DOI:
10.1039/C4AY02297A
(Paper)
Anal. Methods, 2015,
7, 296-300
Detection of Fe(III) and bio-copper in human serum based on fluorescent AuAg nanoclusters†
Received
28th September 2014
, Accepted 4th November 2014
First published on 4th November 2014
Abstract
In this study, a fluorescence assay for the successive determination of Fe3+ and Cu2+ ions based on the quenching fluorescence of composite AuAg nanoclusters (AuAg NCs) was developed. Using this binary fluorescence sensor, the Fe(III) level in a human serum sample can be directly detected without pretreatment. After the nitrification of human serum, the bio-copper level in human serum may be measured with a quick response. Human serum samples were analyzed, and the average concentration of Fe(III) and bio-copper were found to be 2.33 × 10−5 and 2.91 × 10−5 M, respectively. This assay was not only sensitively responsive to blood iron(III) but also to serum copper, suggesting significant potential applications for successively monitoring the Fe(III) and bio-copper levels, and their changes during the progression of a biological process.
1. Introduction
Noble metal clusters are an emerging class of fluorescent nanomaterials, such as Au nanoclusters (NCs) and Ag NCs,1,2 circumventing most of the drawbacks of common fluorescent compounds, which have drawn wide attention in single-molecule optoelectronic nanodevices, biological labeling, optical sensing, novel catalysis, and surface-enhanced Raman spectroscopy (SERS).3–8 Recognition and quantification of metal ions are considerably significant due to the fact that these ions play important roles in various biological and environmental processes.9,10 In the past few years, there have been numerous reports on the design of fluorescent sensors for the detection of various metal ions, such as Hg2+,11 Fe3+,12 Cu2+ and Cd2+,13,14 and Pb2+,15 because of their high sensitivity, specificity, and real-time monitoring with fast response time.
Previous studies have demonstrated that copper and iron deficiency may lead to a wide variety of neurological problems,16,17 cardiovascular disease and kidney damage.18 The development of specific sensors, determining these metal ions in aqueous media, constantly attract attention. Current approaches for detecting Fe3+ and Cu2+ ions include inductively coupled plasma mass spectrometry (ICP-MS),19,20 atomic absorption spectrometry21,22 and electrochemical methods.23,24 Although these methods offer excellent sensitivity, they are rather costly, time-consuming and complex. Most of the developed approaches to detect copper and iron ions are concentrated on the design of selective sensors, only for one kind of metal ion. In contrast, the investigation of sensors on successive or simultaneously detecting two kinds of these ions is rare. Thus, it is important to develop a binary sensor to determine iron and copper in practical samples, especially in the biological samples.
In this study, a type of composite metal nanoclusters, AuAg NCs, with strong fluorescence have been prepared. A fluorescent probe for the successive determination of Fe3+ and Cu2+ ions, based on the quenching fluorescence of AuAg NCs, has been developed. Using this binary fluorescence sensor, a practical application of a human serum sample was performed to determine Fe(III) and bio-copper levels. This assay shows a quick response not only to the blood iron level but also to serum copper, suggesting significant potential applications for successively monitoring Fe(III) and bio-copper concentrations, and their changes during the progression of a biological process.
2. Experimental
2.1 Chemicals
HAuCl4·3H2O, silver nitrate (AgNO3), glutathione (GSH), disodium ethylenediamine tetraacetate (EDTA), NH4F, ferric chloride (FeCl3), and cupric chloride (CuCl2) were purchased from Sinopharm Chemical Reagent Co., Ltd (Shanghai, China). A series of different concentrations of solutions were obtained by dilution. The aqueous solutions were prepared with doubly distilled water. The K+, Ca2+, Na+, Mg2+, Zn2+, Mn2+, Cd2+, Ni2+, Br−, I−, F−, bovine serum albumin (BSA), cysteine, pepsin, Co2+, Fe3+, Cu2+, Pb2+ and Al3+ metal ion solutions and molecules were prepared to examine the metal ion induced emission enhancement or quenching. The concentration of all prepared metal ion solutions was 1 × 10−2 M. All reagents were of analytical reagent grade and used as received.
2.2 Preparation of AuAg NCs
In a typical synthesis, under vigorous stirring (1000 rpm), the AuAg NCs were synthesized using GSH as a template by chemical reduction of HAuCl4, following the literature reported earlier.25 Freshly prepared aqueous solutions of HAuCl4 (20 mM, 0.25 mL), GSH (100 mM, 0.15 mL) and AgNO3 (20 mM, 0.25 mL) were mixed with 4.35 mL of distilled water at 25 °C in a round bottomed flask. The reaction mixture was heated to 110 °C under gentle stirring for 12 h. An aqueous solution of strongly orange-emitting Au/Ag NCs was formed. The orange-emitting Au/AgNC solution could be stored at 4 °C for 6 months with negligible changes in the optical properties.
2.3 AuAg NCs-based sensor for Fe(III) and Cu2+ ions
A series of 20 μL of Fe3+ standard solutions with different concentrations were added to 1 mL of Au/AgNCs, respectively. The reactions were allowed to proceed for 10 min at room temperature, and the fluorescence quenching spectra was then recorded (excitation 450 nm; maximum emission 570 nm). For the determination of Cu2+, there is a certain concentration of Fe3+ (4.9 × 10−7 M) in the target, and thus a series of 20 μL of Cu2+ standard solutions with different concentrations were added to 1 mL of Au/AgNCs, respectively. EDTA was added after the sufficient NH4F (0.1 mol L−1) was fully reacted with Fe3+, and the fluorescence quenching spectra was then recorded (excitation 450 nm; maximum emission 570 nm).
2.4 Human serum sample processing
The serum samples were supplied by healthy volunteers and were stored at 4 °C until use. All experimental procedures were performed in compliance with the relevant laws and institutional guidelines. The untreated serum was used for the detection of Fe3+.
50 μL of the blood sample was diluted with 50 μL of double distilled water, and then 50 μL of concentrated HNO3 was added for 2 h reaction in the disposable centrifuge tube at room temperature. The sample was briefly centrifuged at 5000 rpm for 7 min, when it was completely nitrified. The supernatant was adjusted to neutral using the NaOH solution. This treated serum was used for the detection of Cu2+.
2.5 AuAg NCs-based sensor for Fe(III) and copper in human serum
Take 20 μL of the untreated human serum to the 1 mL AuAg NCs to proceed for 20 min at room temperature; the decrease in the fluorescence intensity was induced by Fe3+, and the concentration of Fe3+ in the sample was quantified according to the calibration curve.
Take 50 μL of the treated serum to the 1 mL AuAg NCs, and then add 50 μL of 0.1 M NH4F and 50 μL of 0.1 M EDTA, sequentially. The fluorescence enhancement after the addition of EDTA was induced by Cu2+. The concentration of Cu2+ in the sample was quantified according to the calibration curve.
2.6 Characterization
The photoluminescence spectra were recorded on an F-4500 (Hitach, Japan) fluorescence spectrometer. UV-vis absorption spectra were recorded by a Lambda 35 spectrophotometer (Perkin-Elmer, USA). Transmission electron microscopy (TEM) was performed on a JEM-2010 transmission electron microscope at 80 kV. The copper level of human serum was also determined by atomic absorption spectrometry using a Perkin-Elmer atomic absorbance spectrophotometer (ASS) (PEAAnalyst 300).
3. Results and discussion
3.1 Preparation and characterization of AuAg NCs
The preparation of AuAg NCs was performed according to the previous method with slight modification.26 Briefly, HAuCl4, AgNO3 and GSH aqueous solutions were allowed to react under gentle stirring at 110 °C for 12 h. A gradual color change appeared within minutes from yellow to colorless, and then to light yellow. The resultant solution exhibited light yellow color under visible light, and there was intense orange luminescence from the solution when irradiated by the UV light. Fig. 1 shows the TEM image of the synthesized AuAg NCs, indicating that the size of the nanoparticles was less than 2 nm with a quite narrow distribution. The UV-vis absorption and fluorescence spectra acquired from AuAg NCs are displayed in Fig. 2. When the as-prepared AuAg NCs are excited by 450 nm light, an emission band at 570 nm is observed, implying the successful preparation of AuAg NCs. The AuAg NCs show excellent stability in water, maintaining excellent photoluminescence. With rhodamine B as the reference, the quantum yield of the as-prepared Au NCs was calculated to be 15.0%.
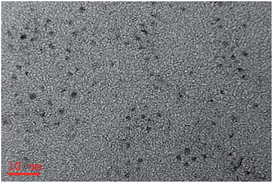 |
| Fig. 1 TEM image of the AuAg NCs. | |
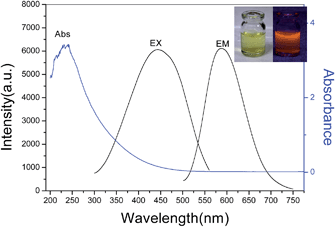 |
| Fig. 2 UV-vis absorption (blue line) and excitation and emission spectra (black line, λex = 450 nm, λem = 570 nm) of the orange-emitting AuAg NCs. Inset: Photographs of an aqueous solution of AuAg NCs under visible light (left) and UV irradiation (λ = 365 nm) (right). | |
3.2 Response of fluorescent AuAg NCs toward different metal ions
The study for sensing metal ions was carried out based on the fluorescent response of the AuAg NCs. With the addition of Fe3+ or Cu2+ ion into the AuAg NCs solution, a rapidly quenched fluorescence occurred. As a comparison, other common metal ions, proteins and small thiol-containing molecules, such as K+, Ca2+, Na+, Mg2+, Zn2+, Mn2+, Cd2+, Ni2+, Co2+, Fe3+, Cu2+, Pb2+, Al3+, Br−, I−, F− as well as BSA, cysteine, and pepsin were investigated under the same conditions. A clear quenching effect similar to that of the Fe3+ and Cu2+ ions was not observed when the concentration of these metal ions and molecules was increased up to 10−4 M, as shown in Fig. 3. Therefore, the quenching fluorescence provides an effective assay for the respective determination of Fe3+ and Cu2+ ions.
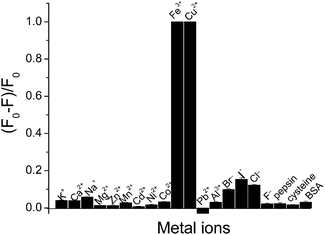 |
| Fig. 3 The fluorescence intensity ratio of changes of the AuAg NCs induced by various ions and biological molecules (1 × 10−4 M). F0 is the initial intensity of the AuAg NCs and F is the fluorescence after the addition of metal ions. The error bars represent the standard deviation of three measurements. | |
3.3 Evaluation of fluorescence response to Fe3+ ion and Cu2+ ion
The fluorescence intensity of the AuAg NCs decreased greatly after the addition of Fe3+. Apparently, the fluorescence intensity of AuAg NCs clearly decreases with the increase in the concentration of Fe3+. Finally, the fluorescence might quench completely along with the increase in the concentration of Fe3+. Fig. 4 shows that the fluorescence intensity of AuAg NCs decreases with an increasing concentration of Fe3+. Due to the high probability of the coexistence of Fe3+ and Cu2+ ions, it is difficult to reveal the source of the quenching fluorescence under the coexistence of these metal ions. The popular chelants, NH4F and EDTA, might be used to differentiate the quenching fluorescence arising from Fe3+ or Cu2+, as illustrated in Scheme 1.
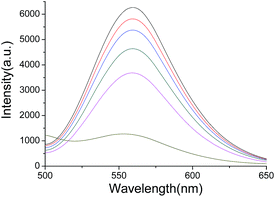 |
| Fig. 4 Fluorescence quenching of AuAg NCs with an increasing Fe(III) concentration (from top to bottom: 0 M, 2 × 10−8 M, 1.9 × 10−7 M, 7.2 × 10−6 M, 3.5 × 10−5 M, and 1.7 × 10−4 M). | |
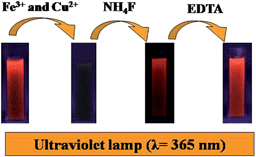 |
| Scheme 1 Schematic diagram of the successive detection of Fe3+ and Cu2+ based on the binary fluorescence sensor of AuAg NCs. | |
The Fe3+ and Cu2+ ions may combine with the COOH and NH2 groups of GSH stabilizing the AuAg NCs, and the combination of metal ions to the surface of the AuAg NCs would induce the fluorescence quenching. However, the response of AuAg NCs towards Cu2+ and Fe3+ were found to be reversible, namely, the quenched florescence was almost recovered to 100% after the addition of NH4F into the mixture system of Fe3+ and AuAg NCs. EDTA was also used to further test the Cu2+ ions. With the addition of EDTA to a mixture of Cu2+ and AuAg NCs, an enhancement of fluorescence up to the initial intensity of fluorescent AuAg NCs was found owing to the formation of a complex between EDTA and Cu2+. These results indicate that the formation of metal complexes makes the metal cations depart from the surface of the AuAg NCs. Therefore, the introduction of NH4F or EDTA to the AuAg NCs provides the possibility to determine Fe3+ and Cu2+ ions, successively.
3.4. Application to human serum samples analysis
As nutrient elements, iron and copper widely exist in biological tissues. Most of the iron exists in the form of protein complexes in biological body fluids and organs. Similarly, the main existing form of copper-containing compounds is present as undissociated copper, such as protein, in the biological body fluids and organs. Human serum samples were analyzed to demonstrate the practical application towards the determination of the Fe(III) and copper levels. When the human serum sample was added to the AuAg NCs, a significant decrease in the fluorescence intensity was found, which indicated that iron and/or copper in the human serum might be directly determined without pretreatment. With the addition of NH4F to the mixture system, a quick and remarkable enhancement of fluorescence occurred and recovered to the initial intensity of the AuAg NCs. As a control experiment, the chelant NH4F was first added to the human serum, and then the biological sample was mixed with the AuAg NCs and no detectable variation in fluorescence occurred, as shown in Fig. S1 (ESI†). These results indicate that the quenched fluorescence resulted from Fe(III) rather than bio-copper in the human serum, which demonstrates that the proposed fluorescent probe can only respond to Fe(III) in the serum, implying that the fluorescence of AuAg NCs would not be interfered by other components in human serum, and this fluorescence assay may selectively determine Fe(III) in the human serum.
To quantitatively detect Fe(III) in the human serum, a calibrate curve was first established and a linear relationship between the degree of quenching fluorescence and Fe3+ concentration within the range of 9 × 10−8–1.1 × 10−6 M was found (Fig. S2†), in which the fluorescence intensity was inversely proportional to the concentration of Fe(III). The equation is displayed as following: (F0 − F)/F0 = 0.03173 + 0.1531c, where F0 is the initial intensity of fluorescent AuAg NCs, and F is the fluorescence intensity after the addition of Fe(III). The linear regression coefficient is 0.9965, and the limit of detection of Fe(III) is 10−8 M. Using this fluorescence sensor, the human serum samples were analyzed to demonstrate the practical application from four volunteers, and the blood Fe(III) concentrations are illustrated in Table 1.
Table 1 The results obtained from the AuAg NCs binary sensor for the Fe(III) and copper levels in human serum samples
Sample |
Fe(III) (M) |
Cu2+ (M) |
1 |
2.11 × 10−5 |
2.75 × 10−5 |
2 |
2.34 × 10−5 |
1.61 × 10−5 |
3 |
1.44 × 10−5 |
4.62 × 10−5 |
4 |
3.44 × 10−5 |
2.65 × 10−5 |
5 |
2.62 × 10−5 |
4.33 × 10−5 |
As discussed aforementioned, this fluorescent probe can only respond to the Cu2+ rather than undissociated copper. Thus, bio-copper in the human serum cannot be directly measured utilizing this fluorescent probe due to few copper ions present in the human body fluids. The same four human serum samples were analyzed to determine the bio-copper levels, following the general procedure. After human serum nitrification, iron and copper existed as an ionic state in the aqueous solution. Before the quantitative analysis of bio-copper, the calibration curve of copper ions was established, as shown in Fig. S3.† Clearly, the addition of various concentrations of Cu2+ to the fluorescent AuAg NCs led to varying degrees of quenching fluorescence, as shown in Fig. S4.† The corresponding ΔF (ΔF = F0 − F) of AuAg NCs increased with the concentration of the metal ion in the solution and shows a linear relationship with the Cu2+ concentration. Therefore, the degree of quenching fluorescence of fluorescent AuAg NCs may be directly reflected by the concentration change of the copper ion. To eliminate the interference of Fe3+ toward the detection of copper, NH4F was added to the nitrification solution of human serum, as shown in Fig. S5.† Then, with the addition of nitrification solution to the AuAg NCs solution, the fluorescence intensity was decreased. The copper concentration can be calculated from the calibration curve. As a result, the bio-copper levels of human serum detected by this assay are also displayed in Table 1. The samples were also determined by AAS, and the obtained results were closely comparable with the result given by the proposed method, suggesting that this assay is of high accuracy. The experimental results indicate that the recovery of this assay is 101.8% corresponding to Cu2+ and 99.6% corresponding to Fe(III), as shown in Table S1.†
4. Conclusions
In summary, a type of composite metal nanoclusters, AuAg NCs, were prepared and a binary fluorescence sensor for successively determining the Fe(III) and copper levels in human serum was developed. The Fe(III) level of human serum can be directly determined without pretreatment with high sensitivity. Human serum samples were analyzed, and the average concentration of Fe(III) was found to be 2.33 × 10−5 M. On the other hand, bio-copper was detected after the nitrification of human serum, and the result is corroborated by AAS, suggesting that this assay is of high accuracy. This proposed assay for the successive detection of Fe(III) and bio-copper levels has high selectivity, low cost, and high sensitivity, which is of potential applications in clinical diagnosis.
Acknowledgements
This work was supported by the National Natural Science Foundation of China (21375036), Hunan Provincial Natural Science Foundation of China (14JJ7054), the Innovation Platform Open Funds for Universities in Hunan Province (13K090), and the Innovation Foundation of Graduate Student of Hunan University of Science and Technology (no. S130028).
Notes and references
- L. Cui, J. Wu, J. Li, Y. Q. Ge and H. X. Ju, Biosens. Bioelectron., 2014, 55, 272 CrossRef CAS PubMed.
- I. Diez and R. H. A. Robin, Nanoscale, 2011, 5, 1963 RSC.
- J. Yu, S. Choi and R. M. Dickson, Angew. Chem., Int. Ed., 2009, 48, 318 CrossRef CAS PubMed.
- T. H. Lee, J. I. Gonzalez, J. Zheng and R. M. Dickson, Acc. Chem. Res., 2005, 38, 534 CrossRef CAS PubMed.
- Y. Z. Lu and W. Chen, Chem. Soc. Rev., 2012, 41, 3594 RSC.
- H. Qian, M. Zhu, Z. Wu and R. Jin, Acc. Chem. Res., 2012, 45, 1470 CrossRef CAS PubMed.
- D. R. Kauffman, D. Alfonso, C. Matranga, H. Qian and R. Jin, J. Am. Chem. Soc., 2012, 134, 10237 CrossRef CAS PubMed.
- W. Li, Z. Gao, R. Su, W. Qi, L. Wang and Z. He, Anal. Methods, 2014, 6, 6789–6795 RSC.
- J. F. Zhang, Y. Zhou, J. Yoon and J. S. Kim, Chem. Soc. Rev., 2011, 40, 3416 RSC.
- Y. Jin, Y. Huang, G. Liu and R. Zhao, Analyst, 2013, 138, 5479 RSC.
- J. Xie, Y. Zheng and J. Y. Ying, Chem. Commun., 2011, 46, 961 RSC.
- J. A. Annie Ho, H. C. Chang and W. T. Su, Anal. Chem., 2012, 84, 3246 CrossRef CAS PubMed.
- X. Liu, N. Zhang, T. Bing and D. Shangguan, Anal. Chem., 2014, 86, 2289 CrossRef CAS PubMed.
- X. L. Tang, X. H. Peng, W. Dou, J. Mao, J. R. Zheng and W. W. Qin, Org. Lett., 2008, 10, 3653 CrossRef CAS PubMed.
- F. Zapata, A. Caballero, A. Espinosa, A. Tárraga and P. Molina, Org. Lett., 2008, 10, 1041 CrossRef PubMed.
- D. W. Domaille, E. Que and C. Chang, Nat. Chem. Biol., 2008, 4, 168 CrossRef CAS PubMed.
- S. R. Jaiser and G. P. Winston, J. Neurol., 2010, 257, 869 CrossRef CAS PubMed.
- J. M. Walsh and Q. J. M. -AnInt, J. Med., 2011, 104, 775 Search PubMed.
- J. G. Wiederhold, C. J. Cramer, K. Daniel, I. Infante, B. Bourdon and R. Kretzschmar, Environ. Sci. Technol., 2010, 44, 4191 CrossRef CAS PubMed.
- G. Li, D. Wu, Y. Wang, W. Xie, X. Zhang and B. Liu, J. Sep. Sci., 2012, 35, 334–340 CrossRef CAS PubMed.
- L. T. Viyannalage, Y. Liu and Q. N. Dimitrov, Langmuir, 2008, 24, 8332 CrossRef CAS PubMed.
- J. Y. Cabon, P. Giamarchi and A. Le Bihan, Anal. Chim. Acta, 2010, 664, 114 CrossRef CAS PubMed.
- E. Tran, A. E. Cohen, R. W. Murray, M. A. Rampi and G. M. Whitesides, J. Am. Chem. Soc., 2009, 131, 2141 CrossRef CAS PubMed.
- M. Cui, Y. Zhao and Q. Song, TrAC, Trends Anal. Chem., 2014, 57, 73 CrossRef CAS PubMed.
- X. L. Guével, V. Trouillet, C. Spies, K. Li, T. Laaksonen, D. Auerbach, G. Jungc and M. Schneider, Nanoscale, 2012, 4, 7624 RSC.
- G. W. Gokel, W. M. Leevy and M. E. Weber, Chem. Rev., 2004, 104, 2723 CrossRef CAS PubMed.
Footnote |
† Electronic supplementary information (ESI) available. See DOI: 10.1039/c4ay02297a |
|
This journal is © The Royal Society of Chemistry 2015 |