DOI:
10.1039/C4RA09364J
(Paper)
RSC Adv., 2014,
4, 55846-55852
Imprinting of bovine serum albumin in a nonwoven polypropylene membrane supported polyacrylamide/calcium alginate interpenetrating polymer network hydrogel
Received
27th August 2014
, Accepted 22nd October 2014
First published on 22nd October 2014
Abstract
A bovine serum albumin (BSA) imprinted nonwoven polypropylene (PP) fibre supported calcium alginate/polyacrylamide (CA/PAM) hydrogel film (PP-s-CA/PAM MIP) was prepared using nonwoven PP as matrix, BSA as template molecule, sodium alginate (SA) and acrylamide (AM) as functional monomers, N,N′-methylenebisacrylamide (MBA) as covalent cross-linker, and CaCl2 as ionic cross-linker by UV radiation-reduced polymerization in an aqueous phase. Factors affecting the adsorption capacity and imprinting efficiency of the BSA-imprinted PP-s-CA/PAM MIPs were investigated. The results showed that PP-s-CA/PAM MIPs exhibited an obvious improvement in terms of adsorption capacity and recognition for BSA compared with the non-imprinted ones. The adsorption capacity of MIP for BSA reached 27.02 mg g−1 when the adsorption time was 900 min, which was 2.8 times the non-imprinted one. The swelling of the CA/PAM hydrogel supported on PP was significantly reduced. In addition, the regeneration property of the BSA-imprinted PP-s-CA/PAM hydrogel was distinctly improved. The imprinting efficiency of PP-s-CA/PAM MIP maintained 92.95% of the initial value even after five repetitions.
1. Introduction
Molecular imprinting is a useful technique for forming complementary binding sites by copolymerizing suitable functional monomers in the presence of suitable template molecules.1,2 Molecularly imprinted polymers (MIPs) are characterized by their thermal and chemical stability, high specificity, ease of mass preparation, low cost and reusability. MIPs have been used in various applications including separation,3,4 solid-phase extraction,5 catalysis,6 biomimetic sensors,7 and drug delivery.8 Although molecular imprinting has been successfully applied in imprinting low-molecular weight compounds,9,10 the imprinting of bio-macromoleculars, in particular proteins, is still a significant challenge due to the inherent technical problems.11,12 Proteins are usually instable and insoluble and can easily be affected by temperature or other environmental factors.
Polyacrylamide (PAM) hydrogels can be easily produced and have been identified as suitable imprinting matrices for biological molecules.13–20 However, PAM hydrogels have poor regeneration properties because of their soft texture.17 The mass transfer of proteins into PAM bulk hydrogels is difficult. Surface imprinting protocols can eliminate the problems associated with mass transfer.18,19 Lu et al.20 fabricated BSA and lysozyme surface-imprinted magnetic gel microspheres by using magnetic composite gel microspheres as seeds via inverse-phase seed suspension polymerization. Qin et al.21 fabricated lysozyme imprinted polymer beads by using chloromethylated polystyrene beads as supports via surface-initiated living radical polymerization in aqueous media. Guo et al.22,23 prepared bovine haemoglobin imprinted chitosan microspheres by trapping selective soft PAM gel in the pores of the cross-linked chitosan beads.
Almost all protein-imprinted PAM hydrogels are particles or microspheres and organic solvents are often used as dispersion medium, which inevitably causes protein denaturation. Films have more advantages than microspheres or granules in biological applications such as cell culture. However, it is difficult to obtain PAM hydrogel thin films. Protein molecularly imprinted alginate film was prepared using an aqueous imprinting method without any organic chemicals, which was favourable in life science applications such as medical devices, food additives, or drug delivery systems.24 Suo et al.25 synthesized highly stretchable and tough hydrogels from sodium alginate (SA) and acrylamide (AM). The hybrid hydrogels may exhibit good performance in maintaining the imprinted cavities and improving the regeneration property of the MIPs. In our previous work, bovine serum albumin (BSA) imprinted polypropylene (PP) fibre-grafted polyacrylamide hydrogel was prepared using non-woven PP fibre as matrix, BSA as template molecule, and acrylamide as functional monomer. The imprinting efficiency of MIP maintained at 76% of the initial value even after five repetitions.26 However, the adsorption capacity of the MIP was low, and the strength of the PAM hydrogel was poor.
In this paper, BSA imprinted nonwoven PP fibre supported calcium alginate/polyacrylamide (PP-s-CA/PAM MIP) hydrogel film was prepared by UV radiation-reduced polymerization using PP as matrix, BSA as template, SA and AM as functional monomers, N,N′-methylenebisacrylamide (MBA) and CaCl2 as crosslinkers. The swelling of CA/PAM hydrogel supported on nonwoven PP was reduced and the regeneration property of BSA imprinted PP-s-CA/PAM hydrogel was distinctly improved. Factors affecting the adsorption capacity and imprinting efficiency of PP-s-CA/PAM MIPs were investigated. The adsorption capacity of MIP reached 27.02 mg g−1, which was 2.8 times of the non-imprinted ones. The MIPs can be easily prepared and applied in protein analysis and testing, cell culture, as well as the controlled release and separation of proteins.
2. Experimental
2.1 Materials
Nonwoven PP fibre (22 g m−2) were purchased from Xianghehuaxin Nonwoven Company, Ltd. (Langfang, China). SA was purchased from Tianjin Yuanhang chemical company. Acrylamide (AM) and MBA were analytical reagents and purchased from Chemistry Reagent Factory of Tianjin (Tianjin, China). Acetic acid (HAc), sodium dodecyl sulfate (SDS), and ammonium persulfate (APS) were obtained from the Institute of Tianjin Guangfu Fine Chemicals (Tianjin, China). BSA, ovalbumin (Ova), bovine hemoglobin (Hb), lysozyme (Lyz), and bovine γ-globulin (Glo) were purchased from Lanji of Shanghai Science and Technology Development Company (Shanghai, China).
2.2 Preparation of PP-s-CA/PAM MIP
Fig. 1 shows the schematic representation of the fabrication procedure of the BSA-imprinted PP-s-PAM/CA. Nonwoven PP fibre (640 mg) was introduced into 30 mL aqueous solution consisting of BSA (45 mg), AM (3 g), APS (30 mg), MBA (2.4 mg) and SA (150 mg). The mixture was then incubated for 1 h at room temperature for the pre-assembly between the template molecules and functional monomers. The nonwoven PP fibres immersed in the mixture were transferred into quartz glass sheet, purged with nitrogen for 8 min, and then sealed. Then the polymerization was conducted for 0.5 h by UV irradiation at room temperature to produce the polymer hydrogel. The hydrogel supported on nonwoven PP fibres were rinsed repeatedly with distilled water to remove unreacted monomers and crosslinkers. The protein in the hydrogel was eluted with HAc solution (10%, v/v) containing SDS (10%, w/v) until no BSA in the supernatant was detected by measuring the UV absorbance at 280 nm. The nonwoven PP supported hydrogel was then extensively washed with deionized water to remove the remnant SDS and HAc. Then the hydrogel supported on nonwoven PP was cross-linked with 2.5% CaCl2 to obtain PP-s-CA/PAM MIP.
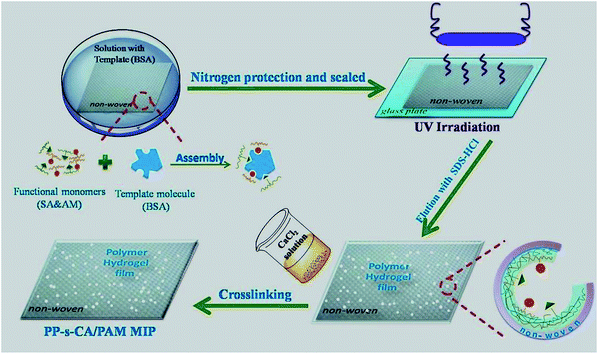 |
| Fig. 1 Schematic representation of the fabrication procedure of BSA-imprinted PP-s-CA/PAM. | |
Nonwoven PP supported non-imprinted CA/PAM (i.e., PP-s-CA/PAM NIP) was also prepared using the same procedures but without the template.
2.3 Preparation of PP-g-PAM MIP and PAM MIP
BSA imprinted nonwoven PP fibre supported polyacrylamide hydrogel film (PP-g-PAM MIP) and polyacrylamide (PAM) hydrogel microspheres (PAM MIP) were prepared according to the literature.27–30 The non-imprinted hydrogels (i.e., PP-g-PAM NIP and PAM NIP) were also prepared using the same procedure but without the template.
2.4 Characterization
The morphologies of PP and PP-s-CA/PAM MIP were observed using a scanning electron microscope (FESEM; S-4800, HITACHI, Japan).
The thickness of wet CA/PAM hydrogel was measured with a digital display micrometer gauge from Shanghai Luchuan co., LTD.
2.5 Swelling performance
Water on the surface of wet CA/PAM and PP-s-CA/PAM was removed using a filter paper, and the weight of the wet hydrogels was measured. The CA/PAM and PP-s-CA/PAM were soaked in normal saline (0.9 wt% NaCl), and the mass of the hydrogels was measured at time t. The swelling ratio (SR) of CA/PAM and PP-s-CA/PAM under different time is defined as follows:where Ww and Wt are the weight of the wet hydrogel before and after swelling at time t, respectively. The weight of the nonwoven PP was reduced from that of the PP-s-CA/PAM in the swelling degree calculation.
2.6 Adsorption experiments
Surface water on the wet PP-s-CA/PAM MIPs or NIPs was absorbed using filter paper. Subsequently, 1.0 g of wet PP-s-CA/PAM MIPs or NIPs were added in each glass bottle containing 10 mL BSA solutions (1.36 mg mL−1) to evaluate the imprinting efficiency and dynamic adsorption or various BSA concentrations (0 mg mL−1 to 3.0 mg mL−1) to determine the adsorption isotherms. At certain intervals, the supernatant solutions were collected and determined with a UV spectrophotometer. The experiments were carried out for three times and the averages of the results were used. The equilibrium adsorption capacity Qe (mg g−1) of the protein adsorbed was calculated according to the following equation:where W (g) is the mass of the dried MIPs or NIP, V (mL) is the volume of the BSA solution, C0 and Ce are the initial and final BSA concentrations at equilibrium, respectively. The imprinting efficiency (IE) of MIPs was defined as the following equation:27–30where QMIP and QNIP are the Qe of MIPs and NIPs, respectively.
Dynamic adsorption capacity Qt at time t was calculated according to the following equation:
where
Ct (mg mL
−1) is the BSA concentration in the supernatant at time
t.
2.7 Recognition performance
Wet PP-s-CA/PAM MIPs or NIPs (0.5 g) were placed into each glass bottle containing 10 mL of 1.36 mg mL−1 different protein solutions for 24 h to evaluate the recognition performances. The recognition performance of MIPs can be evaluated by the static distribution coefficient KD, the separation factor α and the relative separation factors β, which are defined in the following equations:31–32where CP is the concentration of protein on wet gel, and CS is the concentration of protein in the solution.where KD1 and KD2 are the static distribution coefficients of the template and other competitive proteins, respectively.
The relative separation factors β was introduced and defined as follows:
where
αI and
αN are the separation factors of the template on MIPs and NIPs.
3. Results and discussions
3.1 Surface morphology
Fig. 2 shows the digital (wet) and SEM images of the nonwoven PP and PP-s-CA/PAM MIP. The wet CA/PAM was transparent with a thickness of approximately 0.23 mm. Significant lamellar macroporous structure was found on the surface of PP-s-CA/PAM MIP (Fig. 2(d)). The macroporous structure on the surface of CA/PAM hydrogel facilitated the diffusion of the protein template. Imprinting pores were not found because they were too small and the water evaporation in vacuum resulted in pore shrinkage.
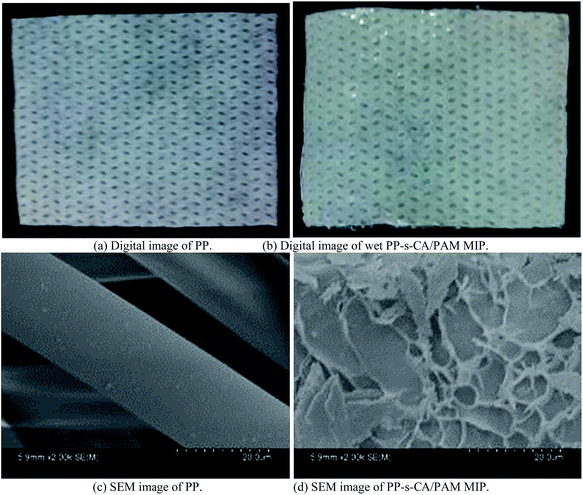 |
| Fig. 2 Digital (wet) and SEM images of nonwoven PP and PP-s-CA/PAM MIP. | |
3.2 Factors affecting Qe and IE of MIP
The effects of different MBA concentrations on the Qe and IE of PP-s-CA/PAM MIP are shown in Fig. 3. The adsorption of BSA on MIP increased gradually with the initial increase in MBA concentration, and then decreased rapidly when MBA concentration ≥0.08 wt%. At low MBA concentration, Qe was also low because MIP is too weak to maintain the imprinted cavities. By contrast, the Qe of MIP decreased when MBA concentration ≥0.08 wt% because excessively crosslinked PP-s-CA/PAM hydrogel hindered protein diffusion. IE reached its maximum value when the proportion of MBA was 0.08 wt%.
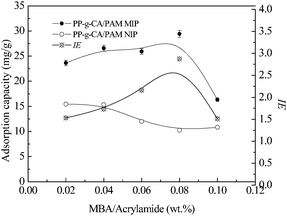 |
| Fig. 3 Effects of different MBA concentrations on the Qe and IE of PP-s-CA/PAM MIP and NIP. Monomer concentration was 10 wt%; SA concentration was 0.5 wt%. | |
The effects of monomer concentration on the Qe and IE of MIP are shown in Fig. 4. The Qe of MIP and NIP both increased with the increase in monomer concentration from 5 wt% to 10 wt%. The Qe values of BSA on both MIP and NIP reached their maximum values when the monomer concentration was 10 wt%. The BSA adsorption on MIP was significantly higher than that of NIPs at low monomer concentration. The Qe of MIP and NIP decreased when the monomer concentration was >10 wt%. When the monomer concentration was 5 wt%, IE reached its maximum value (2.65). At low monomer concentration (<10 wt%), the increase in monomer concentration resulted in an increase in Qe of BSA on MIPs because more imprinting cavities were created. However, the adsorption of BSA on MIPs decreased when the monomer concentration was above 10%, which was attributed to the difficulty in the absolute removal of the templates from the hydrogel matrix.
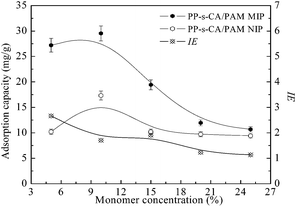 |
| Fig. 4 Effects of monomer concentration on the Qe and IE of PP-s-CA/PAM MIP and NIP. SA concentration was 0.5 wt%; MBA/acrylamide = 0.08 wt%. | |
SA concentration showed critical effect on the Qe and IE of PP-s-CA/PAM MIP. Fig. 5 shows that MIP had maximum Qe and IE values when the concentration of SA was 0.5 wt%. Initially, the adsorption of BSA on MIP rapidly increased with the increase in SA. The Qe of protein decreased gradually when the concentration of SA was above 0.5 wt%. At low SA concentration, the increase in Qe was attributed to the formation of alginate/PAM interpenetrating network, which increased the number of imprinted cavities. In addition, the carboxylate anion of alginate interacted with amino in BSA, which was beneficial for the adsorption of BSA. However, the space mesh of the hydrogel was reduced when SA concentration >0.5 wt%, which hindered mass transfer of BSA, causing the decrease in Qe.
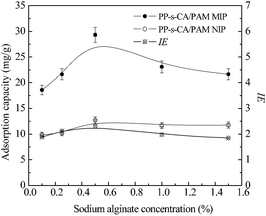 |
| Fig. 5 Effects of SA concentration on the Qe and IE of PP-s-CA/PAM MIP and NIP. Monomer concentration was 10 wt%, MBA/acrylamide = 0.08 wt%. | |
3.3 Adsorption kinetics
The adsorption dynamic curves of PP-s-CA/PAM MIP and NIP are shown in Fig. 6. MIP adsorbed more BSA than NIP, and the Qe of MIP was almost three times of that of NIP. An abrupt increase in the adsorption capacity of MIP was observed during the first 360 min, and the adsorption capacity almost reached equilibrium after 900 min. At the beginning, a large number of imprinted cavities exposed on the surface of MIP, and the template easily reached the specific binding sites, resulting in high IE of the MIP. When the superficial imprinting sites were filled up, the adsorption rate declined and the IE of MIP decreased.
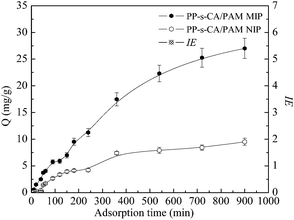 |
| Fig. 6 Adsorption kinetic curve. | |
3.4 Adsorption thermodynamic
Fig. 7 shows the thermodynamic adsorption curves of PP-s-CA/PAM MIP and NIP. The Qe of MIP increased gradually at low BSA concentrations because the amount of BSA was insufficient to fill up the specific binding cavities. However, almost all specific imprinted sites were gradually occupied and the Qe of MIPs became steady with the increase in BSA concentration. PP-s-CA/PAM MIPs showed higher adsorption capacity for BSA than NIPs.
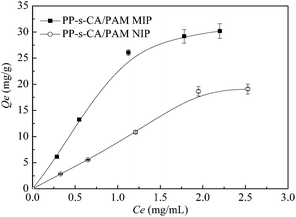 |
| Fig. 7 Thermodynamic adsorption curves of PP-s-CA/PAM MIP and NIP. Monomer concentration was 10 wt%, SA concentration was 0.5 wt%, and MBA/acrylamide = 0.08 wt%. | |
3.5 Regeneration properties
After BSA absorption, the PP-s-CA/PAM MIP was washed with 10% (v/v) acetic acid containing 1% (w/v) SDS to remove BSA, and then was applied to re-adsorb BSA. The imprinting reproducibility of MIP was examined for several repeated adsorption–regeneration cycles, and the regeneration property of BSA-imprinted PAM hydrogel bead was also investigated.27 The IE of PP-s-CA/PAM MIP decreased more slowly than those of PP-g-PAM MIP and PAM MIP and was maintained at 92.95% of the initial value even after five cycles (Fig. 8). However, the IE of the BSA-imprinted PAM microspheres declined with the increase of cycle times; the imprinting effect almost disappeared after five cycle times. The soft texture of the PAM gel microspheres cannot stabilize the shape of the binding sites. By contrast, the strength of PP-s-CA/PAM was significantly improved and the shape of the binding sites was stabilized. In addition, the swelling of CA/PAM hydrogel supported on nonwoven PP was significantly reduced (Fig. 9). A 0.9% NaCl solution was used for swelling performance because 0.9% NaCl solution is often used in cell culture, protein adsorption and release in simulated body fluids. CA/PAM hydrogel remarkably swelled because the Ca2+ in CA/PAM hydrogel was replaced by Na+ in physiological saline. The SR of CA/PAM hydrogel reached 42.94% after 300 min. However, the SR of nonwoven PP supported CA/PAM hydrogel almost reached equilibrium after 20 min, with a value of 8.67%. Thus, PP-s-CA/PAM maintained the imprinted cavities without distortion better than PAM and CA/PAM.
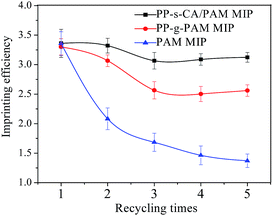 |
| Fig. 8 Curves of the regeneration properties of PP-s-CA/PAM MIP, PP-g-PAM MIP and PAM MIP. | |
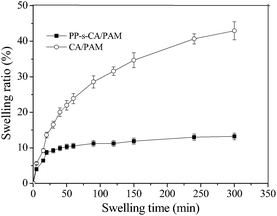 |
| Fig. 9 Swelling ratio of PP-s-CA/PAM and CA/PAM in 0.9 wt% NaCl solution under different time. | |
3.6 Recognition performance
Table 1 shows the Qe, α, and β values of PP-s-CA/PAM MIP and NIP for BSA and competitive proteins. MIP exhibited good adsorption selectivity for BSA template. The Qe of BSA on MIP was higher than those of Lyz, Ova, Hb, and Glo. The α and β of BSA-imprinted PP-s-CA/PAM increased with the increase in protein Mw, except for HB. The higher BSA affinity of MIP is attributed to the generation of BSA high-affinity adsorption sites and the complementary cavities in the hydrogel during polymerization. Thus, the template protein was strongly bound to the MIP. For the competitive proteins, although Lyz was small enough to diffuse into the imprinting cavities, it had less chance to be adsorbed on the PP-s-CA/PAM MIP because it was not complementary with the recognition sites. The Qe of γ-Glo on MIP was significantly lower than that of any protein because the molecular volume of γ-Glo was remarkably larger than those of other proteins. PP-s-CA/PAM NIP showed lower Qe for BSA than MIP, which is attributed to the failure of NIP to form specific recognition sites because of the absence of template BSA. As basic proteins, Lyz and Hb showed more favorable interactions with PP-s-CA/PAM, thereby causing non-specific adsorption and poor adsorption selectivity. Larger protein such as Glo was likely to be excluded from the binding cavities, which resulted in lower Qe and higher α and β values.
Table 1 Qe, α and β values of BSA-imprinted and non-imprinted PP-s-CA/PAM
Protein properties |
Qe(MIP) |
Qe(NIP) |
αMIP |
αNIP |
β |
|
Mw (kDa) |
pI |
BSA MIP |
NIP |
BSA MIP |
NIP |
— |
Lyz |
15 |
10.5 |
17.71 ± 0.99 |
14.50 ± 0.84 |
1.611 ± 0.12 |
0.549 ± 0.03 |
2.94 ± 0.19 |
Ova |
43 |
4.7 |
9.17 ± 0.73 |
10.37 ± 0.71 |
3.177 ± 0.18 |
0.774 ± 0.05 |
4.11 ± 0.22 |
Hb |
64 |
6.9 |
9.36 ± 0.56 |
6.05 ± 0.45 |
3.103 ± 0.22 |
1.338 ± 0.08 |
2.32± 0.12 |
BSA |
67 |
4.9 |
27.02 ± 1.54 |
9.52 ± 0.75 |
— |
— |
— |
Glo |
160 |
7.1 |
5.09 ± 0.32 |
4.94 ± 0.36 |
5.762 ± 0.35 |
1.652 ± 0.096 |
3.49 ± 0.25 |
4. Conclusions
BSA-imprinted PP fibre supported CA/PAM hydrogel film was successfully synthesized by UV radiation-reduced polymerization using nonwoven PP fibre as matrix, BSA as template, SA and AM as functional monomers, MBA as covalent crosslinker, and CaCl2 as ionic crosslinker. The swelling of PP-s-CA/PAM NIP was significantly reduced.
Analyses of the adsorption dynamics and isotherms showed that the PP-s-CA/PAM MIP exhibited significant improvement in adsorption capacity for BSA compared with NIP. The PP-s-CA/PAM MIP recognized the template protein, which was confirmed using Lyz, Ova, Hb, and Glo as control proteins; the selectivity factor (β) was above 2.3. The imprinting efficiency of PP-s-CA/PAM MIP was maintained at 92.95% of the initial value even after five repetitions. PP-s-CA/PAM maintained the imprinted cavities without distortion better than PAM and CA/PAM.
PP-s-CA/PAM MIP is low cost and can be easily prepared and applied in protein analysis and testing, cell culture, as well as controlled release and the separation of proteins.
Acknowledgements
The research is supported by the National Natural Science Foundation of China (51103102, 81173413), Ministry of education doctoral new teacher fund (20111201120004), and the Tianjin Colleges Science and Technology Development Fund (20100308, 20120211).
References
- Z. Zhang, L. X. Chen, F. F. Yang and J. H. Li, RSC Adv., 2014, 4, 31507 RSC.
- S. J. Li, S. S. Cao, M. J. Whitcombe and S. A. Piletsky, Prog. Polym. Sci., 2014, 39, 145–163 CrossRef CAS PubMed.
- W. Rao, R. Cai, Z. H. Zhang, Y. L. Yin, F. Long and X. X. Fu, RSC Adv., 2014, 4, 18503 RSC.
- Z. Y. Xu, J. F. Wan, S. Liang and X. J. Cao, Biochem. Eng. J., 2008, 41, 280 CrossRef CAS PubMed.
- M. Lasáková and P. Jandera, J. Sep. Sci., 2009, 32, 799 CrossRef PubMed.
- K. Y. Zhao, L. Z. Feng, H. Q. Lin, Y. F. Fu, B. B. Lin, W. K. Cui, S. D. Li and J. F. Wei, Catal. Today, 2014, 8, 127 CrossRef PubMed.
- E. Roy, S. K. Maity, S. Patra, R. Madhuri and P. K. Sharma, RSC Adv., 2014, 4, 32881 RSC.
- P. Wang, A. X. Zhang, Y. Jin, Q. Zhang, L. Y. Zhang, Y. Peng and S. H. Du, RSC Adv., 2014, 4, 26063 RSC.
- A. Mirmohseni, M. Shojaei and R. Pourata, RSC Adv., 2014, 4, 20177 RSC.
- G. Z. Kyzas, D. N. Bikiaris and N. K. Lazaridis, Chem. Eng. J., 2009, 149, 263 CrossRef CAS PubMed.
- N. Sankarakumar and Y. W. Tong, RSC Adv., 2013, 3, 1519 RSC.
- M. Anda and A. Denizli, RSC Adv., 2014, 4, 31130 RSC.
- D. M. Hawkins, D. Stevenson and S. Reddy, Anal. Chim. Acta, 2005, 542, 61 CrossRef CAS PubMed.
- S. J. Hjerten, J. L. Liao and K. Nakazato, Chromatographia, 1997, 44, 227 CAS.
- T. Y. Guo, Y. Q. Xia, J. Wang, M. D. Song and B. H. Zhang, Biomaterials, 2005, 26, 5737 CrossRef CAS PubMed.
- G. Q. Fu, J. Zhao, H. Yu, L. Liu and B. He, React. Funct. Polym., 2007, 67, 442–450 CrossRef CAS PubMed.
- X. S. Pang, G. X. Cheng, R. S. Li, S. L. Lu and Y. H. Zhang, Anal. Chim. Acta, 2005, 550, 13 CrossRef CAS PubMed.
- E. Verheyen, J. P. Schillemans, M. Wijk, M. A. Demeniex, W. E. Hennink and C. F. Nostrum, Biomaterials, 2011, 32, 3008 CrossRef CAS PubMed.
- L. Qin, X. W. He, W. Zhang, W. Y. Li and Y. K. Zhang, J. Chromatogr. A, 2009, 1216, 807 CrossRef CAS PubMed.
- S. L. Lu, G. X. Cheng and X. S. Pang, J. Appl. Polym. Sci., 2006, 99, 2401 CrossRef CAS.
- L. Qin, X. W. He, W. Zhang, W. Y. Li and Y. K. Zhang, Anal. Chem., 2009, 81, 7206 CrossRef CAS PubMed.
- T. Y. Guo, Y. Q. Xia, G. J. Hao, M. D. Song and B. H. Zhang, Biomaterials, 2004, 25, 5905 CrossRef CAS PubMed.
- G. Q. Fu, H. Yu and J. Zhu, Biomaterials, 2008, 29, 2138 CrossRef CAS PubMed.
- L. Carolyn, E. P. H. Bayer and N. A. Peppas, J. Biomater. Sci., Polym. Ed., 2011, 22, 1523 CrossRef PubMed.
- J. Y. Sun, X. H. Zhao, W. R. K. Illeperuma, O. Chaudhuri, K. H. Oh, D. J. Mooney, J. J. Vlassak and Z. G. Suo, Nature, 2012, 489, 133 CrossRef CAS PubMed.
- K. Y. Zhao, B. B. Lin, W. K. Cui, L. Z. Feng, T. Chen and J. F. Wei, Talanta, 2014, 121, 256 CrossRef CAS PubMed.
- X. S. Pang, G. X. Cheng, Y. H. Zhang and S. L. Lu, React. Funct. Polym., 2006, 66, 1182 CrossRef CAS PubMed.
- K. Y. Zhao, G. X. Cheng, J. J. Huang and X. G. Ying, React. Funct. Polym., 2008, 68, 732 CrossRef CAS PubMed.
- L. Z. Feng, B. H. Kan, K. Y. Zhao, J. F. Wei, D. W. Zhu and L. H. Zhang, J. Sol-Gel Sci. Technol., 2014, 71, 428 CrossRef CAS PubMed.
- K. Y. Zhao, G. X. Cheng, X. G. Ying and L. Chen, J. Appl. Polym. Sci., 2009, 113, 1133 CrossRef CAS.
- K. Y. Zhao, G. X. Cheng, J. J. Huang and X. G. Ying, React. Funct. Polym., 2008, 68(3), 732 CrossRef CAS PubMed.
- K. Y. Zhao, J. J. Huang, X. G. Ying and G. X. Cheng, J. Appl. Polym. Sci., 2008, 109, 2687 CrossRef CAS.
|
This journal is © The Royal Society of Chemistry 2014 |
Click here to see how this site uses Cookies. View our privacy policy here.