DOI:
10.1039/C5CC02140E
(Communication)
Chem. Commun., 2015,
51, 8528-8531
Enantioselective 1,4-addition of cyclopropylboronic acid catalyzed by rhodium/chiral diene complexes†
Received
13th March 2015
, Accepted 9th April 2015
First published on 10th April 2015
Abstract
Rhodium-catalyzed asymmetric addition of cyclopropylboronic acids to electron-deficient alkenes such as alkenylsulfones, enones, enoates, and nitroalkenes proceeded to give high yields of the corresponding 1,4-addition products with high enantioselectivity.
Asymmetric conjugate addition of organometallic reagents to electron-deficient alkenes catalyzed by Rh complexes is now well-recognized to be one of the most reliable methods for carbon–carbon bond formation introducing aryl and alkenyl groups with high enantioselectivity.1,2 On the other hand, asymmetric conjugate addition of alkyl groups has been developed using Ni and Cu catalysts,3 and thus both catalytic systems perform a complementary role in the transition metal-catalyzed asymmetric conjugate addition reactions.4 The catalytic conjugate addition of simple alkyl metal reagents under the rhodium catalysis using organometallic reagents is difficult because an intermediate alkylrhodium(I) species having a β-hydrogen readily undergoes elimination to give a hydridorhodium species and an alkene.4 As rare examples of the rhodium-catalyzed addition reaction of alkyl metal reagents, we reported asymmetric methylation of imines by the use of Me2Zn or trimethylboroxine as a methylating reagent, where the β-hydrogen to be eliminated does not exist.5,6 von Zezschwitz and co-workers reported asymmetric 1,2- or 1,4-addition of Me3Al to cyclic enones catalyzed by a rhodium/binap complex.7 The stereoselective alkyl transfer from potassium benzylic trifluoroborates to aldehydes was also reported by Aggarwal and co-workers, where it is proposed that the reaction proceeds by direct migration of the benzylic group to the aldehyde without formation of a benzylrhodium species.8 In this context, we focused on the use of a cyclopropylrhodium(I) species for the conjugate addition, which may avoid the β-hydrogen elimination leading to the formation of a highly strained cyclopropene. The asymmetric addition of dicyclopropylzinc to aldehydes was reported by the use of a chiral amino alcohol.9 The diastereoselective addition of cyclopropyllithium or magnesium bromide to imines is achieved using a chiral auxiliary on the nitrogen.10 The Cu-catalyzed enantioselective addition of dicyclopropylzinc to a β-disubstituted nitroalkene was reported to give the addition product in low yield with low enantioselectivity.11,12 Here we report that the asymmetric addition of cyclopropylboronic acid to electron-deficient alkenes catalyzed by Rh/chiral diene complexes. To the best of our knowledge, this is the first example of the metal-catalyzed asymmetric conjugate addition of cyclopropylboronic acids.13
We found that a Rh complex coordinated with a diene ligand has high catalytic activity in the addition of cyclopropylboronic acid to an alkenylsulfone (Table 1). Thus, treatment of alkenylsulfone 1a with cyclopropylboronic acid (2, 2.5 equiv.) in the presence of [RhCl(cod)]2 (3 mol% of Rh) and K3PO4 (1 equiv.) in toluene at 60 °C for 12 h gave the addition product 3a in 60% yield (entry 1, Table 1). An enantioselective addition was achieved by the use of chiral diene ligands.14 A Rh complex coordinated with a ferrocenyl (Fc)-substituted diene ligand based on the tetrafluorobenzobarrelene (tfb) framework,15 which is a superior catalyst in the asymmetric addition of arylboronic acids to alkenyl sulfonyl compounds,16,17 displayed a high catalytic activity and enantioselectivity to give 3a in 96% yield with 97% ee (entry 2). Other tfb ligands substituted with phenyl (Ph) and benzyl (Bn), and bicyclo[2.2.2]octadienes L1 and L2,18 which are derived from a natural product, were less effective in the present addition reaction (entries 3–6). The use of a rhodium-bisphosphine complex [RhCl((R)-binap)]219 did not give the addition product at all (entry 7). Cyclopropylboronic acid neopentylglycolate 2′ can also be used to give a 99% yield of 3a with 94% ee, although the reaction requires a higher reaction temperature (80 °C, entry 8). The absolute configuration of product 3a formed by the use of (S,S)-Fc-tfb* was determined to be S by X-ray crystallographic analysis.
Table 1 Rh-catalyzed addition of cyclopropylboronic acid 2 to alkenylsulfone 1aa
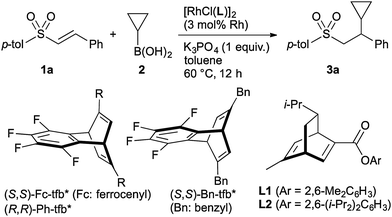
|
Entry |
L
|
Yieldb (%) |
eec (%) |
Reaction conditions: alkenylsulfone 1a (0.10 mmol), 2 (0.25 mmol), the Rh catalyst (3 mol% of Rh), K3PO4 (1 equiv.) in toluene (0.4 mL) at 60 °C for 12 h.
Determined by 1H NMR analysis using 1,4-dimethoxybenzene as an internal standard.
Determined by chiral HPLC analysis.
Isolated yield.
Not determined.
Cyclopropylboronic acid neopentylglycolate 2′ was used instead of 2 in the presence of methanol (3 equiv.) at 80 °C.
|
1 |
cod (1,5-cyclooctadiene) |
60 |
— |
2 |
(S,S)-Fc-tfb* |
96d |
97 |
3 |
(R,R)-Ph-tfb* |
43 |
93 |
4 |
(S,S)-Bn-tfb* |
24 |
60 |
5 |
(R)-L1 |
10 |
—e |
6 |
(R)-L2 |
4 |
—e |
7 |
(R)-Binap |
0 |
— |
8f |
(S,S)-Fc-tfb* |
99 |
94 |
The results obtained for the enantioselective addition of cyclopropylboronic acid (2) to several alkenyl sulfonyl compounds 1 are summarized in Table 2.20 The reaction of alkenyl sulfones having 2-furyl (1b) and 2-thienyl (1c) at the β-position proceeded to give the corresponding addition products 3b and 3c, respectively, in high yields with high enantioselectivity (entries 1 and 2). A pyridyl group on alkenyl sulfone 1d slowed the reaction to give 3d in 51% yield at 80 °C for 12 h, but the enantioselectivity was high (96% ee, entry 3). Alkenyl sulfones substituted with 2-methyl-1-propenyl (1e), butyl (1f), and benzyl (1g)21 are also good substrates to give the corresponding addition products in good to high yields with high enantioselectivity (entries 4–6). Not only alkenyl sulfones, but also sulfonates (1h and 1i) and a sulfonamide (1j) can be applicable with high enantioselectivity (entries 7–9).
Table 2 Asymmetric cyclopropylation of alkenylsulfonyl compounds 1a
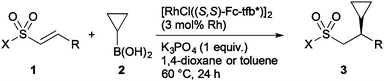
|
Entry |
X |
R |
Yieldb (%) |
eec (%) |
Reaction conditions: alkenylsulfone 1 (0.20 mmol), 2 (0.50 mmol), [RhCl((S,S)-Fc-tfb*)]2 (3 mol% of Rh), K3PO4 (1 equiv.) in 1,4-dioxane (for 1b–d, 1f, 1h; 0.8 mL) or toluene (for 1e, 1g, 1i, 1j; 0.8 mL) at 60 °C for 24 h.
Isolated yields.
Determined by chiral HPLC analysis.
At 80 °C.
Performed with 3.5 equiv. of 2.
Performed with 5 mol% of Rh.
For 12 h.
Na3PO4 was used instead of K3PO4.
|
1 |
p-Tolyl |
2-Furyl (1b) |
97 (3b) |
93 |
2 |
p-Tolyl |
2-Thienyl (1c) |
97 (3c) |
93 |
3d,e,f |
p-Tolyl |
3-Pyridyl (1d) |
51 (3d) |
96 |
4 |
p-Tolyl |
CH CMe2 (1e) |
78 (3e) |
83 |
5g |
p-Tolyl |
Butyl (1f) |
94 (3f) |
96 |
6g,h |
p-Tolyl |
Benzyl (1g) |
92 (3g) |
97 |
7d,e |
2,6-Me2C6H3O |
Ph (1h) |
80 (3h) |
98 |
8 |
EtO |
Ph (1i) |
86 (3i) |
92 |
9 |
N-Morpholyl |
Ph (1j) |
96 (3j) |
98 |
The enantioselective addition of cyclopropylboronic acid proceeded toward the other electron-deficient alkenes than alkenyl sulfones, where the higher enantioselectivity was observed with alkyl-substituted tfb ligands than that with Fc-tfb* (Table 3). In the presence of hydroxorhodium/chiral tfb catalysts,22 the addition to α,β-unsaturated ketones 4a–c, esters 4d and 4e, and di-tert-butyl fumarate (4f) proceeded to give the corresponding addition products in good yields with 81–86% ee (entries 1–6), where ligand L3 substituted with neopentyl groups displayed good enantioselectivity, except for the addition to ethyl ester 4d. Nitroalkenes 4g–i were also applicable acceptors by the use of KHF2 as a base23 instead of K3PO4 to give the corresponding addition products with 89% ee (entries 7–9).
Table 3 Asymmetric cyclopropylation of enones, enoates, and nitroalkenesa
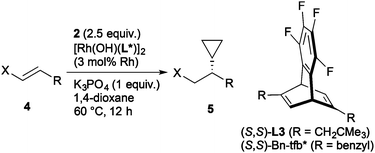
|
Entry |
L*
|
X |
R |
Yieldb (%) |
eec (%) |
Reaction conditions: 4 (0.20 mmol), 2 (0.70 mmol), [Rh(OH)(L*)]2 (3 mol% of Rh), K3PO4 (for 4a–f; 1 equiv.) in 1,4-dioxane (0.8 mL) or KHF2 (for 4g–i; 1 equiv.) in toluene (0.8 mL) at 60 °C for 12 h.
Isolated yields.
Determined by chiral HPLC analysis.
5 mol% of Rh was used.
For 24 h.
At 80 °C.
|
1d,e |
L3
|
COPh |
Ph (4a) |
80 (5a) |
84 |
2 |
L3
|
COPh |
4-ClC6H4 (4b) |
89 (5b) |
84 |
3 |
L3
|
COPh |
4-CF3C6H4 (4c) |
95 (5c) |
86 |
4d,e |
Bn-tfb* |
CO2Et |
Ph (4d) |
63 (5d) |
81 |
5 |
L3
|
CO2CH(CF3)2 |
Ph (4e) |
70 (5e) |
84 |
6 |
L3
|
CO2(t-Bu) |
CO2(t-Bu) (4f) |
99 (5f) |
81 |
7 |
Bn-tfb* |
NO2 |
p-Tolyl (4g) |
92 (5g) |
89 |
8e |
Bn-tfb* |
NO2 |
4-ClC6H4 (4h) |
80 (5h) |
89 |
9e,f |
Bn-tfb* |
NO2 |
4-MeOC6H4 (4i) |
70 (5i) |
89 |
The addition of cyclobutylboronic acid (6) or cyclopentylboronic acid (7) to alkenylsulfone 1a under the same reaction conditions as for cyclopropylboronic acid (2) did not take place and 1a was recovered intact (eqn (1)). On the other hand, the use of n-butylboronic acid (8) gave only saturated sulfone 9 in 26% yield, indicating that the reduction of 1a proceeds via the formation of a hydridorhodium generated by β-hydrogen elimination of a n-butylrhodium species (eqn (2)). The results also imply that the transmetalation of the Rh with cyclobutyl- and cyclopentylboronic acid does not take place under the present reaction conditions.
| 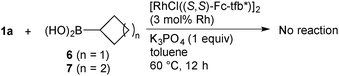 | (1) |
|  | (2) |
Transfer of a substituted cyclopropyl group also took place under the rhodium catalysis (
eqn (3) and (4)). Thus, the addition of a racemic cyclopropylboronate
10 having
trans-2-phenyl to methyl vinyl ketone (
4j) in the presence of [Rh(OH)((
S,
S)-Fc-tfb*)]
2 proceeded to give the addition product
11 in 68% yield (
eqn (3)). The relative configuration of
11 was determined to be
trans, indicating that the transmetalation and the following insertion took place with retention of the configuration.
24 A kinetic resolution of the racemic
10 was also observed (10% ee with Fc-tfb* and 25% ee with Bn-tfb*). In the reaction of deuterated
10-
d2, migration of deuterium, which should be due to the β-hydrogen elimination, was not observed (
eqn (4)). Shintani and Nozaki reported that the polymerization of 3,3-diarylcyclopropenes catalyzed by a rhodium complex, where 1,4-rhodium migration of a cyclopropylrhodium(
I) species
cis to an aromatic ring takes place to form an arylrhodium(
I) intermediate.
25 In the reaction of cyclopropylboronate
10, such a 1,4-rhodium migration was not observed, and thus the result also supports that the intermediate cyclopropylrhodium(
I) is
trans to the phenyl group.
| 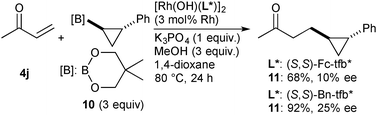 | (3) |
| 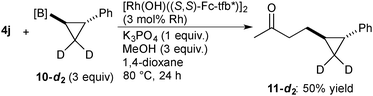 | (4) |
In summary, we have developed Rh-catalyzed asymmetric addition of cyclopropylboronic acids to electron-deficient alkenes. The Rh complexes coordinated with chiral diene ligands based on a tetrafluorobenzobarrelene framework displayed high catalytic activity and enantioselectivity. The addition of a substituted-cyclopropyl group proceeded with the stereoretention, indicating that the transmetalation and the subsequent carborhodation proceed with the retention of the configuration.
This work was supported by JSPS KAKENHI Grant Number 24550117. We thank Prof. A. Osuka for X-ray crystallographic analysis of compound 3a.
Notes and references
- For reviews, see:
(a) M. P. Sibi and S. Manyem, Tetrahedron, 2000, 56, 8033 CrossRef CAS;
(b) N. Krause and A. Hoffmann-Röder, Synthesis, 2001, 171 CrossRef CAS;
(c) K. Fagnou and M. Lautens, Chem. Rev., 2003, 103, 169 CrossRef CAS PubMed;
(d) T. Hayashi and K. Yamasaki, Chem. Rev., 2003, 103, 2829 CrossRef CAS PubMed;
(e) S. Darses and J.-P. Genet, Eur. J. Org. Chem., 2003, 4313 CrossRef CAS PubMed;
(f) J. Christoffers, G. Koripelly, A. Rosiak and M. Rössle, Synthesis, 2007, 1279 CrossRef CAS PubMed;
(g) N. Miyaura, Bull. Chem. Soc. Jpn., 2008, 81, 1535 CrossRef CAS;
(h) J. D. Hargrave, J. C. Allen and C. G. Frost, Chem. – Asian J., 2010, 5, 386 CrossRef CAS PubMed;
(i) H. J. Edwards, J. D. Hargrave, S. D. Penrose and C. G. Frost, Chem. Soc. Rev., 2010, 39, 2093 RSC;
(j) P. Tian, H.-Q. Dong and G.-Q. Lin, ACS Catal., 2012, 2, 95 CrossRef CAS.
-
(a) Y. Takaya, M. Ogasawara, T. Hayashi, M. Sakai and N. Miyaura, J. Am. Chem. Soc., 1998, 120, 5579 CrossRef CAS . For selected examples of the early studies, see: ;
(b) M. T. Reetz, D. Moulin and A. Gosberb, Org. Lett., 2001, 3, 4083 CrossRef CAS PubMed;
(c) M. Kuriyama, K. Nagai, K. Yamada, Y. Miwa, T. Taga and K. Tomioka, J. Am. Chem. Soc., 2002, 124, 8932 CrossRef CAS PubMed;
(d) J. G. Boiteau, R. Imbos, A. J. Minnaard and B. L. Feringa, Org. Lett., 2003, 5, 681 CrossRef CAS PubMed;
(e) Y. Iguchi, R. Itooka and N. Miyaura, Synlett, 2003, 1040 CAS;
(f) C. Defieber, J.-F. Paquin, S. Serna and E. M. Carreira, Org. Lett., 2004, 6, 3873 CrossRef CAS PubMed.
- For selected examples, see: Cu:
(a) H. Mizutani, S. J. Degrado and A. H. Hoveyda, J. Am. Chem. Soc., 2002, 124, 779 CrossRef CAS PubMed;
(b) A. Alexakis, C. Benhaim, S. Rosset and M. Humam, J. Am. Chem. Soc., 2002, 124, 5262 CrossRef CAS PubMed;
(c) I. H. Escher and A. Pfaltz, Tetrahedron, 2000, 56, 2879 CrossRef CAS;
(d) B. L. Feringa, Acc. Chem. Res., 2000, 33, 346 CrossRef CAS PubMed;
(e) M. Kanai and K. Tomioka, Tetrahedron Lett., 1995, 36, 4275 CrossRef CAS;
(f) Q.-L. Zhou and A. Pfaltz, Tetrahedron, 1994, 50, 4467 CrossRef CAS;
(g) M. Van Klaveren, F. Lambert, D. J. F. M. Eijkelkamp, D. M. Grove and G. van Koten, Tetrahedron Lett., 1994, 35, 6135 CrossRef CAS;
(h) G. M. Villacorta, C. Pulla Rao and S. L. Lippard, J. Am. Chem. Soc., 1988, 110, 3175 CrossRef CAS ; Ni: ;
(i) M. Asami, K. Usui, S. Higuchi and S. Inoue, Chem. Lett., 1994, 297 CrossRef CAS;
(j) J. F. G. A. Jansen and B. L. Feringa, Tetrahedron: Asymmetry, 1992, 3, 581 CrossRef CAS;
(k) A. Corma, M. Iglesias, M. Victoria Martín, J. Rubio and F. Sánchez, Tetrahedron: Asymmetry, 1992, 3, 845 CrossRef CAS;
(l) M. Uemura, R. Miyake, K. Nakayama and Y. Hayashi, Tetrahedron: Asymmetry, 1992, 3, 713 CrossRef CAS;
(m) C. Bolm and M. Ewald, Tetrahedron Lett., 1990, 31, 5011 CrossRef CAS;
(n) K. Soai, T. Hayasaka and S. Ugajin, J. Chem. Soc., Chem. Commun., 1989, 517 Search PubMed.
-
The Organometallic Chemistry of the Transition Metals, ed. R. H. Crabtree, John Wiley & Sons, Inc., Hoboken, New Jersey, 2014 CrossRef CAS. For a review of hydride metal complexes, see: H. D. Kaesz and R. B. Saillant, Chem. Rev., 1972, 72, 231 CrossRef CAS . For an example of the synthesis of RhH(PPh3)3 from RhCl(PPh3)3 and (i-Pr)3Al, see: W. Keim, J. Organomet. Chem., 1968, 14, 179 CrossRef.
-
(a) T. Nishimura, Y. Yasuhara and T. Hayashi, Org. Lett., 2006, 8, 979 CrossRef CAS PubMed;
(b) T. Nishimura, A. Ashouri, Y. Ebe, Y. Maeda and T. Hayashi, Tetrahedron: Asymmetry, 2012, 23, 655 CrossRef CAS PubMed . For an example of the addition of methyl- and n-butylboronic acid to alkynoate, see: ;
(c) Y. Yasuhara, T. Nishimura and T. Hayashi, Chem. Commun., 2010, 46, 2130 RSC.
-
(a) R. H. Crampton, S. E. Hajjaji, M. E. Fox and S. Woodward, Tetrahedron: Asymmetry, 2009, 20, 2497 CrossRef CAS PubMed;
(b) K. Yoshida, N. Akashi and A. Yanagisawa, Tetrahedron: Asymmetry, 2011, 22, 1225 CrossRef CAS PubMed.
-
(a) J. Siewert, R. Sandmann and P. von Zezschwitz, Angew. Chem., Int. Ed., 2007, 46, 7122 CrossRef CAS PubMed;
(b) A. Kolb, S. Hirner, K. Harms and P. von Zezschwitz, Org. Lett., 2012, 14, 1978 CrossRef CAS PubMed;
(c) A. Kolb, W. Zuo, J. Siewert, K. Harms and P. von Zezschwitz, Chem. – Eur. J., 2013, 19, 16366 CrossRef CAS PubMed ; for an example of methylation of ketimines, see: ;
(d) S. Hirner, A. Kolb, J. Westmeier, S. Gebhardt, S. Middek, K. Harms and P. von Zezschwitz, Org. Lett., 2014, 16, 3162 CrossRef CAS PubMed.
-
(a) A. Ros and V. K. Aggarwal, Angew. Chem., Int. Ed., 2009, 48, 6289 CrossRef CAS PubMed;
(b) C. Zhang and J. Yun, Org. Lett., 2013, 15, 3416 CrossRef CAS PubMed ; for an example of the addition of α-amido benzyltrifluoroborates to carbonyls, see: ;
(c) A. W. Buesking and J. A. Ellman, Chem. Sci., 2014, 5, 1983 RSC.
- T. Shibata, H. Tabira and K. Soai, J. Chem. Soc., Perkin Trans. 1, 1998, 177 RSC.
- For selected examples, see:
(a) P. W. Smith, P. A. Wyman, P. Lovell, C. Goodacre, H. T. Serafinowska, A. Vong, F. Harrington, S. Flynn, D. M. Bradley, R. Porter, S. Coggon, G. Murkitt, K. Searle, D. R. Thomas, J. M. Watson, W. Martin, Z. Wu and L. A. Dawson, Bioorg. Med. Chem. Lett., 2009, 19, 837 CrossRef CAS PubMed;
(b) P. E. Harrington, M. P. Bourbeau, C. Fotsch, M. Frohn, A. J. Pickrell, A. Reichelt, K. Sham, A. C. Siegmund, J. M. Bailis, T. Bush, S. Escobar, D. Hickman, S. Heller, F. Hsieh, J. N. Orf, M. Rong, T. San Miguel, H. Tan, L. Zalameda and J. G. Allen, Bioorg. Med. Chem. Lett., 2013, 23, 6396 CrossRef CAS PubMed;
(c) Q.-R. Zhou, X.-Y. Wei, Y.-Q. Li, D. Huang and B.-G. Wei, Tetrahedron, 2014, 70, 4799 CrossRef CAS PubMed.
- X. Zeng, J. J. Gao, J. J. Song, S. Ma, J.-N. Desrosiers, J. A. Mulder, S. Rodriguez, M. A. Herbage, N. Haddad, B. Qu, K. R. Fandrick, N. Grinberg, H. Lee, X. Wei, N. K. Yee and C. H. Senanayake, Angew. Chem., Int. Ed., 2014, 53, 12153 CrossRef CAS PubMed.
- For an example of non-asymmetric conjugate addition of cyclopropyl cuprates, see: J. P. Marino and L. J. Browne, J. Org. Chem., 1976, 41, 3629 CrossRef CAS.
- Cyclopropylboronic acid has been often used in Pd- and Cu-catalyzed coupling reactions, which include reductive elimination forming the C–C or the C–N bond from the cyclopropylmetal species. For selected examples, see:
(a) J. P. Hildebrand and S. P. Marsden, Synlett, 1996, 893 CrossRef CAS PubMed;
(b) X. Z. Wang and M. Z. Deng, J. Chem. Soc., Perkin Trans. 1, 1996, 2663 RSC;
(c) S. M. Zhou, M. Z. Deng, L. J. Xia and M. H. Tang, Angew. Chem., Int. Ed., 1998, 37, 2845 CrossRef CAS;
(d) D. J. Wallace and C. Chen, Tetrahedron Lett., 2002, 43, 6987 CrossRef CAS;
(e) T. Tsuritani, N. A. Strotman, Y. Yamamoto, M. Kawasaki, N. Yasuda and T. Mase, Org. Lett., 2008, 10, 1653 CrossRef CAS PubMed.
- For reviews, see:
(a) C. Defieber, H. Grützmacher and E. M. Carreira, Angew. Chem., Int. Ed., 2008, 47, 4482 CrossRef CAS PubMed;
(b) R. Shintani and T. Hayashi, Aldrichimica Acta, 2009, 42, 31 CAS;
(c) C. G. Feng, M.-H. Xu and G.-Q. Lin, Synlett, 2011, 1345 CAS.
- T. Nishimura, H. Kumamoto, M. Nagaosa and T. Hayashi, Chem. Commun., 2009, 5713 RSC.
- T. Nishimura, Y. Takiguchi and T. Hayashi, J. Am. Chem. Soc., 2012, 134, 9086 CrossRef CAS PubMed.
- For examples of asymmetric arylation and alkylation of 2-pyridylsulfones, see:
(a) P. Mauleón and J. C. Carretero, Org. Lett., 2004, 6, 3195 CrossRef PubMed;
(b) P. Mauleón and J. C. Carretero, Chem. Commun., 2005, 4961 RSC;
(c) P. Mauleón, I. Alonso, M. R. Rivero and J. C. Carretero, J. Org. Chem., 2007, 72, 9924 CrossRef PubMed;
(d) J.-N. Desrosiers, W. S. Bechara and A. B. Charette, Org. Lett., 2008, 10, 2315 CrossRef CAS PubMed;
(e) P. H. Bos, A. J. Minnaard and B. L. Feringa, Org. Lett., 2008, 10, 4219 CrossRef CAS PubMed;
(f) P. H. Bos, B. Maciá, M. Á. Fernández-Ibáñez, A. J. Minnaard and B. L. Feringa, Org. Biomol. Chem., 2010, 8, 47 RSC.
-
(a) K. Okamoto, T. Hayashi and V. H. Rawal, Chem. Commun., 2009, 4815 RSC;
(b) T. Nishimura, A. Noishiki, G. C. Tsui and T. Hayashi, J. Am. Chem. Soc., 2012, 134, 5056 CrossRef CAS PubMed.
- T. Hayashi, M. Takahashi, Y. Takaya and M. Ogasawara, J. Am. Chem. Soc., 2002, 124, 5052 CrossRef CAS PubMed.
- The solvent was selected depending on the substrates mainly due to the solubility problem.
- The use of Na3PO4 instead of K3PO4 inhibited the double bond isomerization of 1g.
- The hydroxorhodium complexes displayed higher catalytic activity than the corresponding chlororhodium complexes. The absolute configuration of the products was assigned by consideration of the stereochemical reaction pathway. Therefore, the products obtained with (S,S)-R-tfb* ligands in Table 3 have the opposite configuration to those
in Table 2.
- The use of KHF2 as an effective base was reported in the arylation of nitroalkenes, see:
(a) Z.-Q. Wang, C.-G. Feng, S.-S. Zhang, M.-H. Xu and G.-Q. Lin, Angew. Chem., Int. Ed., 2010, 49, 5780 CrossRef CAS PubMed;
(b) K.-C. Huang, B. Gopula, T.-S. Kuo, C.-W. Chiang, P.-Y. Wu, J. P. Henschke and H.-L. Wu, Org. Lett., 2013, 15, 5730 CrossRef CAS PubMed.
- In Pd-catalyzed cross coupling of cyclopropylboronic acids, the stereoretention was observed, see: ref. 13a–c. The coupling reaction with inversion of the configuration of benzylboron reagents was also reported. T. Ohmura, T. Awano and M. Suginome, J. Am. Chem. Soc., 2010, 132, 13191 CrossRef CAS PubMed.
- R. Shintani, R. Iino and K. Nozaki, J. Am. Chem. Soc., 2014, 136, 7849 CrossRef CAS PubMed.
Footnote |
† Electronic supplementary information (ESI) available: Experimental procedures, compound characterization data, and X-ray crystallographic data of compound 3a. CCDC 1047801. For ESI and crystallographic data in CIF or other electronic format see DOI: 10.1039/c5cc02140e |
|
This journal is © The Royal Society of Chemistry 2015 |