DOI:
10.1039/C5RA09280A
(Paper)
RSC Adv., 2015,
5, 63050-63055
Calf thymus histone-conjugated magnetic poly(2-oxoethyl methacrylate) microspheres for affinity isolation of anti-histone IgGs from the blood serum of patients with systemic lupus erythematosus
Received
18th May 2015
, Accepted 14th July 2015
First published on 14th July 2015
Abstract
Systemic lupus erythematosus (SLE) is a heterogeneous, inflammatory and multisystem autoimmune disease in which antinuclear antibodies are present in blood often years before clinical symptoms occur. Isolating the antibodies is thus of crucial importance to confirm the diagnosis and prognosis of patients with some autoimmune diseases. Isolation can be performed advantageously using magnetic microspheres, which offer easy and quick manipulation with a magnet and avoid sample dilution. Here, we developed calf thymus histone-conjugated magnetic poly(2-oxoethyl methacrylate) (POEMA–His) microspheres using a multiple-stage swelling technique followed by His immobilization. Magnetic POEMA–His microspheres were characterized using scanning and transmission electron microscopy, SQUID, ATR FT-IR spectroscopy, elemental analysis and atomic absorption spectrometry. The microspheres were successfully used for rapid purification of the anti-histone immunoglobulins (IgGs) from blood serum samples of a cohort of systemic lupus erythematosus patients.
Introduction
Magnetic polymer microspheres with a diameter range of one to several micrometers have recently attracted attention for their widespread applications in various fields of biotechnology and medicine, including enzyme immobilization,1 immunoassays,2 drug targeting,3 affinity separations of cells,4 viruses5 and DNA purification.6 The magnetic particles developed for these applications are typically composed of maghemite or magnetite nanoparticles that are embedded in a polymer material that stabilizes them in water media and provides functional groups to conjugate proteins, nucleotides and other biomolecules. The key issue concerning the bioapplication of magnetic microspheres is facilitating the chemical attachment of bioactive ligands to the particle surface. Using iron oxides for the particle core is advantageous because iron oxides have relatively low toxicity.
Different materials have been suggested for fabricating the magnetic microparticles, including polymers,7 biomolecules8 and inorganic materials,9 which includes both degradable polymers, such as poly(ε-caprolactone), poly(lactide-co-glycolide)10 or agarose,6 and non-degradable polymers, such as polystyrene.11 Additionally, various strategies have been used to prepare magnetic polymer microspheres, including suspension,12 emulsion,13,14 microemulsion,15 dispersion polymerization in the presence of iron oxides, and emulsion-templated polymerization using solvent evaporation.16,17 Other approaches involve the deposition of magnetic iron oxides on the microspheres18 and solvent diffusion19 or the precipitation of iron salt inside pores of the monodisperse porous polystyrene microspheres.20 Such particles, however, typically possess broad particle size distribution and lack a high saturation magnetization. Ugelstad pioneered the production of monodisperse magnetic micrometer-size polystyrene particles,21 which has the advantage of size uniformity and thus ensures uniform packing in chromatography columns and equal physical, chemical and biological properties.
This work focused on the synthesis of magnetic poly(glycidyl methacrylate) (PGMA)-based microspheres, which has the advantage of a higher availability of reactive oxirane groups that are suitable for further chemical modifications. To bio-conjugate proteins to the particles, aldehyde groups were introduced into the magnetic microspheres through the simple oxidation of PGMA with sodium periodate. The next aim of this study was to prepare the histone-functionalized magnetic PGMA-based microspheres for purification of anti-histone autoantibodies from blood serum of patients suffering from systemic lupus erythematosus (SLE). This disorder is a model humoral immune response that involves the recognition of intracellular proteins and nucleic acids, which is an important feature of systemic autoimmune diseases. Autoantibodies possessing reactivity to different intracellular antigens that were previously associated with systemic autoimmune diseases22,23 were also found in cancer patients.24 It is well known that anti-nucleosome or anti-chromatin antibodies play a key role in autoimmune disease because these antibodies have been found in ∼90% of SLE patients25 and ∼30% of cancer patients.24 Anti-nucleosome autoantibodies possess both complement-dependent and complement-independent toxicity towards different tumor cells.26 The pathogenic role of anti-nucleosome autoantibodies (ANAs) in autoimmunity was extensively studied,26,27 however, little has been done to elucidate the functions of ANAs in cancer patients.24 Anti-histone antibodies can be directed against single histones, histone complexes or histone–DNA complexes. In particular, histone H2A–H2B dimers frequently elicit humoral anti-histone responses, especially in cases of drug-induced lupus erythematosus.28 Anti-histone antibodies were found in a variety of autoimmune disorders, including inflammatory, hepatic, malignant, infectious and rheumatic diseases.29 Detecting these antibodies in blood serum is thus useful for diagnosing specific diseases and for the prognostication and evaluation of treatment efficacy.
Recently, affinity chromatography on a column with calf thymus histone H1-Sepharose was used for purification of anti-histone antibodies from blood serum of the multiple myeloma and SLE patients. It was found that these antibodies were capable of cleaving histone H1 and myelin basic protein30,31 and proteolytic activity of IgG-antibodies isolated from blood serum of SLE patients correlated with the disease severity.32 It was proved that the appearance of immunoglobulins possessing proteolytic activity (so-called “protabzymes”) is a prominent characteristic of the autoantibodies tightly associated with the autoimmune disorders.33 Thus, characterization of specificity (catalytic activity, cross-reactivity, etc.) of anti-histone antibodies of blood serum of the autoimmune patients is important for the diagnostic purposes. In the present study, we demonstrated that the magnetic poly(2-oxoethyl methacrylate) (POEMA) microspheres bearing the histones of calf thymus are effective tools for rapid one-step isolation of anti-histone IgGs from blood serum in a large cohort of the SLE patients.
Materials and methods
Materials
Glycidyl methacrylate (GMA; Fluka; Buchs, Switzerland) and ethylene dimethacrylate (EDMA; Ugilor S.A., France) were vacuum distilled before use. Dibutyl phthalate (DBP), dioctyl adipate (DOA), dodecan-1-ol, (2-hydroxyethyl) cellulose (HEC), sodium dodecyl sulfate (SDS) and Tween-20 were purchased from Fluka (Buchs, Switzerland). 2,2′-Azobisisobutyronitrile (AIBN) and HRP-conjugated anti-human (high-chain specific) rabbit IgGs were purchased from Sigma-Aldrich (St. Louis, MO, USA) and cyclohexanol was purchased from Lach-Ner (Neratovice, Czech Republic). Poly(vinyl alcohol) Wacker W25/140 (PVA; Mw 80
000; 86–89% hydrolyzed) was purchased from Wacker (Burghausen, Germany). Tris-buffered saline (TBS; 150 mM NaCl and 20 mM Tris HCl; pH 7.4) was freshly prepared. Sodium dodecyl sulfate (SDS; Serva, Germany) was prepared as a 10% aqueous solution and used for preparation of SDS-loading buffer for electrophoresis (see below). Calf thymus histones were kindly donated by Prof. Maxim Lootsik from the Institute of Cell Biology, NAS of Ukraine. All other chemicals were from Lach-Ner.
Synthesis of magnetic poly(2-oxoethyl methacrylate) (POEMA) microspheres
The macroporous PGMA microspheres were prepared using a modification of an earlier described procedure.34 First, polystyrene latex seeds (750 nm) were prepared by emulsifier-free emulsion polymerization, which was followed by repeated swelling of the seeds (0.3 g) with a dispersion of DBP (14.7 g) and DOA (0.3 g) in 0.25 wt% aqueous SDS under sonication. The DBP- and DOA-swollen PS seeds were then swollen with a dispersion of monomers and initiator (192 g GMA, 128 g EDMA and 1.6 g AIBN) and with a dispersion of porogens (8 g dodecan-1-ol and 457 g cyclohexanol) in 0.1 wt% aqueous SDS (1.65 l) for 5 h. The dispersion of monomer/porogen-swollen PS seeds in 0.1 wt% SDS solution was then polymerized in 3 wt% aqueous PVA (240 ml) and 6 wt% aqueous HEC (240 ml) at 70 °C for 16 h under CO2 atmosphere. The resulting macroporous PGMA microspheres were washed with water, methanol, toluene, and methanol (three times each with 1 l), and dried in air.
The PGMA microspheres (7 g) were then hydrolyzed using 0.2 M H2SO4 (50 ml) at room temperature for 5 h under shaking (80 rpm) and at 60 °C for 16 h. The resulting poly(2,3-dihydroxypropyl methacrylate) (PDHPMA) microspheres were washed 5× with water, separated by centrifugation and washed 4× with ethanol. The PDHPMA particles (3.5 g) were then rendered magnetic according to the following procedure. The microspheres were washed with 0.03 M HCl, separated by centrifugation, and 1.7 M FeCl2 solution was added (5 ml). The particles were again separated by centrifugation to load the pores with the FeCl2, dispersed in the 1.7 M FeCl2 solution once more and separated by filtration. The microspheres were then treated with 1.35 M ammonia (5 ml) under Ar atmosphere. The mixture was then vortexed, and water was added (5 ml). After 15 min, the particles were separated by centrifugation and washed twice with water under an Ar atmosphere. The particle suspension in water (10 ml) was then again treated with 1.35 M ammonia (0.5 ml) at room temperature for 16 h while being stirred under air. Finally, the resulting magnetic PDHPMA particles were washed ten times with water, once with 0.03 M HCl (15 ml) and once again with water. This enabled the formation of colloidal magnetite, which was then easily removed both from the surface and from the outside the microspheres. The introduction of magnetite inside the microspheres was repeated once more, as described above.
The magnetic PDHPMA microspheres (3.5 g) were then oxidized with an aqueous solution (7 ml) of sodium periodate (1.5 g) at room temperature for 1 h while shaking under an Ar atmosphere to yield magnetic poly(2-oxoethyl methacrylate) (POEMA) microspheres.
Physico-chemical characterization of the microspheres
The particle size and size distribution were analyzed using a Qanta SF 200 scanning electron microscope (SEM; FEI; Brno, Czech Republic). Sections of the magnetic microspheres embedded in LR white resin prepared using a LKB III ultramicrotome were observed on a Spirit G2 Tecnai transmission electron microscope (TEM; FEI). Infrared spectra were recorded with a Perkin Elmer PARAGON 1000 PC FT-IR spectrometer equipped with Specac MKII Golden Gate Single Reflection ATR System with diamond crystal and a 45° angle of incidence. Elemental analysis was performed on a Perkin-Elmer 2400 CHN apparatus (Norwalk, CT, USA). The Fe content was determined by the atomic absorption spectrometry (AAS Perkin-Elmer 3110) of an extract from a sample obtained by treatment with 70% perchloric and 65% nitric acid at 100 °C for 30 min. Magnetic properties were measured using a MPMS5 superconducting quantum interference device (SQUID; Quantum Design; San Diego, CA, USA) at 3 and 300 K.
Preparation of magnetic POEMA–His microspheres
A solution of calf thymus histones (1 ml; 5 mg ml−1) in 0.1 M NaHCO3 (pH 8) was incubated with magnetic POEMA microspheres (0.5 ml) at room temperature for 3 h with stirring. After the incubation, 1 M ethanolamine (0.15 ml) was added to the microsphere suspension (1.5 ml) to block the free CHO groups; the reaction continued for 1 h at room temperature. Finally, the resulting magnetic POEMA–His particles were washed three times with TBS buffer to remove soluble protein contaminants.
Isolation of anti-histone IgGs from blood serum of SLE patients
Blood serum (50 μl) of SLE patients diluted with TBS (150 μl) was incubated with magnetic POEMA–His microspheres (20 μl) at room temperature for 1 h while stirring. The microspheres were washed with TBS, 0.01% Tween 20, twice with TBS and the bound anti-histone IgGs proteins were eluted with 4 M urea (20 μl). Then, 4× fold SDS-loading buffer (0.2 M Tris–HCl, pH 6.8, 4% SDS and 2% 2-mercaptoetanol) was added to the extracts, and the proteins were analyzed by 10% SDS-PAGE electrophoresis followed by western blotting and an immunoassay. Anti-histone IgGs were additionally analyzed by a Series 200 HPLC gel filtration (Perkin-Elmer, USA) on a Bio-Sil SEC 250 column (Bio-Rad, Marnes-la-Coquette, France) in phosphate buffer (150 mM NaCl, 10 mM Na2HP04–5 mM NaH2P04; pH 6.8) at 1 ml min−1 flow rate. The column was calibrated by molecular mass standards in the same buffer.
Bioethics confirmation
Serum from the peripheral blood of 6 SLE patients diagnosed in accordance with the American College of Rheumatology and 6 clinically healthy individuals were used in the study. A documented consent was obtained from all of the patients and was approved by the Review Board of the Danylo Halytsky Lviv National Medical University, considering the regulations of the Ministry of Health Protection of Ukraine based on the European Convention for the protection of patients' rights.
Results and discussion
Synthesis of the magnetic POEMA–His microspheres
Multiple swelling polymerization of GMA was a convenient method to produce monodisperse macroporous PGMA microspheres that were 9 μm in diameter. Such a particle size was very suitable for chromatography applications. The PGMA microspheres were subsequently hydrolyzed by sulfuric acid to produce macroporous PDHPMA particles. The microspheres' pores had the advantage of alkaline precipitation of Fe(OH)2 from the FeCl2 aqueous solution, which imbibed the particles. In the presence of air oxygen, the Fe(OH)2 was partly transformed into Fe(OH)3, and magnetite (Fe3O4) was formed by this reaction. In the next step, maghemite (γ-Fe2O3) was formed by air oxidation. Ammonia was preferred as a base because the Fe2+ redox potential is higher in acidic media. The magnetic PDHPMA were then oxidized with sodium periodate to yield POEMA particles containing aldehyde groups.
Fig. 1a and b shows SEM micrographs of both starting and magnetic PDHPMA microspheres, documenting their 9 μm diameter and very narrow particle size distribution characterized by polydispersity index (weight-to-number average diameter) <1.05 determined by measuring ∼500 particles. The introduction of magnetic compounds did not significantly affect either the morphology or the particle size. The presence of iron oxides within the pores of the particles and in the vicinity of the surface was documented on a cross-section of a particle (Fig. 1c). Moreover, the presence of the iron oxide in the particles was confirmed by elemental analysis. Though the starting PGMA particles contained 58.96 and 7.17 wt% of C and H, respectively, their content in the PDHPMA microspheres changed to 53.02 and 7.63 wt%, respectively, and the amount of C and H in the magnetic PDHPMA decreased to 43.52 and 6.05 wt%, respectively, which was due to the introduction of iron oxides inside the particles. At the same time, Fe content in the magnetic PDHPMA particles amounted to 13.71 wt%. After the oxidation of the magnetic PDHPMA particles to magnetic POEMA, the C and H content further decreased, and the amount of Fe increased to 42.05, 5.65 and 14.26 wt%, respectively. This amount of iron oxide was sufficient to establish good magnetic properties for the particles. The magnetic POEMA microspheres were characterized using a SQUID magnetometer that recorded a mild hysteresis at 300 K (Fig. 2). The microspheres exhibited relatively low remanent magnetization, which indicated that they contained a small amount of ferrimagnetic iron oxide in addition to the superparamagnetic and paramagnetic iron oxides. Despite the low remanent magnetization, the particles that were attracted to a magnet during the separation process could be in the absence of magnet easily redispersed in water by shaking. The exact phase composition was not analyzed. Because the saturation magnetization (300 K) of the magnetic POEMA microspheres (containing 14.26 wt% Fe, i.e., 20.4 wt% iron oxide) amounted to 13.3 A m2 kg−1, the saturation magnetization of 100% iron oxide was calculated to be 65.2 A m2 kg−1, i.e., reaching ∼72% of bulk magnetite (90 A m2 kg−1).35 Magnetic measurements at 3 K showed that both coercivity and magnetization moderately increased with decreasing temperature (Fig. 2).
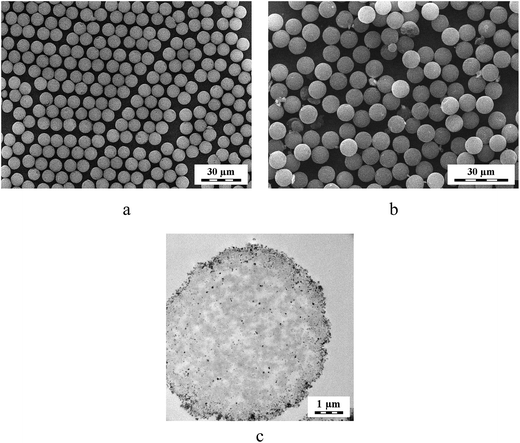 |
| Fig. 1 SEM micrographs of (a) PDHPMA and (b) magnetic PDHPMA microspheres. (c) TEM cross-section of a magnetic PDHPMA microsphere. | |
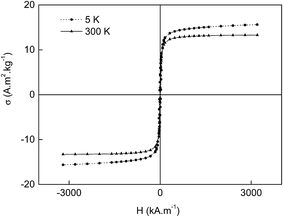 |
| Fig. 2 Hysteresis loops of magnetic POEMA microspheres at 3 and 300 K. | |
Fig. 3 shows the ATR FT-IR spectra of the initial PGMA, PDHPMA and magnetic POEMA microspheres and confirms the successful hydrolysis of PGMA, as the typical band of its oxirane groups at 906 cm−1 was absent in the spectrum of the PDHPMA microspheres. Aldehyde groups of the magnetic POEMA microspheres were overlapped with the carbonyl groups of methacrylates, and small changes in the spectra were observed at 800–1100 cm−1. The main band of iron oxides was observed at 560 cm−1.
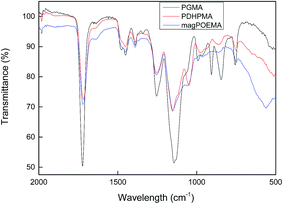 |
| Fig. 3 ATR FT-IR spectra of PGMA, PDHPMA and magnetic POEMA microspheres. | |
Immobilization of the His was achieved through the reaction of aldehyde groups of the magnetic POEMA microspheres with the primary amine of the His.
Biological experiments
As noted in the Introduction, anti-histone antibodies were found in patients with a variety of autoimmune disorders, including inflammatory, hepatic, malignant, infectious and rheumatic diseases.29 Evaluating these antibodies in blood serum might be useful for the diagnosis of specific diseases and for the prognosis and evaluation of treatment efficacy. Hence, the isolation and detection of anti-histone antibodies in blood serum of SLE patients are of diagnostic and prognostic value. Fig. 4 presents the results of the electrophoretic analysis of the anti-histone antibodies purified from blood serum of SLE patients using magnetic POEMA microspheres coupled with total fraction of calf thymus histones (POEMA–His). The incubation of blood serum samples from six SLE patients with POEMA–His allowed for the preferential isolation of polypeptides with the molecular weight of 66, 50 and 25 kDa (Fig. 4A, lanes 1′–6′). The latter two proteins corresponded to heavy and light chains of human IgGs, respectively. The identity of the human IgGs was checked by western blot analysis using HRP-antibodies that are monospecific to heavy chains. A 50 kDA polypeptide intensively bound the anti-human IgGs antibodies (Fig. 4B, lanes 1′–6′).
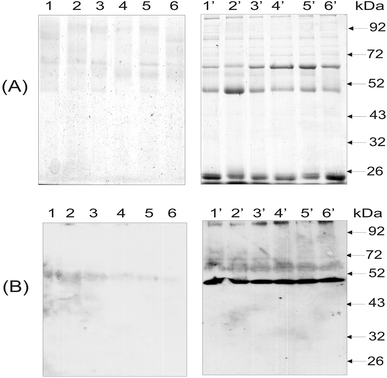 |
| Fig. 4 (A) Electropherograms (10% SDS-PAGE) of proteins isolated from blood serum of healthy human donors (1–6) and patients with systemic lupus erythematosus (1′–6′) by magnetic POEMA–His microspheres. (B) Western blot of isolated proteins detected by HRP-conjugated anti-human (high-chain specific) rabbit IgGs. On the right site of the gels, positions of the protein molecular weight markers are shown. | |
Thus, the protein fractions purified from blood serum on the magnetic POEMA–His affinity sorbent contained the anti-histone antibodies. In contrast to the blood serum of the SLE patients, no anti-histone IgGs were isolated from blood serum of healthy donors (Fig. 4). In that case, neither electrophoresis (Fig. 4A, lanes 1–6) nor western-blot analysis (Fig. 4B, lanes 1′–6′) detected an essential amount of proteins bound to the synthesized microparticles. The homogeneity of the anti-histone antibody was analyzed using HPLC gel filtration (Fig. 5). The antibody consisted of proteins whose molecular weight coincided with the molecular weight of human IgG molecules. The content of other proteins in the anti-histone antibody was less than 20%. Thus, the application of the antigen-coupled microparticles allowed highly purified antibodies to be obtained. It should be stressed that this approach possesses a significant advantage as it allows a complex investigation of the autoantibodies, e.g., anti-histone H1 Ab. This includes not only the detection of Abs, but also their simple and rapid isolation for further analysis, e.g., catalytic activity or cross-reactivity to different antigens. A unique property of the developed microspheres consists in that their porosity significantly increases binding capacity for specific proteins, while the superparamagnetism enables quick and easy magnetic purification avoiding contamination of blood serum proteins and other biomacromolecules.
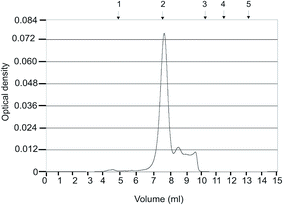 |
| Fig. 5 HPLC gel filtration of the anti-histone IgGs on the Bio-Sil SEC 250 column washed with the phosphate buffer (pH 6.8). The positions of the molecular mass proteins standards are shown above. (1) thyroglobulin (760 kDa), (2) IgG (150 kDa), (3) ovalbumin (44 kDa), (4) myoglobin (17 kDa) and (5) vitamin B12 (1.35 kDa). | |
Conclusions
Magnetic POEMA–His microspheres, 9 μm in diameter, were developed using a multiple-stage swelling technique. The microspheres were uniform in shape and size, which implies that the chemical and physical properties of the particles were consistent. As a result, affinity separation of the biological sample suspensions using a magnetic separator and the magnetic POEMA–His microspheres was efficient and rapid even in diluted biological samples. This enabled a simple purification of anti-histone IgGs from blood serum of patients suffering from systemic lupus erythematosus. The procedure might be suitable not only for patients with systemic lupus erythematosus but also for persons suffering from other autoimmune diseases. Moreover, the particles could be re-suspended in water when the magnet was removed because the particles exhibited predominantly superparamagnetic behavior. Though the magnetic POEMA–His microspheres possessed a lower specific surface area and loading capacity for their biological targets compared with nanoparticles, the introduction of porosity circumvented this disadvantage. Magnetic separation, filtration and washing using magnetic microspheres were more efficient than observed for the nanoparticles and did not require packing of the chromatography columns. The process is amenable to automation with high throughput. The magnetic POEMA–His microspheres can thus be used not only for diagnosing specific diseases but also for monitoring patient prognosis and treatment response.
Acknowledgements
This study was supported by the European FP7 project Nadine, No. 246513. The authors also acknowledge support of Cedars Sinai Medical Center's International Research and Innovation Management Program and the Association for Regional Cooperation in the Fields of Health, Science and Technology (RECOOP HST).
References
- D. S. Jiang, S. Y. Long, J. Huang, H. Y. Xiao and J. Y. Zhou, Biochem. Eng. J., 2005, 25, 15 CrossRef CAS PubMed.
- G. Glöckl, V. Brinkmeier, K. Aurich, E. Romanus, P. Weber and W. Weitschies, J. Magn. Magn. Mater., 2005, 289, 480 CrossRef PubMed.
- H. Chen, A. D. Ebner, M. D. Kaminski, A. J. Rosengart and J. A. Ritter, J. Magn. Magn. Mater., 2005, 293, 616 CrossRef CAS PubMed.
- A. S. Wadajkar, S. Santimano, L. Tang and K. T. Nguyen, Biomaterials, 2014, 35, 654 CrossRef CAS PubMed.
- S. J. McConnell, T. Dinh, M. H. Le and D. G. Spinella, BioTechniques, 1999, 26, 208 CAS.
- P. R. Levison, S. E. Badger, J. Dennis, P. Hathi, M. J. Davies, I. J. Bruce and D. Schimkat, J. Chromatogr. A, 1998, 816, 107 CrossRef CAS.
- J. Ren, H.-Y. Hong, T.-B. Ren and X.-R. Teng, Mater. Lett., 2005, 59, 2655 CrossRef CAS PubMed.
- Z. Chunfu, C. Jinquan, Y. Duanzhi, W. Yongxian, F. Yanlin and T. Jiaju, Appl. Radiat. Isot., 2004, 61, 1255 CrossRef PubMed.
- Y. Sun, L. Duan, Z. Guo, Y. DuanMu, M. Ma, L. Xu, Y. Zhang and N. Gu, J. Magn. Magn. Mater., 2005, 285, 65 CrossRef CAS PubMed.
- M. A. Benoit, B. Baras and J. Gillard, Int. J. Pharm., 1999, 184, 73 CrossRef CAS.
- F. F. Fang, J. H. Kim and H. J. Choi, Polymer, 2009, 50, 2290 CrossRef CAS PubMed.
- X. Liu, Y. Guan, R. Shen and H. Liu, J. Chromatogr. B: Anal. Technol. Biomed. Life Sci., 2005, 822, 91 CrossRef CAS PubMed.
- F. Montagne, O. Mondain-Monval, C. Pichot and A. Elaissari, J. Polym. Sci., Part A: Polym. Chem., 2006, 44, 2642 CrossRef CAS PubMed.
- A. Pich, S. Bhattacharya, A. Ghosh and H. J. P. Adler, Polymer, 2005, 46, 4596 CrossRef CAS PubMed.
- Y. Deng, L. Wang, W. Yang, S. Fu and A. Elaıssari, J. Magn. Magn. Mater., 2003, 257, 69 CrossRef CAS.
- H. Shang, W.-S. Chang, S. Kan, S. A. Majetich and G. U. Lee, Langmuir, 2006, 22, 2516 CrossRef CAS PubMed.
- S. A. Gomez-Lopera, R. C. Plaza and A. V. Delgado, J. Colloid Interface Sci., 2001, 240, 40 CrossRef CAS PubMed.
- E. L. Bizdoaca, M. Spasova, M. Farle, M. Hilgendorff and F. Caruso, J. Magn. Magn. Mater., 2002, 240, 44 CrossRef CAS.
- S.-J. Lee, J.-R. Jeong, S.-C. Shin, J.-C. Kim, Y.-H. Chang, K.-H. Lee and J.-D. Kim, Colloids Surf., A, 2005, 255, 19 CrossRef CAS PubMed.
- J. Ugelstad, A. Berge, T. Ellingsen, R. Schmid, T.-N. Nilsen, P. C. Mork, P. Stenstad, E. Hornes and O. Olsvik, Prog. Polym. Sci., 1992, 17, 87 CrossRef CAS.
- J. Ugelstad, L. Soderberg, A. Berge and J. Bergstrom, Nature, 1983, 303, 95 CrossRef PubMed.
- Y. Sherer, A. Gorstein, M. J. Fritzler and Y. Shoenfeld, Semin. Arthritis Rheum., 2004, 34, 501 CrossRef CAS PubMed.
- J. A. Gomez-Puerta, R. W. Burlingame and R. Cervera, Autoimmun. Rev., 2008, 7, 606 CrossRef CAS PubMed.
- V. P. Torchilin, L. Z. Iakoubov and Z. Estrov, Trends Immunol., 2001, 22, 424 CrossRef CAS.
- E. M. Tan, F.-D. Shi and W. M. Keck, Clin. Exp. Immunol., 2003, 134, 169 CrossRef CAS.
- P.-L. Lima and M. Zouali, Immunol. Lett., 2006, 103, 17 CrossRef PubMed.
- M. Pavlovic, A. Kats, M. Cavallo, R. Chen, J. X. Hartmann and Y. Shoenfeld, Autoimmune Dis., 2010, 462841 Search PubMed.
- G. H. Stummvoll, R. D. Fritsch, B. Meyer, E. Hoefler, M. Aringer, J. S. Smolen and G. Steiner, Ann. Rheum. Dis., 2009, 68, 110 CrossRef CAS PubMed.
- H. Dunorier and S. Muller, Histone autoantibodies, in Autoantibodies, ed. Y. Shoenfeld, P.-L. Meroni and M. E. Gershwin, Elsevier, Amsterdam, 2nd edn, 2007, pp. 169–177 Search PubMed.
- I. Magorivska, R. Bilyy, O. Shalay, V. Loginsky, Y. Kit and R. Stoika, Exp. Oncol., 2009, 31, 1 Search PubMed.
- I. Magorivska, R. Bilyy, A. Havryluk, V. Chopyak, R. Stoika and Y. Kit, J. Mol. Recognit., 2010, 23, 495 CrossRef CAS PubMed.
- Y. Kit, I. Magorivska, V. Chopyak, R. Tolstiak, R. Bilyy and R. Stoika, Novel biological activities of autoantibodies as the potential markers of systemic lupus erythematosus, in Systemic Lupus Erythematosus (SLE): Prevalence, Pathophysiology and Prognosis, ed. S. Koarada, Nova Sci. Publ., Hauppauge, 2013, pp. 101–109 Search PubMed.
- A. Belogurov Jr, A. Kozyr, N. Ponomarenko and A. Gabibov, BioEssays, 2009, 31, 1161 CrossRef PubMed.
- S. Grama, Z. Plichta, M. Trchová, J. Kovářová, M. Beneš and D. Horák, React. Funct. Polym., 2014, 77, 11 CrossRef CAS PubMed.
- R. M. Cornell and U. Schwertmann, The iron oxides: Structure, properties, reactions, occurrence and uses, VCH, New York, 1996, p. 117 Search PubMed.
|
This journal is © The Royal Society of Chemistry 2015 |