DOI:
10.1039/C4AY02212B
(Paper)
Anal. Methods, 2015,
7, 309-312
High performance liquid chromatography determination of formaldehyde in engine exhaust with unsymmetrical dimethylhydrazine as a new derivatization agent
Received
22nd September 2014
, Accepted 4th November 2014
First published on 4th November 2014
Abstract
A rapid, simple and sensitive method for the determination of formaldehyde in exhaust gas was developed. Formaldehyde determination is based on the derivative reaction between formaldehyde and unsymmetrical dimethylhydrazine as a new derivatization agent. The derivative, unsymmetrical dimethylhydrazone, was detected by high performance liquid chromatography equipped with a diode array detector. Under the optimum experimental conditions, the response of the detection system was linear in the range of 0.0392–7.84 mg L−1, with a correlation coefficient of 0.9999. The detection limit (3S/N) of the method was 0.005 mg L−1, the mean recovery was 88.9–102.5%, and the repeatability was 2.6–5.4%. The evaluation of validation data verified that the proposed method was feasible for the determination of formaldehyde in engine exhaust.
1. Introduction
In recent years, the dual worldwide crises of petroleum fuel depletion and environmental degradation have posed increasingly enormous threats to humans. To mitigate these threats from vehicles, methanol/gasoline blended fuel is widely used by many countries. Methanol has excellent combustion properties and low emission characteristics, which has made it an attractive non-petroleum-based alternative fuel for the automotive industry,1,2 but it produces more toxic unregulated emissions such as formaldehyde.3–6
Formaldehyde is considered as a carcinogen, and it poses a significant hazard to human health.7 Therefore, the reduction of formaldehyde from automobile exhaust gas is very important. However, before taking reduction measures, it is necessary to identify and measure formaldehyde in exhaust gas.
A variety of approaches have been developed for the determination of formaldehyde in engine exhaust, including gas chromatography (GC),8,9 spectrometry,10–12 and high-performance liquid chromatography (HPLC).13–16,18,19 Currently, the method of HPLC is based on the derivative reaction between formaldehyde and the derivatization agent. The most common derivatization agents are 2-diphenylacetyl-1,3-indandione-1-hydrazone,13 4-(N,N-dimethylaminosulfonyl)-7-N-methylhydrazino-2,1,3-benzoxadiazole (MDBDH),14 and 2,4-dinitrophenylhydrazine (DNPH).15,16 However, these methods consume more time, require an acid catalyst and still require several procedures after the derivative reaction, including extraction, dissolution and evaporation.
In this work, unsymmetrical dimethylhydrazine (UDMH) was used as a new derivatization agent to determine the concentration of formaldehyde in automobile exhaust by HPLC.
2. Materials and methods
2.1. Reagents and instruments
A Hewlett Packard HPLC system Series 1100 equipped with a quaternary gradient pump, column oven and UV/VIS diode array detector (DAD) was used. Chromatographic separation was performed on a C18 column (150 mm × 4.6 mm I.D., 5 μm) (Agilent, Palo Alto, CA, USA). All samples were weighed by an AE240 electronic balance (Mettler, USA). Unsymmetrical dimethylhydrazine (UDMH, >99.5% purity as measured by the gas chromatography normalisation method) was obtained from Shanghai Riso Chemical Co., Ltd. Formaldehyde (analytical reagent grade) was purchased from Tianjin Day Peak Chemical Co., Ltd. The methanol used was HPLC grade (Fisher Chemicals). Sodium dihydrogen phosphate and sodium hydroxide (analytical reagent grade) were obtained from Xi'an Chemical Reagent Factory. The water used was deionised and further purified via an E-Pure 4-Holder system (Barnstead).
2.2. Preparation of UDMH solution
76.0 μL UDMH was pipetted into a 100 mL volumetric flask by microsyringe and brought to volume by water. The concentration of the UDMH solution was 8.0 × 10−3 mol L−1.
2.3. Preparation of the standard formaldehyde solution
1.4 mL formaldehyde was pipetted into a 500 mL volumetric flask containing 0.5 mL 20% H2SO4 and brought to volume by water. The concentration of standard formaldehyde solution was determined by the iodometric method17 and it was 980.1 mg L−1. Using different volumes of formaldehyde solution, a series of the standard formaldehyde solution was prepared at concentrations of 0.0392, 0.0980, 0.196, 0.294, 0.392, 0.784, 1.57, 3.92, 5.88 and 7.84 mg L−1.
2.4. Derivative reaction between formaldehyde and UDMH
In neutral or weakly acidic conditions, unsymmetrical dimethylhydrazone, a stable product, can be formed when formaldehyde reacts with UDMH. The reaction equation is shown as follows in Fig. 1.
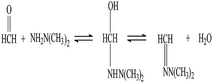 |
| Fig. 1 Derivative reaction of formaldehyde with UDMH. | |
50.0 mL of standard formaldehyde solution was injected into the reaction vessels containing 10.0 mL 0.50 mg mL−1 UDMH solution. The mixture was allowed to react for 20 min at room temperature in the shaker.
2.5. Collection and derivatization of the gas sample
10.0 mL of 0.50 mg mL−1 UDMH solution was added to 100.0 mL ampere bottles that had been subjected to vacuum. The mass of the bottle containing the solution was recorded as m1. The exhaust gas was collected in ampere bottles through pipes from the exit of the engine exhaust manifold of cars fuelled with methanol/gasoline blends named M10, M20, M80 and M90, based on the volume fraction of methanol (10%, 20%, 80% and 90%), respectively, until no bubbles appeared in the bottles. The mass of the bottle filled with the exhaust gas was recorded as m2. Therefore, the mass of the collected exhaust gas was calculated and recorded as m2 − m1. Every sample was collected in five sets, respectively. The mixture solution was allowed to react in the shaker for 20 min at room temperature and was reserved for determination.
2.6. Chromatographic conditions
The mobile phase was methanol: 15 mM phosphate buffer solution (pH = 6.5) (20
:
80, v/v), and filtered through a 0.45 μm membrane filter, prior to use. The flow rate and column temperature were set at 1.0 mL min−1 and 30 °C, respectively. The detection wavelength for the formaldehyde-UDMH derivative was set at 237 nm. The injection volume of sample solution was 10.0 μL.
3. Results and discussion
3.1. Ultraviolet spectrum of the reaction between formaldehyde and UDMH
10.0 mL 0.50 mg mL−1 UDMH solution was mixed with 10.0 mL of standard formaldehyde solution of different concentrations (0.0392, 0.0980, 0.196, 0.294, 0.392 mg L−1), respectively. The ultraviolet spectrum was obtained with the UDMH solution as reference solution. The result was shown in Fig. 2, which revealed that the relationship between concentration of formaldehyde solution and the absorbance of derivative was linear.
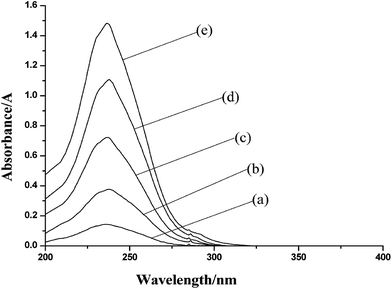 |
| Fig. 2 The Ultraviolet spectrum of UDMH mixed with formaldehyde of different concentrations: (a) 0.0392 mg L−1; (b) 0.0980 mg L−1; (c) 0.196 mg L−1; (d) 0.294 mg L−1; (e) 0.392 mg L−1. | |
3.2. Optimization of the derivatization procedure
3.2.1. Optimization of the pH of derivative reaction.
To optimise the pH of the buffer solution, 10.0 mL of phosphate buffer solution in the range of pH 2.0–7.0 was used. 10.0 μL of UDMH and 50.0 mL standard formaldehyde solution were injected into phosphate buffer solution for a fixed derivation time. The results are presented in Fig. 3. The peak area increased with the pH of the buffer solution in the range of 2.0–6.0. When the pH reached 6.0, the peak area was at a maximum. When the pH was further increased to 7.0, the peak area no longer increased significantly. In the following studies, the phosphate buffer solution of pH = 6.5 was utilised as the optimum buffer.
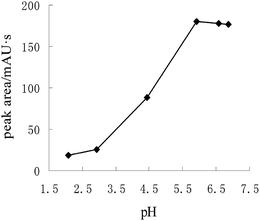 |
| Fig. 3 The influence of pH to the derivative reaction. | |
3.2.2. Optimization of the derivation temperature.
The reaction vessel containing 10.0 mL of UDMH solution and 50.0 mL standard formaldehyde solution was shaken for a fixed time at 20, 30, 40, 50 or 60 °C, respectively. The experiment result showed that the temperature does not affect the derivative reaction. Therefore, room temperature was selected as the optimum temperature.
3.2.3. Optimization of the derivatization agent concentration.
To optimise the concentration of derivatization agent, 1.0, 2.0, 4.0, 6.0, 8.0 and 10.0 μL of UDMH and standard formaldehyde solution were injected into 10.0 mL of phosphate buffer solution for a fixed derivation time. The results, shown in Fig. 4, revealed that the peak area increased with UDMH concentration in the range of 0.10–0.48 mg mL−1. As the concentration of UDMH increased further, the peak area remained constant, which indicated that the derivatization had run to completion. Therefore, 0.50 mg mL−1 of UDMH solution was adopted as the derivatization agent in the following studies.
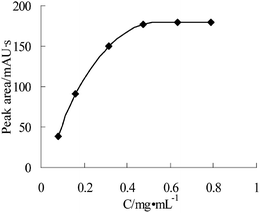 |
| Fig. 4 The influence of derivatization agent concentration to the derivative reaction. | |
3.2.4. Optimization of derivation time.
50.0 mL of standard formaldehyde solution and 10.0 μL of UDMH were slowly injected into the reaction vessel at room temperature. The solution was determined by HPLC at a reaction time of 5, 10, 20, 30, 40, 50 and 60 min, respectively. The result showed that the derivative reaction could be guaranteed to complete within 20 min. Therefore, 20 min was selected as the optimum derivation time.
3.3. Linearity, detection limit, repeatability and recovery
3.3.1. Linearity and detection limit.
Under the optimal conditions, the method presented had good linearity in the range of 0.0392–7.84 mg L−1. The regression equation of the standard curve was as follows:
where A is the peak area of the formaldehyde derivatives, and C is the formaldehyde concentration. Note that the correlation coefficient was 0.9999, and under these conditions, the detection limit was 0.005 mg L−1.
3.3.2. Repeatability.
In order to investigate the repeatability of this method, every sample was collected in five sets, respectively. According to the abovementioned method, the formaldehyde contents of four different proportions of methanol/gasoline blended fuel exhaust gas samples were detected by HPLC. The repeatability of this method, expressed as the RSD (n = 5), was determined by five sets of sample. The results were shown in Table 1, and the RSD was ≤5.4%. This demonstrated that our sample preparation methodology and determination was satisfactory.
Table 1 Repeatability of the assay (n = 5)
Sample |
Formaldehyde content/mg L−1 |
RSD/% |
M10 |
0.196 |
0.190 |
0.200 |
0.209 |
0.218 |
5.4 |
M20 |
0.226 |
0.223 |
0.239 |
0.225 |
0.231 |
2.8 |
M80 |
0.520 |
0.528 |
0.494 |
0.525 |
0.525 |
2.7 |
M90 |
0.492 |
0.467 |
0.489 |
0.499 |
0.479 |
2.6 |
3.3.3. Recovery.
Recovery determination can reflect the derivatization efficiency and sample loss during sample preparation. Adding known amounts of formaldehyde standards in the reaction vessel before sample derivatization can be used to assess the recovery of formaldehyde in real samples. Percentage recovery was determined from the amount of formaldehyde added compared with the amount found. The rates of recovery calculated were in the range of 88.9–102.5%, as shown in Table 2.
Table 2 Recoveries of the assay
Sample |
Content (mg L−1) |
Added (mg L−1) |
Found (mg L−1) |
Recovery (%) |
M10 |
0.202 ± 0.011 |
0.080 |
0.284 ± 0.014 |
102.5 |
M20 |
0.229 ± 0.006 |
0.090 |
0.309 ± 0.010 |
88.9 |
M80 |
0.518 ± 0.014 |
0.200 |
0.715 ± 0.016 |
98.5 |
M90 |
0.485 ± 0.013 |
0.200 |
0.676 ± 0.019 |
95.5 |
3.4. Sample analysis
Under the abovementioned conditions, exhaust gas from the exit of the engine exhaust manifold of cars fuelled with methanol/gasoline blends named M10, M20, M80 and M90, based on the volume fraction of methanol (10%, 20%, 80% and 90%, respectively) was determined. The chromatogram was shown in Fig. 5. The results shown in Table 1 indicated that formaldehyde emissions increased with the methanol content, which was due to the excess formaldehyde resulting from incomplete oxidation under high temperature and pressure.3,6 However, as the methanol content increased to a certain level, the formaldehyde emissions decreased. The mechanism of this change will be studied further.
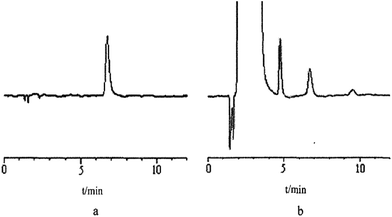 |
| Fig. 5 The chromatogram: (a) the standard formaldehyde solution; (b) exhaust gas from the exit of engine exhaust manifold of cars fuelled with methanol/gasoline blends named M10. | |
4. Conclusion
A rapid, simple and sensitive method for the determination of formaldehyde in exhaust gas was developed. Using UDMH as a new derivatization agent, the formaldehyde in exhaust gas was determined by HPLC. Compared with the method using DNPH as the derivatization agent,15,16,18,19 as shown in Table 3, UDMH has the following advantages. (a) The use of UDMH, which does not absorb in the ultraviolet region, does not interfere in the determination of samples and simplifies the selection of chromatographic separation conditions. (b) The derivative can be determined directly without precipitate or extraction, shortening the analytical time and increasing the repeatability. (d) The derivative, unsymmetrical dimethylhydrazone, has better solubility than 2,4-dinitrophenylhydrazone in the mobile phase. As a result, the mobile phase needed less organic reagent.
Table 3 Comparison of the two different methods
Derivatization agent |
Derivatizing reaction Procedure |
The mobile phase |
LOD/ug L−1 |
λ
max(Derivatization agent)/nm |
λ
detection/nm |
Detector |
Ref. |
UDMH |
pH = 6.5, room temperature, 20 min |
Methanol: PBS (20 : 80, v/v) |
5 |
No absorption |
237 |
HPLC-DAD |
This method |
DNPH |
(A) 1 mol L−1 HCl precipitate, (B) recrystallize, (C) dissolve |
Gradient elute |
0.2 |
341 |
360 |
HPLC-DAD |
15
|
(A) DNPH coated sampling cartridges, (B) elution |
Gradient elute |
0.72 |
341 |
— |
HPLC-MS |
16
|
(A) Micro-wave, (B) centrifuge, (C) dissolve |
Acetonitrile : water (60 : 40, v/v) |
0.12 |
341 |
352 |
HPLC-UV |
18
|
(A) 50 °C, 25 min in MSCX resin (B) filtrate, (C) elution |
Methanol : water(75 : 25, v/v) |
6.0 |
341 |
360 |
HPLC-UV |
19
|
Therefore, we selected UDMH as a new derivatization agent for the determination of formaldehyde in exhaust. The detection limit (3S/N) of the method was found to be 0.005 mg L−1, the mean recovery was 88.9–102.5%, and the repeatability was 2.6–5.4%. The experimental results show that the proposed method can be applied to determine formaldehyde in engine exhaust sensitively, rapidly and precisely.
Acknowledgements
This work was financially supported by the Shaanxi Provincial Education Industrialization Cultivation Project (2011JG02).
References
- M. Abu-zaid, O. Badran and J. Yamin, Energy Fuels, 2004, 18, 312–315 CrossRef CAS.
- E. Zervas, X. Montagne and J. Lahaye, Environ. Sci. Technol., 2002, 36, 2414–2421 CrossRef CAS.
- S. H. Liu, E. R. C. Clemente, T. G. Hu and Y. J. Wei, Appl. Therm. Eng., 2007, 27, 1904–1910 CrossRef CAS PubMed.
- T. G. Hu, Y. J. Wei, S. H. Liu and L. B. Zhou, Energy Fuels, 2007, 21, 71–75 CrossRef.
- J. Li, C. M. Gong, B. Liu, Y. Su, H. L. Dou and X. J. Liu, Energy Fuels, 2009, 23, 4937–4942 CrossRef CAS.
- Y. J. Wei, S. H. Liu, F. J. Liu, J. Liu, Z. Zhu and G. L. Li, Energy Fuels, 2009, 23, 3313–3318 CrossRef CAS.
- A. Sakuragawa, T. Yoneno and K. Inoue, J. Chromatogr. A, 1999, 844, 403–408 CrossRef CAS.
- L. Benning and A. Wahner, J. Atmos. Chem., 1998, 31, 105–117 CrossRef CAS.
- M. C. Hunter, K. D. Bartle, A. C. Lewis, J. B. McQuaid, P. Myers and P. W. Seakins, High Resolut. Chromatogr., 1998, 21, 75–80 CrossRef CAS.
- N. Teshima, S. K. M. Fernández, M. Ueda, H. Nakai and T. Sakai, Talanta, 2011, 84, 1205–1208 CrossRef CAS PubMed.
- Q. Li, P. Stritharathikhum, M. Oshima and S. Motomizu, Anal. Chim. Acta, 2008, 612, 165–172 CrossRef CAS PubMed.
- U. S. Akshath, L. S. Selvakumar and M. S. Thakur, Anal. Methods, 2012, 4, 699–704 RSC.
- S. Uzu, S. Kanda, K. Imai, K. Nakashima and S. Akiyama, Analyst, 1990, 115, 1477–1482 RSC.
- N. Jachmann and U. Karst, J. Anal. Chem., 2001, 369, 47–53 CAS.
- A. Levart and M. Veber, Chemosphere, 2001, 44, 701–708 CrossRef CAS.
- S. M. van Leeuwen, L. Hendriksen and U. Karst, J. Chromatogr. A, 2004, 1058, 107–112 CrossRef CAS PubMed.
- G. Svehla, L. Koltai and L. Erdey, Anal. Chim. Acta, 1963, 29, 442–447 CrossRef CAS.
- X. Xu, R. Su, X. Zhao, Z. Liu and Z. Wang, Talanta, 2011, 85, 2632–2638 CrossRef CAS PubMed.
- H. Wang, J. Ding, X. Du, X. Sun and L. Ding, Food Chem., 2012, 131, 380–385 CrossRef CAS PubMed.
Footnote |
† Permanent address: No. 229 North Taibai Road, Xi'an, Shaanxi, China, 710069. |
|
This journal is © The Royal Society of Chemistry 2015 |
Click here to see how this site uses Cookies. View our privacy policy here.