Photodegradation of α-cypermethrin in soil in the presence of trace metals (Cu2+, Cd2+, Fe2+ and Zn2+)
Received
9th August 2014
, Accepted 2nd November 2014
First published on 6th November 2014
Abstract
The influence of trace metals (Cu2+, Zn2+, Cd2+ and Fe2+) on the photodegradation of α-cypermethrin (α-CYM) in agricultural soil was studied. The soil samples were spiked with α-cypermethrin with/without the presence of metal ions, irradiated under a UV irradiation chamber for a regular period of time and analyzed by using HPLC. The dark control sterile and unsterile soil samples spiked with α-cypermethrin and selected trace metals were incubated for the same interval of time at 25 °C. The results obtained indicated that α-cypermethrin photodegradation followed biphasic kinetics. α-cypermethrin photodegradation half-lives (t1/2) were increased to 0.71 and. 4.5 hours from 0.64 hours respectively in the presence of elevated Zn2+ and Cu2+ concentrations. Fe2+ and Cd2+ increased the photodegradation reaction kinetics from −1.078 h−1 to −1.175 h−1 and −1.397 h−1 and varied the t1/2 from 0.64 ± 1.41 to 0.59 ± 2.07 and 0.49 ± 2.01 in the soil. Microbes also affected the degradation of α-cypermethrin in metal contaminated soil. The degradation rate was inhibited in unsterile soil and was found to be in the following order: Cd2+< Zn2+< Cu2+< Fe2+. The degradation/persistence of α-cypermethrin was affected linearly with the increasing soil metal concentrations.
Environmental impact
The study focuses on trace metals induced photodegradation studies of α-cypermethrin – a very persistent pesticide, but still used for numerous crops, in soil. The results of the study were quite remarkable, as Fe2+ and Cd2+ were evidenced to significantly enhance the rate of photodegradation of α-cypermethrin and decrease its half-life while Cu2+ and Zn2+retard the photodegradation rate. Thus balanced trace metal levels in the soils may prove helpful in getting rid of the problems that arise due to very persistent nature of α-cypermethrin.
|
1. Introduction
The use of pesticides to increase the crop production is a common practice all over the world. This practice, however, generates residues that may be noxious to the environment. The accumulation and degradation of these pesticides and their dispersion in the environment depends on the characteristics and overall functions of the ecosystem.1 α-Cypermethrin (α-CYM) is widely used to control the Helicoverpa spp., the major pests of cotton. It is highly hydrophobic as reflected by its low water solubility and high octanol–water partition coefficient (Table 1). Low solubility and high lipoaffinity make it a highly toxic agent to fish and aquatic invertebrates even at very low levels (<0.5 μg L−1, LD50 values).2 Moreover, it is metabolized and eliminated significantly more slowly by fish than by mammals or birds which explains its higher toxicity in fish than in other organisms.3 Generally, the lethality of pyrethroids to fish increases with increasing octanol–water partition coefficients.4 US Environmental protection agency (EPA) has also classified it as a possible human carcinogen.
Table 1 Elementary properties of the pesticide along with its degradation profile in soila
Pesticide |
Mol wt (g) |
Solubility in water (mg L−1) |
Henry constant |
Vapor pressure |
Application rate (g ha−1) |
Chemical stability in the dark |
Aerobic/anaerobic transformation in soil |
Phototransformation soil surface |
Stable degradation products in soil |
Tomlin 2004 (ref. 73).
Hayes and Laws, 1990 (ref. 74).
Kidd and James, 1991 (ref. 75).
US Department of Agriculture,1990 (ref. 76).
Raikwar and Nag, 2011 (ref. 41).
|
α-Cypermethrin C22H19Cl2NO3 |
416.3 |
3.97 (pH = 7), 25 °C 1.25 (DDW) μg L−1 at 20a °C |
2.5b × 10−7 atm m−3 mol−1 |
5.1 × 10−7 nPa at 70c °C 4 × 10−8 mm Hg at 70b °C |
10–15 g ha−1 |
Very stable in neutral and acidic media, hydrolyzed in strongly alkaline media, DT50 (pH 4, 50 °C) stable over 10 days, (pH 7, 20 °C), 101 days (pH 9, 20 °C) 7.3a days |
2–8 weeks/63a,d days |
UV; 56.08 min on clay loam, 94.07 min on loam, sunlight 2.24 days on clay loam, 3.14 on loame |
3-PBAa |
A large proportion of cotton grown is irrigated by drainage water, thus the risk of environmental damage may also be significant.5,6 Moreover, pesticides when applied to soil as insecticides, are not selective and may also kill beneficial soil microorganisms.7,8 α-Cypermethrin is moderately persistent in the soil environment with field half-lives ranging from 4 to 12 weeks.9,10 Due to its high hydrophobic property it causes strong sorption to soil particles, which may cause a build-up of bound residues.11–13
Organic wastes and sludge are commonly applied to the agricultural soils as a source of organic materials and to improve the soil properties.14 However, some studies have shown that the addition of organic manure and N and P fertilizers can affect the pesticide degradation in soils.15–18 Moreover, the use of these materials can lead to the problems associated with their heavy metal contents, especially their successive applications may result in heavy metal accumulation in the soil.
Pyrethroid can undergo photolysis in the soil with half-lives ranging from 5 to 170 days.9 Enhanced concentrations of heavy metals and their strong binding with soil organic matter and clay minerals may lead to their persistence in the soil. This results in a slow dispersion of synthetic pyrethroids and their potential for long-term effects on beneficial soil microorganisms and aquatic species.1,19 Liu et al. (2007) have reported that the presence of Cu2+ (10 mg kg−1) in the soil may inhibit the degradation of cypermethrin (increases t1/2 from 8.1 to 10.9 days) that may be explained as the reduction in activity of bacterial biomass due to Cu2+.20 Some of the metals like iron are known to enhance the degradation of pesticides and reduce their half-lives.21,22 The dissipation/persistence of pesticides in the presence of trace metals was due to their effect on growth rate of the pesticide degrading bacterial population.23,24 For example the carbendazim degrading Variovorax and the diuron degrading Rhodococcus strains were extremely sensitive to cadmium as it decreased their degrading activity even at low concentrations. Cu2+ ions strongly inhibited the degradation process of ethylenethiourea (ETU) which is an important degradation product of ethylenebisdithiocarbamate fungicides while 2,4-D-degradation by Variovorax was highly accelerated by Cu2+ ions. Zinc, copper (Cu2+) and manganese (20–50 mg L−1) accelerated the carbendazim and diuron degradation.23,24 Therefore the goal of the present study was to determine the influence of Cu2+, Cd2+, Fe2+and Zn2+ ions on the dissipation/persistence of α-cypermethrin in soil. The study is important because the trace metal levels in agricultural soil can enhance the catalytic photodegradation of pesticides. So major hazards related to the excessive and repeated use of pesticides in the agricultural soils may be abated in this way.
2. Materials and methods
2.1 Test materials and reference standards
Reference standard of α-cypermethrin (99% purity) was obtained from Sigma-Aldrich, Ltd. (USA). The physical properties of α-cypermethrin according to “OECD25 guidelines for photodegradation of pesticides on soil surface” are listed in Table 1. HPLC grade methanol, acetonitrile, ferrous sulphate, zinc chloride, cadmium chloride, copper sulphate (CuSO4·5H2O) and anhydrous Na2SO4 (analytical Grade) were purchased from Merck (Darmstadt, Germany). Highly pure double distilled water for use during the experiment was prepared with a Milli-Q system from Millipore-Waters Co. (Bedford, MA). Na2SO4 was baked at 500 °C for 4 h before the beginning of the experiment and then stored in an airtight glass bottle until use.
2.2 Soil collection and characterization
Soil (0–20 cm top soil) used in the study was collected from botanical garden of Lahore College for Women University, Lahore. Prior to use, the soil was passed through 2 mm sieve, and maintained at a 75% water holding capacity (WHC) in accordance with the method described elsewhere.26 It was then stored in the dark at 20 °C until analysis. Soil texture was determined by using the hydrometer.27 The physical and chemical properties of the soil sample were measured by using the methods of Saltanpour and Schwab (1977)28 and are summarized in Table 2. Soil was cleaned from pesticides by stirring it with acetone for 24 h (three times) and after decanting the acetone, dried it first at room temperature and then in oven at 105 °C. Soil samples were sterilized by autoclaving for 2 h in a capped 100 mL Erlenmeyer flask at 121 °C.29
Table 2 Physico-chemical properties of the studied soil
Soil type |
Soil texture |
M. C % |
O.M % |
pH (1 : 2) |
CEC (mmol/kg) |
Trace metals (mg kg−1) |
% Clay |
% Sand |
% Silt |
Fe |
Zn |
Cd |
Cu |
Sandy |
4.5 |
87 |
8.5 |
2.34 |
4.62 |
7.4 |
8.3 |
863 |
26.9 |
0.7 |
15.9 |
2.3 Photochemical experimental set up
Irradiation of the soil samples was performed in a self-designed photoreactor, equipped with a 6 W UV tube (Atlas, Linsengericht, Germany), surrounded with a thermopore jacket and water bath that circulated water through the floor of the photolysis chamber for temperature control. An electric fan (3 volt) fitted inside the radiation chamber allowed constant purging of the sample headspace. The spiked soil samples contained in Pyrex Petri plates were continuously irradiated with the UV tube placed 23 cm above. A reference plate containing the unspiked soil sample was also irradiated for same time interval. Soil moisture values were recorded first after every hour and subsequently after every 6 h. If necessary at each sampling, the weight of each soil tray was manually adjusted with distilled water to ensure that the soil was being maintained at its initial weight and moisture content.
2.4 Control sterilized and unsterilized soil dark samples
In the laboratory, control soil samples were subdivided into two groups to investigate the dissipation rates under sterilized and unsterilized dark conditions. The unsterilized samples were used as bioactive controls and were not given any acetone wash. Each portion (10 g, dry weight) of the sample used for sterilization was autoclaved three times (at 24 h apart) for 30 min in a capped 100 mL Erlenmeyer flask at 121 °C. Double de-ionized water was added to the germ-free (autoclaved) original (un-autoclaved) soils, to obtain water content of 75% by WHC. These moistened sub-samples were spiked with the pesticide and then incubated at 25 °C in the dark for 0, 24, 48, 96, 144, 192, 384 and 762 h respectively.
2.5 Standard solution preparations and spiking procedure
The spiking solutions (0.5 μg g−1) of α-cypermethrin were prepared by appropriate dilution of stock solution (5 μg g−1) with acetonitrile. For metal assisted degradation tests, stock solutions of CuSO4·5H2O, FeSO4·7H2O, CdCl2 and ZnCl2 were prepared at concentrations of 1000 mg L−1 in water. These stock solutions were then diluted to 100 mg L−1 for use as a source of external Cu2+, Fe2+, Cd2+ and Zn2+ ions. Soil samples were spiked with α-cypermethrin at the maximum field concentration of 0.5 mg kg−1. The final concentrations of Cu2+ in the soil were set at 15.9 (control treatment), 25.9, 35.9 and 45.9 mg kg−1, for Zn2+ final concentrations were 26.9 (control treatment), 36.9, 46.9 and 56.9 mg kg−1, for Cd+ 0.7 (control), 10.7, 17.7 and 27.7 mg kg−1and for Fe the final concentrations were 863 (control), 873, 883 and 893 mg kg−1 (triplicate sample of each concentration were measured). After soil treatments, the samples were incubated at 25 °C in the dark at a moisture content of 75%. The residual contents in the sterilized and unsterilized samples were monitored at regular intervals as described above.
Soil slurries were prepared by mixing 10.0 g of soil (dry weight) with 7.5 mL of water in Petri plates. The soil was evenly spread across the plate to a depth of 2 mm and then spiked with an appropriate concentration of the pesticide. Subsequently, these soil samples were spiked separately with Cu2+, Cd2+, Fe2+ and Zn2+. To this effect, different volumes of diluted metal solutions were dispensed evenly across the soil surface via micro-syringe, while maintaining the similar moisture level for all the samples. Soil samples were manually shaken to homogenize the samples. The Petri plates were then placed inside the photoreactor and irradiated for 0, 4, 24, 48, 96, 144, 192, 384 and 762 h respectively. The control experiments with no addition of trace metals were carried out simultaneously. After irradiation, the triplicate samples and control were removed from the photoreactor and processed further.
2.6 Pesticide extraction and analysis
The USE method which is an extension of the EPA method 3550C was used for extraction of α-cypermethrin from soil.30 Briefly, the irradiated soil samples were placed in 50 mL Erlenmeyer flasks and extracted with 10 mL of ethyl acetate. These samples were first manually agitated and then exposed thrice to USE in a 100H (80/160 W) ultrasonic bath (Sonorex, Germany) for 15 min. After each extraction, extracts were collected by pouring the extractant through a funnel plugged with a small piece of cotton wool overlaid by a portion of anhydrous sodium sulfate, which had been previously washed with the same solvent. In order to achieve the adequate concentration factor, 10 g aliquot of sample was submitted for extraction and the final extract (ca. 30 mL) was evaporated to dryness using a rotary evaporator and gentle steam of nitrogen without the need for any clean-up procedure and reconstituted in 1 mL acetonitrile. The extraction method showed good efficiency and reproducibility with mean recoveries of 73–92% with standard deviations of lower than 2.4% for the whole procedure.
α-Cypermethrin was analyzed by using the method of Metwally et al., 1997 (ref. 31) and Martnez et al. 1996.32 The HPLC system consisting of an Agilent model 1100 pump, equipped with a DAD detector, an autosampler (model G1313A) and a C8 chromatographic column (Bondsil, 15 × 0.46 cm, 5 urn particle size; Analytichem International) was used for analysis. Acetonitrile/water 75/25 at a flow rate of 1 mL min−1 was used as the mobile phase. The areas of eluted peaks detected at 225 nm were recorded by using a multi-wavelength UV detector Model G 1315B. The retention time of α-cypermethrin under the above conditions was 8.3 min. Calibration was performed each time the samples were analyzed by using external standards and the linear regression analysis was used for quantification. The HPLC procedure was linear in the range 0.01–100 μg mL−1 at 225 nm with the regression coefficient of 0.994 (±0.02) (n = 12); the detection limit was 0.02 μg mL−1 and the limit of quantification was 0.18 μg mL−1
2.7 Data analysis
In the soil, the photolytic decline of a pesticide slows down with time, either due to the adsorption of the pesticide to soil or its movement out of a photic zone. When the lag phase was involved, the hockey-stick model was used for kinetics evaluation. This model consists of two sequential first-order curves. The pesticide concentration initially declines according to first-order kinetics with a rate constant k1. At a certain point in time (referred to as the breakpoint), the rate constant changes to a different value of k2.33
where M = total amount of the chemical present at time t, M0 = total amount of the chemical applied at time t = 0, k1 = rate constant until t = tb, k2 = rate constant from t = tb, tb = breakpoint (time at which rate constant changes)
The tests were carried out in triplicate and the data were expressed as an average effect of the test points.
3. Results and discussion
α-Cypermethrin was chemically stable under neutral soil condition with a half-life of 101 days. It was microbialy degraded with t1/2 of 13 weeks, but its photodegradation was only reported on the soil surface as a thin film (Table 1). No soil incorporated photodegradation study has been reported until now.
3.1 Photodegradation of soil incorporated α-cypermethrin
The presence of unstable groups such as isobutyl and double bonds in the structure of pyrethroids renders them to degrade usually through photolysis, photooxidation and photoisomerization34 in the natural environment. The photodegradation data of α-cypermethrin obtained after irradiation of soil samples under the UV system versus irradiation time are depicted in Fig. 1 as natural logarithmic declines. The data for control samples are also elaborated in the same figure for comparison. The photodegradation and photocatalysis rates of pesticides on soil surfaces under UV light depend on different parameters such as temperature, soil particle sizes, soil depth responsible for photodegradation, and catalyst loads.35 The data revealed that irradiation of moist soil follows biphasic dissipation reaction kinetics in accordance with data provided by the Focus report.33 The present study revealed that α-cypermethrin photodegradation in soil involves two distant phases, first, active phase and second, lag phase. Swarcewicz and Gregorczyk (2012) also reported this type of biphasic degradation pattern for herbicide pendimethalin in soils. The dissipation curves had the initial rapid rate of loss and were slower after 8 days in the dark unsterile control.36
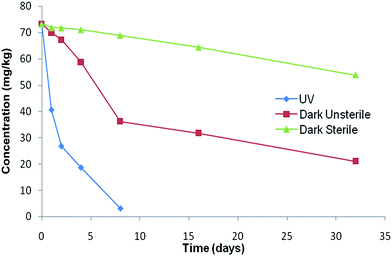 |
| Fig. 1 Photodegradation of soil incorporated α-cypermethrin. | |
UV light increased the reaction rate (k1) from −0.151 h−1 to −1.078 by reducing the active phase from 8 days in unsterile dark to only 1 day thereby enhancing the degradation DT50 from 33.6 days to 0.64 hours (Table 3). Previous studies have reported the half-lives of 8–16 days for the photodegradation of cypermethrin on soil surfaces.37 In sandy soils, its half life was reported to be 2–4 weeks.38 It has been found that cypermethrin degrades more rapidly on sandy loam and sandy clay soils than on clay soils and more rapidly in soils with low organic matter.39,40 Raikwar and Nag (2011) reported the half-life of α-cypermethrin under the UV system to be 0.93 h in clay loam and 1.57 h on loam soil.41 In fact only 8% of radiant solar energy comprises the UV radiation and on reaching the earth's surface, its intensity is further decreased. In the case of lab experiments, the source emits 100% only UV radiation with most of the intensity directed on the samples, that is why lower half lives were observed in the present study and in all other studies carried out under laboratory UV irradiated systems as compared to the studies carried out on sunlit soil surfaces (Fig. 2).
Table 3 Dissipation statistics of degradation of α-cypermethrinb
Pesticide |
Environment |
Trace metal |
Model |
k
1 (h−1) |
r
2
|
t
b (days) |
k
2 (h−1) |
r
2
|
t
1/2 (days) |
DT50 is in hours.
t
b not reached till 32 days of study period. So, DT50 was not possible to be calculated accurately by the HS model.
|
α-Cypermethrin |
UV |
|
HS |
−1.078 |
0.686 |
1 |
−0.236 |
0.916 |
0.64 ± 1.41a |
Dark unsterile |
|
HS |
−0.151 |
0.99 |
8 |
−0.046 |
0.747 |
33.63 ± 1.92 |
Dark sterile** |
|
HS |
−0.024 |
0.994 |
32 |
— |
— |
— |
UV |
Fe2+ |
HS |
−1.175 |
0.94 |
2 |
−0.024 |
0.663 |
0.59 ± 2.07a |
Dark unsterile |
|
HS |
−0.925 |
0.989 |
2 |
−0.035 |
0.855 |
54.03 ± 1.4 |
Dark sterile** |
|
HS |
−0.032 |
0.936 |
32 |
— |
— |
— |
UV |
Zn2+ |
HS |
−0.978 |
0.945 |
2 |
−0.017 |
0.935 |
0.71 ± 1.2a |
Dark unsterile |
|
HS |
−0.701 |
0.955 |
1.2 |
−0.021 |
0.697 |
41.6 ± 2.9 |
Dark sterile** |
|
HS |
−0.022 |
0.96 |
32 |
— |
— |
— |
UV |
Cd2+ |
HS |
−1.397 |
0.813 |
1 |
−0.088 |
0.734 |
0.49 ± 2.01a |
Dark unsterile |
|
HS |
−0.152 |
0.84 |
4 |
−0.042 |
0.918 |
17.7 ± 0.429 |
Dark sterile** |
|
HS |
−0.029 |
0.991 |
32 |
— |
— |
— |
UV |
Cu2+ |
HS |
−0.147 |
0.937 |
8 |
−0.067 |
0.944 |
4.7 ± 1.04a |
Dark unsterile |
|
HS |
−0.282 |
0.908 |
4 |
−0.024 |
0.847 |
49.7 ± 1.12 |
Dark sterile** |
|
HS |
−0.023 |
0.996 |
32 |
— |
— |
— |
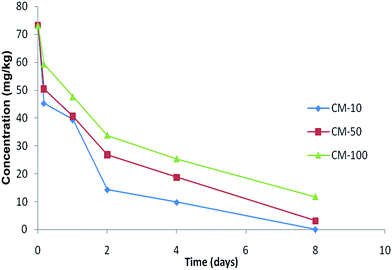 |
| Fig. 2 Effect of concentrations of α-cypermethrin on its photodegradation. | |
Pesticides photodegradation was slow in dry soil as light was unable to penetrate deep into the underneath soil and there were no chances of interaction of light with the pesticide, thus moist soil was used in the present study in accordance with findings of Graebing et al. (2004). α-Cypermethrin is likely to volatilize as indicated by its low Henrys Law constant therefore it can move into the photolytic zone of soil through evapo-condensation cycles where it degraded efficiently on irradiation. Furthermore, indirect photolysis by the hydroxyl radical, singlet oxygen, and other radical species was believed to enhance the rate of photodegradation under moist conditions.42
3.2 Microbial degradation of α-cypermethrin
Microbes also play significant roles in degrading and detoxifying the α-cypermethrin residues in the environment.43,44 α-Cypermethrin was very slowly degraded during the whole study period (t0.5 not reached till 32 days) under dark sterile conditions. The dissipation rate (k1) increased from −0.024 to −0.151 which indicated the role of microbial degradation (Fig. 1 and Table 3). Tallur et al. (2007) studied that Micrococcus sp. present in soil utilized cypermethrin as a sole source of carbon leading to hydrolysis of the ester linkage to yield 3-phenoxy benzoate.45 Sterilization eliminates the microbial population of the soil and thus increases the persistence of the pesticide. The α-cypermethrin dissipation in the sterile soil in the dark may be attributed to the chemical dissipation because the possibility of photodegradation was ruled out by incubating the samples in the dark.43 In the soil, the chemical dissipation of cypermethrin takes place through hydrolysis whereby the ester linkage is first hydrolysed leading to the formation of 3-phenoxybenzoic acid (PBA) and cyclopropanecarboxylic acid derivatives,46 principally, 3-(2,2-dichlorovinyl)-2,2-dimethyl cyclopropanecarboxylic acid (DCVA).43 This dissipation route was very lethargic and had DT50 longer than 60 days (Table 1). Although it is a biodegradable pesticide, the microbial release of bound residues occurs rather slowly.47
3.3 Effect of trace metals on photodegradation
The photodegradation rates of certain pesticides may be enhanced in the presence of certain metals in the soil by altering the enzymatic activity of soil microorganisms.48–52 Similarly, trace metals are also known to inhibit the enzymatic reactions of microorganisms by complexation with the substrates, combination with the protein-active sites of the enzymes, or reaction with the enzyme–substrate complex. Thus bacterial biomass activity may also be inhibited in metal polluted soils. Kools et al. (2005) have reported a positive correlation between glyphosate degradation rates and soil metal pollution (Kools et al., 2005).
3.3.1 Effect of Cu2+on α-cypermethrin.
Photodegradation rates (k1 and k2) of α-cypermethrin were decreased from −1.078 h−1 and −0.236 h−1 to −0.147 h−1 and −0.067 h−1 when 10 mg kg−1 of Cu2+ was added to the soil as evidenced by an increase in t1/2 from 0.64 to 4.5 hours at p < 0.05 (Table 3). The percent photodegradation of α-cypermethrin in the presence of 25.9 mg kg−1 (Co + 10 mg kg−1) decreased from 95.7 to 61.7% after 8 days of continuous UV irradiation. This retarding effect became pronounced when Cu2+ concentration was increased to 45.9 mg kg−1 (Co + 30 mg kg−1), the % photodegradation was observed to be reduced to only 50.5%. Copper is known to enhance the photodegradation of pyrethroids in the presence of UV light.1,52 According to Sykora (1997) copper compounds may act as catalysts for photodegradation of various pollutants in irradiated systems. The pollutants like α-cypermethrin may act as ligands in the coordination sphere of the copper and a Cu2+–Cu1+ photocatalytic redox cycle was believed to occur in copper amended solutions. This catalytic effect might also arise due to secondary thermal reactions of the active species produced photochemically from the copper complex.53 The degradation rate of pesticides in the soil was closely related to its availability to the enzymatic systems of microorganisms.54,55
The dissipation rates of α-cypermethrin decreased significantly at p > 0.05 in unsterilized soil during 32 days of incubation when compared with the dark control sterile treatments (Fig. 5a). The half-life of α-cypermethrin was observed to be increased from 33.6 to 49.7 days in unsterile control treatment that indicated that copper affects the activity of soil microbes involved in degradation of the pesticide (Table 3). The results of the present study were compatible with the findings of Liu et al. (2007), who reported that the persistence of α-cypermethrin was increased from 8.1 to 10.9 days with the presence of 10 mg kg−1 copper ion in unsterile soil. The observed degradation might be due to the fact that metals react with the sulfhydral group of enzymes thereby leading to inhibition of their enzyme activity.1 Ellis et al. (2001) and Fernandes et al. (2005) found that copper-tolerant communities may have replaced the soil microorganisms that were able to co-metabolize the pyrethroids.56,57 Moreover, soil microbial community was adversely impacted by the presence of elevated concentrations of Cu2+. Copper ions have been reported to strongly inhibit the degradation process of ethylthiourea (ETU).24 Different initial concentrations were observed in Fig. 3. The observation evidenced that the addition of Cu2+ caused the persistence of α-cypermethrin in the soil just after its addition. Fig. 4 also depicted an initially high rate of degradation that later on was reduced after some days and then stalled completely. This fact was interpreted on the basis that desorption controls the biodegradation process.58 The sorption of the substance determines its availability for microbial degradation. The sorbed chemicals are less accessible to microorganisms that utilize exclusively or preferentially chemicals in solution. Thus with passage of time, the sorbed quantity of pesticide is increased and thus rate of degradation is reduced. It is generally accepted that sorption limits the degradation of pesticides by reducing their partitioning into the soil.
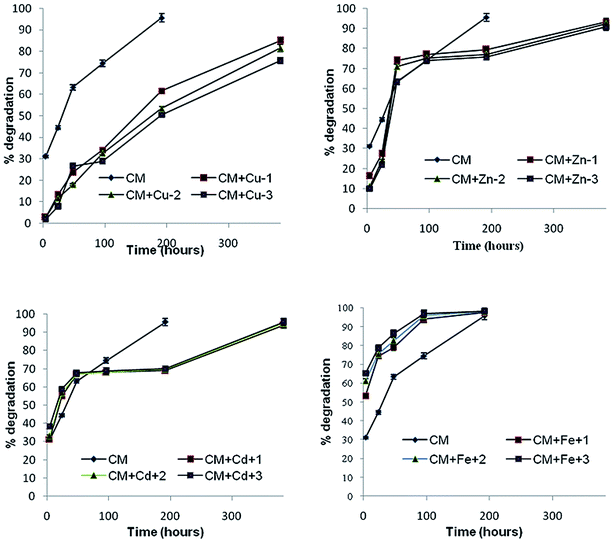 |
| Fig. 3 Effect of metal concentrations on photodegradation of α-cypermethrin. | |
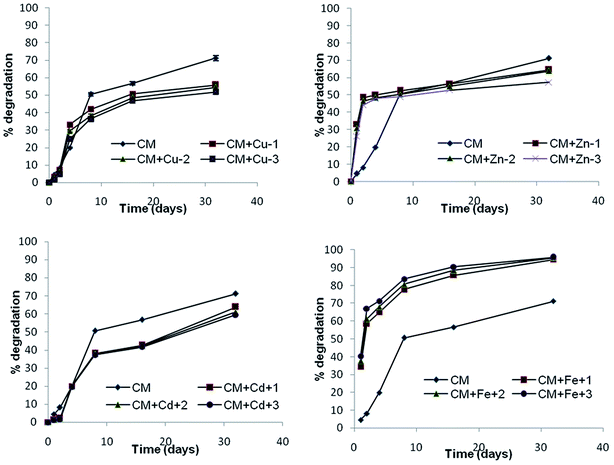 |
| Fig. 4 Effect of metal concentrations on microbial degradation of α-cypermethrin. | |
The Cu2+ metal also affects the abiotic degradation of α-cypermethrin and thus exhibits an inhibitory effect. The inhibitory effect was more pronounced when the Cu2+ concentration was increased up to 45.9 mg kg−1. The percent degradation of α-cypermethrin decreased from 26.5% to 20.5% (Fig. 5c). These findings pointed toward the fact that the α-cypermethrin dissipation in soils containing low concentrations of added Cu2+ was more dependent on biological dissipation than chemical dissipation, but when high concentrations of added Cu2+ were present in soils it depended on chemical dissipation.
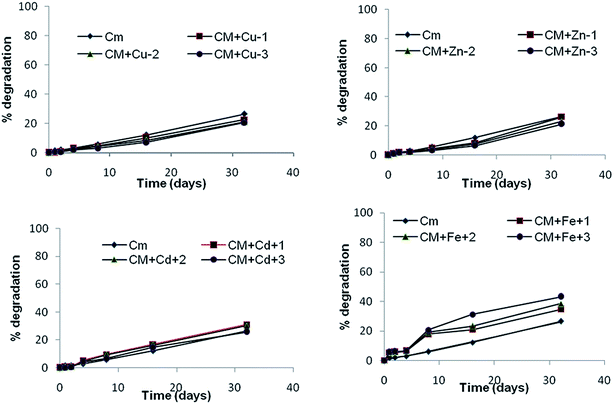 |
| Fig. 5 Effect of metal concentrations on abiotic degradation of α -cypermethrin. | |
3.3.2 Effects of Zn2+ on α-cypermethrin.
Zn2+ addition decreased the degradation of α-cypermethrin but the inhibitory effect was less severe than for Cu2+ (Fig. 3c and Table 3). The photodegradation of α-cypermethrin was decreased from 95.69 to 79.5% after 8 days of continuous UV irradiation. UV photodegradation rate constants k1 and k2 decreased from −0.701 h−1 and −0.021 h−1 to −1.078 h−1 and −0.236 h−1 respectively. The degree of inhibition was increased with an increase in the soil Zn concentration from 16.9 mg kg−1 to 36.5 mg kg−1 (i.e. from Co + 10 mg kg−1 to Co + 30 mg kg−1). Different initial concentrations were observed in Fig. 3, evidencing that the addition of Zn2+ in the soil caused the persistence of α-cypermethrin just after its addition. The % dissipation was decreased from 64.55 to 57.26% after 32 days of continuous incubation (Fig. 4b). This might be due to a change in the functional diversity of the microbial community. Under the Zn stress i.e., high Zn2+ concentrations, some soil microbial populations were shifted from sensitive to less sensitive areas and hence affected the soil microbial population and weakened the microbial activities (Gu et al., 2010). Kamitani et al. (2006) reported that there was a positive correlation between available Zn2+ content of soil and soil metabolic quotient, and a negative correlation between available Zn2+ content and microbial biomass, carbon microbial biomass, nitrogen and the microbia1 quotient.59 No significant difference was observed in dissipation of α-cypermethrin under dark sterile conditions at p > 0.05 in the presence of soil Zn2+ load. It was therefore suggested that microorganisms are the major agents that are involved in the dissipation of pyrethroids in the soil environment.60
3.3.3 Effects of Cd2+ on α-cypermethrin.
The photodegradation reaction rate k1 of α-cypermethrin in soil was observed to increase from −1.078 h−1 to −1.397 h−1 at p < 0.05. This resulted in decrease in persistence of α-cypermethrin from 0.64 ± 1.41 to 0.49 ± 2.01 hours under the UV-irradiation system. The results were consistent with the findings of Barakat et al. (2013), who reported the successful elimination of the harmful pesticide (methomyl) by using Cd based photocatalysts under the sunlight radiation within a very short time with a removal capacity being 1000 mg pesticide per gram of the photocatalyst.61
Cd2+ also decreased the half-life of α-cypermethrin under dark unsterile conditions from 33.63 ± 1.92 to 17.7 ± 0.43 days (Table 3). It is explained on the basis of the fact that certain pesticide degrading strains of bacteria are extremely sensitive to cadmium. Cadmium decreases their degrading activity even at low concentrations thus increasing the half life of the pesticide.24 In fact both the biotic and abiotic dissipation of pyrethroids might occur in soil simultaneously.62 Under sterile conditions in soils, chemical dissipation becomes more important, in the presence of high concentrations of added Cd2+.
3.3.4 Effects of Fe2+ on α-cypermethrin.
Iron is one of the major elements present in the soil mostly in the forms of hydroxides/oxides/chlorides. Generally the most dominant oxidation state is Fe3+ and when reducing conditions (like subsurface environment) prevail, iron exists as Fe2+ (ref. 63). Iron is known to accelerate the photolysis of pesticides through the photosensitizing effect.64–68 Rafique et al. (2014) evidenced that a 3-fold increase in percent degradation of imidacloprid was observed in moist soils by the catalytic addition of iron to soil.52 The present study also evidenced an accelerated photodegradation of α-cypermethrin under the UV chamber by the addition of iron. The reaction rate was observed to increase from −1.078 h−1 to −1.175 h−1. Hence, a decrease in the half-life was observed from 0.61 ± 1.41 to 0.59 ± 2.07 hours. These results are in agreement with the findings of several other authors who reported that Fe2+ catalyzed the photodegradation of several pesticides.21,52,69 The degradation of α-cypermethrin in soil was more efficient when soil iron levels were enhanced. It was degraded up to approximately 94 to 96% of initial concentration after 4 days of continuous UV irradiation in the presence of Fe2+ (Co + 10 mg kg−1 and Co + 30 mg kg−1) as compared with control of 74%. This enhanced effect was the result of direct Fe2+ catalyzed photodegradation or indirect photolysis due to reaction of Fe2+ with the OH− radical from moist soil.21 Different initial concentrations of pesticides are observed in Fig. 3, before and after the addition of Fe2+ in the soil samples that evidenced the fact that the addition of Fe2+ in the soil caused the instant degradation of α-cypermethrin in the soil.
Soil microbes are more efficient in degrading α-cypermethrin in the presence of higher Fe levels (CFe + 30 mg kg−1) as evidenced by % degradation which increased from 71.2% to 96% in the presence of 30 mg kg−1 Fe2+ after 32 days of incubation. The zero valent iron (Fe0) has already been used as a remedial tool to enhance the degradation of HCHs and DDX in soil.70 The soil Fe levels also affected the abiotic dissipation of α-cypermethrin in soil with increase in the reaction rate to −0.032 from −0.024 at p < 0.05 (Table 3). The % degradation enhanced from 26.6 to 46.1% after 32 days of incubation in the dark at 25 °C. Singhal et al. (2012) reported the degradation of malathion by zero-valent Fe nano-particles.71 When it was added to soil under anaerobic conditions, corrosion (oxidation) of the iron might be effectively coupled to reductive dechlorination and nitro group reduction.72
4. Conclusions
It is concluded that photodegradation half-life of α-cypermethrin was retarded in the presence of elevated concentrations of Cu2+ and Zn2+. Cu2+ was evidenced to possess a slightly greater inhibition effect than Zn2+ and increased the t1/2 from 0.64 hours to 4.5 and 0.71 hours, respectively. These metals also retarded the microbial degradation of α-cypermethrin. The proliferated soil Fe2+ and Cd2+ levels, however, enhanced the photo and microbial degradation of α-cypermethrin.
Acknowledgements
The authors are highly thankful to the financial support provided by the Higher Education commission of Pakistan under the Indigenous 5000 Ph.D. Fellowship scheme. The authors are also thankful to Dr Matten Abbas and Abdul Muqeet khan for providing the support for HPLC analysis.
References
- T. F. Liu, S. Cheng, T. N. Hongjun, Y. Shao-gui and C. X. Chen, Effect of copper on the degradation of pesticides cypermethrin and cyhalothrin, J. Environ. Sci., 2007, 19, 1235–1238 CrossRef CAS.
- S. P. Bradbury and J. R. Coats, Toxicokinetics and toxicodynamics of pyrethroid insecticides. Fish, Environ. Toxicol. Chem., 1989, 8, 373–380 CrossRef CAS.
-
U.S. Environmental Protection Agency, Pesticide Fact Sheet Number 199: Cypermethrin. Office of Pesticide Programs, Registration Div., US EPA, Washington, DC, 1989 Search PubMed.
- K. Haya, Toxicity of pyrethroid insecticides to fish, Environ. Toxicol. Chem., 1989, 8, 381–391 CrossRef CAS.
-
J. Lhost and M. L'Hotellier, Effects of Deltamethrin on the Environment, Deltamethrin Monograph, Roussel Uclaf, Paris, 1982, vol. 9, pp. 321–353 Search PubMed.
- S. Zhou, D. Changqun, X. Wang, W. Hang, G. Michelle, Z. Yu and H. Fu, Assessing cypermethrin-contaminated soil with three different earthworm test methods, J. Environ. Sci., 2008, 20(11), 1381 CrossRef CAS.
-
F. Matsumura, Toxicology of insecticides, 503 Seiten, Plenum Press, New York und. London, 1975, DOI:wiley.com/10.1002/food.19770210222.
-
R. Edwards and P. Millburn, The metabolism and toxicity of insecticides to fish, in Progress in Pesticide Biochemistry and Toxicology, ed. D. H. Hutson and T. R. Roberts, Wiley, New York, 1985, Vol. 5, insecticides, pp. 249–274 Search PubMed.
- D. A. Laskowski, Physical and chemical properties of pyrethroids, Rev. Environ. Contam. Toxicol., 2002, 174, 49–170 CAS.
- X. Z. Gu, G. Y. Zhang, L. Chen, R. L. Dai and Y. C. Yu, Persistence and dissipation of synthetic pyrethroid pesticides in red soils from the Yangtze River Delta area, Environ. Geochem. Health, 2008, 30, 67–77 CrossRef CAS PubMed.
- T. R. Roberts and M. E. Standen, Further studies of the degradation of the pyrethroid insecticide cypermethrin in soils, Pestic. Sci., 1981, 12, 285–296 CrossRef CAS.
-
J. P. Leahey, Metabolism and Environmental Degradation in the Pyrethroid Insecticides, ed. J. P. Leahey, Taylor and Francis, London, 1985, pp. 263–342 Search PubMed.
- H. Jin and G. R. B. Webster, Persistence, penetration, and surface availability of cypermethrin and its major degradation products in elm bark, J. Agric. Food Chem., 1998, 46, 2851–2857 CrossRef CAS.
- A. Baran, G. Cayci, C. Kutuk and R. Hartmann, The effect of grape marc as growing medium on growth of hypostases plant, Bioresour. Technol., 2001, 78, 103–106 CrossRef CAS.
- E. Topp, L. Tessier and E. G. Gregorich, Dairy manure incorporation stimulates rapid atrazine mineralization in an agricultural soil, Can. J. Soil Sci., 1996, 76, 403–409 CrossRef CAS PubMed.
- K. H. Han, C. S. Kim, H. M. Ro, S. M. Lee and S. H. Yoo, Degradation of ethoprophos in three physicochemically different soils treated with composted manure and chemical fertilizer, Bull. Environ. Contam. Toxicol., 2003, 70, 520–526 CrossRef CAS PubMed.
- A. B. Caracciolo, G. Giuseppe, P. Grenni, C. Cremisini, R. Ciccoli and C. Ubaldi, Effect of urea on degradation of terbuthylazine in soil, Environ. Toxicol. Chem., 2005, 24, 1035–1040 CrossRef CAS.
- X. Wenjun and J. Zhou, Cypermethrin persistence and soil properties as affected by long term fertilizer management, Acta Agric. Scand., Sect. B, 2008, 58(4), 314–321 Search PubMed.
- S. J. Maund, M. J. Hamer, M. C. Lane, E. Farrelly, J. H. Rapley, U. M. Goggin and W. E. Gentle, Partitioning, bioavailability, and toxicity of the pyrethroid insecticide cypermethrin in sediments, Environ. Toxicol. Chem., 2002, 21, 9–15 CrossRef CAS.
- K. E. Giller, E. Witter and S. P. Mcgrath, Toxicity of heavy metals to microorganism and microbial processes in agricultural soils: A review, Soil Biol. Biochem., 1998, 30(10–11), 1389–1414, DOI:10.1016/S0038-0717(97)00270-8.
- H. Zheng and C. Ye, Photodegradation of Acetochlor and Butachlor in waters Containing Humic Acid and Inorganic Ion, Bull. Environ. Contam. Toxicol., 2001, 67(4), 601–608 CrossRef CAS.
- N. Rafique, S. R. Tariq and M. Abas, Effect of Fe2+ amendment on photodegradation kinetics of imidacloprid in moist soil, Environ. Earth Sci., 2014, 71, 2869–2874, DOI:10.1007/s12665-013-2663-x.
-
C. s. Vágvölgyi, B. Škrbić, J. Krisch and L. Manczinger, Remediation of pesticide-polluted soils by microbial bioaugmentation, in 12th Regional Conference on Environment and Health, Novi Sad, Serbia, 14/15 Sep 2010 Search PubMed.
-
B. Škrbić, C. s. Vágvölgyi, A. Tjapkin, Z. Predojević and J. Krisch, L. Manczinger, Development of xenobiotic-degrading bioaugmentation products-BIOXEN project. International Conference on Science and Technique in the agri-food business, ICoSTAF, Szeged, 3–4th November 2010, Abstracts 2010 Search PubMed.
- OECD Draft guidelines “Phototransformation of chemicals on soil surfaces” 2002.
- M. P. Frank, P. W. Graebing and J. S. Chib, Effect of soil moisture and sample depth on pesticide photolysis, J. Agric. Food Chem., 2002, 50, 2607–2614 CrossRef CAS PubMed.
-
S. D. Bao, Soil and Agricultural Chemistry Analysis, China Agricultural Press, Beijing, 2000, pp. 25–114 Search PubMed.
-
A new soil test for simultaneous extraction of macro and micro-nutrients in alkaline soils, Communications in Soil Science and Plant Analysis, ed. P. N. Soltanpour and A. P. Schwab, 1977, vol. 8, pp. 195–207 Search PubMed.
- S. Darbar and A. Razavi, Lakzian, Evaluation of chemical and biological consequences of soil sterilization methods, Caspian J. Environ. Sci., 2007, 5(2), 87–91 Search PubMed.
- C. Goncalves and M. F. Alpendurad, Assessment of pesticide contamination in soil samples from an intensive horticulture area, using ultrasonic extraction and gas chromatography–mass spectrometry, Talanta, 2005, 65, 1179–1189 CrossRef CAS PubMed.
- M. E.-S. Metwally, M. S. Osmanb and R. Al-Rushaid, A high-performance liquid chromatographic method for the determination of cypermethrin in vegetables and its application to kinetic studies after greenhouse treatment, Food Chem., 1997, 59(2), 283–290 CrossRef CAS.
- G. M. Martinez, V. J. Martinez, F. A. Garrido and G. Garcia, J. Chromatogr. A, 1996, 727, 39–46 CrossRef.
-
FOCUS, Guidance document on estimating persistence and degradation kinetics from environmental fate studies on pesticides in EU registration. Report of the FOCUS Work Group on Degradation Kinetics, EC Document Reference Sanco/10058/2005, Version 1.0, European Commission, Brussels, 2006 Search PubMed.
- G. Bi, S. Z. Tian and Z. G. Feng, Study on the sensitized photolysis of pyrethroids: kinetic characteristic of photooxidation by singlet oxygen, Chemosphere, 1996, 32(7), 1237–1243 CrossRef.
- L.-h. Zhang, L. Pei-jun, G. Zong-qiang and A. Adeola, Photochemical behavior of benzo (a) pyrene on soil surfaces under UV light irradiation, J. Environ. Sci., 2006, 18(6), 1226–1232 CrossRef CAS.
- M. K. Swarcewicz and A. Gregorczyk, The effects of pesticide mixtures on degradation of pendimethalin in soils, Environ. Monit. Assess., 2012, 184, 3077–3084 CrossRef CAS PubMed.
-
M. H. Walker and L. H. Keith, EPA's Pesticide Fact Sheet Database, Lewis Publishers, Chelsea, MI, 1992 Search PubMed.
- R. A. Chapman, C. M. Tu, C. R. Harris and C. Cole, Persistence of five pyrethroid insecticides in sterile and natural, mineral and organic soil, Bull. Environ. Contam. Toxicol., 1981, 26, 513–519 CrossRef CAS.
-
U.S. Environmental Protection Agency, Pesticide Fact Sheet Number 199: Cypermethrin, Office of Pesticide Programs, Registration Div., US EPA, Washington, DC, 1989 Search PubMed.
- N. Takahashi, N. Mikami, T. Matsuda and J. Miyamoto, Photodegradation of the pyrethroid insecticide cypermethrin in water and on soil surface, J. Pestic. Sci., 1985, 10, 629–642 CrossRef CAS.
- M. K. Raikwar and S. K. Nag, Photolysis of alphacypermethrin as thin film on soil surface under UV and natural light, GERF Bull. Biosci., 2011, 2(2), 6–10 Search PubMed.
- P. Graebing and J. S. Chib, Soil Photolysis in a Moisture- and temperature-controlled environment. insecticides, J. Agric. Food Chem., 2004, 52, 2606–2614 CrossRef CAS PubMed.
-
D. D. Kaufman, B. A. Russell, C. S. Helling and A. J. Kayser. Movement of cypermethrin, decamethrin, permethrin, and their degradation products in soil, Journal of Agricultural and Food Chemistry, American Chemical Society, Washington D.C., 1981, pp. 239–245 Search PubMed.
- X.-z. Gu, L. Zhang, G.-y. Zhang, C.-x. Fan and L. Chen, Preliminary Evidence that Copper and Zinc Inhibits the Dissipation of Synthetic Pyrethroid in Red Soil, Water, Air, Soil Pollut., 2010, 212, 345–355 CrossRef CAS.
- P. N. Tallur, V. B. Megadi and H. Z. Ninnekar, Biodegradation of Cypermethrin by Micrococcus sp. strain CPN 1, Biodegradation, 2008, 19, 77–82 CrossRef CAS PubMed.
- S. Sakata, M. Nobuyoshi, T. Matsuda and J. Miyamoto, Degradation and leaching behavior of the pyrethroid insecticide cypermethrin in soils, J. Pestic. Sci., 1986, 11, 71–79 CrossRef CAS.
- B. Gevao, K. T. Semple and K. C. Jones, Bound pesticide residues in soils: a review, Environ. Pollut., 2000, 108, 3–14 CrossRef CAS.
- D. M. Helal, S. M. Imam and H. F. Khalifa, Interaction of pesticides with humic compounds and their metal complexes, Radiochemistry, 2006, 48(4), 419–425 CrossRef.
- S. A. E. Kools, M. V. Roovert and C. A. M. V. Gestel, Glyphosate degradation as a soil health indicator for heavy metal polluted soils, Soil Biol. Biochem., 2005, 37(7), 1303–1307 CrossRef CAS PubMed.
- C. Saron, F. Zulli, M. Giordano and M. I. Felisberti, Influence of copper- phthalocyanine on the photodegradation of polycarbonate, Polym. Degrad. Stab., 2006, 91, 3301–3311 CrossRef CAS PubMed.
- G. Q. Shen, Y. T. Lu and Q. X. Zhou, Interaction of polycyclic aromatic hydrocarbons and heavy metals on soil enzyme, Chemosphere, 2005, 61(8), 1175–1182 CrossRef CAS PubMed.
- N. Rafique, S. R. Tariq and F. Zaidi, Photocatalytic degradation studies of imidacloprid and lambda-cyhalothrin by copper metal, IJEPCM, 2012, 4(2), 139–145 Search PubMed.
- J. Sykora, Photochemistry of copper complexes and their environmental aspects, Coord. Chem. Rev., 1997, 159, 95–108 CrossRef CAS.
- H. Lutnicka, T. Bogacka and L. Wolska, Degradation of pyrethroids in an aquatic ecosystem model, Water Res., 1999, 33(16), 3441–3446 CrossRef CAS.
- M. E. Sanchez, I. B. Estrada and O. Martnez, Influence of the application of sewage sludge on the degradation of pesticides in the soil, Chemosphere, 2004, 57(7), 673–679 CrossRef CAS PubMed.
- R. J. Ellis, B. Neish and M. W. Trett, Comparison of microbial and meiofaunal community analyses for determining impact of heavy metal contamination, J. Microbiol. Methods, 2001, 45, 171–185 CrossRef.
- S. A. P. Fernandes, W. Bettiol and C. C. Cerri, Effect of sewage sludge on microbial biomass, basal respiration metabolic quotient and soil enzymatic activity, Appl. Soil Ecol., 2005, 30, 65–77 CrossRef PubMed.
-
D. G. Williamson, R. C. Loehr and Y. Kimura, Measuring release and biodegradation kinetics of aged hydrocarbons from soils, in In Situ and on Site Bioremediation. ed. B. C. Alleman and A. Leeson, Battelle Press, Columbus, OH, 1997, Vol. 5, pp. 605–610 Search PubMed.
- T. Kamitani, H. Oba and N. Kaneko, Microbial biomass and tolerance of microbial community on an aged heavy metal polluted floodplain in Japan, Water, Air, Soil Pollut., 2006,(172), 185–200 CrossRef CAS.
- A. K. Fenlon, K. Andreou, C. K. Jones and T. K. Semple, The extractability and mineralisation of cypermethrin aged in four UK soils, Chemosphere, 2011, 82, 187–192 CrossRef PubMed.
- N. A. Barakat, M. M. Nassar, T. E. Farrag and M. S. Mahmoud, Effective photodegradation of methomyl pesticide in concentrated solutions by novel enhancement of the photocatalytic activity of TiO2 using CdSO4 nanoparticles, Environ. Sci. Pollut. Res. Int., 2014, 21(2), 1425–1435, DOI:10.1007/s11356-013-2027-9.
- H. Z. Huang, C. D. Fleming, K. Nishi, M. R. Redinbo and B. D. Hammock, Stereoselective hydrolysis of pyrethroid-like fluorescent substrates by human and other mammalian liver carboxylesterases, Chem. Res. Toxicol., 2005, 18, 1371–1377 CrossRef CAS PubMed.
- L. Tajeddine, H. Mountacer and M. Sarrakha, Effect of iron and humic acid on photodegradation of some pesticides adsorbed on clay surfaces, Arabian J. Chem., 2010, 3, 73–77 CrossRef CAS PubMed.
- S. D. Comfort, P. J. Shea, T. A. Machacek, H. Gaber and B. T. Oh, Field-scale remediation of a metolachlor-contaminated spill site using zero valent iron, J. Environ. Qual., 2001, 30, 1636–1643 CrossRef CAS.
- T. Eggen and A. Majcherczyk, Effects of zerovalent iron (Fe0) and temperature on the transformation of DDT and its metabolites in lake sediment, Chemosphere, 2006, 62, 1116–1125 CrossRef CAS PubMed.
- G. Valeria, G. Ferenc, A. Biljana, N. Banic and Z. Papp, Comparison of different iron-based catalysts for Photocatalytic removal of imidacloprid, React. Kinet., Mech. Catal., 2010, 99, 225–233 Search PubMed.
- S. R. Tariq, N. Rafique, S. Kiran and A. M. Khan, Photo-induced degradation of emamectin benzoate: effect of iron amendments and solvent system, Environ. Earth Sci., 2014,(4), 983–988 CrossRef CAS PubMed.
- R. Salah, M. Gilles and S. Mohamed, Highly efficient photodegradation of the pesticide metolcarb induced by Fe complexes, Environ. Chem. Lett., 2006, 4(4), 213–217 CrossRef.
- L. E. Kochany, Degradation of nitrobenzene and nitrophenols in homogeneous aqueous solution. Direct photolysis versus photolysis in the presence of hydrogen peroxide and the Fenton reagent, Water Qual. Res. J. Can., 1992, 27, 97–122 Search PubMed.
- S. C. Yang, M. Lei, T. B. Chen, X. Y. Li, Q. Liang and C. Ma, Application of zerovalent iron (Feo) to enhance degradation of HCHs and DDX in soil from a former organochlorine pesticides manufacturing plant, Chemosphere, 2010, 79(7), 727–732 CrossRef CAS PubMed.
- R. K. Singhal, B. Gangadhar, H. Basu, V. Manisha, G. R. K. Naidu and A. V. R. Reddy, Remediation of Malathion contaminated soil using Zero valent Iron Nano-Particle, Am J Anal Chem, 2012, 3, 76–82, DOI:10.4236/ajac.2012.31011.
- A. Reddy, V. Bhaskar, V. Madhavi, R. K. Gangadhara and G. Madhavi, Remediation of Chlorpyrifos-contaminated soils by laboratory-synthesized zero-valent nano iron particles: Effect of pH and Aluminium salts, J. Chem., 2013, ID 521045, 7 Search PubMed.
-
The Pesticide Manual, ed. C. Tomlin, Crop Protection Publications, Surrey, U.K., 12th edn, 2000 Search PubMed.
-
Handbook of Pesticide Toxicology, Classes of Pesticides, ed. W. J. Hayes and E. R. Laws, Academic Press, Inc., NY, 1990, vol. 3 Search PubMed.
-
The Agrochemicals Handbook, ed. H. Kidd and D. R. James, Royal Society of Chemistry Information Services, Cambridge, UK, 3rd edn, 1991, pp. 2–13, (as updated) Search PubMed.
- US Department of Agriculture, Soil Conservation Service. SCS/ARS/CES Pesticide Properties Database: Version 20 (Summary) USDA—Soil Conservation Service, Syracuse, 1990.
|
This journal is © The Royal Society of Chemistry 2015 |