. Marsicano, P. V. Piazza and D. Cota, Int. J. Obes., 2012, 36, 880–885 CrossRef CAS PubMed
.
- P. Vitaglione, R. Barone Lumaga, A. Stanzione, L. Scalfi and V. Fogliano, Appetite, 2009, 53, 338–344 CrossRef CAS PubMed
.
- R. Barone Lumaga, D. Azzali, V. Fogliano, L. Scalfi and P. Vitaglione, Food Funct., 2012, 3, 67–75 CAS
.
- I. Matias, M. P. Gonthier, P. Orlando, V. Martiadis, L. De Petrocellis, C. Cervino, S. Petrosino, L. Hoareau, F. Festy, R. Pasquali, R. Roche, M. Maj, U. Pagotto, P. Monteleone and V. Di Marzo, J. Clin. Endocrinol. Metab., 2006, 91, 3171–3180 CrossRef CAS PubMed
.
- E. Gatti, D. Noe, F. Pazzucconi, G. Gianfranceschi, M. Porrini, G. Testolin and C. R. Sirtori, Eur. J. Clin. Nutr., 1992, 46, 161–166 CAS
.
- G. Petersen, C. Sørensen, P. C. Schmid, A. Artmann, M. Tang-Christensen, S. H. Hansen, P. J. Larsen, H. H. Schmid and H. S. Hansen, Biochim. Biophys. Acta, 2006, 1761, 143–150 CrossRef CAS PubMed
.
- J. Fu, G. Astarita, S. Gaetani, J. Kim, B. F. Cravatt, K. Mackie and D. Piomelli, J. Biol. Chem., 2007, 282, 1518–1528 CrossRef CAS PubMed
.
- A. Artmann, G. Petersen, L. I. Hellgren, J. Boberg, C. Skonberg, C. Nellemann, S. H. Hansen and H. S. Hansen, Biochim. Biophys. Acta, 2008, 1781, 200–212 CrossRef CAS PubMed
.
- R. Abia, Y. M. Pacheco, E. Montero, V. Ruiz-Gutierrez and F. J. Muriana, Life Sci., 2003, 72, 1643–1654 CrossRef CAS
.
- F. Borrelli and A. A. Izzo, Best Pract. Res. Clin. Endocrinol. Metab., 2009, 23, 33–49 CrossRef CAS PubMed
.
- N. V. Di Patrizio, G. Astarita, G. Schwartz, X. Li and D. Piomelli, Proc. Natl. Acad. Sci. U. S. A., 2011, 108, 12904–12908 CrossRef CAS PubMed
.
- M. I. Friedman, Am. J. Clin. Nutr., 1998, 67, 513S–518S CAS
.
- C. L. Lawton, H. J. Delargy, J. Brockman, F. C. Smith and J. E. Blundell, Br. J. Nutr., 2000, 83, 473–482 CAS
.
- P. Casas-Agustench, P. López-Uriarte, M. Bulló, E. Ros, A. Gómez-Flores and J. Salas-Salvadó, Clin. Nutr., 2009, 28, 39–45 CrossRef CAS PubMed
.
- R. C. Alfenas and R. D. Mattes, Obes. Res., 2003, 11, 183–187 CrossRef PubMed
.
- C. G. MacIntosh, S. H. Holt and J. C. Brand-Miller, J. Nutr., 2003, 133, 2577–2580 CAS
.
- A. Flint, B. Helt, A. Raben, S. Toubro and A. Astrup, Obes. Res., 2003, 11, 1449–1455 CrossRef CAS PubMed
.
- N. Brogden and E. Almiron-Roig, Appetite, 2010, 55, 551–555 CrossRef PubMed
.
- J. M. Brunstrom, N. G. Shakeshaft and N. E. Scott-Samuel, Appetite, 2008, 51, 604–614 CrossRef PubMed
.
- S. Frank, K. Linder, L. Fritsche, M. A. Hege, S. Kullmann, A. Krzeminski, A. Fritsche, P. Schieberle, V. Somoza, J. Hinrichs, R. Veit and H. Preissl, Am. J. Clin. Nutr., 2013, 98, 1360–1366 CrossRef CAS PubMed
.
- K. S. Panickar, Mol. Nutr. Food Res., 2013, 57, 34–47 CAS
.
|
This journal is © The Royal Society of Chemistry 2015 |
Click here to see how this site uses Cookies. View our privacy policy here.