DOI:
10.1039/C5CE01364J
(Highlight)
CrystEngComm, 2015,
17, 7461-7468
Divergent 4,2′:6′,4′′- and 3,2′:6′,3′′-terpyridines as linkers in 2- and 3-dimensional architectures
Received
12th July 2015
, Accepted 13th August 2015
First published on 3rd September 2015
Abstract
4,2′:6′,4′′-Terpyridine (4,2′:6′,4′′-tpy) and 3,2′:6′,3′′-terpyridine (3,2′:6′,3′′-tpy) typically coordinate through the outer pyridine rings, leaving the central ring non-coordinated. They therefore present divergent sets of N,N′-donor atoms and are ideal linkers for connecting metal nodes in coordination polymers and networks. This Highlight illustrates the strategies that are currently used to encourage the formation of 2- and 3-dimensional architectures as opposed to 1-dimensional chains. Functionalization in the tpy 4′-position with substituents such as pyridyl or carboxylate that can bind metal ions is one strategy. The second is to increase the coordination number of the metal centre. The third is to design ligands that contain multiple 4,2′:6′,4′′-tpy or 3,2′:6′,3′′-tpy domains.
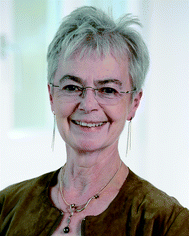
Catherine E. Housecroft
| Catherine E. Housecroft is Professor of Chemistry at the University of Basel. She is co-director of a highly active research group with her husband, Professor Edwin C. Constable, and has a broad range of interests spanning structural, organometallic, coordination and materials chemistries. Current research focuses on the application of coordination chemistry to sustainable energy and functional coordination polymers; she is actively involved in the Swiss Nanoscience Institute and the National Centre for Competence in Research for Molecular Systems Engineering. Catherine has published ca. 400 research papers and 60 review articles, in addition to numerous chapters in edited books and reference works. She is an internationally recognized author of undergraduate textbooks: Chemistry (coauthored with Edwin Constable) and Inorganic Chemistry (originally with the late Alan Sharpe) are both in their fourth editions and Inorganic Chemistry has been translated into six languages. |
Introduction
2,2′:6′,2′′-Terpyridine (2,2′:6′,2′′-tpy) presents a bis-chelating donor set and coordination complexes are dominated by octahedral {M(2,2′:6′,2′′-tpy)2} domains which, if bearing peripheral donor groups, function as ‘expanded ligands’ (Scheme 1) in supramolecular assemblies including coordination polymers.1 The coordination chemistry of 2,2′:6′,2′′-tpy is abundantly represented, whereas the coordination behaviours of the other 47 isomers of terpyridine have attracted less attention. Two isomers especially demand exploration because of their ease of synthesis and functionalization in the 4′-position:2,3 4,2′:6′,4′′-terpyridine (4,2′:6′,4′′-tpy) and 3,2′:6′,3′′-terpyridine (3,2′:6′,3′′-tpy). Both ligands typically coordinate metals through only the outer pyridine rings, and Scheme 2 illustrates that, while the directionality of the donor atoms in 4,2′:6′,4′′-tpy is fixed, that in 3,2′:6′,3′′-tpy is varied by virtue of rotation about the inter-ring C–C bonds. In terms of using these ligands as linkers in the construction of coordination polyers, this distinction between 4,2′:6′,4′′-tpy and 3,2′:6′,3′′-tpy presents interesting design issues, and it might appear that assemblies directed using 4,2′:6′,4′′-tpy may be more predictable than those using 3,2′:6′,3′′-tpy. However, even with its apparent preorganization, 4,2′:6′,4′′-tpy can yield surprises when coordinating metals as is illustrated below.
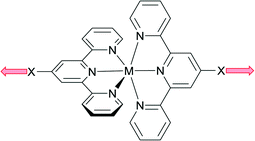 |
| Scheme 1 Divergent metal binding mode of {M(2,2′:6′,2′′-tpy)2} unit where X is a donor group, e.g. pyridyl, (spacer)-CO2−. | |
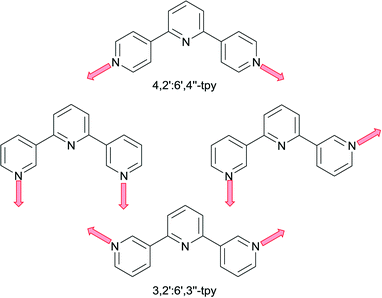 |
| Scheme 2 Divergent N,N′-metal binding mode of 4,2′:6′,4′′-tpy, and variable binding modes of 3,2′:6′,3′′-tpy accessible through inter-ring bond rotation. | |
The coordination chemistry of 3,2′:6′,3′′-terpyridines remains largely unexplored. A search of the Cambridge Structural Database (CSD v. 5.36 with updates to May 2015,4 using Conquest v. 1.175), gives 205 hits for 4,2′:6′,4′′-tpy and only 25 hits for 3,2′:6′,3′′-tpy (ligands and complexes). The first coordination polymer of 4,2′:6′,4′′-tpy was reported in 19986 and since the potential of this divergent ligand was recognized, there has been significant growth in the number of publications in which 4′-functionalized 4,2′:6′,4′′-tpys act as linkers in coordination polymers and networks.7 In the current Highlight, we focus on ways in which reactions between metal nodes and 4,2′:6′,4′′-tpy or 3,2′:6′,3′′-tpy ligands can be directed to give 2- or 3-dimensional architectures. One guideline is to move away from the ubiquitous use of zinc halides or zinc acetate. The former usually lead to 1-dimensional chains7 or discrete metallomacrocycles,7,8 and the latter to chains containing linear {Zn2(μ-OAc)4} nodes.7,9
The peripheral donor
A strategy adopted by many groups to switch the assembly preference from 1-dimension to 2- or 3-dimensions is to incorporate a peripheral donor group. Popular choices are heterocycles (e.g. pyridine, pyrimidine) or carboxylic acids. Note the analogy between 4′-(4-pyridyl)-4,2′:6′,4′′-terpyridine (py-4,2′:6′,4′′-tpy) and 2,4,6-tris(4-pyridyl)-1,3,5-triazine (Scheme 3), a building block par excellence in coordination networks.10 If all three outer pyridine rings bind metals, py-4,2′:6′,4′′-tpy acts as a planar, 3-connecting node. In some cases, serendipity rules, and one or more donors remain coordinatively innocent when the ligand reacts with a metal salt.11 A pertinent example is ligand 1 (Fig. 1a). Its reaction with Zn(acac)2 unexpectedly gives a discrete complex in which 1 coordinates to Zn through one pyridine ring only (Fig. 1b), along with a linear polymer (Fig. 1b); CH⋯N contacts are implicated as one controlling factor in these two assemblies.12
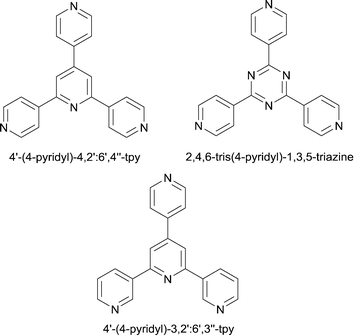 |
| Scheme 3 Structural analogy between 4′-(4-pyridyl)-4,2′:6′,4′′-tpy (py-4,2′:6′,4′′-tpy) and 2,4,6-tris(4-pyridyl)-1,3,5-triazine, and the structure of 4′-(4-pyridyl)-3,2′:6′,3′′-tpy (py-3,2′:6′,3′′-tpy). | |
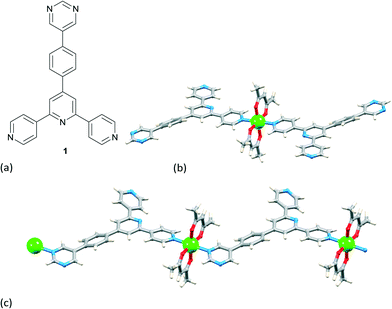 |
| Fig. 1 (a) Structure of 1, an example of a 4,2′:6′,4′′-tpy functionalized in the 4′-position with a metal-recognition site; Zn(acac)2 reacts with 1 to give (b) molecular [Zn(1)2(acac)2] and (c) polymeric [Zn(1)(acac)2]n. CSD refcodes QIXYIH and QIXYON. | |
The examples discussed below are not comprehensive, but rather exemplify how peripheral pyridyl or carboxylate groups are applied to direct the assembly of 2- and 3-dimensional frameworks. A few recent examples have been selected, and citations within these publications guide the reader to a wider literature coverage of the area.
The cationic metal–organic framework (MOF) present in [{Cu(py-4,2′:6′,4′′-tpy)}·NO3·MeOH]n has been prepared using a solvothermal approach, with in situ reduction of copper(II) to copper(I). Both Cu and py-4,2′:6′,4′′-tpy are 3-connecting nodes, and the resulting 4-fold interpenetrating 3-dimensional framework (Fig. 2a) retains channels in which anions and solvent molecules are accommodated. Solvent can be exchanged (MeOH for H2O) without a change in the lattice. The nitrate anions in the hydrated MOF can be exchanged with a range of anions, allowing the system to be applied for anion sensing.13 Retaining the 3-connecting Cu and py-4,2′:6′,4′′-tpy nodes, but introducing bridging cyanido ligands into the MOF leads to [Cu3(μ-CN)3(py-4,2′:6′,4′′-tpy)]n which is also 4-fold interpenetrating (Fig. 2b).14
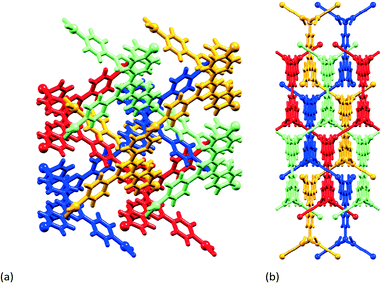 |
| Fig. 2 Part of the 4-fold interpenetrating frameworks in (a) [{Cu(py-4,2′:6′,4′′-tpy)}·NO3·MeOH]n viewed down the c-axis with anions and solvent omitted, and (b) [Cu3(μ-CN)3(py-4,2′:6′,4′′-tpy)]n viewed down the c-axis. CSD refcodes RIGPEE and COXGIG. | |
The trinuclear unit {Fe2NiO(Piv)6} (HPiv = pivalic acid) is preorganized as a planar, 3-connecting node and combined with py-4,2′:6′,4′′-tpy gives a 2-dimensional (6,3) net.15 Replacement of py-4,2′:6′,4′′-tpy by py-3,2′:6′,3′′-tpy leads to a switch from a (6,3) net to (4.82)-fes topology.16 An attractive application of py-4,2′:6′,4′′-tpy is as a ‘lock’ in MOFs to prevent loss of guest molecules. This is demonstrated by the introduction of 4,2′:6′,4′′-tpy to [{Na4Zn2(fda)4(H2O)2}·H2O]n (H2fda = 2,5-furandicarboxylic acid) which contains channels with a diameter comparable to that of the 4,2′:6′,4′′-tpy ligand.17
4′-(4-Carboxyphenyl)-4,2′:6′,4′′-terpyridine (4′-(4-Hcp)-4,2′:6′,4′′-tpy, Scheme 4) has been used in a range of coordination polymers, a number assembled in the presence of a co-ligand.18 If the peripheral carboxylate is monodentate or acts as a chelating ligand, 4′-(4-cp)-4,2′:6′,4′′-tpy can function as a planar 3-connecting node and is therefore potentially analogous to py-4,2′:6′,4′′-tpy. But, as seen above, one or more donor sites may remain non-coordinated leading to lower dimensionality structures than anticipated. An example is [Co(4′-(4-cp)-4,2′:6′,4′′-tpy)(H2O)2]n which is a 1D-coordination polymer with 4′-(4-cp)-4,2′:6′,4′′-tpy acting an a bridging N,O-donor.19 The addition of co-ligands in some investigations makes it difficult to appreciate clear design strategies in the use of 4′-(4-carboxyphenyl)-4,2′:6′,4′′-terpyridines. Nonetheless, the examples below illustrate the potential for their use in 2- and 3-dimensional assemblies.
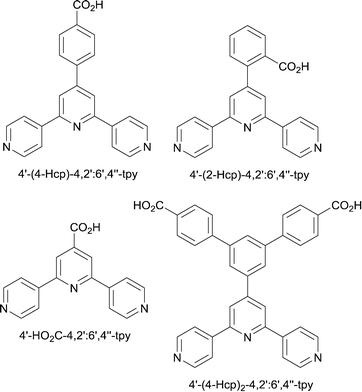 |
| Scheme 4 Structures of 4,2′:6′,4′′-terpyridines containing carboxylic acid functionalities. | |
Interpenetrating sheets are observed in [{Cd(4′-(4-cp)-4,2′:6′,4′′-tpy)(OAc)(H2O)}·H2O·DMA]n (Fig. 3a), but a change in cadmium(II) salt, solvothermal conditions and solvent leads to [{Cd(4′-(4-cp)-4,2′:6′,4′′-tpy)2}·2DMF]n which consists of a non-interpenetrated net with Cd and ligand acting as 5- and 3-connecting nodes respectively.20 The same connectivity pattern is observed in [{Cd(4′-(4-cp)-4,2′:6′,4′′-tpy)2}·1.5H2O]n, but in this case, the nets interpenetrate.21 [{Zn2(4′-(4-cp)-4,2′:6′,4′′-tpy)2Cl2}·0.5H2O]n is structurally analogous to [{Cd(4′-(4-cp)-4,2′:6′,4′′-tpy)(OAc)(H2O)}·H2O·DMA]n (Fig. 3a), whereas [{Cd2(4′-(4-cp)-4,2′:6′,4′′-tpy)4}·3.5H2O]n exhibits doubly interpenetrating (4,4) nets.22
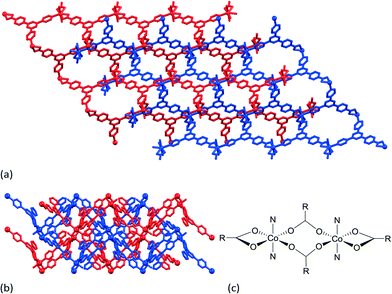 |
| Fig. 3 (a) Double interpenetrating (6,3) sheets in [{Cd(4′-(4-cp)-4,2′:6′,4′′-tpy)(OAc)(H2O)}·H2O·DMA]n (DMA = N,N-dimethylacetamide); CSD refcode HEXTAU. (b) Entangled framework in [Co(4′-(4-cp)-4,2′:6′,4′′-tpy)2]n CSD refcode DIQHAO. | |
One structure deserves particular attention. The reaction of CoCl2·6H2O with 4′-(4-Hcp)-4,2′:6′,4′′-tpy under basic solvothermal conditions leads to [Co(4′-(4-cp)-4,2′:6′,4′′-tpy)2]n. The assembly of the entangled framework depicted in Fig. 3b arises from the 8-connecting node shown in Fig. 3c in which ‘N’ represents an N-bound 4′-(4-cp)-4,2′:6′,4′′-tpy domain, and ‘RCO2’ represents the carboxylate functionality in 4′-(4-cp)-4,2′:6′,4′′-tpy. The assembly is of note because of coexisting interpenetration, self-threading and polythreading.23
Despite possible steric crowding of the 2-substituted functionality in 4′-(2-cp)-4,2′:6′,4′′-tpy (Scheme 4), this ligand has been shown to be a versatile building block in coordination networks.24 The effects of removing the phenylene spacer on going from 4′-(4-Hcp)-4,2′:6′,4′′-tpy to 4′-HO2C-4,2′:6′,4′′-tpy have also been explored,25 and recently, Wang and co-workers have shown that the introduction of two peripheral carboxylic acid groups opens up the pathway to zinc-containing MOFs which exhibit selective CO2 capture.26
Going for higher coordination numbers
The previous section illustrates the approach adopted by many research teams to increase the dimensionality of coordination networks which incorporate divergent tpy linkers. We now move to our own strategies which have developed from an initial penchant for coordination polymers containing zinc(II) nodes.7 To encourage the assembly of 2- or 3-dimensional networks using 4,2′:6′,4′′-tpy linkers, our approach was to increase the connectivity of the metal node. We originally showed that going from zinc to cadmium27 facilitated a switch from helical 1-dimensional chains [ZnCl2(4′-aryl-4,2′:6′,4′′-tpy)]n containing 2-connecting Zn nodes, to 2-dimensional sheets [Cd(NO3)2(2)2]n containing 4-connecting Cd nodes (2 = 4′-phenyl-4,2′:6′,4′′-tpy, Scheme 5). The 6-coordinate Cd atom (with trans-nitrato ligands) binds four 2 ligands to give a (4,4) net with each divergent 4,2′:6′,4′′-tpy ligand bridging adjacent Cd atoms. This is a motif that reappears in a number of related compounds with 4-connecting Cd nodes and 4′-aryl-functionalized 4,2′:6′,4′′-tpy ligands, for example with ligand 328 (Scheme 5). However, the assembly principle is not general, and the assembles formed with ligands 4–9 (Scheme 5) provide pertinent examples; 4–9 are derivatives of 2 bearing alkoxy tails. Cd(NO3)·4H2O reacts with 4 (methoxy substituent) to give a 1-dimensional ladder (Fig. 4a) under room temperature crystallization conditions with an input Cd
:
ligand ratio of 3
:
1.29 A ladder is again seen when Cd(NO3)·4H2O is combined with 8 (n-hexoxy substituent, Fig. 4b) in a molar ratio of 1
:
1, but if the amount of ligand is increased in the crystallization experiment (ratio of moles of Cd
:
8 = 1
:
3), the assembly switches to a (4,4) net (Fig. 5a).30 A closely related (4,4) net is formed with 7, but on going to 5 with the shorter n-propyl chain, a (6,3) net assembles in [{Cd2(NO3)4(5)3}·3CHCl3]n (Fig. 5b). Powder diffraction was used to confirm the homogeneity of the bulk samples, and also indicates that reaction of Cd(NO3)·4H2O with 6 leads to a (6,3) net. In this network, packing involves the accommodation of the n-propoxy chain in a pocket comprising three ligands 5; this pocket is big enough to accommodate an n-butoxy chain, but not a longer chain. Hence, going from an n-butoxy to n-pentoxy chain switches the architecture from a (6,3) to (4,4) net.30 Reactions of Cd(NO3)2·4H2O with 10 or 11 also give ladders (Fig. 4c).31 The study of network assembly with cadmium nitrate and ligands 5–9 was systematic, using a 1
:
3 ratio of Cd
:
ligand, and constant volumes of the same solvents (MeOH and CHCl3).30 Overall, however, rationalizing the observations for Cd(NO3)2/4,2′:6′,4′′-tpy networks with ligands 2–11 is made difficult by variable ‘input ratios’ of metal
:
ligand and solution concentrations, and the fact that not all bulk samples have been analysed by X-ray powder diffraction.
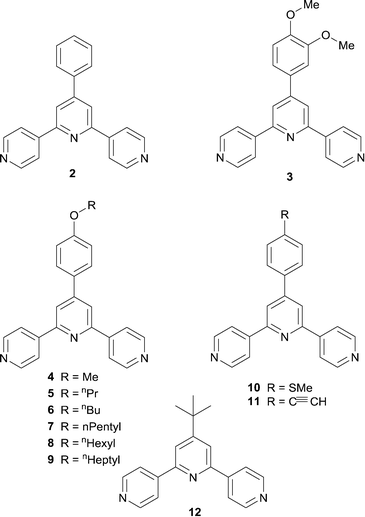 |
| Scheme 5 Structures of ligands 2–12. | |
 |
| Fig. 4 1-Dimensional ladders in (a) [{Cd2(NO3)4(4)3}·CHCl3·MeOH]n,29 (b) [Cd2(NO3)4(MeOH)(8)3]n,30 and (c) [{Cd2(NO3)4(11)3}·2CHCl3·4MeOH]n.31 Overlay of the structure and a TOPOS32 representation. Nitrato ligands and solvent molecules are omitted. | |
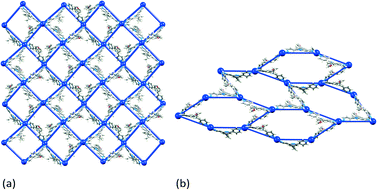 |
| Fig. 5 2-Dimensional nets: (a) (4,4) net in [{Cd2(NO3)4(8)4}·CHCl3·MeOH]n, and (b) (6,3) net in [{Cd2(NO3)4(5)3}·3CHCl3]n.30 Overlay of the structure and a TOPOS32 representation. | |
The preference for the ladder motif is unclear. Given that the reaction of cadmium(II) nitrate with ligand 8 can lead to either a ladder or a (4,4) net, it seems unlikely that substituent effects are the dominant factor, and the preference is possibly associated with concentration effects. Concentration effects are the well-established roots for the competitive formation of metallomacrocycles versus metallopolymers.33 Of relevance is our recent observation that, in the same crystallization vessel but in different zones resulting from different concentration gradients, the assembly of the coordination polymer [ZnCl2(L)]n (L = 4′-(2′,3′,4′,5′,6′-pentafluorobiphenyl-4-yl)-4,2′:6′,4′′-terpyridine) competes with that of the metallosquare [{ZnCl2(L)}4].34
It is tempting to think that an octahedral {MX2N4} metal centre (N = donor atom from a 4,2′:6′,4′′-tpy ligand, X = ancillary ligand) is predisposed to acting as a 4-connecting node only if the ancillary ligands are mutually trans. This is not the case. The reactions of cadmium(II) nitrate with 7, 8 and 9 all lead to (4,4) nets, but in [{Cd2(NO3)4(7)4}·3CHCl3]n and [{Cd2(NO3)4(8)4}·CHCl3·MeOH]n, the nitrato ligands are trans, while in [{Cd(NO3)2(9)2}·2MeOH]n, they are cis. Fig. 6 illustrates views through part of a sheet in each of [{Cd2(NO3)4(8)4}·CHCl3·MeOH]n and [{Cd(NO3)2(9)2}·2MeOH]n showing how trans/cis isomerisation at Cd affects the profile of the net; the profile of the sheet in [{Cd2(NO3)4(7)4}·3CHCl3]n mimics that in [{Cd2(NO3)4(8)4}·CHCl3·MeOH]n (Fig. 6a).
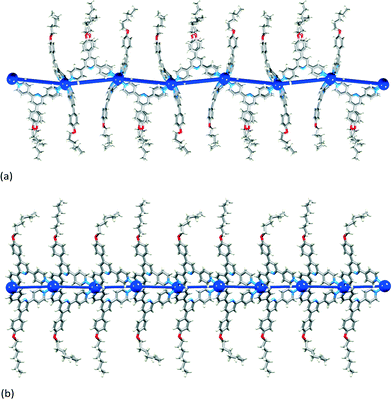 |
| Fig. 6 Comparison of the profiles of the (4,4) nets in (a) [{Cd2(NO3)4(8)4}·CHCl3·MeOH]n with trans-NO3− ligands and (b) [{Cd(NO3)2(9)2}·2MeOH]n with cis-NO3− ligands. Nitrato ligands and solvent molecules are omitted for clarity. | |
The structural similarities between the (4,4) nets in [CdX2(3)2]n (X = NO3, Br, I) and [CoCl2(3)2]n,28 demonstrate the use of cobalt(II) as an alternative to cadmium(II) for increasing the dimensionality of architectures involving 4,2′:6′,4′′-tpy linkers. The (4,4) nets in [{2Co(NCS)2(2)2·5H2O}n]35 and [{Cd(NO3)2(2)2}·MeOH·CHCl3]n27 mimic one another, and reaction of Co(NCS)2 with 12 (Scheme 5) produces a (4,4) net with a ‘ball-and-socket’ packing of adjacent sheets involving CHtert-Bu⋯Npyridine interactions.36 The latter is an interesting example in which a change in solvate causes a switch in packing of the (4,4) nets. In [{4Co(NCS)2(12)2·MeOH}·H2O]n, the sheets are equally spaced (Fig. 7a) while in [{Co(NCS)2(12)2}·0.5H2O]n,37 adjacent sheets have alternating close and wide spacings (Fig. 7b).
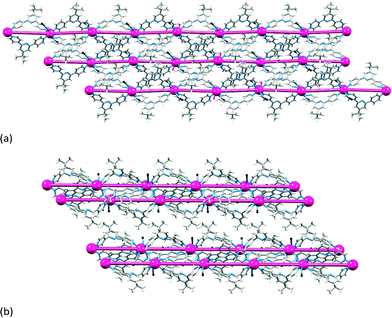 |
| Fig. 7 Comparison of the packing of sheets in (a) [{4Co(NCS)2(12)2·MeOH}·H2O]n (CSD refcode FAKRIU),36 and (b) [{Co(NCS)2(12)2}·0.5H2O]n.37 | |
Expanding dimensionality using ditopic bis(4,2′:6′,4′′-tpy) and bis(3,2′:6′,3′′-tpy) ligands
The tool-kit of the supramolecular chemist is made richer by the use of multitopic ligands, i.e. ‘tying together’ metal-binding domains in preorganized arrays. Ligands containing multiple 2,2′:6′,2′′-tpy metal-binding sites are invaluable for accessing a range of exciting supramolecular architectures.38 In contrast, ligands with multiple 4,2′:6′,4′′- or 3,2′:6′,3′′-tpy are still little investigated. In 2013, Yoshida et al. demonstrated the potential for such ditopic ligands in reactions of 13 or 14 (Scheme 6) with bis(3-cyanopentane-2,4-dionato)cobalt(II).39 Ligands 13 and 14 are both 4-connecting, and the combination of bis(3-cyanopentane-2,4-dionato)cobalt(II) with 13 gives 2-dimensional nets that are triply interpenetrating.
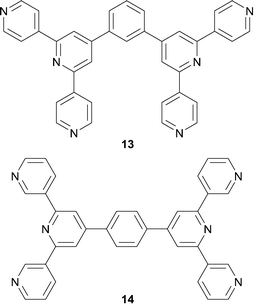 |
| Scheme 6 Structures of the isomeric ligands 13 and 14.39 | |
The rather poor solubilities of bis(4,2′:6′,4′′-tpy) ligands with phenylene spacers can be rectified by introducing solubilizing alkyl chains. This turns out to have an added advantage: the octoxy tails in ligand 15 (Scheme 7) direct the assembly of 2D→2D parallel interpenetrated sheets in [{Zn2Cl4(15)}·4H2O]n and [Zn2Br4(15)]n.40,41 The interpenetration is lost when the long alkoxy chains are replaced by methoxy groups in [{Zn2Br4(16)}·2C6H4Cl2]n and [{Zn2I4(16)}·2.3C6H4Cl2]n (see Scheme 7 for ligand 16). All four coordination polymers consist of similar (4,4) nets in which the ligand (15 or 16) behaves as a planar, 4-connecting node. This is illustrated in Fig. 8 for [{Zn2Br4(16)}·2C6H4Cl2]n; the centroid of each phenylene ring in 16 (i.e. the 4-connecting node in the network) is shown in red in Fig. 8, emphasizing that the zinc centres do not play a role in defining the connectivity of the network. Each sheet in [{Zn2Cl4(15)}·4H2O]n, [Zn2Br4(15)]n, [{Zn2Br4(16)}·2C6H4Cl2]n and [{Zn2I4(16)}·2.3C6H4Cl2]n has a corrugated profile (Fig. 8b). In the coordination networks with ligand 16, the MeO groups point above and below the sheet, and sheets nest together with inter-sheet face-to-face π-stacking interactions.41 In contrast, in [{Zn2Cl4(15)}·4H2O]n and [Zn2Br4(15)]n, the octoxy chains (in extended conformations) run through the plane of the sheet and play a role in guiding the 2D→2D parallel interpenetration40 shown in the TOPOS32 representation in Fig. 9.
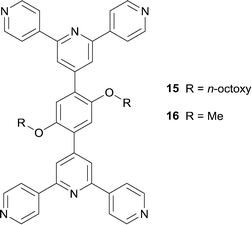 |
| Scheme 7 Structures of the ditopic bis(4,2′:6′,4′′-tpy) ligands 15 and 16. | |
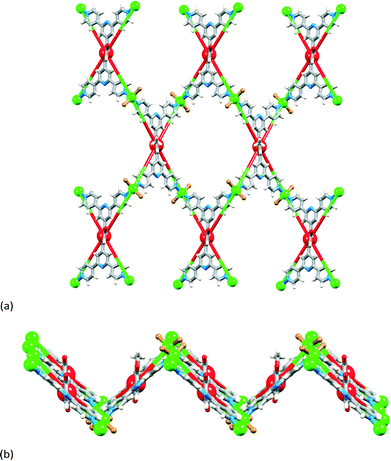 |
| Fig. 8 (a) Part of one (4,4) sheet in [{Zn2Br4(16)}·2C6H4Cl2]n with a TOPOS representation of the net overlaid on the structure. (b) Side view of the net showing the corrugated profile. The centroid of central phenylene ring in 16 is shown in red (4-connecting node), and Zn atoms in green.41 | |
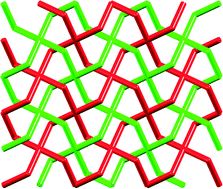 |
| Fig. 9 2D→2D parallel interpenetration of (4,4) nets in [{Zn2Cl4(15)}·4H2O]n and [Zn2Br4(15)]n.40,41 | |
In the examples above, the connectivity of the 2-dimensional networks is entirely governed by the ditopic ligand, and this is clearly seen in Fig. 8a. In contrast, the reaction of 1,4-bis(n-octoxy)-2,5-bis(3,2′:6′,3′′-terpyridin-4′-yl)benzene, 17, with Co(NCS)2 leads to a 3-dimensional network in which both ligand and cobalt atom act as 4-connecting nodes. Effectively, this combines the strategy of ‘going for higher coordination numbers’ described in the previous section, with the approach of using multiple metal-binding domains introduced above. The rotational freedom of ligands 15 and 16, and of their 3,2′:6′,3′′-analogues exemplified by 17, allows them to act as 4-connecting nodes on a path that lies between the limits of planar to approximately tetrahedral (Scheme 8). [Co(NCS)2(17)·4CHCl3]n adopts a 3-dimensional {42.84} lvt net (Fig. 10) in which the Co atom (with trans-thiocyanato ligands) is a planar 4-connecting node, and ligand 17 is an approximately tetrahedral 4-connecting node. The paucity of {42.84} lvt nets among MOFs makes [Co(NCS)2(17)·4CHCl3]n a particularly noteworthy assembly.42
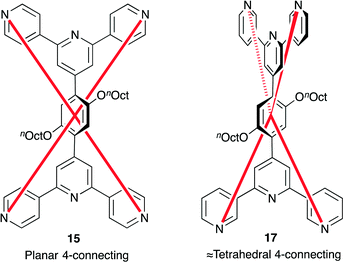 |
| Scheme 8 Bis(4,2′:6′,4′′)- or bis(3,2′:6′,3′′-tpy) ligands 15 and 17 as planar or ≈tetrahedral 4-connecting nodes. Either ligand could adopt either node-geometry. | |
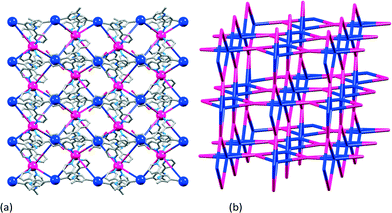 |
| Fig. 10 [Co(NCS)2(17)·4CHCl3]n: (a) superimposition of the structure of part of the assembly with a TOPOS32 representation, looking down the b-axis; the octyl chains and H atoms are omitted for clarity; (b) the {42.84} lvt net with Co nodes in pink and ligand nodes (centroid of the phenylene ring) in blue. | |
Conclusions
The design of 2- and 3-dimensional networks in which the linkers are divergent isomers of terpyridine is a rapidly expanding area of research. Ultimate goals include MOFs for gas adsorption, and photo- or redox-active networks. This Highlight has demonstrated approaches to encourage the formation of 2- and 3-dimensional architectures, thus moving away from the 1-dimensional chains that pervaded earlier investigations of the coordination chemistry of 4,2′:6′,4′′-tpy. Functionalization in the tpy 4′-position with, for example, pyridyl, pyrimidyl or carboxylate donors is an approach widely adopted. However, the ligand is not necessarily coordinately saturated (the central pyridine ring of the tpy domain being ignored in this definition of ‘saturated’) and the addition of co-ligands also complicates the design ‘plan’. Our own strategies are two-fold: (i) to increase the coordination number of the metal centre and retain potentially two-coordinate, divergent 4,2′:6′,4′′- or 3,2′:6′,3′′-tpy linkers, and (ii) to design ligands than contain multiple 4,2′:6′,4′′- or 3,2′:6′,3′′-tpy domains. The latter strategy has proved to be particularly successful, and one that is ripe for further development.
Acknowledgements
The Swiss National Science Foundation, the European Research Council (Advanced Grant 267816 LiLo) and the University of Basel are acknowledged for financial support for our work in this area; my dedicated coworkers in this work are acknowledged in the cited references. My grateful thanks go to my husband, Edwin Constable, for our endless discussions.
Notes and references
- E. C. Constable, Coord. Chem. Rev., 2008, 252, 842 CrossRef CAS PubMed.
- F. Kröhnke, Synthesis, 1976, 1 CrossRef.
- J. Wang and G. S. Hanan, Synlett, 2005, 1251 CAS.
- F. H. Allen, Acta Crystallogr., Sect. B: Struct. Sci., 2002, 58, 380 CrossRef PubMed.
- I. J. Bruno, J. C. Cole, P. R. Edgington, M. Kessler, C. F. Macrae, P. McCabe, J. Pearson and R. Taylor, Acta Crystallogr., Sect. B: Struct. Sci., 2002, 58, 389 CrossRef PubMed.
- M. Barquín, J. Cancela, M. J. González Garmendia, J. Quintanilla and U. Amador, Polyhedron, 1998, 17, 2373 CrossRef.
- C. E. Housecroft, Dalton Trans., 2014, 43, 6594 RSC.
- See for example: E. C. Constable, C. E. Housecroft, J. A. Zampese and G. Zhang, CrystEngComm, 2011, 13, 6864 RSC; E. C. Constable, C. E. Housecroft, S. Vujovic and J. A. Zampese, CrystEngComm, 2014, 16, 328 RSC; E. C. Constable, C. E. Housecroft, A. Prescimone, S. Vujovic and J. A. Zampese, CrystEngComm, 2014, 6, 8691 RSC.
- Y. M. Klein, E. C. Constable, C. E. Housecroft, J. A. Zampese and A. Crochet, CrystEngComm, 2014, 16, 9915 RSC.
- B. Therrien, J. Organomet. Chem., 2011, 696, 637 CrossRef CAS PubMed and references therein.
- See for example: Y. M. Klein, E. C. Constable, C. E. Housecroft and J. A. Zampese, Polyhedron, 2014, 81, 98 CrossRef CAS PubMed; B.-C. Wang, Q.-R. Wu, H.-M. Hu, X.-L. Chen, Z.-H. Yang, Y.-Q. Shangguan, M.-L. Yang and G.-L. Xue, CrystEngComm, 2010, 12, 485 RSC; E. C. Constable, G. Zhang, C. E. Housecroft and J. A. Zampese, CrystEngComm, 2011, 13, 6864 RSC; J. Song, B.-C. Wang, H.-M. Hu, L. Gou, Q.-R. Wu, X.-L. Yang, Y.-Q. Shangguan, F.-X. Dong and G.-L. Xue, Inorg. Chim. Acta, 2011, 366, 134 CrossRef PubMed.
- J. Granifo, R. Gaviño, E. Freire and R. Baggio, J. Mol. Struct., 2014, 1063, 102 CrossRef CAS PubMed.
- Y.-Q. Chen, G.-R. Li, Z. Chang, Y.-K. Qu, Y.-H. Zhang and X.-H. Bu, Chem. Sci., 2013, 4, 3678 RSC.
- C. Liu, Y.-B. Ding, X.-H. Shi, D. Zhang, M.-H. Hu, Y.-G. Yin and D. Li, Cryst. Growth Des., 2009, 9, 1275 CAS.
- V. N. Dorofeeva, S. V. Kolotilov, M. A. Kiskin, R. A. Polunin, Z. V. Dobrokhotova, O. Cador, S. Golhen, L. Ouahab, I. L. Eremenko and V. M. Novotortsev, Chem. – Eur. J., 2012, 18, 5006 CrossRef CAS PubMed.
- S. A. Sotnik, R. A. Polunin, M. A. Kiskin, A. M. Kirillov, V. N. Dorofeeva, K. S. Gavrilenko, I. L. Eremenko, V. M. Novotortsev and S. V. Kolotilov, Inorg. Chem., 2015, 54, 5169 CrossRef CAS PubMed.
- H. Wang, J. Xu, D.-S. Zhang, Q. Chen, R.-M. Wen, Z. Chang and X.-H. Bu, Angew. Chem., Int. Ed., 2015, 54, 5966 CrossRef CAS PubMed.
- See for example: J. Yang, S.-W. Yan, X. Wang, D.-R. Xiao, H.-Y. Zhang, X.-L. Chi, J.-L. Zhang and E. Wang, Inorg. Chem. Commun., 2013, 38, 100 CrossRef CAS PubMed; Y. Gong, M. M. Zhang, P. Zhang, H. F. Shi, P. G. Jiang and J. H. Lin, CrystEngComm, 2014, 16, 9882 RSC.
- M.-S. Wang, M.-X. Li, X. He, M. Shao and Z.-X. Wang, Inorg. Chem. Commun., 2014, 42, 38 CrossRef CAS PubMed.
- L. Wen, X. Ke, L. Qiu, Y. Zou, L. Zhou, J. Zhao and D. Li, Cryst. Growth Des., 2012, 12, 4083 CAS.
- C. Niu, A. Ning, C. Feng, X. Wan and C. Kou, J. Inorg. Organomet. Polym., 2012, 22, 519 CrossRef CAS.
- F. Yuan, R. An, H.-M. Hu, S.-S. Shen, X. Wang, M.-L. Yang and G. Xue, Inorg. Chem. Commun., 2015, 56, 1 CrossRef CAS PubMed.
- J. Yang, J. Liu, X. Wang, X. Chi, J. Zhang, H. Zhang, D. Xiao and Q. Luo, CrystEngComm, 2013, 15, 10435 RSC.
- B. Xu, J. Xie, H.-M. Hu, X.-L. Yang, F.-X. Dong, M.-L. Yang and G.-L. Xue, Cryst. Growth Des., 2014, 14, 1629 CAS.
- See for example: F. Yuan, J. Xie, H.-M. Hu, C.-M. Yuan, B. Xu, M.-L. Yang, F.-X. Dong and G.-L. Xue, CrystEngComm, 2013, 15, 1460 RSC; Y. Li, Z. Ju, B. Wu and D. Yuan, Cryst. Growth Des., 2013, 13, 4125 Search PubMed; F. Yuan, S.-S. Shen, H.-M. Hu, R. An, X. Wang, Z. Chang and G. Xue, Inorg. Chim. Acta, 2015, 430, 17 CrossRef CAS PubMed and references therein.
- B. Liu, L. Hou, W.-P. Wu, A.-N. Dou and Y.-Y. Wang, Dalton Trans., 2015, 44, 4423 RSC.
- E. C. Constable, G. Zhang, C. E. Housecroft, M. Neuburger and J. A. Zampese, CrystEngComm, 2009, 11, 2279 RSC.
- U. P. Singh, S. Narang, P. Pachfule and R. Banerjee, CrystEngComm, 2014, 16, 5012 RSC.
- Y. M. Klein, E. C. Constable, C. E. Housecroft and A. Prescimone, Inorg. Chem. Commun., 2014, 49, 41 CrossRef PubMed.
- Y. M. Klein, A. Prescimone, E. C. Constable and C. E. Housecroft, CrystEngComm, 2015, 17, 6483 RSC.
- E. C. Constable, G. Zhang, C. E. Housecroft, M. Neuburger and J. A. Zampese, CrystEngComm, 2010, 12, 3733 RSC.
-
V. A. Blatov and A. P. Shevchenko, TOPOS Professional v. 4.0, Samara State University, Russia Search PubMed.
- E. C. Constable, E. L. Dunphy, C. E. Housecroft, W. Kylberg, M. Neuburger, S. Schaffner, E. R. Schofield and C. B. Smith, Chem. – Eur. J., 2006, 12, 4600 CrossRef CAS PubMed and references therein.
- E. C. Constable, C. E. Housecroft, A. Prescimone, S. Vujovic and J. A. Zampese, CrystEngComm, 2014, 16, 8691 RSC.
- E. C. Constable, C. E. Housecroft, M. Neuburger, S. Vjovic, J. A. Zampese and G. Zhang, CrystEngComm, 2012, 14, 3554 RSC.
- E. C. Constable, C. E. Housecroft, P. Kopecky, M. Neuburger, J. A. Zampese and G. Zhang, CrystEngComm, 2012, 14, 446 RSC.
- Y. M. Klein, A. Prescimone, E. C. Constable and C. E. Housecroft, Polyhedron, 2015 Search PubMed , in press.
- See for example: E. C. Constable, A. M. W. Cargill Thompson, P. Harveson, L. Macko and M. Zehnder, Chem. – Eur. J., 1995, 1, 360 CrossRef CAS PubMed; E. C. Constable, Chem. Soc. Rev., 2007, 36, 246 RSC; E. C. Constable, Coord. Chem. Rev., 2008, 252, 842 CrossRef PubMed; A. Wild, A. Winter, F. Schluetter and U. S. Schubert, Chem. Soc. Rev., 2011, 40, 1459 RSC; Y. Yan and J. Huang, Coord. Chem. Rev., 2010, 254, 1072 CrossRef PubMed; E. C. Constable, Chimia, 2013, 67, 388 CrossRef PubMed; D. Rajwar, X. Liu, Z. B. Lim, S. J. Cho, S. Chen, J. M. H. Thomas, A. Trewin, Y. M. Lam, T. C. Sum and A. C. Grimsdale, RSC Adv., 2014, 4, 17680 RSC.
- J. Yoshida, S.-I. Nishikiori and H. Yuge, J. Coord. Chem., 2013, 66, 2191 CrossRef CAS PubMed.
- E. C. Constable, C. E. Housecroft, S. Vujovic and J. A. Zampese, CrystEngComm, 2014, 16, 3494 RSC.
- S. Vujovic, E. C. Constable, C. E. Housecroft, C. D. Morris, M. Neuburger and A. Prescimone, Polyhedron, 2015, 92, 77 CrossRef CAS PubMed.
- Y. M. Klein, E. C. Constable, C. E. Housecroft and A. Prescimone, CrystEngComm, 2015, 17, 2070 RSC.
|
This journal is © The Royal Society of Chemistry 2015 |
Click here to see how this site uses Cookies. View our privacy policy here.