DOI:
10.1039/C4RA07577C
(Review Article)
RSC Adv., 2014,
4, 45128-45135
Comparison of different probes based on labeled annexin V for detection of apoptosis
Received
24th July 2014
, Accepted 10th September 2014
First published on 10th September 2014
Abstract
Apoptosis is a well-organized mechanism developed by eukaryotic organisms during maturation. The importance of apoptosis as a major form of cellular suicide with a causative or contributing role in a variety of diseases has become progressively obvious. A large body of evidence implicates the association of apoptosis with an arranged series of physical and biochemical alterations comprising the nucleus, cytoplasm and cell membrane. Understanding the process of apoptosis is not only important for maintaining physiological conditions, but also is critical for therapy development. In this review, we briefly outline the various ways to detect apoptosis with distinct assays and molecules, and provide an outlook on the latest imaging techniques for the visualization of phosphatidylserine (PS) externalization, with specific focus on annexin V based targeting of PS and its conjugates. Furthermore, the distinct advantages and disadvantages of each technique are reviewed. Recognition of the major benefits and drawbacks of each assay based on annexin V conjugates would allow us to use the appropriate method to detect apoptosis for instance, in a variety of diseases, such as cancer and will open novel prospects for treatment approaches.
Introduction
Cell death plays crucial roles in a wide variety of diseases such as cancer and myocardial infarction. Cell death occurs through a series of morphologically and biochemically distinct pathways, including apoptosis, necrosis and autophagy.1 The term “apoptosis”2 was initially introduced to describe cell death, which might be outlined by typical morphological options, such as cell shrinkage, chromatin granule condensation, loss of nuclear membrane integrity, semi-permeable membrane blebbing and eventually apoptotic body formation. Although apoptosis can be characterized by cell morphological changes, biochemical events must accompany this process.3 These events include initiator and effector caspase activation,4 cytochrome C release from mitochondria, externalization of phosphatidyl-serine on the plasma membrane, poly (ADP ribose) polymerase (PARP) cleavage and inter-nucleosomal DNA fragmentation.5 These biochemical events are not unique to apoptosis, and not all events occur in all apoptotic cells, in all stages of apoptosis or in response to all apoptosis induction agents.4 The period involving these biochemical processes has been reported to be different depending on several factors, such as the type of cell line or tissue, apoptosis-inducing agent and exposure time. The cascade of events in apoptosis can be subdivided into three stages, including initiation, decision and accomplishment. This complicated process is done after induction of a death receptor (initiation phase) by a set of enzymes called caspases (decision phase). Accomplishment is associated with a variety of alterations in the cells. First, cells change at the mitochondrion level. Subsequently, alterations occur on the outer membrane, such as changes in the membrane mechanical and electrical properties, cell shrinkage, and redistribution of phospholipids between the inner and outer membrane. For example, phosphatidylserine (PS), which are located, on the inner membrane, can be externalized (Fig. 1A). At the subsequent stage, the nuclear membrane is damaged; DNA fragments and chromatin begin to condense. Finally, apoptotic bodies, which contain the remains from the cell, are made. An intact imagination of apoptosis can be achieved by the investigation of various cellular targets and via time-lapse imaging to observe alterations over time. One of the main methods for the detection and isolation of apoptotic cells is through targeting PS moiety.6 The PS externalization7 is reported to be unique to apoptosis and does not occur in the case of necrosis, therefore, several tools have been provided to target PS moieties. Apoptosis visualization may allow both early monitoring of therapy capability and assessment of disease development. To aim that, in the current review, we focus on the exposure of PS on the plasma membrane during the apoptosis process. The successful candidate for the detection of this phenomenon is annexin V. The applications of labeled annexin V proteins as analysis probes8–10 and as in vivo diagnostic tracer to monitor specific diseases have already been reported elsewhere.11–13 In this review, we have compared the methods that have been conducted for this purpose.
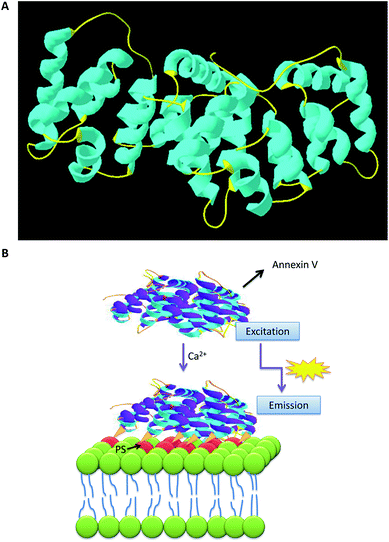 |
| Fig. 1 (A) 3D structure of annexin V depicted with SWISS PDB Viewer. (B) Schematic representation of the biological basis of the annexin V binding assay. In normal cells, phosphatidylserine (PS) is predominantly located at the inner leaflet of the plasma membrane. When cells initiate apoptosis, PS is rapidly translocated to the outer leaflet. In the presence of Ca2+, annexin V binds to PS with high affinity. | |
Molecular structure of annexin and detection of apoptosis
A small protein, annexin V (35 kDa), which binds to PS in the presence of Ca2+,14 is considered one of the most commonly used agents that interact with phospholipid moieties.15,16 In both cases, the recognition agent is coupled to a second part which can be detected (optical detection, radio labeling, magnetic particles for cell sorting, immunodetection and etc.).9,10,17,18 Large numbers of the annexin-superfamily proteins are reported to be expressed in eukaryotes.19 Annexins are widespread, greatly conserved and mostly intracellular proteins that generally are distributed in tissues. Though a set of physiological functions is associated with each individual protein, all annexins share an extremely homologous core domain containing four “annexin” repeats. Each domain has seventy amino acids in length, with an alpha-helical structure (Fig. 1B). The variable N-terminal region is believed to confer practical adaptability to different annexins.20 Annexins have been reported to participate in signal transduction, endocytosis, cytoskeleton organization, cellular proliferation and differentiation.21 Interactions with membranes are the indication of their bioactivity, while, the ability to bind to anionic phospholipids in a Ca2+-dependent manner is considered one of their main biological features.
The labeled annexins can be assayed not only by the basic tools such as fluorescence microscopy22 but also by advanced tools, such as flow cytometry and molecular imaging. In the flow cytometry method, the annexin-labeled apoptotic cells are transported to the optical unit using a fluidics system that can be illuminated by lasers, and then the filtered light signals are sensed by detectors. The suspension cells, such as Jurkat cells (human T-cell leukemia) or U9737 are common for the detection of PS externalization based on annexin V protein. In these cases, the cells can be treated with apoptosis stimuli. After collection and washing, the cells can be resuspended in various annexin binding buffer containing CaCl2 (adequate concentration is 1.5–2.5 mM) as shown in (Fig. 2A). To discern between dead and apoptotic cells, a membrane impermeable DNA stain, such as propidium iodide (PI) can be added simultaneously to the cell suspension containing apoptosis inducing agent.23 Thus, normal, apoptotic and dead cells are evaluated based on a double-labeling for annexin V and PI, which can be further analyzed by flow cytometry. In the pattern of flow cytometry, two major populations of the cells can be observed: the cells that were viable and not undergoing apoptosis (annexin V and PI negative), the cells undergoing apoptosis (annexin V positive and PI negative). A small population of the cells, moreover, may detect as both annexin V and PI positive. These cells are in end-stage of apoptosis or already dead (Fig. 2B).
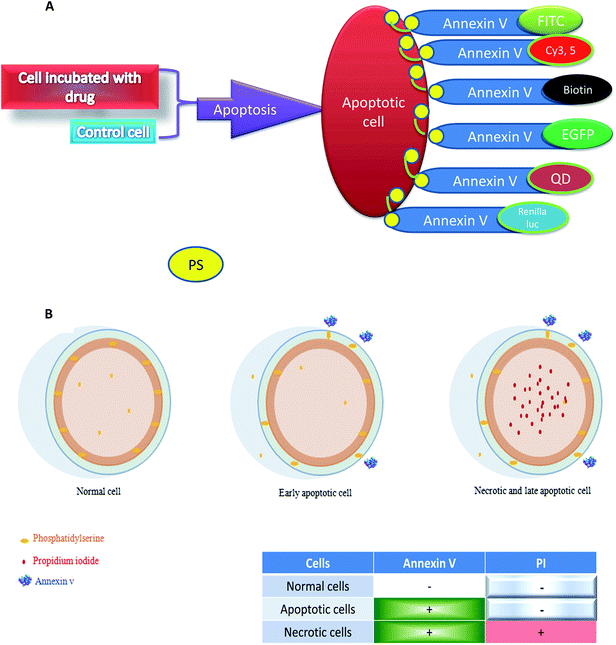 |
| Fig. 2 (A) Schematic representation of the probes, their surface modification and bioconjugation with protein, annexin V. (B) These probe–annexin V conjugates were shown to bind strongly to the induced apoptotic cells compared with normal cells, due to the externalization of phosphatidylserine residues. Diagram showing healthy and apoptotic cells with markers for detection of apoptosis. | |
The annexin-based probes may also be useful for whole animal investigations. In vivo imaging has been used as a noninvasive tool for the detection of apoptosis in small living animals.24 In a fascinating method for in vivo apoptosis assay, the interested cells, such as EL4 and S180 were harvested, washed with PBS, and re-suspended in suitable medium at a concentration of about 107 cells per ml. The tumor cell suspension was implanted to the animal model (six-week old nude/nude mice) by the intravenous or subcutaneous injection.25 Then the apoptosis was induced by irradiation or chemical agents in the tumor. The tumor-bearing model was imaged using annexin V-based probes such as 99mTc–HYNIC–annexin V after treatment. In this step, probes can be injected into animal model through the vein and images can be obtained usually 2 to 6 hours later in a dark imaging chamber, using suitable cameras such as single-head γ camera. The illuminated annexinV-based probes might be monitored by a charge-coupled detector (CCD).25
The above method provides semi-quantitative information for monitoring the effectiveness of the interventions. And in contrast to the cell or tissue culture-based experiments, which usually are assayed by flow cytometry, the studies in intact whole-animals incorporate all of the interacting physiological factors such as neuronal, hormonal, nutritional, and immunological on apoptosis process.
Fluorescein-labeled annexin V
Fluorescein-labeled annexin V was used by Koopman et al. to monitor apoptotic cells by flow cytometry.9 Due to the impenetrable nature of the probe into the phospholipid bilayer, annexin V cannot bind to normal vital cells. However, in dead cells the outer leaflet of the membrane can bind to extrinsically applied annexin V due to loss of the plasma membrane integrity. The amine-reactive fluorescein derivatives have been shown to be the most accepted fluorescent derivatization reagents for covalently labeling proteins. Relatively high absorptivity, great fluorescence quantum yield and good water solubility with an excitation maximum (494 nm) that closely matches the 488 nm spectral line of the argon-ion laser makes the fluorescein an important fluorophore for confocal laser-scanning microscopy26 and flow cytometry applications.9,27 Furthermore, fluorescent protein conjugates are not extraordinarily prone to precipitation; therefore they can be prepared in high purity for further applications. Even though the fluorescent dye has been labeled to a variety of biomolecules for many years, fluorescein-based dyes and their conjugates represent numerous distinct disadvantages, such as: (a) a comparatively high degree of photobleaching28 (b) pH-sensitive fluorescence intensity29 (pKa ∼ 6.4) that can be apparently decreased below pH 7, (c) a relatively wide fluorescence emission spectrum that may limit their usage in some multicolor experiments30,31 and (d) A tendency toward quenching of their fluorescence on conjugation to biopolymers, particularly at high rates of labeling.30,31 Moreover, the coupling reaction is difficult to be controlled precisely; therefore, the final product may be a heterogeneous mixture of differentially various labeled protein molecules; therefore, these drawbacks have reinforced scientists to develop an alternative assay.
EGFP-labeled annexin V
To overcome some limitation of the fluorescent labels, such as heterogeneity of coupling process, Stöcker et al., generated more homogenous population by fusing annexin V to enhanced green fluorescent protein (EGFP), which represents to be more photostable compared to FITC.32 The sufficient and reproducible expressions of annexin V–EGFP fusion proteins in eukaryotic cells suggest an explicit approach for the efficient generation of this diagnostically compatible fusion protein. The secretory expression of annexin V–EGFP fusion proteins can be a constructive alternative in comparison with the usual production processes. The structural homogeneity of the product compared to FITC-conjugates, and brighter emission with higher photostability are other advantages of annexin V–EGFP. This might be valuable in imaging applications when vivid microscopic pictures and where greater exposure times to incident light are required. The fluorescent proteins typically can be expressed from strong promoters. Furthermore, the novel applications of annexin V-based labeling can be practical that include in vivo transfection of annexin V–EGFP plasmids for detection of apoptosis and necrosis in situ in development or in disease processes.32 Although EGFP-labeled annexin V is an efficient probe for the detection of apoptosis, the annexin V–EGFP is naturally insoluble32 and its production in eukaryotic systems might be a limiting step to produce in large scale.
Europium-labeled annexin V
Europium is a chemical element with the symbol Eu and atomic number 63 Fig. 3A. It is named after the continent of Europe. It is a quite hard, silvery metal which easily oxidizes in air and water. Being a typical member of the lanthanide series, europium usually assumes the oxidation state +3, but the oxidation state +2 is also accepted and all europium compounds with oxidation state +2 represent slightly reducing effects.11 Europium has no significant biological role and is almost non-toxic in comparison with other heavy metals. Most applications of europium use the phosphorescence of europium compounds. Europium labeled annexin V was used by Engbers-Buijtenhuijs et al.,17 as a sensitive tool, for the detection of apoptosis to analyze anoikis in adherent cell cultures using the principles of the Dissociation Enhanced Lanthanide Fluoro Immuno Assay (DELFIA®33 Wallac Oy, Turku, Finland). DELFIA® assays use a lanthanide metal (europium) chelate label, which is practically non-fluorescent, binding of europium-labeled annexin V to PS results in the dissociation of Europium from the labeled compound that is caused by the low pH of the commercially available enhancement solution. The free europium ion rapidly forms a new highly fluorescent and stable chelate with the components of enhancement solution. A schematic depiction of the DELFIA® assay is shown in Fig. 3B.
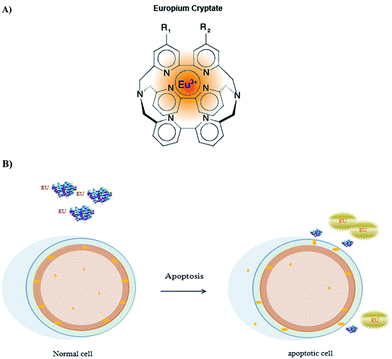 |
| Fig. 3 Europium-labeled annexin V, (A) europium structure and (B) chemical process indicating binding of europium to annexin V. | |
Radio-labeled annexin V
It has been demonstrated that several radio-chemically designed annexin V probes are being developed to target apoptotic cells, non-invasively.12,34,35 The halogen radioisotope 123I has been reported to be used for labeling of annexin V. Although 123I–annexin V represents good and specific regional imaging application, for instance, in the abdominal area, it has some limitations, such as high production cost and a complicated labeling method. 123I–annexin V, moreover, is subject to quick in vivo dehalogenation.36,37 Another radionuclide for the labeling of annexin V is technetium-99m (99mTc) with decay properties and acceptable cost that can be effectively applied for single-photon imaging. However, 99mTc cannot be tagged directly to annexin V and needs accurate and specific conjugation principles. Various 99mTc-labeling chelators have been demonstrated such as n-1-imino-4-mercaptobutyl (imino),38 ethylenedicysteine (EC),39,40 4,5-bis(thioacetamido)pentanoyl (BTAP),41,42 and hydrazinonicotinamido (HYNIC).42–44 99mTc–BTAP–annexin V has been used to observe chemosensitivity in a variety of cancer cells, such as lung cancer, lymphoma and breast cancer. However slow blood clearance, nonspecific uptake, prolonged and complex formation method can hinder its usage in clinical applications.42,45 99mTc–EC–annexin V is also considered as an alternative agent for apoptosis imaging, while in comparison with 99mTc–HYNIC–annexin V and 99mTc–BTAP–annexin V, there are a few studies indicating the use of 99mTc–EC–annexin V in clinical practice. 99mTc–HYNIC–annexin V has been demonstrated to be the most widely used derivative and an appropriate tracer for clinical use that can be applied to monitor apoptosis by single photon emission computed tomography (SPECT).46–48 Although most radiopharmaceuticals are described for SPECT, positron emission tomography (PET) represents several advantages compared to SPECT, such as better resolution, greater sensitivity and exact evaluation. Nevertheless, high cost of production and use of the cyclotron limits its further application. 18F–annexin V and 68Ga–annexin V have been examined in the preclinical area showing appropriate half-life for diagnostic imaging. Unfortunately, there are no common clinical trials indicating the use of PET radionuclides to date, but are likely to become noticeable soon.49 Novel imaging tools including SPECT/CT, PET/CT and PET/MRI that can combine molecular and anatomic imaging are considered the best alternatives for the use in clinical studies.50–52
Quantum dots-labeled annexin V
Quantum dots (QDs) with high photostability and extreme brightness, are a new class of probes that are widely used in single-molecule imaging53 and single virus tracking.54 QDs can be described as inorganic semiconductor nanocrystals, generally containing a cadmium selenide (CdSe) core and a zinc sulphide (ZnS) shell with excitons restricted in all three structural dimensions, generating the typical fluorescent properties. For biological applications, QDs can be coated with a passivating layer to enhance solubility, and can be tagged to targeting biomolecules, such as antibodies or streptavidin (Fig. 4A). As fluorescent probes, QDs are identified by wide absorption profiles, high extinction coefficients and spectrally tunable emission profiles. Small CdSe QD cores (2.3 nm diameter) emit blue light, while the larger crystals (5.5 nm diameter) emit red light, generating size-dependent optical properties55 (Fig. 4B). van den Berg et al., for the first time, stained apoptotic cells with annexin V-functionalized QDs.56 Two approaches were concomitantly investigated: (a) QDs were first functionalized with annexin V and then were subsequently added to apoptotic cells, or (b) apoptotic cells were pre-incubated with annexin V, and QDs were added after binding annexin V to PS moieties. It has been reported that both methods could give comparable results, similar to those of conventional staining techniques (organic dyes) indicating the high efficiency of QDs functionalized with annexin V to stain apoptotic cells.56 van Tilborg et al., have reported a new probe based on the annexin V conjugated QDs as apoptosis detection agents to monitor anticancer drugs using a fabricated microfluidic device. The microfluidic technique has been reported to decrease annexin V–QDs diffusion time and to make a connection between the QDs rated in vitro cell imaging and the analysis of the apoptotic cascade in the microfluidic device. This method has been demonstrated to be applied in several biological systems, including real-time observing of apoptosis cascade and analysis of anticancer treatments.57 Moreover, the method can examine the dose-dependent effect of several anticancer drugs on the cells and can reduce the incubation time for the agent to sense apoptotic cells due to decreased reaction environment inside the device. It also detects the dynamic behavior, at the single level, for redistribution of PS from the inner plasma membrane. The early execution stage of apoptosis presented as cell shrinkage imaging has been addressed to be monitored more apparently by QD–AV than by QD–FITC methods. The necrosis processes and the reactivity of QD–AV were reported to be preferable to the other commercial annexin V–FITC apoptosis detection methods.
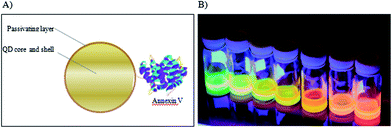 |
| Fig. 4 (A) Schematic representation of the CdSe/ZnS QDs, their surface modification and bioconjugation with protein, annexin V. (B) Quantum dots, based on the size, emit light at different frequencies. | |
Renilla luciferase-labeled annexin V
We have recently cloned and expressed a new annexin-based probe based on Renilla luciferase (RLuc) for monitoring the apoptosis induction using anti-cancer drugs.58,59 This probe has been reported to be a fusion protein that was expressed in a prokaryotic system using E. coli BL21 (DE3), and thus, easy to produce. The system is based on luminescence process with the possibility to be produced in eukaryotic cells while, it does not represent the problems associated with fluorescent dyes; such as photo-bleaching and susceptibility to pH. Although luciferases are less appropriate for cellular visualization, they can be applied as agents for transcriptional regulation at much lower concentrations in comparison with fluorescent tracers. It also has no problem to produce in an eukaryotic system that EGFP–annexin has. The sensitivity of bioluminescence also provides an important advantage compared to fluorescent proteins. The apoptosis assay using the RLuc/annexin V probe is carried out using a luminometer. Although RLuc/annexin V probe is an advanced probe both for suspended and adherent cells, its potential to assay apoptosis in vivo remains to be answered.
Nanoparticles-based bioprobes conjugated to annexin V
To visual contrast agent at nano- to picomolar concentrations, nuclear and optical methods have been developed. The optical imaging techniques are restricted by tissue infiltration, while nuclear techniques represent a low structural resolution image in contrast to a weak anatomical background. Magnetic resonance imaging (MRI) shows the non-invasive detection of magnetic resonance (MR) contrast tracer ranging from micro- to millimolar in concentration with adequate structural resolution anatomical images across the whole living animal. Additional labeling of MRI contrast tracer using florescent agents can result in detection of co-localization in MR images that determined at the macroscopic and cellular levels by optical techniques. Two different types of bimodal contrast probes have been reported by van Tilberg et al., for monitoring apoptotic cells using both MRI and optical methods. MR contrast was prepared using entrapment of iron oxide particles within PEGylated micelles or by fusion of Gd–DTPA–bis(stearylamide) (Gd–DTPA–BSA) lipids within the lipid bilayer of PEGylated liposomes. Iron oxide-based nanoparticles have been provided to enable molecular MRI of lipoproteins60,61 or adhesion molecule expression.62 High affinity of these probes for PS18 allowed low doses of the probes to be applied for visualization of apoptosis, both in vitro63 and in vivo,64 thus minimizing the potential for nonspecific uptake of these probes. PEGylated liposomes have been successfully applied as drug carriers, especially in tumor therapy (Fig. 5).42,65,66 Incorporation of anti-apoptotic drugs with annexin V-conjugated paramagnetic liposomes would allow for the association of multimodal detection of apoptotic cells and anti apoptotic therapy. These agents have been demonstrated to target the extravascular space in tissue showing enhanced permeability.67 They can be organized on the outer leaflet of the cell membranes and have been reported to be appropriate for in vivo MR detection of early apoptotic cell death, contributing to the diagnostic process of various diseases, including acute myocardial infarction and cancer.68 Finally, with these systems; the diagnostics could be developed into specified therapeutic applications with additional integration of drugs.67 Other fluorescent lipids have also been reported to be integrated in the contrast agent lipid bilayer for parallel visualization by optical methods.
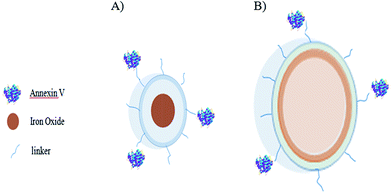 |
| Fig. 5 Annexin V-modified superparamagnetic micelles (left panel) and annexin V-modified paramagnetic liposomes (right panel). | |
Conclusion
The approaches and techniques for apoptosis monitoring are altering quite rapidly; thus, there are several issues that must be considered. Up to now, different cell events during programmed cell death have been targeted for apoptosis assay. Detection of loss of cell viability, DNA fragmentation and DNA condensation are conventional laboratory methods to study the apoptosis. These cellular events occur late in apoptosis and their assessment is time-consuming, and end-pointed.27,69–71 The study of most early events in apoptosis, such as the expression of thrombospondin binding sites,72 loss of sialic acid residues,73 and apoptosome formation74,75 has some technical difficulties. Therefore, it is complicated to make decision about which set of tracers or imaging procedures will be appropriate for distinct applications. It appears those molecules that bind to the surface of the apoptotic cells, such as annexin V; represent the advantage regarding sensitivity and specificity compared to metabolically directed tracers.76–78 As already discussed, the annexin V assay is considered one of the most sensitive methods which is simple and easily applicable for detection of apoptosis.79 Better understanding of various biochemical aspects of apoptosis may provide chances to design novel classes of imaging agents that may compete with existing ones.80 There are several reports showing the use of fluorescein labeled annexin V as an adequate research probe for in vitro studies, while Renilla luciferase-labeled annexin V may become popular, especially in high throughput screening assays. On the other hand, nanoparticles-based bioprobes conjugated to annexin V have been described to be used as a bifunctional tracer in MRI and fluorescence imaging, raising the possibility of applying these probes for accurate imaging, especially in vivo, while, QDs conjugated to annexin V have been suggested for high sensitivity and specificity detection (Table 1). Further progresses of apoptotic cell specificity and development of agent pharmacokinetics to obtain distinct targeting are required to promote clinical usages and wide application of apoptosis probes.36,80 The ability to visualize PS expression by annexin V in a variety of diseases such as cancer and myocardial infarction has been reported. The use of annexin V and its conjugates can be further extended for the therapeutic decision making in apoptosis-related diseases and interventions.79,80
Table 1 Characteristics of annexin-based probes for apoptosis detection
|
Probe based |
Coupling method |
Applications |
Fluorescein-labeled annexin V |
Fluorescein dye |
Chemical |
In vitro assay |
Quantum dots–annexin V |
Quantum dot nano particles (CdSe core and ZnS shell) |
Chemical |
High sensitivity and high specificity |
EGFP-labeled annexin V |
EGFP protein |
Produced in eukaryotic expression system |
In vitro assay (it is more photo-stable and brighter than dye based probes) |
Radio-labeled annexin V |
Radionucleotide such as 99mTc |
Chemical |
In vivo imaging |
Renilla luciferase-labeled annexin V |
Renilla luciferase protein |
Produced in prokaryotic expression system |
High throughput assay |
Nanoparticle based-annexin V |
Nanoparticles such as liposome and magnetic |
Chemical |
High sensitivity and specificity – in vivo imaging |
Beside the above advantages, there are several disadvantages that must be considered for annexin V-based probes. The assays based on these probes may require the use of expensive equipments such as the flow cytometry and the in vivo imaging devices.56 The preparation of some mentioned probes has laboratory difficulties, chemical modification of annexin V needs different manipulations of the protein and results in a heterogeneous mixture of labelled proteins. Moreover, it was shown that FITC–annexin quenches by 40–50% upon binding phosphatidylserine.81 More importantly, annexin-based probes are protein-based and sensitive against inactivating factors, such as proteases and temperature.
Taken together, the annexin V-based assay seems to be a valuable, sensitive and functional technique, opening novel prospects to treatment approaches since it can be used in vitro, ex vivo and in vivo. Finally, it may be predictable that the capabilities for the application of annexin V conjugates in analysis probes, diagnostic tools, treatment assessment, and clinical approaches in the future can be wide.
References
- G. Kroemer, W. El-Deiry, P. Golstein, M. Peter, D. Vaux, P. Vandenabeele, B. Zhivotovsky, M. Blagosklonny, W. Malorni and R. Knight, Cell Death Differ., 2005, 12, 1463–1467 CrossRef CAS PubMed.
- J. F. Kerr, A. H. Wyllie and A. R. Currie, Br. J. Cancer, 1972, 26, 239 CrossRef CAS.
- J. D. Ly, D. R. Grubb and A. Lawen, Apoptosis, 2003, 8, 115–128 CrossRef CAS.
- S. L. Fink and B. T. Cookson, Infect. Immun., 2005, 73, 1907–1916 CrossRef CAS PubMed.
- Z. Herceg and Z.-Q. Wang, Mutat. Res., 2001, 477, 97–110 CrossRef CAS.
- M. Van Engeland, L. J. Nieland, F. C. Ramaekers, B. Schutte and C. P. Reutelingsperger, Cytometry, 1998, 31, 1–9 CrossRef CAS.
- S. Martin, C. Reutelingsperger, A. J. McGahon, J. A. Rader, R. Van Schie, D. M. LaFace and D. R. Green, J. Exp. Med., 1995, 182, 1545–1556 CrossRef CAS.
- J. Dachary-Prigent, J.-M. Freyssinet, J.-M. Pasquet, J.-C. Carron and A. T. Nurden, Blood, 1993, 81, 2554–2565 CAS.
- G. Koopman, C. Reutelingsperger, G. Kuijten, R. Keehnen, S. Pals and M. Van Oers, Blood, 1994, 84, 1415–1420 CAS.
- G. A. van Tilborg, W. J. Mulder, P. T. Chin, G. Storm, C. P. Reutelingsperger, K. Nicolay and G. J. Strijkers, Bioconjugate Chem., 2006, 17, 865–868 CrossRef CAS PubMed.
- G. Baysinger, National Institute of Standards and Technology, 2014.
- F. G. Blankenberg, P. D. Katsikis, J. F. Tait, R. E. Davis, L. Naumovski, K. Ohtsuki, S. Kopiwoda, M. J. Abrams, M. Darkes and R. C. Robbins, Proc. Natl. Acad. Sci. U. S. A., 1998, 95, 6349–6354 CrossRef CAS.
- P. W. Thimister, L. Hofstra, H. Liem, H. H. Boersma, G. Kemerink, C. P. Reutelingsperger and G. A. Heidendal, J. Nucl. Med., 2003, 44, 391–396 Search PubMed.
- H. Andree, C. Reutelingsperger, R. Hauptmann, H. C. Hemker, W. T. Hermens and G. Willems, J. Biol. Chem., 1990, 265, 4923–4928 CAS.
- R. G. Hanshaw, C. Lakshmi, T. N. Lambert, J. R. Johnson and B. D. Smith, ChemBioChem, 2005, 6, 2214–2220 CrossRef CAS PubMed.
- E. Kimura, S. Aoki, E. Kikuta and T. Koike, Proc. Natl. Acad. Sci. U. S. A., 2003, 100, 3731–3736 CrossRef CAS PubMed.
- P. Engbers-Buijtenhuijs, M. Kamphuis, G. van der Sluijs Veer, C. Haanen, A. Poot, J. Feijen and I. Vermes, Apoptosis, 2005, 10, 429–437 CrossRef CAS PubMed.
- G. P. Wang, E. Q. Song, H. Y. Xie, Z. L. Zhang, Z. Q. Tian, C. Zuo, D. W. Pang, D. C. Wu and Y. B. Shi, Chem. Commun., 2005, 4276–4278 RSC.
- S. E. Moss and R. O. Morgan, Genome Biol., 2004, 5, 219 CrossRef PubMed.
- P. Raynal and H. B. Pollard, Biochim. Biophys. Acta, 1994, 1197, 63–93 CrossRef CAS.
- V. Gerke, C. E. Creutz and S. E. Moss, Nat. Rev. Mol. Cell Biol., 2005, 6, 449–461 CrossRef CAS PubMed.
- E. Szliszka, Z. P. Czuba, Ł. Sędek, A. Paradysz and W. Król, Pharmacol. Rep., 2011, 63, 139–148 CrossRef CAS.
- A. M. Rieger, K. L. Nelson, J. D. Konowalchuk and D. R. Barreda, J. Visualized Exp., 2011, 24, 2597 Search PubMed.
- B. A. Smith, B. W. Xie, E. R. van Beek, I. Que, V. Blankevoort, S. Xiao, E. L. Cole, M. Hoehn, E. L. Kaijzel and C. W. Löwik, ACS Chem. Neurosci., 2012, 3, 530–537 CrossRef CAS PubMed.
- M. f. Guo, Y. Zhao, R. Tian, L. Li, L. Guo, F. Xu, Y. m. Liu, Y. b. He, S. Bai and J. Wang, J. Exp. Clin. Cancer Res., 2009, 28, 136–145 CrossRef PubMed.
- S. Wells and I. Johnson, Three-Dimensional Confocal Microscopy: Volume Investigation of Biological Specimens, 1994, pp. 101–129 Search PubMed.
- I. Vermes, C. Haanen, H. Steffens-Nakken and C. Reutellingsperger, J. Immunol. Methods, 1995, 184, 39–51 CrossRef CAS.
- L. Song, E. Hennink, I. T. Young and H. J. Tanke, Biophys. J., 1995, 68, 2588–2600 CrossRef CAS.
- R. Sjöback, J. Nygren and M. Kubista, Spectrochim. Acta, Part A, 1995, 51, L7–L21 CrossRef.
- G. Der-Balian, N. Kameda and G. Rowley, Anal. Biochem., 1988, 173, 59–63 CrossRef CAS.
- R. Zuk, G. Rowley and E. Ullman, Clin. Chem., 1979, 25, 1554–1560 CAS.
- M. Stöcker, A. Pardo, C. Hetzel, C. Reutelingsperger, R. Fischer and S. Barth, Protein Expression Purif., 2008, 58, 325–331 CrossRef PubMed.
- I. Hemmilä, Clin. Chem., 1985, 31, 359–370 Search PubMed.
- F. G. Blankenberg, P. D. Katsikis, J. F. Tait, R. E. Davis, L. Naumovski, K. Ohtsuki, S. Kopiwoda, M. J. Abrams and H. Strauss, J. Nucl. Med., 1999, 40, 184–191 CAS.
- K. Ohtsuki, K. Akashi, Y. Aoka, F. G. Blankenberg, S. Kopiwoda, J. F. Tait and H. W. Strauss, Eur. J. Nucl. Med., 1999, 26, 1251–1258 CrossRef CAS.
- H. H. Boersma, B. L. Kietselaer, L. M. Stolk, A. Bennaghmouch, L. Hofstra, J. Narula, G. A. Heidendal and C. P. Reutelingsperger, J. Nucl. Med., 2005, 46, 2035–2050 CAS.
- C. Lahorte, C. Van De Wiele, K. Bacher, B. Van Den Bossche, H. Thierens, S. Van Belle, G. Slegers and R. Dierckx, Nucl. Med. Commun., 2003, 24, 871–880 CAS.
- G. J. Kemerink, H. Liem, L. Hofstra, H. H. Boersma, W. C. Buijs, C. P. Reutelingsperger and G. A. Heidendal, J. Nucl. Med., 2001, 42, 382–387 CAS.
- H. Kurihara, D. J. Yang, M. Cristofanilli, W. D. Erwin, D.-F. Yu, S. Kohanim, R. Mendez and E. E. Kim, Appl. Radiat. Isot., 2008, 66, 1175–1182 CrossRef CAS PubMed.
- D. J. Yang, A. Azhdarinia, P. Wu, D.-F. Yu, W. Tansey, S. K. Kalimi, E. E. Kim and D. A. Podoloff, Cancer Biother. Radiopharm., 2001, 16, 73–83 CrossRef CAS PubMed.
- H. Boersma, I. Liem, G. Kemerink, P. Thimister, L. Hofstra, L. Stolk, W. van Heerde, M. W. Pakbiers, D. Janssen and A. Beysens, Br. J. Radiol., 2014, 76, 553–560 CrossRef PubMed.
- G. J. Kemerink, H. H. Boersma, P. W. Thimister, L. Hofstra, M.-T. W. Pakbiers, D. Janssen, C. P. Reutelingsperger and G. A. Heidendal, Eur. J. Nucl. Med, 2001, 28, 1373–1378 CrossRef CAS PubMed.
- N. Tokita, S. Hasegawa, K. Maruyama, T. Izumi, F. G. Blankenberg, J. F. Tait, W. H. Strauss and T. Nishimura, Eur. J. Nucl. Med. Mol. Imaging, 2003, 30, 232–238 CrossRef CAS PubMed.
- C. Van de Wiele, C. Lahorte, H. Vermeersch, D. Loose, K. Mervillie, N. D. Steinmetz, J.-L. Vanderheyden, C. A. Cuvelier, G. Slegers and R. A. Dierck, J. Clin. Oncol., 2003, 21, 3483–3487 CrossRef PubMed.
- C. van De Wiele, H. Vermeersch, D. Loose, A. Signore, N. Mertens and R. Dierckx, Cancer Biother. Radiopharm., 2004, 19, 189–194 CrossRef CAS PubMed.
- M. Kartachova, N. van Zandwijk, S. Burgers, H. van Tinteren, M. Verheij and R. A. V. Olmos, J. Clin. Oncol., 2007, 25, 2534–2539 CrossRef CAS PubMed.
- S. Rottey, D. Loose, L. Vakaet, C. Lahorte, H. Vermeersch, S. Van Belle and C. Van De Wiele, Q. J. Nucl. Med. Mol. Imaging, 2007, 51, 182–188 CAS.
- S. Rottey, B. van Den Bossche, G. Slegers, S. van Belle and C. van de Wiele, Q. J. Nucl. Med. Mol. Imaging, 2009, 53, 127–132 CAS.
- C. Vangestel, M. Peeters, G. Mees, R. Oltenfreiter, H. H. Boersma, P. H. Elsinga, C. Reutelingsperger, N. Van Damme, B. De Spiegeleer and C. Van de Wiele, Mol. Imaging, 2011, 10, 340–358 CAS.
- M. S. Kartachova, R. A. V. Olmos, R. L. Haas, F. J. Hoebers, M. W. Van den Brekel, N. van Zandwijk, M. Van Herk and M. Verheij, Eur. J. Nucl. Med. Mol. Imaging, 2006, 33, 893–899 CrossRef CAS PubMed.
- K. Schutters and C. Reutelingsperger, Apoptosis, 2010, 15, 1072–1082 CrossRef CAS PubMed.
- M. Verheij, Cancer Metastasis Rev., 2008, 27, 471–480 CrossRef PubMed.
- P. Zrazhevskiy and X. Gao, Nat. Commun., 2013, 4, 1619 CrossRef PubMed.
- K. I. Joo, Y. Fang, Y. Liu, L. Xiao, Z. Gu, A. Tai, C. L. Lee, Y. Tang and P. Wang, ACS Nano, 2011, 5, 3523–3535 CrossRef CAS PubMed.
- R. C. Somers, M. G. Bawendi and D. G. Nocera, Chem. Soc. Rev., 2007, 36, 579–591 RSC.
- S. Le Gac, I. Vermes and A. van den Berg, Nano Lett., 2006, 6, 1863–1869 CrossRef CAS PubMed.
- L. Zhao, P. Cheng, J. Li, Y. Zhang, M. Gu, J. Liu, J. Zhang and J. J. Zhu, Anal. Chem., 2009, 81, 7075–7080 CrossRef CAS PubMed.
- R. Emamzadeh, M. Nazari and S. Najafzadeh, Anal. Methods, 2014, 6, 4199–4204 RSC.
- M. Nazari, R. Emamzadeh, S. Hosseinkhani, L. Cevenini, E. Michelini and A. Roda, Analyst, 2012, 137, 5062–5070 RSC.
- D. P. Cormode, T. Skajaa, M. M. van Schooneveld, R. Koole, P. Jarzyna, M. E. Lobatto, C. Calcagno, A. Barazza, R. E. Gordon and P. Zanzonico, Nano Lett., 2008, 8, 3715–3723 CrossRef CAS PubMed.
- G. A. van Tilborg, E. Vucic, G. J. Strijkers, D. P. Cormode, V. Mani, T. Skajaa, C. P. Reutelingsperger, Z. A. Fayad, W. J. Mulder and K. Nicolay, Bioconjugate Chem., 2010, 21, 1794–1803 CrossRef CAS PubMed.
- M. Nahrendorf, F. A. Jaffer, K. A. Kelly, D. E. Sosnovik, E. Aikawa, P. Libby and R. Weissleder, Circulation, 2006, 114, 1504–1511 CrossRef CAS PubMed.
- E. A. Schellenberger, A. Bogdanov Jr, D. Hogemann, J. Tait, R. Weissleder and L. Josephson, Mol. Imaging, 2002, 1, 102–107 CrossRef CAS.
- M. Zhao, D. A. Beauregard, L. Loizou, B. Davletov and K. M. Brindle, Nat. Med., 2001, 7, 1241–1244 CrossRef CAS PubMed.
- K. I. Joo, L. Xiao, S. Liu, Y. Liu, C. L. Lee, P. S. Conti, M. K. Wong, Z. Li and P. Wang, Biomaterials, 2013, 34, 3098–3109 CrossRef CAS PubMed.
- Y. Liu, J. Fang, Y.-J. Kim, M. K. Wong and P. Wang, Mol. Pharmaceutics, 2014, 11, 1651–1661 CrossRef CAS PubMed.
- G. A. van Tilborg, W. J. Mulder, N. Deckers, G. Storm, C. P. Reutelingsperger, G. J. Strijkers and K. Nicolay, Bioconjugate Chem., 2006, 17, 741–749 CrossRef CAS PubMed.
- K. Hiller, C. Waller, M. Nahrendorf, W. R. Bauer and P. M. Jakob, Mol. Imaging, 2006, 5, 115–121 Search PubMed.
- A. Facchinetti, L. Tessarollo, M. Mazzocchi, R. Kingston, D. Collavo and G. Biasi, J. Immunol. Methods, 1991, 136, 125–131 CrossRef CAS.
- C. Sarraf and I. Bowen, Cell Proliferation, 1988, 21, 45–49 CrossRef CAS PubMed.
- W. G. Telford, L. E. King and P. J. Fraker, Cytometry, 1992, 13, 137–143 CrossRef CAS PubMed.
- A. Krispin, Y. Bledi, M. Atallah, U. Trahtemberg, I. Verbovetski, E. Nahari, O. Zelig, M. Linial and D. Mevorach, Blood, 2006, 108, 3580–3589 CrossRef CAS PubMed.
- J. Savill, V. Fadok, P. Henson and C. Haslett, Immunol. Today, 1993, 14, 131–136 CrossRef CAS.
- M. Torkzadeh-Mahani, F. Ataei, M. Nikkhah and S. Hosseinkhani, Biosens. Bioelectron., 2012, 38, 362–368 CrossRef CAS PubMed.
- R. Cheraghi, S. Hosseinkhani, J. Davoodi, M. Nazari, Z. Amini-Bayat, H. Karimi, M. Shamseddin and F. Gheidari, Int. J. Biol. Macromol., 2013, 58, 336–342 CrossRef CAS PubMed.
- F. G. Blankenberg, J. Nucl. Med., 2008, 49, 81S–95S CrossRef CAS PubMed.
- U. Fischer and K. Schulze-Osthoff, Pharmacol. Rev., 2005, 57, 187–215 CrossRef CAS PubMed.
- T. Yang, A. Haimovitz-Friedman and M. Verheij, Exp. Oncol., 2012, 34, 269–276 CAS.
- W. L. van Heerde, S. Robert-Offerman, E. Dumont, L. Hofstra, P. A. Doevendans, J. F. Smits, M. J. Daemen and C. P. Reutelingsperger, Cardiovasc. Res., 2000, 45, 549–559 CrossRef CAS.
- G. Niu and X. Chen, J. Nucl. Med., 2010, 51, 1659–1662 CrossRef CAS PubMed.
- J. D. Ernst, L. Yang, J. L. Rosales and V. C. Broaddus, Anal. Biochem., 1998, 260, 18–23 CrossRef CAS PubMed.
|
This journal is © The Royal Society of Chemistry 2014 |