Novel dibenzo[a,e]pentalene-based conjugated polymers†
Received
19th August 2013
, Accepted 11th October 2013
First published on 15th October 2013
Abstract
Dibenzo[a,e]pentalene (DBP), a ladder-type fused-ring system with 16π anti-aromatic nature, is integrated into conjugated polymers with oligothiophene building blocks to examine the potential of DBP as a new building block for semiconducting polymers. Depending on the incorporation manner of the DBP unit in the polymer backbone, via the 5,10- or 2,7-positions, the polymers show distinct colours, reflecting the different electronic structures, though the HOMO and LUMO energy levels estimated from cyclic voltammograms are almost the same. Interestingly, the impact of the incorporation manner was observed in the characteristics of their field-effect transistors (FETs). For PDTDBP2Ts, in which the DBP units are integrated into the polymer backbone via the 5,10-positions, the DBP units behave like a “dibenzo-annulated 1,3-butadiene” moiety, and their FET characteristics are strongly affected by orientational ordering and crystallinity, similar to ordinary “donor-only” polymers such as P3HT. On the other hand, iPDTDBP2Ts, in which the whole 16π DBP unit is integrated into the polymer backbone via the 2,7-positions, behave like a certain kind of donor-acceptor polymers, and the FET characteristics are independent of orientational order: the field-effect mobilities are higher than 0.1 cm2 V−1 s−1 regardless of the polymer orientation in the thin film. From these results, we can recognize the 16π anti-aromatic DBP unit as a useful building block with transmutable nature for the development of new conjugated polymers.
Introduction
Ladder-shaped polycyclic aromatic hydrocarbons with extended π-conjugation are of great interest because of their potential applications in opto/electronic devices such as organic light emitting diodes (OLEDs), organic field-effect transistors (OFETs), and organic photovoltaics (OPVs).1 In particular, considerable attention has been paid to the large acene compounds owing to their superior charge carrier mobilities in OFETs.2 On the other hand, similar ladder-shaped aromatic hydrocarbons but with non-alternant structures have recently emerged as potential electronic materials, owing to their relatively low-lying LUMO energy levels and small HOMO–LUMO gaps.3 Among such ladder-shaped, non-alternant hydrocarbons, dibenzo[a,e]pentalene (DBP, Fig. 1), a polycyclic hydrocarbon containing an anti-aromatic pentalene substructure, has received recent attention,4 and in particular, 5,10-diaryl substituted DBPs with increased stability have been evaluated as active semiconducting materials in opto/electronic devices. Kawase and co-workers reported 6,13-diphenyldinaphtho[a,e]pentalene, which can act as a p-channel organic semiconductor both in OFETs and bilayer OPVs with C60, showing decent hole mobility (1.8 × 10−3 cm2 V−1 s−1) and photovoltaic behaviour with power conversion efficiencies (PCEs) of ∼1%, respectively.4c
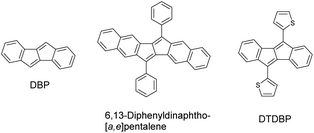 |
| Fig. 1 Molecular structure of dibenzo[a,e]pentalene (DBP) and related compounds. | |
Another interesting DBP-based structure is 5,10-di(2-thienyl)dibenzo[a,e]pentalene (DTDBP), first reported by Cava and coworkers,5 as it has reactive thiophene α-positions which can be handles for further functionalization and π-extension. In fact, simple theoretical MO calculations6 predict that additional thiophene rings at the thiophene α-positions on the DTDBP substructure, resulting in 5,10-bis(2,2′-bithiophen-5-yl)dibenzo[a,e]pentalene (1a), result in a delocalized HOMO (Fig. 2). On the other hand, its isomeric compound, 2,5,7,10-tetra(2-thienyl)dibenzo[a,e]pentalene (2a) is calculated to have a distinct electron density distribution of HOMO from that of 1a (Fig. 2), indicating that the DTDBP unit could be a unique building block that can tune the electronic structure of the resulting π-conjugated systems by changing the incorporation manner. These theoretical calculations have impelled us to examine the DTDBP unit as an anti-aromatic building block for conjugated polymers. Recently, new conjugated polymers consisting of anti-aromatic cyclopenta[hi]aceanthrylene have been reported as all carbon-based donor (D)–acceptor (A) copolymers.7 Owing to the electron deficiency of the cyclopenta[hi]aceanthrylene units, the polymers have relatively low-lying LUMO energy levels and small bandgaps, but the actual contribution of the anti-aromaticity of cyclopenta[hi]aceanthrylene units to device characteristics is yet unclear.8
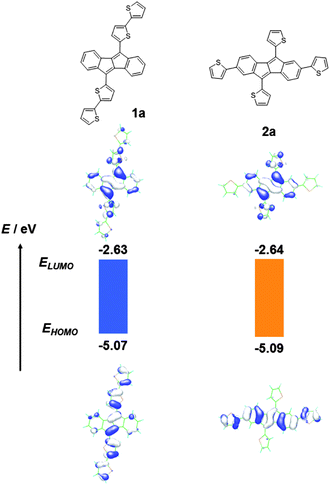 |
| Fig. 2 Electronic structures of 5,10-bis(2,2′-bithiophen-5-yl)dibenzo[a,e]pentalene (1a) and 2,5,7,10-tetra(2-thienyl)- dibenzo[a,e]pentalene (2a) calculated by the TD-DFT methods (B3LYP/6-31G*). | |
We here report the synthesis and characterization of two types of DTDBP-based copolymers with bithiophene units (PDTDBP-2T and iPDTDBP-2T, Scheme 1). These two polymers have isomeric main chain structures, in which the DBP units are incorporated in different manners, potentially enabling to manifest the different perturbation from the anti-aromatic DBP unit. We also fabricated their solution-processed OFET devices to reveal the potential of the anti-aromatic-based semiconducting polymers and found that these polymers showed a relatively high mobility of up to 0.2 cm2 V−1 s−1.
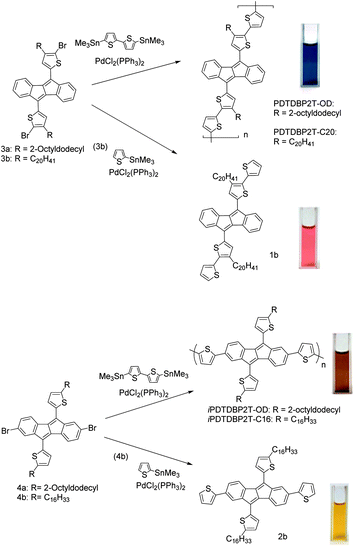 |
| Scheme 1 Synthesis of PDTDBP2Ts and the model compounds, 1b and 2b. | |
Results and discussion
Synthesis and characterizations
The synthesis of PDTDBP2Ts, consisting of the DTDBP unit connected at the α-positions of the 5,10-thienyl groups, is shown in Scheme 1. The monomer units (3) were prepared by the reaction of 4b,5,9b,10-tetrahydrodibenzo[a,e]pentalene-5,10-dione9 with 4-alkyl-2-thienyl lithium followed by the dehydration reaction and subsequent bromination at the α-positions of the thienyl groups (Scheme S1†). Then, 3 was copolymerized with 5,5′-bis(trimethylstannyl)-2,2′-bithiophene by the microwave-assisted Stille coupling reaction to afford PDTDBP2T-OD and PDTDBP2T-C20. The isomeric polymers, iPDTDBP2T-OD and -C16, in which the whole DBP 16π-system is incorporated in the polymer backbone, were also synthesized similarly by the microwave-assisted Stille polymerization of 2,7-dibromo-5,10-dithienyldibenzo[a,e]pentalene (4), which was prepared from newly developed 2,7-dibromo-4b,5,9b,10-tetrahydrodibenzo[a,e]-pentalene-5,10-dione (Scheme S2†), with bis(trimethylstannyl)-bithiophene. It is interesting to note that the each type of polymer shows distinct colour both in the solid state and in solution: PDTDBP2Ts are dark blue, whereas iPDTDBP2Ts are dark red in colour. In addition to the polymers, their corresponding repeating units, 5,10-bis(2,2′-bithiophen-5-yl)dibenzo[a,e]pentalene (1b) and 2,5,7,10-tetra(2-thienyl)dibenzo[a,e]pentalene (2b), were also synthesized from 3b and 4b with 2-(trimethylstannyl)thiophene, respectively, as references (Scheme 1). These DBP-based compounds, 1b, 2b, PDTDBP2Ts, and iPDTDBP2Ts, were found to be stable both in the solution and solid state (see Fig. S4–8†).
Evaluation of electronic structures
We first confirmed the electronic properties of 1b and 2b, the model compounds of the respective polymers, by means of cyclic voltammogram (CV) and UV-vis absorption spectra in solution (Fig. 3). From the onset of the oxidation and reduction waves in the CVs (Fig. 3a), the HOMO and LUMO energy levels (EHOMO and ELUMO) of both 1b and 2b are estimated to be −5.3 eV and −3.3 eV, respectively.10 Almost the same EHOMOs and ELUMOs for both compounds are qualitatively consistent with the results on the theoretical MO calculations (Fig. 2), though there are slight differences between the experimental and theoretical values. The optical HOMO–LUMO gap (Eg) estimated from the onset of absorption spectra is ca. 2.0 eV for both compounds (Fig. 3b), which qualitatively agrees with the electrochemical Egs. The theoretical calculations of 1 and 2 predicted that their overall electronic structures, judging from the spatial distribution of their HOMOs and LUMOs, should be different from each other. In fact, their solutions have characteristic colours, red for 1b and yellow for 2b, respectively (Scheme 1). To understand further, we carried out TD-DFT calculations for 1a and 2a (Fig. S3 and Table S1†). The calculated spectra of 1a show an intensive absorption band cantered at ca. 560 nm, which is assigned to be the HOMO–LUMO transition. On the other hand, the HOMO–LUMO transition of 2a is predicted to have no oscillator strength, and thus the peak most red-shifted should appear at ca. 500 nm, according to the calculations. The experimental spectrum of 2b, in contrast, has a long tail up to 610 nm, which can be thus assigned to the forbidden HOMO–LUMO transition.
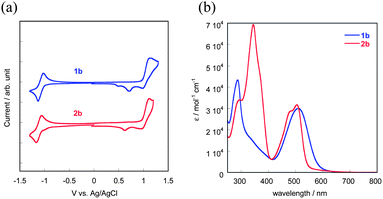 |
| Fig. 3 Cyclic voltammograms (a) and UV-vis absorption spectra of 1b and 2b (b) in dichloromethane solution. | |
We also calculated nucleus-independent chemical shifts (NICSs)11 for the pentalene and benzene rings in the DBP moieties in 1a and 2a (Fig. 4). The NICS values in the unsubstantiated DTDBP are 9.20 ppm for the pentalene and −4.32 ppm for the benzene moiety, respectively, which clearly indicates the anti-aromatic and aromatic nature of the respective rings. When the additional thiophene rings are attached to the thiophene α-positions on the DTDBP unit (i.e., 1a), both the NICSs for the pentalene (8.09 ppm) and the benzene (−4.43 ppm) become smaller than the reference DTDBP, which indicates that the anti-aromaticity of the pentalene moiety is reduced, whereas the aromaticity of the benzene rings is enhanced. In other words, the contribution of one of the resonance structures of DBP expressed as a localized 1,3-butadiene moiety with two fused benzene rings is enhanced; the 16π nature of the DBP unit is said to be split into the 1,3-butadiene moiety (4π) on the C5–C4–C9–C10 carbon strand and two benzene rings (6π × 2). On the other hand, the NICS values calculated for 2a, 2,5,7,10-tetra(2-thienyl)dibenzo[a,e]pentalene, show different tendencies; although the NICS for the pentalene is reduced (8.53 ppm), those for the benzene rings are greatly enlarged (−3.76 ppm), which indicates that the anti-aromaticity of the delocalized 16π nature of the DBP unit is more enforced in 2a than that of DTDBP.
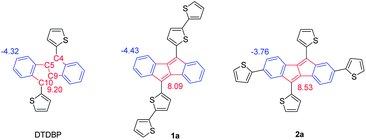 |
| Fig. 4 Calculated NICS values (ppm) for DTDBP, 1a, and 2a. | |
Table 1 Properties of TD-DBP based polymers
|
M
n
/kDa |
M
w
/kDa |
PDIa |
E
HOMO
/eV |
E
LUMO
/eV |
E
g
/eV |
λ
max (λonset)/nm |
µ/cm2 V−1 s−1 |
I
on/Ioff |
V
th/V |
Solution |
Film |
Determined by GPC (o-dichlorobenzene, 140 °C).
HOMO (EHOMO) and LUMO energy levels (ELUMO) were estimated from the onset of oxidation and reduction peaks, respectively, in cyclic voltammograms.
Estimated from the absorption onset in solution.
Owing to poor solubility of polymers, the values can not be determined.
|
PDTDBP2T-C20 |
32.0 |
67.0 |
2.1 |
—d |
—d |
—d |
—d |
666, 416 (810) |
0.15 |
2.0 × 104 |
−1.4 |
PDTDBP2T-OD |
38.0 |
229.0 |
6.0 |
−5.1 |
−3.4 |
1.7 |
548, 387 (680) |
636, 408 (810) |
0.024 |
1.0 × 103 |
+13 |
iPDTDBP2T-C16 |
21.0 |
43.0 |
2.1 |
—d |
—d |
—d |
—d |
538, 464 (780) |
0.19 |
2.5 × 104 |
−13 |
iPDTDBP2T-OD |
18.0 |
41.0 |
2.3 |
−5.0 |
−3.3 |
1.7 |
510, 440 (700) |
538, 464 (780) |
0.15 |
1.2 × 104 |
−1.8 |
Solution properties of polymers
Depicted in Fig. 5 are CVs and UV-vis absorption spectra of two polymers with 2-octyldodecyl substituents, PDTDBP2T-OD and iPDTDBP2T-OD. As in the case for their repeating units, both polymers showed clear oxidation and reduction waves in their voltammograms. From the onset of the oxidation and reduction, EHOMO and ELUMO of PDTDBP2T-OD are determined to be −5.1 eV and −3.4 eV, respectively, which are almost the same with those of iPDTDBP2T-OD, EHOMO: −5.0 eV and ELUMO: −3.3 eV (Table 1). Compared to those of the repeating units (1b and 2b), it is obvious that, although the EHOMOs of polymers shifted upward by 0.2–0.3 eV, there is no noticeable difference in ELUMOs. This can be qualitatively understood by the spatial distribution of HOMO and LUMO on the repeating units (Fig. 2). Regardless of the direction of the DBP units in the conjugated system, the LUMOs tend to localize on the DBP units, whereas the HOMOs delocalize over the π-extended direction, which strongly implies that the HOMOs of both polymers effectively delocalize along the polymer backbones. In contrast, the strongly localized LUMOs on the DBP units hardly have the delocalization effects in the polymers, resulting in almost unchanged ELUMOs in the polymers (Fig. 5c).
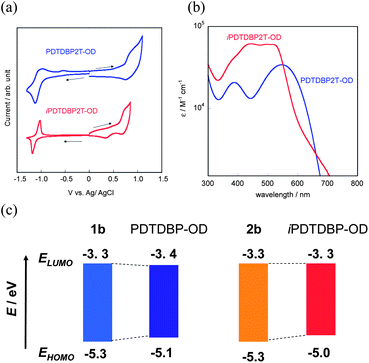 |
| Fig. 5 Cyclic voltammograms in chlorobenzene/benzonitrile (4/1 v/v) solution (a) and UV-vis absorption spectra in chlorobenzene (b) of PDTDBP2T-OD and iPDTDBP2T-OD, and schematic energy diagrams of 1b, 2b, PDTDBP2T-OD, and iPDTDBP2T-OD (c). | |
While the electrochemical behaviours of these two polymers are quite similar, their overall absorption spectra are different (Fig. 5b), though the optical bandgaps estimated from the absorption onsets are both ca. 1.7 eV. The red-sifted absorption band cantered at ca. 550 nm for PDTDBP2Ts compared to the repeating unit (1b, 520 nm) is characteristic, whereas the intensive, broad bands cantered at ca. 500 nm for iPDTDBP2Ts are noticeable, reflecting on their distinct colour in solution (Scheme 1). This could be understood by effects originating from the polymerization; difference in the electronic structure of the repeating units (1b and 2b) is pronounced by polymerization.
Characterization of the electronic structure of polymer thin films and applications to OFETs
Thin films of polymers were deposited by spin-coating from chlorobenzene solution on ITO or quartz glass substrate. Ionization potentials (IPs) of the polymer thin films evaluated by photoelectron spectroscopy in air were 5.1 eV for PDTDBP2Ts and 4.9 eV for iPDTDBP2Ts (Fig. 6a). Compared to EHOMOs estimated from CV, the IPs of the latter polymers are slightly lowered. On the other hand, a pronounced red shift is observed in the thin film absorption spectra of the former polymers compared to the solution absorption spectra (Fig. 5b and 6b). These results imply the existence of intermolecular interaction in the thin film state, but the mode of interaction could not be the same in the two polymer system.
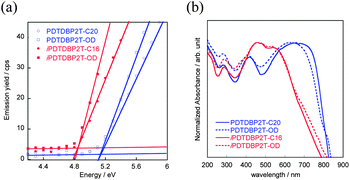 |
| Fig. 6 Photoelectron spectra in air (a) and UV-vis absorption spectra (b) of the thin films of polymers. | |
Transistor characteristics of the polymers were evaluated using top-contact, bottom gate devices with the polymer thin films spin-coated from chlorobenzene solutions on 1H,1H,2H,2H-perfluorodecyltriethoxysilane (FDTS)-modified Si/SiO2 substrates.12Fig. 7 depicts typical transfer and output curves of the PDTDBP2T-C20, PDTDBP2T-OD, iPDTDBP2T-C16, and iPDTDBP2T-OD-based devices, respectively. All devices showed p-channel transistor responses under ambient conditions. Hole mobilities of PDTDBP2T-C20 and PDTDBP2T-OD extracted from the saturation regime are as high as 0.15 and 0.024 cm2 V−1 s−1, respectively. Similar mobilities, 0.19 and 0.15 cm2 V−1 s−1, respectively, were obtained for the iPDTDBP2T-C16- and iPDTDBP2T-OD-based OFETs (Table 1).
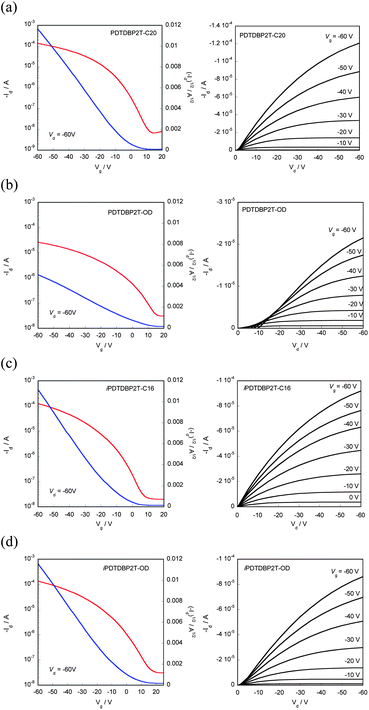 |
| Fig. 7 Transfer and output characteristics of PDTDBP polymers: (a) PDTDBP2T-C20, (b) PDTDBP2T-OD, (c) iPDTDBP2T-C16, and (d) iPDTDBP2T-OD. | |
These results suggest that DBP is a promising core for semiconducting polymers regardless of the incorporation direction. It is interesting to note that in the former polymer system, the mobility tends to be affected by the shape of the alkyl groups attached, linear or branched, whereas the mobility of the latter system is independent of the shape of the alkyl groups (vide infra).
Ordering structures of polymer in the thin film state
Two-dimensional grazing incidence X-ray diffraction (2D-GIXD) measurements clearly showed different ordering nature of each polymer in the thin film on the Si/SiO2 substrate (Fig. 8). Nicely crystalline peaks assignable to the edge-on orientation (dlamellar = 23.3 Å) on the qz axis up to the fifth order and the π-stacking peak on the qxy axis (dπ = 3.6 Å) are observed for the PDTDBP2T-C20 thin film. On the other hand, the crystalline order of PDTDBP2T-OD with the same backbone but the branched alkyl groups is very poor; the lamellar peaks are observed as the large arc, indicating that the polymers are significantly misorientated, and the film is rather amorphous-like. These distinct crystallinities of the PDTDBP2T-based polymers depending on the attached alkyl groups can well explain their different OFET characteristics; highly crystalline PDTDBP2T-C20 gives better mobility (0.15 cm2 V−1 s−1) by ca. one order of magnitude than the amorphous-like PDTDBP2T-OD does (0.024 cm2 V−1 s−1).
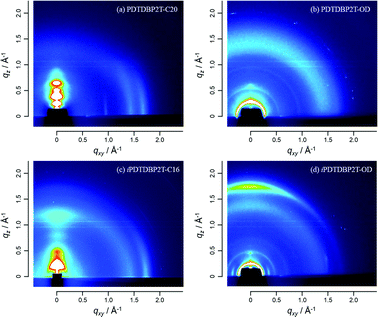 |
| Fig. 8 2D-GIXD images of the polymer thin films: PDTDBP2T-C20 (a), PDTDBP2T-OD (b), iPDTDBP2T-C16 (c), iPDTDBP2T-OD (d). | |
Similar but much pronounced influence of the alkyl groups on the polymer crystallinity and orientation is observed for the isomeric polymers, iPDTDBP2T. The iPDTDBP2T-C16 thin film shows a typical pattern for the edge-on orientation with the lamellar diffractions (dlamellar = 24.6 Å) along the qz axis and π-stacking (dπ = 3.6 Å) along the qxy axis, which well explains the high mobility in its thin film transistors (0.19 cm2 V−1 s−1). On the other hand, the polymer with the branched alkyl groups, iPDTDBP2T-OD, gives a quite different 2D-GIXD pattern; not just all the diffractions appeared as arcs, but a clear and intensive diffraction assignable to the π-stacking appears on the qz axis (qz = ca. 1.7 Å−1, dπ = 3.6 Å), which indicates that the polymer has a preferential face-on orientation. Despite the face-on orientation, which is not suitable for the transverse carrier transport in OFETs, the mobility of iPDTDBP2T-OD-based OFETs is almost the same (0.15 cm2 V−1 s−1) as those of the iPDTDBP2T-C16 thin film with crystalline, edge-on orientation.
In general, the semiconducting polymers having better crystallinity and the edge-on order tend to show high mobilities in the “typical” polymer OFETs.13 The high mobilities of the PDTDBP2T-C20- and iPDTDBP2T-C16-based OFETs and decreased mobility of the PDTDBP2T-OD-based ones are understood within this general picture. In this context, almost identical performances of the iPDTDBP2T-OD-based OFETs with the formers, despite its preferential face-on orientation, are rather unusual.
In contrast, several D–A polymers recently developed have shown high mobility, even though they take the preferential face-on orientation in their thin films. In such “unusual” D–A polymers, it is said that strong local π–π inter-chain interactions14 and/or intra-chain D–A interaction may help smooth charge carrier transport. Although it is not confirmed yet, a similar carrier transport mechanism is plausible for the present iPDTDBP2T-OD-based OFETs, since the DBP unit has moderate electron deficient nature owing to its 16π electron system, which makes it a kind of D–A polymer.3,8
It is also interesting to point out that such orientation-independent high mobility is only observed for the iPDTDBP2T polymer system, not for the PDTDBP2T system. These different contributions from the DBP units in the two polymer systems can be understood again by the distinct local electronic structure of DBP units in the polymer main chains. The DBP unit in the PDTDBP2T polymers behaves as a “dibenzo-annulated 1,3-butadiene” moiety, making the resulting polymers “typical” ones consisting of donor units only, similar to P3HT and other polythiophene derivatives. On the other hand, in the iPDTDBP2T polymers with the whole 16π DBP units integrated in the polymer main chain, the electron deficiency of the DBP units can be manifested, and as a result, the polymers behave as D–A polymers (Fig. 9). From all these evaluations, the DBP unit can be recognized as a transmutable building block that can afford different electronic systems by choosing the incorporation manners.
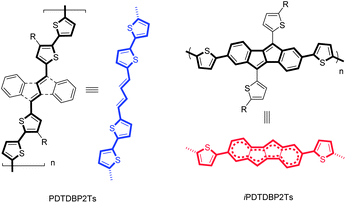 |
| Fig. 9 Schematic representation of the conjugated structures of two polymers. | |
Conclusion
Focusing on the 16π anti-aromatic DBP unit as a potential building block for semiconducting polymers, we synthesized two types of DBP-based conjugated polymers with different incorporation manners. Comparison of physicochemical properties and theoretical calculations for the corresponding repeating units and polymers themselves revealed that the electronic structure can be altered by the incorporation manner of the DBP unit in the polymer backbones, but their EHOMOs and ELUMOs are not significantly affected. The most pronounced effects from the incorporation manner are observed on the OFET characteristics. For PDTDBP2Ts, in which the DBP units are integrated into the polymer backbone via the 5,10-positions, the DBP unit could behave as a “dibenzo-annulated 1,3-butadiene” moiety, and their OFET characteristics are strongly affected by orientational ordering and crystallinity, similar to ordinary donor-only polymers such as P3HT. On the other hand, iPDTDBP2Ts, in which the whole 16π DBP units are integrated into the polymer backbone, behave like a certain kind of D–A polymers in their OFETs, and the OFET performances are independent of orientational order.
From these results, we conclude that the DBP unit can be a useful building block for the development of new conjugated polymers. In particular, DBP can behave as the acceptor units in D–A polymers, when the whole 16π DBP system is integrated into the polymer backbone. On the other hand, in the case of the DBP units connected via the 5,10-positions in the polymer backbone, the anti-aromatic nature of DBP is less pronounced. As a result, the DBP unit with the transmutable nature can be regarded as a versatile building block for semiconducting polymers both for D-only polymers as well as D–A polymers.
Acknowledgements
This work was financially supported by Grants-in-Aid for Scientific Research (no. 23245041 and 24685030) from MEXT, Japan and The Strategic Promotion of Innovative Research and Development from the Japan Science and Technology Agency. HRMSs were carried out at the Natural Science Centre for Basic Research and Development (N-BARD), Hiroshima University. Combustion elemental analyses were carried out at the Materials Characterization Support Unit in the RIKEN Advanced Technology Support Division. 2D-GIXD experiments were performed at the BL19B2 of SPring-8 with the approval of the Japan Synchrotron Radiation Research Institute (JASRI) (Proposal no. 2012A1611). One of the authors (M.N.) is grateful for the Postdoctoral Fellowship of Japan Society for the Promotion of Science (JSPS).
Notes and references
-
(a)
Functional Organic Materials, T. J. J. Muller and U. H. F. Bunz, Wiley-VCH, Weinheim, 2007 Search PubMed;
(b)
Organic light emitting Devices: Synthesis Properties and Applications, ed. K. Mullen and U. Scherf, Wiley-VCH, Weinheim, 2006 Search PubMed;
(c)
Carbon-Rich Compounds, ed. M. M. Haley and R. R. Tykwinski, Wiley-VCH, Weinheim, 2006 Search PubMed.
-
(a) D. J. Gundlach, Y. Y. Lin, T. N. Jackson, S. F. Nelson and D. G. Schlom, IEEE Electron Device Lett., 1997, 18, 87–89 CrossRef CAS;
(b) Y. Y. Lin, D. J. Gundlach, S. F. Nelson and T. N. Jackson, IEEE Electron Device Lett., 1997, 18, 606–608 CrossRef CAS;
(c) H. Klauk, M. Halik, U. Zschieschang, G. Schmid, W. Radlik and W. Weber, J. Appl. Phys., 2002, 92, 5259–5263 CrossRef CAS;
(d) T. W. Kelley, L. D. Boardman, T. D. Dunbar, D. V. Muyres, M. J. Pellerite and T. P. Smith, J. Phys. Chem. B, 2003, 107, 5877–5881 CrossRef CAS.
-
(a) M. Saito, Symmetry, 2010, 2, 950–969 CrossRef CAS;
(b) D. T. Chase, A. G. Fix, B. D. Rose, C. D. Weber, S. Nobusue, C. E. Stockwell, L. N. Zakharov, M. C. Lonergan and M. M. Haley, Angew. Chem., Int. Ed., 2012, 50, 11103–11106 CrossRef PubMed;
(c) D. T. Chase, B. D. Rose, S. P. McClintock, L. N. Zakharov and M. M. Haley, Angew. Chem., Int. Ed., 2011, 50, 1127–1130 CrossRef CAS PubMed;
(d) X. Yin, Y. Li, Y. Zhu, Y. Kan, Y. Li and D. Zhu, Org. Lett., 2011, 13, 1520–1523 CrossRef CAS PubMed;
(e) A. R. Mohebbi and F. Wudl, Chem.–Eur. J., 2011, 17, 2642–2646 CrossRef CAS PubMed;
(f) A. R. Mohebbi, J. Yuen, J. Fan, C. Munoz, M. F. Wang, R. S. Shirazi, J. Seifter and F. Wudl, Adv. Mater., 2011, 23, 4644–4648 CrossRef CAS PubMed.
-
(a) Z. U. Levi and T. D. Tilley, J. Am. Chem. Soc., 2010, 132, 11012–11014 CrossRef CAS PubMed;
(b) Z. U. Levi and T. D. Tilley, J. Am. Chem. Soc., 2009, 131, 2796–2797 CrossRef CAS PubMed;
(c) T. Kawase, T. Fujiwara, C. Kitamura, A. Konishi, Y. Hirao, K. Matsumoto, H. Kurata, T. Kubo, S. Shinamura, H. Mori, E. Miyazaki and K. Takimiya, Angew. Chem., Int. Ed., 2010, 49, 7728–7732 CrossRef CAS PubMed;
(d) H. Zhang, T. Karasawa, H. Yamada, A. Wakamiya and S. Yamaguchi, Org. Lett., 2009, 11, 3076–3079 CrossRef CAS PubMed;
(e) T. Kawase, A. Konishi, Y. Hirao, K. Matsumoto, H. Kurata and T. Kubo, Chem.–Eur. J., 2009, 15, 2653–2661 CrossRef CAS PubMed;
(f) F. Xu, L. Peng, A. Orita and J. Otera, Org. Lett., 2012, 14, 3970–3973 CrossRef CAS PubMed;
(g) J. Zhao, K. Oniwa, N. Asao, Y. Yamamoto and T. Jin, J. Am. Chem. Soc., 2013, 135, 10222–10225 CrossRef CAS PubMed.
- J. Yang, M. V. Lakshmikantham, M. P. Cava, D. Lorcy and J. R. Bethelot, J. Org. Chem., 2000, 65, 6739–6742 CrossRef CAS.
- MO calculations were carried out with the DFT/TD-DFT method at the B3LYP/6-31G(d) level using the Gaussian 03 program package. See details in the ESI.†.
- J. L. Jellison, C.-H. Lee, X. Zhu, J. D. Wood and K. N. Plunkett, Angew. Chem., Int. Ed., 2012, 51, 12321–12324 CrossRef CAS PubMed.
- During preparation of this manuscript, thiophene-based antiaromatic small molecules were examined as the active material for ambipolar organic field-effect transistors: see, T. Nishinaga, T. Ohmae, K. Aita, M. Takase, M. Iyoda, T. Arai and Y. Kunugi, Chem. Commun., 2013, 49, 5354–5356 RSC.
- R. S. D. Mittal, S. C. Sethi and S. Dev, Tetrahedron, 1973, 29, 1321–1325 CrossRef CAS.
- C. M. Cardona, W. Li, A. E. Kaifer, D. Stockdale and G. C. Bazan, Adv. Mater., 2011, 23, 2367–2371 CrossRef CAS PubMed.
- P. v. R. Schleyer, C. Maerker, A. Dransfeld, H. Jiao and N. J. R. v. E. Hommes, J. Am. Chem. Soc., 1996, 118, 6317–6318 CrossRef CAS.
-
(a) S. Kobayashi, T. Nishikawa, T. Takenobu, S. Mori, T. Shimoda, T. Mitani, H. Shimotani, N. Yoshimoto, S. Ogawa and Y. Iwasa, Nat. Mater., 2004, 3, 317–322 CrossRef CAS PubMed;
(b) K. Nakayama, M. Uno, T. Nishikawa, Y. Nakazawa and J. Takeya, Org. Electron., 2010, 11, 1620–1623 CrossRef CAS PubMed.
- H. Sirringhaus, P. J. Brown, R. H. Friend, M. M. Nielsen, K. Bechgaard, B. M. W. Langeveld-Voss, A. J. H. Spiering, R. A. J. Janssen, E. W. Meijer, P. Herwig and D. M. de Leeuw, Nature, 1999, 401, 685–688 CrossRef CAS PubMed.
-
(a) J. Rivnay, M. F. Toney, Y. Zheng, I. V. Kauvar, Z. Chen, V. Wagner, A. Facchetti and A. Salleo, Adv. Mater., 2010, 22, 4359–4363 CrossRef CAS PubMed;
(b) J. Rivnay, R. Steyrleuthner, L. H. Jimison, A. Casadei, Z. Chen, M. F. Toney, A. Facchetti, D. Neher and A. Salleo, Macromolecules, 2011, 44, 5246–5255 CrossRef CAS;
(c) I. Osaka, M. Akita, T. Koganezawa and K. Takimiya, Chem. Mater., 2012, 24, 1235–1243 CrossRef CAS;
(d) X. Zhang, L. J. Richter, D. M. DeLongchamp, R. J. Kline, M. R. Hammond, I. McCulloch, M. Heeney, R. S. Ashraf, J. N. Smith, T. D. Anthopoulos, B. Schroeder, Y. H. Geerts, D. A. Fischer and M. F. Toney, J. Am. Chem. Soc., 2011, 133, 15073–15084 CrossRef CAS PubMed;
(e) J. Mei, D. H. Kim, A. L. Ayzner, M. F. Toney and Z. Bao, J. Am. Chem. Soc., 2011, 133, 20130–20133 CrossRef CAS PubMed.
Footnote |
† Electronic supplementary information (ESI) available. See DOI: 10.1039/c3tc31630k |
|
This journal is © The Royal Society of Chemistry 2014 |
Click here to see how this site uses Cookies. View our privacy policy here.