DOI:
10.1039/C4RA04759A
(Paper)
RSC Adv., 2014,
4, 38119-38123
Pyrene-based BODIPY: synthesis, photophysics and lasing properties under UV-pumping radiation†
Received
20th May 2014
, Accepted 4th August 2014
First published on 4th August 2014
Abstract
The synthesis, photophysical and laser properties of a pyrene-based tetramethyl difluoroboron-dipyrromethane (PYBDP) are reported in the present paper. The PYBDP presents a higher fluorescence quantum yield and Stokes shift than other phenyl-substituted laser dyes in the green region. Under transversal pumping conditions, the new dye exhibits highly efficient and stable amplified spontaneous emissions centering at 531 nm. Moreover, the new dye presents a maximum narrow band ASE lasing efficiency of 10.86% with a wide tunable range (525 nm to 560 nm) on excitation with a Q-switched Nd:YAG (355 nm) laser in toluene.
Introduction
Although dye molecules dissolved in liquid solvents are the most popular scheme for organic lasers, the relationships between structure–property and lasing performance are still not known.1,2 Amplified spontaneous emission (ASE) experiments, the easiest way to evaluate a potential material for use as an active laser medium, has been widely studied in the last several years.3 When pump light is guided into the active media, the spectral narrowing is normally accompanied by a large enhancement of the output intensity and accounts for the presence of gain due to stimulated emission.4,5 The ASE experiments are useful for organic chemists searching for appropriate laser dyes by developing new fluorophores and modifying the structures of some conventional ones.6–13
Among the various laser materials, the dyes based on difluoroboron dipyrromethane (known as BODIPY) have emerged as good candidates for tunable lasers because of their excellent photophysical properties such as high fluorescence quantum yields, large molar absorption coefficients, low intersystem crossing rates, good photostability, and efficient laser emissions in organic matrices.14–19 In particular, BODIPY dyes show better conversion efficiency and photostability than those of xanthenes or other dyes, and are thus considered to be standard laser dyes in the green-yellow region.20 Moreover, benefiting from recent developments in the BODIPY chemistry, their laser performance and photophysical properties can be finely tuned by introducing substituents at appropriate positions.21–28
As part of our interest in developing highly efficient laser materials, we have reported a group of multi-branched TPA-BODIPY derivatives and studied their lasing properties in different solutions.29 In that process, we introduced pyrenyl group at the meso position in BODIPY and carried out a more comprehensive photophysical study of this PYBDP compound. Under demanding transversal pumping at 355 nm in toluene, the highly fluorescent PYBDP dye exhibits efficient and stable amplified spontaneous emissions in the green region.
Results and discussion
Synthesis
The desired pyrene-based tetramethyl difluoroboron-dipyrromethane (PYBDP) was synthesized through the bicondensation of 1-pyrenecarboxaldehyde and 2,4-dimethylpyrrole in the presence of trifluoroacetic acid as catalyst, followed by oxidation with p-chloranil. The boron difluoride bridge was introduced by treatment with BF3·Et2O in the presence of triethylamine (Scheme 1) and the resultant PYBDP was well characterized using various spectroscopic techniques.
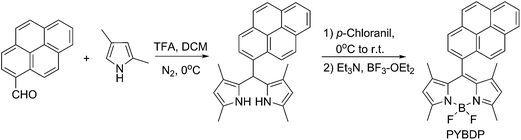 |
| Scheme 1 Synthesis of laser dye PYBDP. | |
Photophysical studies
The PYBDP dyes are readily soluble in common solvents such as hexane, toluene, ether, THF, ethyl acetate, chloroform, dichloromethane, acetone and ethanol. The UV-Vis absorption spectrum of this dye, depicted in Fig. 1a, is characterized by a strong (εmax = 3.7 × 104 M−1 cm−1) band in the visible region, showing the typical F-BODIPY features with a very strong S0–S1 (π–π*) transition centered at 502 ± 2 nm. The shoulder peak at about 470 nm is attributed to the S0–S1 vibrational transition. The higher energy absorption peak, much weaker and broader at 350–400 nm, is assigned to the S0–S2 (π–π*) transition.30 The bands below 350 nm are assigned to different S0–Sn electronic transitions of the pyrene chromophore with their typical and clear vibrational structure (Fig. S1, ESI†). The fact that the absorption peaks of PYBDP show no particular trend as a function of solvent polarity suggests that the excited state of the dyes is immune to surrounding dipolar variations.
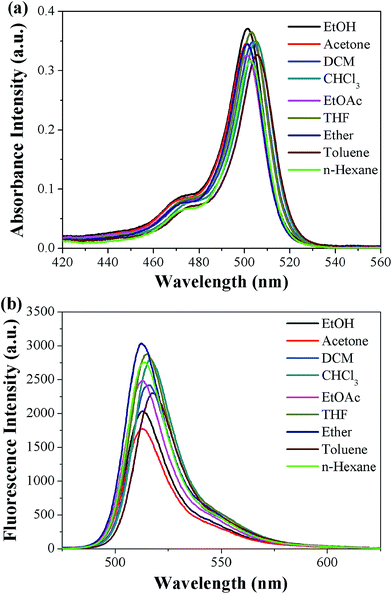 |
| Fig. 1 Overlapped (a) absorbance and (b) fluorescence spectra of PYBDP (10 μM) in different solvents. | |
The fluorescence emission behaviors of PYBDP were investigated and are shown in Fig. 1b. When the S0–S1 absorption band of the BODIPY chromophore is directly excited at 480 nm, the typical fluorescence emission appears centered at 515 ± 2 nm, which corresponds to the BODIPY entity. The Stokes shift for PYBDP is small (about 450 ± 5 cm−1), which is nearly equivalent in different solvents. The fluorescence quantum yields of PYBDP in medium and nonpolar solvents are considerably high (ΦF > 0.75), but the fluorescence quantum yields for PYBDP decrease upon changing the solvent from dichloromethane to acetone (ΦF < 0.6) (Table 1). The fact that the excitation spectra perfectly match the absorption spectra implies that the emitted light originates from the singlet excited state without any contribution of a charge transfer state.31
Table 1 Photophysical properties of PYBDP in different solvents
Solvent |
ET(30) |
λabs [nm] |
εmaxa |
λem [nm] |
Δυstb [cm−1] |
FWHM [nm] |
ΦFc |
εmax × ΦF |
Molar extinction coefficient (εmax, 104 M−1 cm−1). Stokes shifts Δνst = 1/λabs − 1/λem. Relative fluorescence quantum yields (ΦF, ±0.05) with fluorescein (ΦF = 0.95 in 0.1 M NaOH) as standards. |
Hexane |
31.0 |
502.4 |
3.17 |
514.2 |
456.8 |
23 |
0.82 |
2.60 |
Toluene |
33.9 |
505.4 |
3.27 |
517.4 |
458.9 |
28.5 |
0.78 |
2.55 |
Ether |
34.5 |
501.2 |
3.45 |
512.4 |
443.9 |
22.8 |
0.91 |
3.14 |
THF |
37.4 |
503.2 |
3.65 |
515 |
455.3 |
23.4 |
0.86 |
3.14 |
EtOAc |
38.1 |
501.2 |
3.26 |
513 |
458.9 |
23.3 |
0.82 |
2.67 |
CHCl3 |
39.1 |
505.2 |
3.50 |
517.2 |
459.3 |
23 |
0.84 |
2.94 |
CH2Cl2 |
40.7 |
503.6 |
3.48 |
515.6 |
462.1 |
23.4 |
0.77 |
2.68 |
Acetone |
42.2 |
501.2 |
3.46 |
512.6 |
443.7 |
23.2 |
0.56 |
1.94 |
EtOH |
51.9 |
501.4 |
3.71 |
513 |
451 |
23.2 |
0.59 |
2.19 |
The examination of fluorescence emission properties in various organic solvents showing little solvent effects implies that emission occurs from the weakly dipolar, relaxed Franck–Condon excited state of the dyes.32 Key spectral characteristics of the dye PYBDP are summarized in Table 1. Using the values of the molar absorption coefficient εmax at λabs and the fluorescence quantum yield ΦF, the brightness (defined as the product εmax × ΦF, 104 M−1 cm−1) for a selection of solvents was computed. The maximum values of brightness for PYBDP in THF and ether are given in Table 1 and they prove that the tetramethyl-substituted PYBDP is a highly fluorescent compound, supporting the speculation that the methyl substitution at the adjacent positions to the meso part blocks the pyrene group from rotating internally and places the PYBDP in a more rigid and perpendicular disposition, thus improving the fluorescent ability.33
Lasing properties
Owing to the high absorptivity and the unified fluorescence emission behavior of PYBDP, we performed laser experiments to examine the possibility of lasing properties in various solvents. The effect of solvents on the dye laser action was analyzed in solutions at the optimized dye concentrations with the highest ASE conversion efficiency (the ratio between the ASE and pump energies). The low solubility of PYBDP in hexane prevented us from producing highly concentrated solutions that was required for the laser experiments under the pumping conditions selected in the present work. The ASE efficiency of PYBDP in ethyl ether and THF were 3.9% and 2.64% (Table S1, ESI†), much lower than that in toluene. But in solvents with intermediate and high polarity such as ethyl acetate, chloroform and ethanol, no ASE phenomenon was observed, no matter how strong the pump intensity was.
The transition from normal fluorescence, through threshold, to complete ASE can be clearly seen in Fig. 2. By increasing the excitation power above the threshold, the intensity of the ASE spectra is greatly increased, while the full width at half maximum (FWHM) of the spectra is further narrowed. At the pump energy of ∼0.8 mJ, the FWHM of the ASE radiation is 2 nm centering at 531 nm, as shown in Fig. 3.
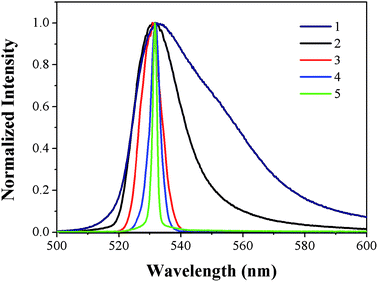 |
| Fig. 2 Normalized emission spectra of PYBDP (10 mM) in toluene with increasing optically pumped pulse densities (1-5). | |
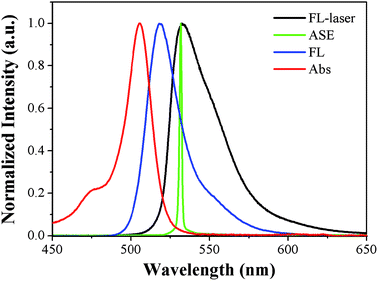 |
| Fig. 3 Normalized absorbance spectra (red), fluorescence spectra (blue), spontaneous emission spectra (black) and ASE spectra (green) of PYBDP in toluene. | |
The dependence of laser action of PYBDP on dye concentration was analyzed in toluene by varying the laser density while keeping all the other experimental parameters constant. The PYBDP dye exhibited good ASE conversion efficiencies with the highest value of 10.86% achieved by the 3 mM solution (Fig. 4). Further increases in the dye concentration resulted in a slight decrease in the ASE efficiency. This trend must be related to the effect of reabsorption/re-emission phenomena on the emission intensity because the possibility of excited molecules by absorption of a photon previously emitted by another molecule in the medium.34 The partial overlapping between absorption and fluorescence spectra (Fig. 3), which is affected by the dye concentration, could also be the origin of the decrease in the lasing efficiency observed in more concentrated solutions.35
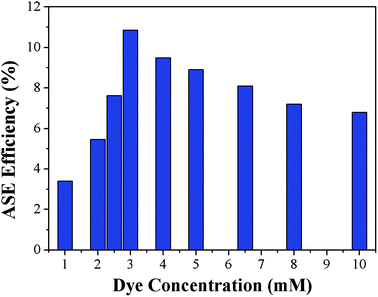 |
| Fig. 4 ASE conversion efficiency of the dye PYBDP as a function of the dye concentration in toluene solution. | |
The tuning capability, one of the most important features of laser dyes, was determined for the PYBDP dyes. As illustrated in Fig. 5, the laser emission spectrum of PYBDP shifts to lower energies as the concentration increases, allowing a tuning range from 525 to 550 nm with a maximum at 531 nm and an efficiency of 10.86%, which is comparable to many other commercially available laser dyes.36 As for a test for stability, the ASE efficiency changed little after irradiation at 355 nm (10 ns, 10 Hz) pumping in a period of one hour.
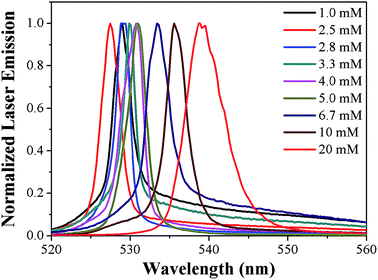 |
| Fig. 5 Laser-emission spectra of PYBDP in toluene as a function of the dye concentration. | |
Conclusion
In this work, we developed a pyrene-based tetramethyl BODIPY derivative PYBDP and investigated the absorption and fluorescent properties in different solutions. The presence of a pyrene group at the meso position in BODIPY results in stronger fluorescence emission behavior and higher fluorescence quantum yield in solvents. The PYBDP dye exhibits a maximum narrow band ASE lasing efficiency of 10.86% with a wide tunable range (525 nm to 560 nm) under demanding transversal pumping at 355 nm in toluene. The lasing efficiency remains unaffected in a period of one hour but a dramatic decrease or even the loss of the laser action is observed in intermediate or highly polar solvents. The combination of excellent photostability and tunability of ASE makes PYBDP a potentially green emitting laser dye in the green-orange region. Work is in progress to assess the lasing properties of PYBDP incorporated into solid matrices of PMMA and dye-aligned films.
Experimental section
Materials
The solvents used in the reactions were carefully dried according to the standard procedure. 1-Pyrenecarboxaldehyde was procured from TCI (Shanghai) Development Co., Ltd. All other reagent-grade chemicals were purchased from Energy-chemical (Shanghai) Reagent Co., Ltd and used without further purification. Toluene (AR grade) was purchased from Sinopharm Chemical Reagent Co., Ltd and purified before use.
Synthesis of PYBDP
The laser dye PYBDP was synthesized according to the synthetic route shown in Scheme 1. A solution of 1-pyrenecarboxaldehyde (1.0 mmol, 0.23 g), 2, 4-dimethyl pyrrole (2.0 mmol, 0.2 g) and one drop of trifluoroacetic acid (TFA) in anhydrous CH2Cl2 (30 mL) was stirred overnight under nitrogen atmosphere at room temperature. The red solution was treated with p-chloranil (1.0 mmol, 0.25 g), stirring was continued for 30 min followed by the addition of Et3N (3 mL) and BF3·Et2O (3 mL) under ice-cold conditions. The mixture was stirred at room temperature for a further 3 h. The reaction mixture was washed with water (3 × 100 mL), and the organic layers were combined and dried over anhydrous MgSO4. The solvent was concentrated under reduced pressure, and the residue was purified by column chromatography (silica gel, petroleum ether
:
EtOAc = 10
:
1, v/v) to give compound 2 as a brown powder in 25% yield.1H NMR (300 MHz, CDCl3): δ 8.32–8.00 (m, 8H), 7.89 (d, J = 7.8 Hz, 1H), 5.95 (s, 2H), 2.63 (s, 6H), 0.88 (s, 6H) ppm; 13C NMR (75 MHz, CDCl3) δ 154.01, 147.85, 145.55, 141.39, 140.04, 132.94, 129.27, 128.94, 126.69, 123.95, 117.40, 111.76, 90.26, 29.52, 13.64 ppm; IR (KBr, cm−1): ν = 2937, 2739, 1636, 1542, 1506, 1467, 1409, 1307, 1192, 1156, 1088, 981, 883, 714. ESI-MS: m/z = 448.2.
Instruments
1H and 13C NMR spectra were recorded using a Bruker Avance 300 spectrometer with tetramethylsilane (TMS; δ = 0 ppm) as an internal standard. IR spectra were run with KBr on a Thermo Nicolet 5700 FT-IR spectrometer (νmax in cm−1). All the photoluminescence spectra were measured with a JASCO FP-6500 fluorescence spectrophotometer. The UV-visible absorption spectra were recorded on a Shimadzu UV-3600 spectrophotometer. The ESI mass spectra were recorded with a Thermo Finnigan Deca XP MAX LC/MSn spectrometer system without using the liquid chromatography component. The ASE investigation was performed using Q-switched pulsed Nd:YAG laser with a repetition rate of 1 Hz and pulse duration of about 10 ns. The narrow-line width laser emission and tuning ranges of dye solutions were collected using an Ocean Optics Maya2000 Pro Fiber Optic Spectrometer. The laser power was detected by Newport 2936C laser power meter.
Laser experiments
Liquid solutions of dyes were contained in 1 cm optical-path quartz cells that were carefully sealed to avoid solvent evaporation during experiments. The excitation 355 nm laser source was normally focused onto the quartz cells (the focused pumping stripe along the alignment of the quartz cell) in a 6 mm × 0.6 mm stripe by a cylindrical lens. The polarization direction of pumping source was perpendicular to the focused pumping stripe. The ASE radiation was generated and radiated equally in two opposite directions along the sample cells; ASE output power from one end of the sample was measured as a function of the 355 nm pump power. The ASE power that was measured out of one end of the sample was only half of the total generated power, therefore the true conversion efficiency for the total generated ASE was twice of the measured efficiency.
Acknowledgements
The authors gratefully thank the financial supports of the Natural Science Foundation of Inner Mongolia Province, China (no. 2014BS0205), the Doctoral Scientific Research Foundation of Inner Mongolia University for the Nationalities (no. BS311) and the Scientific Research Program of Inner Mongolia University for the Nationalities (no. NMD1311).
References
- U. Brackmann, Laser dyes, Lambda Physik AG, Germany, 3rd edn, 2000 Search PubMed.
- I. D. W. Samuel and G. A. Turnbull, Chem. Rev., 2007, 107, 1272 CrossRef CAS PubMed.
- M. D. McGehee and A. J. Heeger, Adv. Mater., 2000, 12, 1655 CrossRef CAS.
- E. M. Calzado, P. G. Boj and M. A. Díaz-García, Int. J. Mol. Sci., 2010, 11, 2546 CrossRef CAS PubMed.
- S. Chénais and S. Forget, Polym. Int., 2012, 61, 390 CrossRef PubMed.
- S. Qu, Y. Li, L. Wang, Q. Lu and X. Liu, Chem. Commun., 2011, 47, 4207 RSC.
- S. Qu, H. Wang, W. Zhu, J. Luo, Y. Fan, L. Song, H. X. Zhang and X. Liu, J. Mater. Chem., 2012, 22, 3875 RSC.
- C. Y. Wang, Y. S. Yeh, E. Y. Li, Y. H. Liu, S. M. Peng, S. T. Liu and P. T. Chou, Chem. Commun., 2006, 2693 RSC.
- L. Sznitko, J. Mysliwiec, K. Parafiniuk, A. Szukalski, K. Palewska, S. Bartkiewicz and A. Miniewicz, Chem. Phys. Lett., 2011, 512, 247 CrossRef CAS PubMed.
- A. Monguzzi, M. Frigoli, C. Larpent and F. Meinardi, RSC Adv., 2012, 2, 11731 RSC.
- Z. Diao, S. Deng, W. Huang, L. Xuan, L. Hu, Y. Liu and J. Ma, J. Mater. Chem., 2012, 22, 23331 RSC.
- K. K. Jagtap, N. Shivran, S. Mula, D. B. Naik, S. K. Sarkar, T. Mukherjee, D. K. Maity and A. K. Ray, Chem.–Eur. J., 2013, 19, 702 CrossRef CAS PubMed.
- S. Mula, D. Hablot, K. K. Jagtap, E. Heyer and R. Ziessel, New J. Chem., 2013, 37, 303 RSC.
- J. Banuelos, F. L. Arbeloa, T. Arbeloa, V. Martinez and I. L. Arbeloa, BODIPY laser dyes applied in sensing and monitoring environmental properties, Applied Science Innovations Pvt. Ltd, 2012, ch. 19 Search PubMed.
- F. J. Duarte, Tunable laser applications, Taylor & Francis Group, LLC, 2nd edn, 2008 Search PubMed.
- J. Bauelos, V. Martin, C. F. A. Gomez-Duran, I. J. A. Cordoba, E. Pena-Cabrera, I. García-Moreno, A. Costela, M. E. Perez-Ojeda, T. Arbeloa and I. L. Arbeloa, Chem.–Eur. J., 2011, 17, 7261 CrossRef PubMed.
- G. Duran-Sampedro, A. R. Agarrabeitia, I. García-Moreno, A. Costela, J. Bañuelos, T. Arbeloa, I. L. Arbeloa, J. L. Chiara and M. J. Ortiz, Eur. J. Org. Chem., 2012, 6335 CrossRef CAS PubMed.
- T. Zhang, X. Zhu, W. K. Wong, H. L. Tam and W. Y. Wong, Chem.–Eur. J., 2013, 19, 739 CrossRef CAS PubMed.
- V. Leen, V. Z. Gonzalvo, W. M. Deborggraeve, N. Boens and W. Dehaen, Chem. Commun., 2010, 46, 4908 RSC.
- S. C. Guggenheimer, J. H. Boyer, K. Thangaraj, M. Shah, M. L. Soong and T. G. Pavlopoulos, Appl. Opt., 1993, 32, 3942 CrossRef CAS PubMed.
- L. Jiao, C. Yu, J. Li, Z. Wang, M. Wu and E. Hao, J. Org. Chem., 2009, 74, 7525 CrossRef CAS PubMed.
- V. Lakshmi and M. Ravikanth, J. Org. Chem., 2013, 78, 4993 CrossRef CAS PubMed.
- L. Wang, B. Verbelen, C. Tonnelé, D. Beljonne, R. Lazzaroni, V. Leen, W. Dehaen and N. Boens, Photochem. Photobiol. Sci., 2013, 12, 835 CAS.
- I. Esnal, J. Banuelos, I. L. Arbeloa, A. Costela, I. García-Moreno, M. Garzon, A. R. Agarrabeitia and M. J. Ortiz, RSC Adv., 2013, 3, 1547 RSC.
- A. Loudet and K. Burgess, Chem. Rev., 2007, 107, 4891 CrossRef CAS PubMed.
- G. Ulrich, R. Ziessel and A. Harriman, Angew. Chem., Int. Ed, 2008, 47, 1184 CrossRef CAS PubMed.
- N. Boens, V. Leen and W. Dehaen, Chem. Soc. Rev., 2012, 41, 1130 RSC.
- A. Bessette and G. S. Hanan, Chem. Soc. Rev., 2014, 43, 3342 RSC.
- Y. Yang, L. Zhang, B. Li, L. Zhang and X. Liu, RSC Adv., 2013, 3, 14993 RSC.
- O. A. Bozdemir, F. Sizmen, O. Buyukcakir, R. Guliyev, Y. Cakmak and E. U. Akkaya, Org. Lett., 2010, 12, 1400 CrossRef CAS PubMed.
- I. Esnal, J. Banuelos, I. L. Arbeloa, A. Costela, I. Garcia-Moreno, M. Garzon, A. R. Agarrabeitia and M. J. Ortizc, RSC Adv., 2013, 3, 1547 RSC.
- J. Banuelos, I. J. Arroyo-Cordoba, I. Valois-Escamilla, A. Alvarez-Hernandez, E. Pena-Cabrera, R. Hu, B. Z. Tang, I. Esnal, V. Martınez and I. L. Arbeloa, RSC Adv., 2011, 1, 677 RSC.
- R. Ziessel, C. Goze, G. Ulrich, M. Cesario, P. Retailleau, A. Harriman and J. P. Rostron, Chem.–Eur. J., 2005, 11, 7366 CrossRef CAS PubMed.
- I. García-Moreno, D. Zhang, A. Costela, V. Martín, R. Sastre and Y. Xiao, J. Appl. Phys., 2010, 107, 073105 CrossRef PubMed.
- D. Zhang, V. Martín, I. García-Moreno, A. Costela, M. E. Pérez-Ojeda and Y. Xiao, Phys. Chem. Chem. Phys., 2011, 13, 13026 RSC.
- I. Garcia-Moreno, L. Wang, A. Costela, J. Banuelos, I. L. Arbeloa and Y. Xiao, ChemPhysChem, 2012, 13, 3923 CrossRef CAS PubMed.
Footnote |
† Electronic supplementary information (ESI) available. See DOI: 10.1039/c4ra04759a |
|
This journal is © The Royal Society of Chemistry 2014 |
Click here to see how this site uses Cookies. View our privacy policy here.