DOI:
10.1039/C4RA10451J
(Paper)
RSC Adv., 2014,
4, 64371-64378
Design, synthesis and photoswitching of broad-spectrum fluorescent hexaarylbiimidazoles†
Received
15th September 2014
, Accepted 19th November 2014
First published on 19th November 2014
Abstract
A series of novel hexaarylbiimidazole (HABI)-containing fluorophores with broad-spectrum emissions ranging from blue, green and red emissions, have been designed and synthesized. Their photochromism and fluorescence switching properties are investigated by UV-Vis absorption and fluorescence spectra in solutions and PMMA films. By connecting carbazole (Cz), naphthalimide (NI) and perylene-mono-imide (PMI) to HABI with a spacer, three molecular photoswitches with broad-spectrum fluorescence varying from deep blue (Cz–O–HABI, λem = 390 nm, ΦF = 0.27), green (NI–O–HABI, λem = 490 nm, ΦF = 0.25) to far red (PMI–O–HABI, λem = 650 nm, ΦF = 0.25) have been obtained. The “ON”/“OFF” ratios are calculated to be about 8.9, 8.0 and 3.7 (calculated from FL spectra) for Cz–O–HABI, NI–O–HABI and PMI–O–HABI, respectively. Such a series of photoswitchable fluorophores constitute prototypes of a photochromic molecular library in which fluorescence resonance energy transfer can be reversibly modulated by an external stimulus, namely, UV light.
Introduction
Photochromic materials are a well-known class of molecules that change their color upon UV light irradiation. Of them, azobenzene,1 spiropyran,2 and diarythene3 are the three mostly investigated photochromic materials. Hexaarylbiimidazole (HABI) was first discovered in the 1960s by Hayashi and Maede through oxidizing 2,4,5-triphenylimidazole in a potassium hydroxide aqueous solution.4 2,4,5-triphenylimidazole has the common name lophine, hexaarylbiimidazoles (HABIs) and 2,4,5-triarylimidazolyl radicals are sometimes called lophine dimers and lophyl radicals, respectively. Lophine is also known as the first chemiluminescent materials discovered by Radziszewski in 1877.5 Usually, HABIs are colorless in solution and after UV light irradiation the dimer homolytical cleavage to form a pair of colored 2,4,5-triarylimidazolyl radicals. Interestingly, HABI is also sensitive to pressure and thermal stimuli, which makes it different from usual photochromic materials.4,6
The fluorescence technique has been widely applied to numerous fields like optical materials, analytical chemistry, protein conformation studies to biological assays by taking advantage of its exquisite sensitivity, cost-effectiveness, facile operation, and super-spatial and temporal resolutions.7 And the photo-switching emission phenomenon, which could control fluorescence upon irradiation of appropriate wavelength light, has attracted much attention since it emerged at the end of the 20th and the beginning of the 21st century. Proper photo-switches could be realized by integrating a selected fluorophore to a photochromic component within a common covalent skeleton.8–10 Accordingly, numerous examples of fluorophore–photochrome dyads have already been demonstrated,11–15 and their operating principles for fluorescence modulation have recently been extended to nano-structured constructs2,16–19 and extensively reviewed.15 Under these conditions, the reversible interconversion of photochrome between the colorless and colored states results in the modulation of the fluorescence intensity by electron or energy transfer. Photo-switches were originally considered for optical data storage,20 and they have also found diverse applications in super-resolution fluorescence microscopy.21,22
The application in super-resolution imaging generally relies on the reversible transformation between the fluorescent “ON” and “OFF” states upon photo excitation of the photoswitches. We have demonstrated that both polymer nanoparticles (SP–PCL) and small molecule (DTE–TPE) could be applied to super-resolution fluorescence imaging.23 And as a groundbreaking attempt of HABI in super-resolution imaging, we have also designed and synthesized tetraphenylethene–HABI conjugate (TPE–HABI).24 It has been found that TPE–HABI with photoswitchable aggregation-induced emission (AIE) properties is suitable for super-resolution imaging in condensed state. Thus, designing fluorescent HABIs with high “ON/OFF” ratio and fast photobleaching rates might open the door for HABI in super resolution imaging application. Abe and coworkers have recently reported the investigation of a fluorescent HABI-based photoswitch using a conjugated [2.2]-paracyclophane HABI-fluorescein molecule.25 And we also reported a fluorescent HABI-based photochrome, in which a highly fluorescent naphthalimide (NI) derivative is connected to HABI in a non-conjugated fashion.26 However, both of the two systems are limited to green emissive HABIs. In order to achieve a broad-spectrum emission with blue, green and red wavelength, a molecular design strategy toward emissive HABIs with high quantum yield, high “ON/OFF” ratio and good fatigue resistance is demonstrated through isolating the fluorophore and HABI with a spacer.
In this paper, we choose carbazole, naphthalimide and perylene-mono-imide as the blue, green and red fluorophores and connect them to HABI with a spacer, which are Cz–O–HABI, NI–O–HABI and PMI–O–HABI. Their pronounced photochromism properties are investigated by UV-Vis absorption and fluorescence spectrometer. The as-synthesized photoswitchable fluorophores exhibits moderate fluorescence quantum yield of 27%, 25% and 25% in benzene using anthracene or Rhodamine B as standard. Moreover, they show reversible switching of fluorescence to various certain extents upon intermittent UV irradiation.
Results and discussion
The molecular design and photochromism of the fluorescent HABI-based photochromes and their model fluorophores are illustrated in Scheme 1. Our major purpose here is to develop a series of broad-spectrum photoswitchable fluorophores including blue (Cz–O–HABI), green (NI–O–HABI) and red (PMI–O–HABI) emissions. C8H17–O–HABI has been synthesized as a non-fluorescent model molecule. Generally, 1 is colorless and non-fluorescent in solution but turns into blue color after UV irradiation. Carbazole (Cz), naphthalimide (NI) and perylene-mono-imide (PMI) are used as blue (Cz–O–HABI), green (NI–O–HABI) and red (PMI–O–HABI) fluorophores. Upon proper light excitation, these fluorophores emit blue, green and red fluorescence and after UV irradiation, the four HABIs decompose into two radicals named as C8H17–O–TPIR, Cz–O–TPIR, NI–O–TPIR and PMI–O–TPIR, respectively. The radicals could be used as fluorescence quencher for all-spectrum emission because of their activated excited state scavenger role. In this way, the full color: blue, green and red fluorescent HABIs are designed and synthesized.
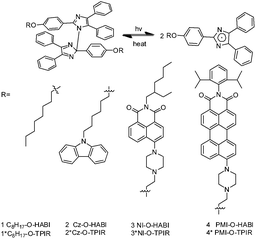 |
| Scheme 1 The photochromism process of HABIs. | |
The synthetic routes of HABIs including C8H17–O–HABI, Cz–O–HABI and PMI–O–HABI are shown in Scheme 2. The synthetic procedures and characterization information like H NMR, MS are presented in ESI† about 3 (NI–O–HABI) could be found in our former work.26
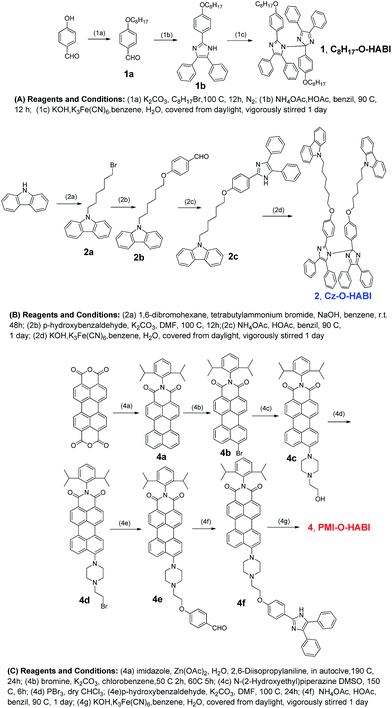 |
| Scheme 2 The synthetic procedures of (A) C8H17–O–HABI, (B)Cz–O–HABI and (C) PMI–O–HABI. | |
Photochromism and fluorescence switching
We first investigate the photochromism properties of C8H17–O–HABI in benzene as the model compound of Cz–O–HABI, NI–O–HABI and PMI–O–HABI, which is slightly different from HABI because of the electron-donating nature of alkoxy group. As we can see from Fig. 1, C8H17–O–HABI shows a peak around 290–300 nm (this could be seen either in another solvent instead of benzene). After 5 seconds of UV (302 nm, 0.85 mW cm−2, we use the same UV source in this work except for special statements) irradiation, two peaks at 390 nm (absorbance, A = 2.69) and 610 nm (A = 1.02) are observed with the solution color changing from colorless to blue. The optical density increases to 3.12 (390 nm), 1.21 (610 nm) and 3.22 (390 nm), 1.26 (610 nm) for 10 s and 20 s with an isosbetic point at 326 nm. At 40 s and 60 s the optical intensity slightly decreases which are 3.20 (390 nm), 1.26 (610 nm) and 3.17 (390 nm), 1.23 (610 nm). The optical intensity decreases probably because the radicals recombine to form C8H17–O–HABI after it has been irradiated over saturated state. These data are consistent with the book by Rolf Dessauer.27
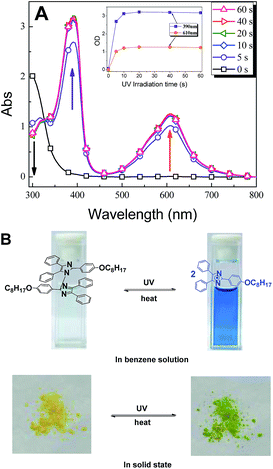 |
| Fig. 1 (A) UV-Vis spectra of C8H17–O–HABI in benzene (5.0 × 10−5 mol L−1) with different UV light (302 nm) irradiation time from 0, 5, 10, 20,40 to 60 s; (B) photograph of C8H17–O–HABI in benzene and solid state before and after UV (302 nm) irradiation. | |
C8H17–O–HABI is non-fluorescent both in dimer and radicals. Therefore we decorated HABI with emissive fluorophores allowing for the measurements and tracing by UV-vis absorption and fluorescence spectroscopy. The photochromic properties of Cz–O–HABI, NI–O–HABI and PMI–O–HABI have been investigated in benzene solutions.
The oxygen at the para-position of 2-phenyl ring of the imidazole blocks the π-conjugation between HABI and the corresponding fluorophores, thus resulting in the distinct UV-Vis spectra of conjugated HABIs.24,26 Before UV irradiation, the solution of Cz–O–HABI (5 × 10−5 mol L−1) is colorless with three peaks at 316 nm, 328 nm and 344 nm, which could be attributed to the characteristic peak of carbazole. After 5 seconds of UV irradiation, a sharp peak at 390 nm (A = 1.62) and a broad peak between 500–700 nm with λAbs,max = 610 nm (A = 0.59) are observed and the solution changes from colorless to blue. Meanwhile, the absorbance of the three peaks at 316 nm, 328 nm and 344 nm increases with more defined structures. These characters indicate the formation of Cz–TPIRs after UV irradiation from Cz–O–HABI dimer. The absorbance at 390 nm and 610 nm increases from 1.62 and 0.59 to 2.22 and 0.81, respectively, after 10 s of UV irradiation. These absorption peaks steadily increase by further extending the irradiation time to 20 s. However, the spectrum does not change after 30 s UV irradiation, which might be because the process from Cz–O–HABI to Cz–O–TPIR becomes saturated (see Fig. 2A).
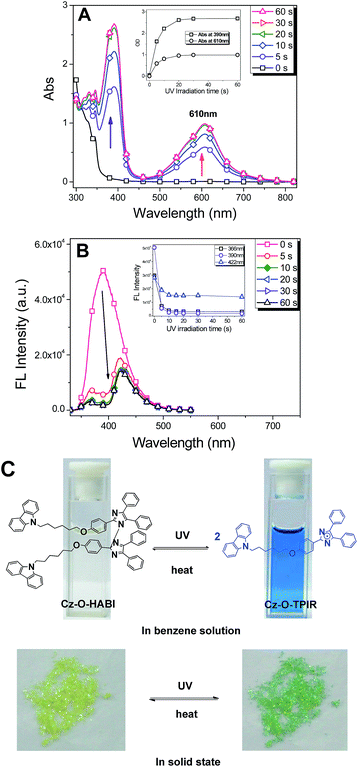 |
| Fig. 2 (A) UV-Vis spectra and (B) FL spectra of Cz–O–HABI (5 × 10−5 mol L−1) in benzene with different irradiation time from 0, 5, 10, 15, 20, 30 to 60 s, excitation wavelength λex = 320 nm, excitation bandwidth and emission bandwidth 1 and 1; (C) photograph of Cz–O–HABI in benzene and solid before and after UV light (302 nm, 0.85 mW cm−2) irradiation. | |
The corresponding changes in FL spectra of Cz–O–HABI upon UV irradiation have also been investigated. Before FL measurement, the excitation spectrum of Cz–O–HABI is performed by detecting the emission intensity at 390 nm (see Fig. S1†). The following experiments are performed using 320 nm as excitation wavelength which is determined as the best excitation wavelength from the excitation spectrum. It is noteworthy that Cz–O–HABI shows slight photochromism upon 320 nm excitation, thus resulting in slice fluorescence quenching. However, the resulting emission loss is negligible compared with the fluorescence quenching from alien UV irradiation (see Fig. S2(A)†).
The Cz–O–HABI solution emits strong deep blue fluorescence, with a broad peak between 350–500 nm and a maximum emission wavelength at 390 nm. The fluorescence quantum yield ΦF of Cz–O–HABI is calculated to 0.27 using anthracene as standard (see Fig. S3†). After 5 s of UV irradiation, the FL intensity at 390 nm decreases rapidly by almost 10 times. Two new emission peaks at 366 nm and 422 nm are observed with decreased intensity (see Fig. 2B). With 20 s of UV irradiation, the emission intensity slightly decreases and hardly changes after 30 s of UV irradiation, which is consistent with the UV spectra. This indicates the photochromism in dilute solution of Cz–O–HABI becomes saturated after 30 s of 302 nm irradiation.
Interestingly, the emission spectra of Cz–O–HABI changes from single peak (390 nm) to two peaks (366 nm and 422 nm) with decreased intensity. The emission of Cz–O–HABI at 390 nm is because of the fluorogenic carbazole in Cz–O–HABI. Upon UV irradiation, Cz–O–HABI homolytic dissociates into two Cz–O–TPIRs. As we can see from C8H17–O–HABI, C8H17–O–TPIR possesses a sharp maximum absorption wavelength at 390 nm, which could act as a quencher for the emission of carbazole. When carbazole has been excited, the emission at 390 nm could be transfer to the TPIR part via fluorescence resonance energy transfer mechanism (see Fig. 3). As a result, most of the emission decreases centered at 390 nm affording twin emission peaks at 366 nm and 422 nm (Fig. 2B).
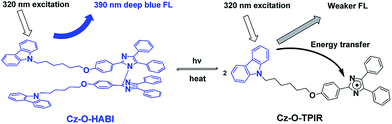 |
| Fig. 3 The proposed photochromism and fluorescent quenching process of Cz–O–HABI. | |
The corresponding optical properties of NI–O–HABI are presented in Fig. 4. The NI–O–HABI exhibits one UV-absorption peak at around 390 nm. Upon UV irradiation, the resulting UV-Vis spectra (Fig. 4A) shows the apparent increase of two bands centered at 390 nm and 600 nm, in which the former band overlaps with the absorption band of the naphthalimide moiety. These two new peaks are due to homolytic cleavage of NI–O–HABI and the formation of radical pairs, NI–O–TPIRs. This transitions accompanied by a color change from light yellow-green to deep blue (Fig. 4C).
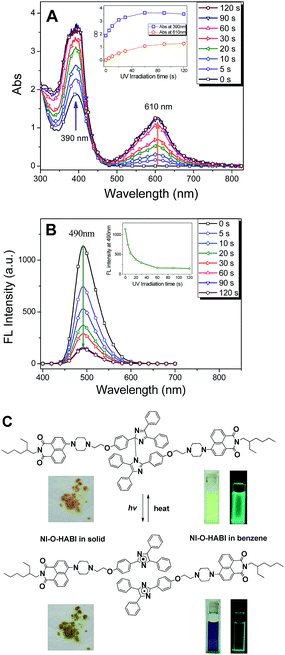 |
| Fig. 4 (A) UV-Vis spectra and (B) FL spectra of NI–O–HABI in benzene (5 × 10−5 mol L−1) with different irradiation time from 0, 5, 10, 20, 30, 60, 90 to 120 s; (C) photograph of NI–O–HABI in benzene and solid before and after UV light (302 nm, 0.85 mW cm−2) irradiation. | |
The NI–O–HABI solution emits strong green fluorescence, with a broad peak between 450–600 nm and a maximum emission wavelength at 490 nm. The fluorescence quantum yield ΦF of NI–O–HABI is calculated to 0.25.26 After 10 s of UV irradiation, the FL intensity at 490 nm decreases to half and the emission intensity remains nearly unchanged at 60 s. Similarly, the emission at 490 nm could be attributed to the fluorogenic naphthalimide moiety in NI–O–HABI. After UV irradiation, NI–O–HABI dissociated into two NI–O–TPIRs accompanied with the color of the solution changing from light yellow-green to deep blue and fluorescence quenching (Fig. 4C).
The situation of PMI–O–TPIR looks different from those of Cz–O–HABI and NI–O–HABI. Before UV irradiation, PMI–O–HABI exhibits characteristic peak of PMI at 525 nm with another two peaks at 353 nm and 376 nm. After UV irradiation, the peak at 525 nm red-shifts to 535 nm and a sharp peak at 380 nm and a broad peak around 600 nm are observed (see Fig. 5A). The intensity of the peaks increases with extending the irradiation time and reach maximum at 2 min. These characters are different from those of C8H17–O–HABI, Cz–O–HABI and NI–O–HABI. For both Cz–O–HABI and NI–O–HABI, we can see the two distinguish peaks at 391 nm and 610 nm, which are from the radicals (the peak 391 nm is overlapped between NI and the radical spectra). To investigate this difference, we make a plot between the optical density changes of PMI–O–HABI and UV irradiation time (see Fig. 5B). In this way, the typical radical spectra with two peaks at 391 nm and 610 nm are observed. Naturally, this could be attributed to absorption spectra overlap between PMI and radicals. The strong and sharp peak at 525 nm covers with the peak at 610 nm and makes it “red-shift” to 535 nm as a result. Similarly, the peaks at 353 nm and 376 nm overlap with the peak at 390 nm and “blue-shift” it to 380 nm.
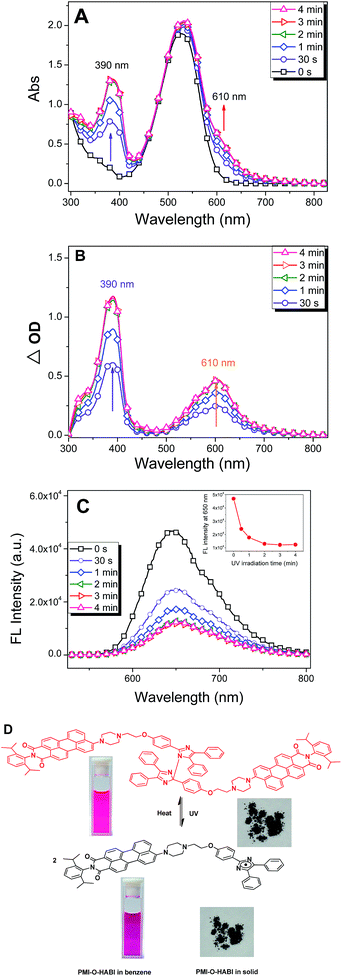 |
| Fig. 5 (A) UV-Vis spectra and (B) ΔOD (optical density) and (C) FL spectra of PMI–O–HABI in benzene (5 × 10−5 mol L−1) with different irradiation time from 0, 0.5, 1, 2, 3 to 4 min; (D) photograph of PMI–O–HABI in solid and benzene before and after UV light (302 nm, 0.85 mW cm−2) irradiation. | |
The FL spectra of PMI–O–HABI by different UV irradiation time are shown in Fig. 5C. PMI–O–HABI emits strong red light (λem = 650 nm) with moderate fluorescent quantum yield (ΦF = 0.25, see Fig. S4†). After 30 s UV irradiation, the fluorescent intensity at 650 nm decreases to 52% of the initial, and continuously decreases to 37% (1 min of UV irradiation), 27% (2 min UV irradiation) and keeps constant at 25% (3 min UV irradiation). As further increasing the irradiation time does not affect the UV-Vis and FL spectra, and we suppose the conversion between PMI–O–HABI and PMI–O–TPIR becomes saturated. As there is only slight overlap between the emission spectra of PMI and red-edge shoulder of TPIR absorption band plus the two groups in close proximity, we believe the incomplete fluorescence quenching results from the fluorescence resonance energy transfer from the PMI to the TPIR.
Photochromism properties of HABIs in PMMA films
The photochromism properties of the synthesized HABIs are investigated in PMMA films since the color changes in solid state for photochromic materials are also very important. The samples are prepared by dissolving 2 mg of the corresponding samples into 1 mL of PMMA solution in chloroform. The PMMA solution is made by dissolving 10 g PMMA (MW = 35
000, Sinopharm Chemical Reagent Co. Ltd.) into 50 mL distilled chloroform. This solution was stirred overnight and then it was used for the spin-coating. During the spin-coating process, the speed is controlled to 1000 rad s−1 for 1 min. In this way, thin films for UV-Vis measurements are prepared.
As we can see from Fig. 6A, a sharp peak at 390 nm and a broad peak around 610 nm appeared after 5 s UV light irradiation. The peak around 280–320 nm decreased with an isosbetic point at 335 nm. During the experiments, the color of the film changed from colorless to blue after exposure to UV light. These two peaks kept increasing as we extending the irradiation time to 60 s and remaining unchanged at 90 s. This could be explained in the same way as that in solutions. These two new peaks are from the Cz–O–TPIRs formed from the photocleavage of Cz–O–HABI. The amount of Cz–O–TPIR increases with the irradiation time until it was saturated at 90 s. The peaks around 300–325 nm in Fig. 6B were generated from the characteristic absorption of carbazole moiety. The peak at 390 nm increases in both solutions (Fig. 4A) and films (Fig. 6C) is contributed to the overlap between TPIR and naphthalimide as we have reported before.26
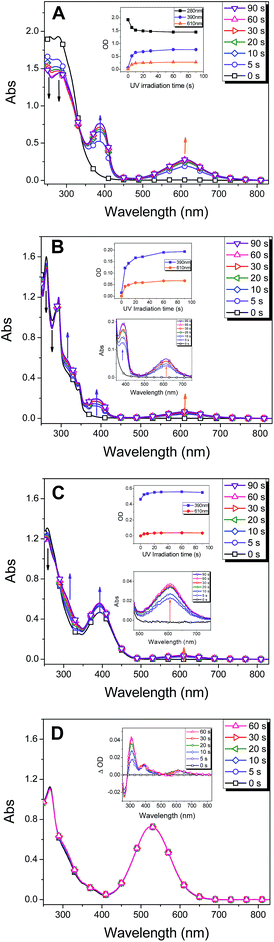 |
| Fig. 6 UV-Vis spectra of (A) C8H17–O–HABI, (B)Cz–O–HABI, (C) NI–O–HABI and (D) PMI–O–HABI in PMMA film under UV light (302 nm, 0.85 mW cm−2) irradiation, t = 0, 5, 10, 20, 30, 60 and 90 s. | |
This phenomenon was further observed in the case of PMI–O–HABI since the broad peak around 610 nm is greatly overlapped with the absorption of perylene-mono-imide. As a result no significant spectra changes were witnessed for PMI–O–HABI from the UV-Vis spectra compared with other synthesized HABIs (Fig. 6D). When we use the “ΔOD” (changes of the optical density) instead of “Abs”, two clear peaks: 390 nm and 610 nm appeared (see inset of Fig. 6D). From the UV-Vis spectra of Cz–O–HABI, NI–O–HABI and PMI–O–HABI as exhibited in Fig. 6B–D, we observed similar spectra changes, which could be attributed to the same reason of photochromism properties after UV irradiation. These data demonstrated that all the designed fluorescent HABIs exhibited great color changes in both solutions and polymer films.
Thermal recombination of HABI, C8H17–O–HABI, Cz–O–HABI, NI–O–HABI and PMI–O–HABI
The back reactions from TPIRs to HABIs are investigated by UV-Vis absorption spectroscopy. The experiments are performed by irradiating the solutions and then detecting the optical density changes at 610 nm. For comparison, the decay profile of HABI is also performed in the same condition (the detecting wavelength is 555 nm for HABI). As we can see from Fig. 7, in the first 200 s, the absorbance of HABI decreases rapidly with a half lifetime τ1/2 = 132 s and then the curve becomes more and more flat, which exhibits a second order kinetic for the recombination of the two TPIRs. The other HABIs behave in a similar manner and the τ1/2 of C8H17–O–HABI, Cz–O–HABI, NI–O–HABI and PMI–O–HABI are calculated to be 848, 1250, 1365 and 1085 s.
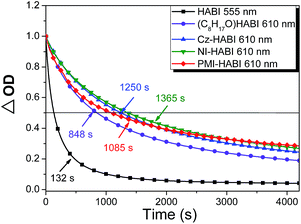 |
| Fig. 7 Decay profiles of the colored species generated from HABI, C8H17–O–HABI, Cz–O–HABI, NI–O–HABI and PMI–O–HABI, monitored at 555 nm for HABI and 610 nm for the others in degassed benzene (5 × 10−5 mol L−1). The measurements were performed at room temperature (25 °C). | |
According to the book wrote by Rolf, the dimerization constant of alkoxy-substituted HABI (at para-position of 2-phenyl ring) is the smallest compared to others, which supports the result that τ1/2 (C8H17–O–HABI) is larger than that of HABI. What's more, τ1/2 (Cz–O–HABI), τ1/2 (NI–O–HABI) and τ1/2 (PMI–O–HABI) are all even larger than that of τ1/2 (C8H17–O–HABI), which indicates the thermal recombination speeds of them are much slower than that of C8H17–O–HABI.
As we all know, when substituted HABIs are transformed to TPIRs upon UV irradiation, the radicals would diffuse randomly in the surrounding solvents. If we treat the recombination of two TPIRs forming HABIs as chemical reaction processes, naturally, the collision between two molecular TPIRs containing bulk groups will become less effective compared to that of C8H17–O–HABI and HABI, and as a result all of them exhibit a larger τ1/2 value. On the other hand, a bulk groups substituted TPIR might be clamped much easier by another pair of TPIRs during the recombination process, forming a “sandwich like” structure making the solution remaining colored and delaying the back reaction speed of TPIRs as a result.
The decay profiles of C8H17–O–HABI, Cz–O–HABI, NI–O–HABI and PMI–O–HABI are also investigated in PMMA film (see Fig. S6†). All of them exhibit similar characters with negligible differences.
Fatigue resistance properties of Cz–O–HABI, NI–O–HABI and PMI–O–HABI in solutions
The fatigue resistance properties of Cz–O–HABI, NI–O–HABI and PMI–O–HABI are performed by detecting the absorbance and FL intensity changes in solutions using UV and darkness treatments. The detail procedures for all the experiments are presented in ESI, Fig. S5.† We can see that all of these three fluorescent HABIs: Cz–O–HABI, NI–O–HABI and PMI–O–HABI exhibit efficient fatigue resistance in benzene solutions.
Conclusions
In this paper, we design and synthesis a series of novel hexaarylbiimidazole (HABI)-containing fluorophores with broad-spectrum emissions ranging from blue, green to red emissions. All of them show moderate fluorescent quantum yield of 25–27% in benzene. Their photochromism properties are proved by UV-Vis absorption and FL spectra in solution and PMMA thin film. We conclude that the photochromism properties of all the designed non-conjugated emissive HABIs (Cz–O–HABI, NI–O–HABI and PMI–O–HABI) are determinate by the HABI part by comparison with C8H17–O–HABI. Upon UV irradiation, the fluorescence quenches by a FRET mechanism from the fluorophores (Cz, NI, PMI) to the TPIRs and in this way, blue (390 nm), green (490 nm) and red (650 nm) fluorescent HABIs photoswitches have been established. We also investigate their kinetic of the radical recombination (back reaction) and fatigue resistance properties by UV-Vis and FL spectra. Although, the back reaction speeds of them are slower than that of HABI and C8H17–O–HABI, we believe this defect could be solved by inhibiting the diffusion of the radicals as formerly proposed by Jiro Abe's group. Accompanying the former green fluorescent HABI (NI–O–HABI), we demonstrate that the full color: blue, green and red fluorescent HABIs can be achieved by proper molecular design. Further chemistry work that designing diffusion-inhibited HABIs are undertaken in our lab.
Acknowledgements
This work was supported by the NSFC (21174045, 21474034), National Basic Research Program of China (Grant no. 2013CB922104). We also thank the support from the Analytical and Testing Center of Huazhong University of Science and Technology.
Notes and references
-
(a) G. Berkovic, V. Krongauz and V. Weiss, Chem. Rev., 2000, 100, 1741–1754 CrossRef CAS PubMed;
(b) H. M. D. Bandara and S. C. Burdette, Chem. Soc. Rev., 2012, 41, 1809 RSC.
-
(a) M.-Q. Zhu, G.-F. Zhang, C. Li, M. P. Aldred, E. Chang, R. A. Drezek and A. D. Q. Li, J. Am. Chem. Soc., 2011, 133, 365 CrossRef CAS PubMed;
(b) M.-Q. Zhu, L. Zhu, J. J. Han, W. Wu, J. K. Hurst and A. D. Q. Li, J. Am. Chem. Soc., 2006, 128, 4303–4309 CrossRef CAS PubMed;
(c) L. Zhu, M. Q. Zhu, J. K. Hurst and A. D. Q. Li, J. Am. Chem. Soc., 2005, 127, 8968–8970 CrossRef CAS PubMed;
(d) L. Zhu, W. Wu, M. Q. Zhu, J. J. Han, J. K. Hurst and A. D. Q. Li, J. Am. Chem. Soc., 2007, 129, 3524–3526 CrossRef CAS PubMed;
(e) D. Hu, Z. Tian, W. Wu, W. Wan and A. D. Q. Li, J. Am. Chem. Soc., 2008, 130, 15279–15281 CrossRef CAS PubMed.
-
(a) S. Maeda, Spirooxazines, in Organic Photochromic and Thermochromic Compounds, ed. J. C. Crano and R. J. Guglielmetti, Kluwer Academic/Plenum Publishers, New York, 1999, vol. 1, pp. 85–109 Search PubMed;
(b) J. D. Hepworth and B. M. Heron, in Functional dyes, ed. S.-H. Kim, Amsterdam, Elsevier, 2006, pp. 85–135 Search PubMed.
- T. Hayashi and K. Maeda, Bull. Chem. Soc. Jpn., 1960, 33, 565–566 CrossRef CAS.
- B. Radziszewski, Ber. Dtsch. Chem. Ges., 1877, 10, 70 CrossRef.
-
(a) D. M. White and J. Sonnenberg, J. Am. Chem. Soc., 1966, 88, 3825–3829 CrossRef CAS;
(b) T. Hayashi and K. Maeda, Bull. Chem. Soc. Jpn., 1970, 43, 429–438 CrossRef;
(c) R. L. Cohen, J. Org. Chem., 1971, 36, 2280–2284 CrossRef CAS;
(d) L. A. Cescon, G. R. Coraor, R. Dessauer, E. F. Silversmith and E. J. Urban, J. Org. Chem., 1971, 36, 2262–2267 CrossRef;
(e) A. Kikuchi, T. Iyoda and J. Abe, Chem. Commun., 2002, 1484–1485 RSC.
-
(a) A. P. Demchenko, Introduction to Fluorescence Sensing, Springer, Berlin, Heidelberg, 2008 Search PubMed;
(b) R. Martinez-Manez and F. Sancenon, Chem. Rev., 2003, 103, 4419–4476 CrossRef CAS PubMed;
(c) K. Rurack and U. Resch-Genger, Chem. Soc. Rev., 2002, 31, 116–127 RSC;
(d) W. S. Han, H. Y. Lee, S. H. Jung, S. J. Lee and J. H. Jung, Chem. Soc. Rev., 2009, 38, 1904–1915 RSC;
(e) F. Mancin, E. Rampazzo, P. Tecilla and U. Tonellato, Chem.–Eur. J., 2006, 12, 1844–1854 CrossRef CAS PubMed;
(f) L. Prodi, New J. Chem., 2005, 29, 20–31 RSC.
- M. Irie, Chem. Rev., 2000, 100, 1683–1890 CrossRef CAS PubMed.
- M. G. Kuz'min and M. V. Koz'menko, in Organic Photochromes, ed. A. V. El'tsov, Consultants Bureau, New York, 1990, pp. 245–265 Search PubMed.
-
(a) F. M. Raymo and M. Tomasulo, Chem. Soc. Rev., 2005, 34, 327–336 RSC;
(b) F. M. Raymo and M. Tomasulo, J. Phys. Chem. A, 2005, 109, 7343–7352 CrossRef CAS PubMed;
(c) J. Cusido, E. Deniz and F. M. Raymo, Eur. J. Org. Chem., 2009, 2031–2045 CrossRef;
(d) I. Yildiz, E. Deniz and F. M. Raymo, Chem. Soc. Rev., 2009, 38, 1859–1867 RSC.
-
(a) J. Walz, K. Ulrich, H. Port, H. C. Wolf, J. Wonner and F. Effenberg, Chem. Phys. Lett., 1993, 213, 321–324 CrossRef CAS;
(b) M. Seibold, H. Port and H. C. Wolf, Mol. Cryst. Liq. Cryst., 1996, 283, 75–80 CrossRef;
(c) H. Port, M. Hennrich, M. Seibold and H. C. Wolf, Proc. Electrochem. Soc., 1998, 98, 61–70 Search PubMed;
(d) H. Port, A. Hartschuh, M. Hennrich, H. C. Wolf, J. M. Endtner and F. Effenberger, Mol. Cryst. Liq. Cryst., 2000, 344, 145–150 CrossRef CAS;
(e) I. B. Ramsteiner, A. Hartschuh and H. Port, Chem. Phys. Lett., 2001, 343, 83–90 CrossRef CAS.
-
(a) T. B. Norsten and N. R. Branda, Adv. Mater., 2001, 13, 347–349 CrossRef CAS;
(b) A. J. Myles and N. R. Branda, J. Am. Chem. Soc., 2001, 123, 177–178 CrossRef CAS;
(c) T. B. Norsten and N. R. Branda, J. Am. Chem. Soc., 2001, 123, 1784–1785 CrossRef CAS;
(d) C. Li, H. Yan, G. F. Zhang, W. L. Gong, T. Chen, M. P. Aldred and M. Q. Zhu, Chem.–Asian J., 2014, 9, 104–109 CrossRef CAS PubMed;
(e) A. Myles and N. R. Branda, Adv. Funct. Mater., 2002, 12, 167–173 CrossRef CAS.
-
(a) T. Kawai, T. Sasaki and M. Irie, Chem. Commun., 2001, 711–712 RSC;
(b) L. Giordano, T. M. Jovin, M. Irie and E. A. Jares-Erijman, J. Am. Chem. Soc., 2002, 124, 7481–7489 CrossRef CAS PubMed;
(c) M. Irie, T. Fukaminato, T. Sasaki, N. Tamai and T. Kawai, Nature, 2002, 420, 759–760 CrossRef CAS PubMed;
(d) M. S. Kim, T. Kawai and M. Irie, Opt. Mater., 2002, 275–278 Search PubMed;
(e) T. Fukaminato, T. Sasaki, T. Kawai, N. Tamai and M. Irie, J. Am. Chem. Soc., 2004, 126, 14843–14849 CrossRef CAS PubMed;
(f) E. A. Jares-Erijman, L. Giordano, C. Spagnuolo, J. Kawior, R. J. Verneij and T. M. Jovin, Proc. SPIE-Int. Soc. Opt. Eng., 2004, 5323, 13–26 CrossRef CAS PubMed.
-
(a) J. L. Bahr, G. Kodis, L. de la Garza, S. Lin, A. L. Moore, T. A. Moore and D. Gust, J. Am. Chem. Soc., 2001, 123, 7124–7133 CrossRef CAS PubMed;
(b) J. Andreasson, G. Kodis, Y. Terazono, P. A. Liddell, S. Bandyopadhyay, R. H. Mitchell, T. A. Moore, A. L. Moore and D. Gust, J. Am. Chem. Soc., 2004, 126, 15926–15927 CrossRef CAS PubMed;
(c) Y. Terazono, G. Kodis, J. Andreasson, G. Jeong, A. Brune, T. Hartmann, H. Dürr, T. A. Moore, A. L. Moore and D. Gust, J. Phys. Chem. B, 2004, 108, 1812–1814 CrossRef CAS;
(d) S. D. Straight, J. Andreasson, G. Kodis, S. Bandyopadhyay, R. H. Mitchell, T. A. Moore, A. L. Moore and D. Gust, J. Am. Chem. Soc., 2005, 127, 9403–9409 CrossRef CAS PubMed;
(e) S. D. Straight, P. A. Liddell, Y. Terazono, T. A. Moore, A. L. Moore and D. Gust, Adv. Funct. Mater., 2007, 17, 777–785 CrossRef CAS.
-
(a) M. Tomasulo, E. Deniz, R. J. Alvarado and F. M. Raymo, J. Phys. Chem. C, 2008, 112, 8038–8045 CrossRef CAS;
(b) E. Deniz, S. Sortino and F. M. Raymo, J. Phys. Chem. Lett., 2010, 1, 1690–1693 CrossRef CAS;
(c) E. Deniz, M. Tomasulo, R. A. DeFazio, B. D. Watson and F. M. Raymo, Phys. Chem. Chem. Phys., 2010, 12, 11630–11634 RSC;
(d) E. Deniz, S. Ray, M. Tomasulo, S. Impellizzeri, S. Sortino and F. M. Raymo, J. Phys. Chem. A, 2010, 114, 11567–11575 CrossRef CAS PubMed.
-
(a) I. L. Medintz, S. A. Trammell, H. Mattoussi and J. M. Mauro, J. Am. Chem. Soc., 2004, 126, 30–31 CrossRef CAS PubMed;
(b) I. L. Medintz, A. R. Clapp, S. A. Trammell and H. Mattoussi, Proc. SPIE-Int. Soc. Opt. Eng., 2004, 5593, 300–307 CrossRef PubMed.
-
(a) E. J. Harbron, D. A. Vicente and M. T. Hoyt, J. Phys. Chem. B, 2004, 108, 18789–18792 CrossRef CAS;
(b) E. J. Harbron, D. A. Vicente, D. H. Hadley and M. R. Imm, J. Phys. Chem. A, 2005, 109, 10846–10853 CrossRef CAS PubMed;
(c) A. F. Grimes, S. E. Call, D. A. Vicente, D. S. English and E. J. Harbron, J. Phys. Chem. B, 2006, 110, 19183–19190 CrossRef CAS PubMed;
(d) S. M. Lewis and E. J. Harbron, J. Phys. Chem. C, 2007, 111, 4425–4430 CrossRef CAS.
-
(a) E. Jares-Erijman, L. Giordano, C. Spagnuolo, K. A. Lidke and T. M. Jovin, Mol. Cryst. Liq. Cryst., 2005, 430, 257–265 CrossRef CAS;
(b) S. Mikoski, L. Giordano, M. H. Etchelon, G. Menendez, K. A. Lidke, G. M. Hagen, T. M. Jovin and E. Jares-Erijman, Proc. SPIE-Int. Soc. Opt. Eng., 2006, 6096, 60960X CrossRef PubMed.
-
(a) J. Finden, T. K. Kunz, N. R. Branda and M. O. Wolf, Adv. Mater., 2008, 20, 1998–2002 CrossRef CAS;
(b) M. Q. Zhu, T. Chen, G. F. Zhang, C. Li, W. L. Gong, Z. Q. Chen and M. P. Aldred, Chem. Commun., 2014, 50, 2664–2666 RSC.
- M. Irie, Chem. Rev., 2000, 100, 1685–1716 CrossRef CAS PubMed.
- M. Heilemann, P. Dedecker, J. Hofkens and M. Sauer, Laser Photonics Rev., 2009, 3, 180 CrossRef CAS.
-
(a) P. Dedecker, J. Hotta, C. Flors, M. Sliwa, H. Uji-i, M. B. Roeffaers, R. Ando, H. Mizuno, A. Miyawaki and J. Hofkens, J. Am. Chem. Soc., 2007, 129, 16132–16141 CrossRef CAS PubMed;
(b) C. Flors, J. Hotta, H. Uji-i, P. Dedecker, R. Ando, H. Mizuno, A. Miyawaki and J. Hofkens, J. Am. Chem. Soc., 2007, 129, 13970–13977 CrossRef CAS PubMed;
(c) R. Ando, H. Mizuno and A. Miyawaki, Science, 2004, 306, 1370–1373 CrossRef CAS PubMed;
(d) S. Habuchi, R. Ando, P. Dedecker, W. Verheijen, H. Mizuno, A. Miyawaki and J. Hofkens, Proc. Natl. Acad. Sci. U. S. A., 2005, 102, 9511–9516 CrossRef CAS PubMed.
-
(a) M. Q. Zhu, G. F. Zhang, Z. Hu, M. P. Aldred, C. Li, W. L. Gong, T. Chen, Z. L. Huang and S. Y. Liu, Macromolecules, 2014, 47(5), 1543–1552 CrossRef CAS;
(b) C. Li, W. L. Gong, Z. Hu, M. P. Aldred, G. F. Zhang, T. Chen, Z. L. Huang and M. Q. Zhu, RSC Adv., 2013, 3, 8967–8972 RSC.
- W. L. Gong, Z. Hu, C. Li, G. F. Zhang, T. Chen, M. P. Aldred, Z. L. Huang and M. Q. Zhu, Front. Optoelectron., 2013, 6, 458–467 CrossRef PubMed.
- K. Mutoh, M. Sliwa and J. Abe, J. Phys. Chem. C, 2013, 117, 4808 CAS.
- W. L. Gong, G. F. Zhang, C. Li, M. P. Aldred and M. Q. Zhu, RSC Adv., 2013, 3, 9167–9170 RSC.
- R. Dessauer, in Photochemistry, History and Commercial Applications of Hexaarylbiimidazoles, Elsevier, Amsterdam, London, 2006 Search PubMed.
Footnote |
† Electronic supplementary information (ESI) available: Details on the materials for synthesis, instrumentation for analysis and spectral characterizations. See DOI: 10.1039/c4ra10451j |
|
This journal is © The Royal Society of Chemistry 2014 |