DOI:
10.1039/C4RA01993H
(Paper)
RSC Adv., 2014,
4, 17022-17027
Selective bromination of 2,5-bis(2-thienyl)pyrroles and solid-state polymerization through the β-carbon of pyrrole†
Received
8th March 2014
, Accepted 27th March 2014
First published on 27th March 2014
Abstract
Bromination of 2,5-bis(2-thienyl)pyrrole with NBS in AcOH–THF prefers the β-position of pyrrole over the α-position of thiophene. A DFT study and experiments in different solvent systems suggest the important role of the solvent in tuning the selectivity. Unusual solid-state polymerization (SSP) of β,β′-dibrominated 2,5-bis(2-thienyl)pyrrole was observed through the β-C of pyrrole to afford the bromine doped conjugated polymer. DSC, CHN and SEM analyses were carried out to study the SSP process.
1. Introduction
Conducting polymers have attracted significant interest because of their applications in organic electronic devices such as organic photovoltaics (OPVs), organic light emitting diodes (OLEDs) and organic field effect transistors (OFETs).1 Five membered heterocycles such as thiophene and pyrrole are extensively used to design new conjugated polymers for their applications in organic electronics. However, there are only a few studies on copolymers containing both thiophene and pyrrole. Such polymers may provide an attractive approach to tailored electroactive materials with the added advantage of incorporating solubilizing groups on the pyrrole nitrogen to synthesize the solution processable polymers. Comparative study by Oliva et al. on thiophene–pyrrole oligomers and oligothiophenes indicated that incorporation of pyrroles in connection with thiophenes in cooligomers improved both the oxidation capacity and the luminescence properties relative to their homogeneous oligothiophenes.2 OFET,3 OPV and electroluminescent devices,4 have been fabricated from the oligomers and polymers incorporating N-aryl-2,5-bis(2-thienyl)pyrrole.
The synthesis and material processing are two important aspects of the development of new conjugated materials for organic electronics. Pyrrole is more reactive than thiophene toward aromatic electrophilic substitution (SEAr). The reactivity difference between α- and β-positions of pyrrole is less than that of thiophene. These two points creates interesting trend in reactivity difference of α-position of thiophene and β-position of pyrrole in the SEAr reactions.5
Development of new processing technique to obtain the polymer with a higher degree of order has immense importance for device performance. Conjugated polymers can be directly obtained in solid state from a soluble and structurally pre-organized crystalline monomer by solid-state polymerization (SSP). It may afford nearly defect-free and highly ordered polymers. SSP of a suitable monomer in a well-ordered crystalline state was first introduced by Wenger with polydiactylenes6 and by Cohen et al. with poly(sulphur nitride) (SN)x.7 There are very few reports on solid-state polymerization process till date, where doped polythiophene and polyselenophene were successfully synthesized with high conductivities.8
Here we report the relative reactivity of β-position of pyrrole and α-position of thiophene in SEAr reaction (bromination) of 2,5-bis(2-thienyl)pyrrole. Bromination reaction with NBS in AcOH–THF preferred β-position of pyrrole over the α-position of thiophene. Heating of β,β′-dibrominated 2,5-bis(2-thienyl)pyrrole afforded bromine doped conjugated polymer through β-C of pyrrole via solid state polymerization. It is a first report on SSP of pyrrole through β-position of pyrrole.
2. Result and discussion
2.1. Synthesis and selectivity towards bromination
Compound 1 and 5 was prepared by Paal–Knorr reaction. Compound 1 and 5 are crystalline solid and viscous liquid, respectively. The hydroxyl group has significant role in intermolecular interaction which drives compound 5 to be solid and crystalline. Bromination reaction of compound 1 with 2.2 equiv. of NBS in THF–AcOH afforded exclusively β,β′-dibrominated crystalline solid compound 2 with 81% yield (Scheme 1). It indicated the higher reactivity of β-position of pyrrole for bromination than the α-position of thiophene. To get more insight into the reactivity order, compound 1 was reacted with varying equiv. of NBS under similar reaction conditions. The reaction of 1 with 1.1 and 4.4 equiv. of NBS afforded 3 and 4, respectively. Thus the bromination at α-C of thiophene takes place after blocking β-C of pyrrole. Further reaction of 5 with 2.2 equiv. of NBS exclusively afforded 6 in high yields which suggests the applicability of the methodology for selective bromination on the β-position of pyrrole over the α-position of thiophene, in case of T–Py–T like system. When DMF and AcOH were used as solvent in the reaction of 1 with 2.2 equiv. of NBS, very complicated product mixture was obtained.
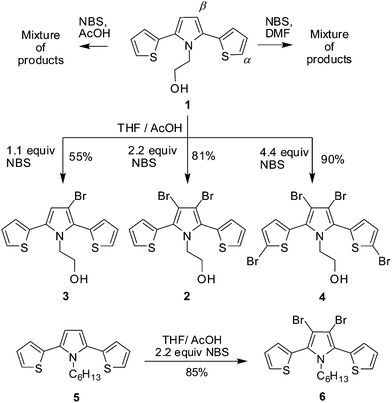 |
| Scheme 1 Chemoselective bromination of compound 1 and 5. | |
It is evident from the literature that for thiophenes-capped pyrrole the relative reactivities of pyrrole β-C and thiophene α-C in SEAr reactions vary based on N-substitution and reaction conditions. Bromination, formylation, acylation and Mannich reaction on N-unsubstituted 2,5-di(2-thienyl)pyrrole preferred β-position of pyrrole.9 Reaction of N-unsubstituted 2,5-di(2-thienyl)pyrrole with n-butylmagnesium bromide followed by reaction with ethylene oxide afforded β-hydroxyethyl substituted 2,5-di(2-thienyl)pyrrole.9 Hydroxymethylation was reported to take place at β-position of pyrrole in N-alkyl-2,5-di(2-thienyl)pyrrole.10 Bromination of N-aryl-2,5-di(2-thienyl)pyrroles with NBS afforded α-substitution at thiophene ring.11 Acylation and formylation were reported at β-position of pyrrole irrespective of N-substitution.10,12 Lithiation and electrophilic substitution by tetracyanoethylene prefer α-position of thiophene irrespective of N-substitution.13
2.2. Theoretical calculation
To shed more light on the experimentally observed reactivity of bromination reactions of 1 with NBS, we have carried out DFT calculations at the B3LYP/6-31G(d) level (corrected for unscaled ZPVE) using Gaussian 09.14 The reaction pathways were calculated for the formation of 3 and 7 by α-bromination of thiophene ring and β-bromination of pyrrole ring of compound 1, respectively. Further, the calculation was extended for second bromination step on 3 and again consider two pathways similar to that of first step to afford 2 and 8, respectively. The results of the calculations are depicted in Fig. 1. DFT calculations showed that the activation barriers for the β-bromination of pyrrole rings of 1 and 7 were less than that for the α-bromination of thiophene rings. The products 3 and 2 resulted from β-bromination of pyrrole rings were thermodynamically more stable than the products 7 and 8 resulted from the α-bromination of thiophene ring. However, the energy difference between the activation barriers (within 1–3 kcal mol−1) was insignificant to consider the chemoselectivity of one of the positions. It implies that small changes in the reaction conditions including the choice of solvent might change the course of the reaction. Indeed, as discussed above, by changing solvent from THF–AcOH to DMF, we have observed significant change in the reaction outcome of the bromination of 1 with NBS. Literature reports also indicated the change in reactivity trend with change in N-substitution of 2,5-di(2-thienyl)pyrrole and nature of electrophile. To include the solvent effect, we have corrected the gas phase values by using single-point B3LYP/6-31G(d) calculations for acetic acid and DMF as a solvent with the Polarized Continuum Model (PCM) using B3LYP/6-31G(d) optimized geometries (Fig. S1†).15 Incorporation of solvent effect significantly decreases the activation barriers in all cases, however, trend in the reactivity remained unchanged.
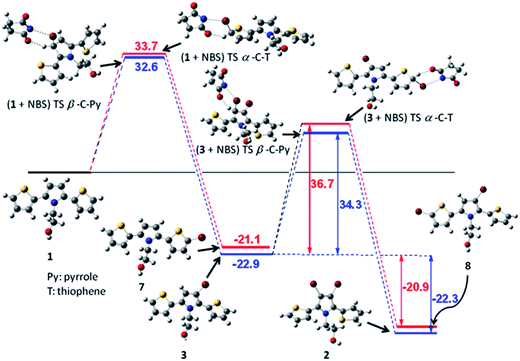 |
| Fig. 1 DFT calculation of the reaction pathway of bromination of 1 by using B3LYP/6-31G(d) (energy values are given in kcal mol−1 including ZPVE). | |
2.3. Solid state polymerization
Compound 2, a colourless crystalline solid, was soluble in all common organic solvents and stable in solution. On storage in closed flask in the solid state at room temperature, we have observed the slow transformation of 2 from colourless crystals to the black material with subsequent evolution of brown vapour of bromine. This observation indicates the possibility of solid state polymerization of 2 to afford the polymer. When white crystals of 2 were heated at 60 °C, it transformed slowly into lustrous black crystals within 7–8 h while retaining the crystal shape and size (Fig. 2). It is interesting to note that monobrominated compound 3 did not show any transformation to black crystal upon prolonged heating. The SEM analysis showed that the roughness of surface of the crystal was increased after SSP due to evaporation of bromine although shape and size remain unchanged (Fig. 3). The resulting black crystals were completely insoluble in common organic solvents. Elemental analysis of vacuum-dried samples indicated that P2 contain 1.4 atoms of bromine per thiophene–pyrrole–thiophene unit. Low conductivity values (10−4 to 10−5 S cm−1) of doped polymers can be ascribed to the lower conjugation through the β-position of pyrrole and steric hindrance due to α-thienyl substitution on pyrrole ring. The FT-IR spectra of P2 obtained by SSP showed very strong broad absorption bands for O–H stretching (3300 cm−1), characteristic C–O stretching (1200 and 1070 cm−1) and aromatic C–C double bond stretching (1600 cm−1) (Fig. S2 in ESI†).
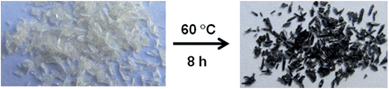 |
| Fig. 2 Photographs of crystals of 2 (left) and doped P2 (right) obtained on heating the crystals of the monomer at 60 °C for 8 h. | |
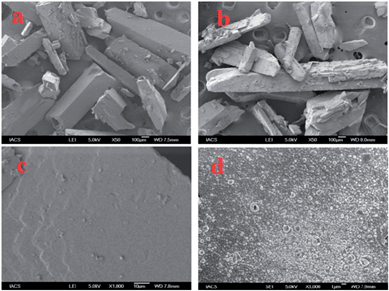 |
| Fig. 3 SEM picture of the surface of 2 (a) with a 100 μm scale bar; (c) with 10 μm scale bar; P2 obtained by solid-state polymerization (b) with 100 μm scale bar and (d) with 1 μm scale bar. | |
2.4. DSC study
Differential scanning calorimetry (DSC) was used to follow the SSP process of dibrominated monomer 2. On heating the dibrominated compound 2 at a scan rate of 10 °C min−1 showed an exothermic peak at 98.7 °C corresponding to the polymerizations (Fig. 4a). DSC of 2 was recorded as a function of scan rate (Fig. S3 in ESI†). The kinetics of the solid-state polymerization of 2 was studied by isothermal DSC. Heating of 2 at different temperatures (65–80 °C) below its melting point resulted in exothermic polymerization (Fig. 4b). The measured reaction enthalpy was 10.1 kcal mol−1 (at 80 °C) which was significantly lower than that observed for DBEDOT (14.1 kcal mol−1)14 and DBEDOS (18.5 kcal mol−1). The activation energy of solid-state polymerization of 2 obtained by using Arrhenius equation was 38.5 kcal mol−1 (Fig. 4c).
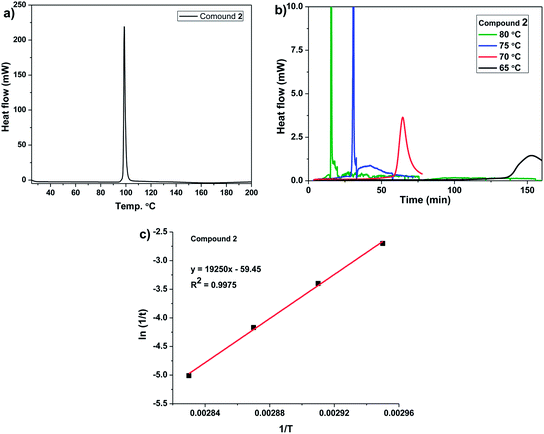 |
| Fig. 4 a) DSC curves of compound 2 with a heating scan-rate 10 °C min−1; (b) isothermal DSC curves of compound 2; (c) Arrhenius plotting to determine the activation energy of SSP reaction of 2. | |
2.5. X-ray crystallography
Short intermolecular Br–Br distance (shorter than the sum of van der Waals (vdW) radii of bromine (3.9 Å)) may favour solid-state polymerization through the expulsion of molecular bromine and concomitant C–C bond formation.8 Single crystal X-ray crystallographic analysis of 2 (Fig. 5) showed three type of intermolecular Br–Br distances of 3.84, 4.10 and 4.51 Å, respectively (Fig. S4 in ESI†). In crystal structure of 2 the alternate hydrogen bonding and bromine short contact (3.84 Å) form one dimensional chains. These molecular chains arrange to form molecular sheets along b-axis. This arrangement of molecules created bromine channels in the molecular sheets. It may be one of the favourable factors for SSP.
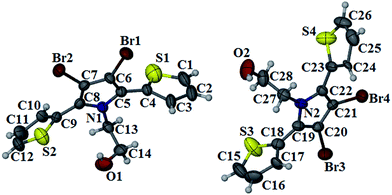 |
| Fig. 5 ORTEP diagram of crystal of 2. | |
3. Conclusion
In conclusion, we have explored the relative reactivity of β-position of pyrrole and α-position of thiophene toward NBS bromination (SEAr reaction) in cooligomer 1. Bromination reaction showed solvent dependent chemoselectivity. DFT calculations indicated that although the reactivity of β-position of pyrrole is higher than α-position of thiophene, the energy difference is not significant to consider the chemoselectivity. Thus, the choice of solvent influenced the outcome of the reaction. Dibrominated products 2 formed bromine doped conducting polymers via solid state polymerization. Polymers by solid state polymerization through the 3,4-position of pyrrole of thiophene–pyrrole–thiophene system is reported for the first time. These results indicate that the care should be taken while working with pyrrole containing oligomers as electro- and solid-state polymerization of pyrrole can even go through β-position of pyrrole.
4. Experimental
4.1. Materials and characterization methods
Dry toluene and THF were distilled from sodium/benzophenone prior to use. Acetonitrile was distilled from P2O5 prior to use. Thiophene, succinyl chloride N-bromosuccinamide (NBS) were purchased from Aldrich and used without further purification. Ethanolamine, hexylamine, propionic acid purchased from Merck. Crystal of 2 was obtained from hexane–ethylacetate solvent mixture by slow evaporation method. The crystal was carefully chosen using a stereomicroscope supported by a rotatable polarizing stage. Crystal data was collected at rt on Bruker's KAPPA APEX II CCD Duo with graphite monochromated Mo-Kα radiation (0.71073 Å). Crystal was glued to a thin glass fibre using FOMBLIN immersion oil and mounted on the diffractometer. The intensity data were processed using Bruker's suite of data processing programs (SAINT), and absorption corrections were applied using SADABS.16 Crystal structures were solved by direct methods using SHELXS-97 and the data was refined by full matrix least-squares refinement on F2 with anisotropic displacement parameters for non-H atoms, using SHELXL-97.17
4.2. Synthetic procedure
Compound 1,4-di(thiophen-2-yl)butane-1,4-dione18 was synthesized by following reported literature.
4.3. General procedure for Knorr–Paal synthesis
A 50 mL two-necked round-bottom flask equipped with a Dean–Stark trap and condenser was charged with 2 mmol of 1,4-di(thiophen-2-yl)butane-1,4-dione and 10 mmol of ethanolamine (or hexylamine) in dry toluene (20 mL) and propionic acid in catalytic amount. The reaction mixture was refluxed at 110 °C for 16 h and then cooled to rt. Toluene was evaporated under reduced pressure and resulting crude solid was purified by column chromatography (silica gel) using 3
:
1 hexanes–CH2Cl2.
Compound 1: colourless solid. Yield: 82%. 1H NMR (400 MHz, DMSO-d6, ppm) δ: 3.41 (m, 2H), 4.22 (t, J = 8.6 Hz, 2H), 4.96 (t, J = 6.6 Hz, 1H), 6.28 (s, 2H), 7.14 (m, 2H), 7.25 (m, 2H), 7.56 (m, 2H). 13C NMR (100 MHz, DMSO-d6, ppm) δ: 40.1, 60.1, 110.5, 125.8, 126.0, 127.8, 128.0, 134.0. HRMS (ESI) m/z calculated for C14H13NOS2 [M+] 275.0439; found 275.0431.
4.4. General procedure for bromination
Compound 1 (or 5) (3 mmol) was dissolved in THF–AcOH (1
:
1, 30 mL) mix solvent. Reaction mixture was cooled by ice-water. NBS (6.5 mmol) was added at once in the absent of light. The reaction mixtured was allowed to warm at rt and stirred for 24 h. After removal of solvent the crude mixture was directly loaded to column of silica gel for purification and eluted with hexane–ethylacetate (98
:
2) solvent mixture.
Compound 2: colourless crystals. Yield: 81%. 1H NMR (400 MHz, DMSO-d6, ppm) δ: 3.24 (m, 2H), 3.96 (t, J = 8.2 Hz, 2H), 4.84 (t, J = 6.7 Hz, 1H), 7.22 (m, 2H), 7.31 (m, 2H), 7.78 (m, 2H). 13C NMR (100 MHz, DMSO-d6, ppm) δ: 39.9, 60.0, 110.5, 125.8, 126.0, 127.7, 128.0, 134.0. HRMS (ESI) m/z calculated for C14H11Br2NOS2 [M+] 430.8649; found 430.8656.
Compound 3: colourless solid. Yield: 51%. 1H NMR (400 MHz, DMSO-d6, ppm) δ: 3.29 (m, 2H), 4.07 (t, J = 8.4 Hz, 2H), 4.90 (t, J = 6.6 Hz, 1H), 6.45(s, 1H), 7.15 (m, 1H), 7.21 (m, 1H), 7.27 (m, 1H), 7.31 (m, 1H), 7.63 (m, 1H), 7.75 (m, 1H). 13C NMR (100 MHz, DMSO-d6, ppm) δ: 47.2, 59.8, 98.2, 112.1, 125.4, 126.1, 127.1, 127.4, 127.8, 128.2, 128.5, 130.2, 130.4, 132.5. HRMS (ESI) m/z calculated for C14H12BrNOS2 [M+] 352.9544; found 352.9550.
Compound 4: pale yellow solid. Yield: 92%. 1H NMR (400 MHz, DMSO-d6, ppm) δ: 3.27 (m, 2H), 3.97 (t, J = 7.6 Hz, 2H), 4.92 (t, 1H, J 6.9 Hz), 7.17 (d, J = 4.2 Hz, 2H), 7.34 (d, J = 4.4 Hz, 2H). 13C NMR (100 MHz, DMSO-d6, ppm) δ: 49.1, 60.0, 102.0, 114.4, 125.6, 131.3, 132.0, 132.4. HRMS (ESI) m/z calculated for C14H9Br4NOS2 [M+] 586.6859; found 586.6868.
Compound 6: colourless viscous liquid. Yield: 82%. 1H NMR (400 MHz, DMSO-d6, ppm) δ: 0.71 (m, 3H), 0.91 (m, 4H), 0.93–1.03 (m, 2H), 1.30 (m, 2H), 3.89 (t, J = 6.3 Hz, 2H), 7.22 (m, 2H), 7.28 (m, 2H) 7.80 (m, 2H). 13C NMR (100 MHz, DMSO-d6, ppm) δ: 13.6, 21.5, 25.1, 29.8, 30.1, 40.0, 100.7, 125.4, 127.5, 128.9, 129.8, 130.4. HRMS (ESI) m/z calculated for C18H19Br2NS2 [M+] 470.9326, found 470.9318.
4.5. General procedure for solid state polymerization
In a typical experimental procedure, crystalline 2 (200 mg) was placed in a 50 mL round bottle flask that was closed with stopper. The flask was heated at 50 °C for 24 h, during which period the original white color of dibromo compounds turned black. The sequence of color changes (from white → gray → dark black) of the material and the appearance of brown bromine vapor in the flask was indicative of the progress of the solid state polymerization. Performing the reaction in an inert atmosphere (Ar or N2) or in a vacuum-sealed vial did not have any apparent effect on the polymerization time or properties of the product. P2: elemental analysis found: N, 3.61; C, 43.55; H, 2.74%.
Acknowledgements
We thank DRDO, India for funding. PBP thanks UGC, India for fellowship.
References
-
(a) Handbook of Conducting Polymers, ed. T. A. Skotheim and J. R. Reynolds, CRC Press, Boca Raton, FL, 3rd edn, 2007 Search PubMed;
(b) Handbook of Organic Conductive Molecules and Polymers, ed. H. S. Nalwa, John Wiley & Sons, New York, 1997, vol. 2 Search PubMed;
(c) Handbook of Thiophene-Based Materials: Applications in Organic Electronics and Photonics, ed. I. F. Perepichka and D. F. Perepichka, John Wiley & Sons, New York, 2009, vol. 1 and 2 Search PubMed.
- M. M. Oliva, T. M. Pappenfus, J. H. Melby, K. M. Schwaderer, J. C. Johnson, K. A. McGee, D. A. da Silva Filho, J. L. Bredas, J. Casado and J. T. N. Navarrete, Chem. - Eur. J., 2010, 16, 6866–6876 CrossRef CAS PubMed.
-
(a) T. Nishinaga, T. Miyata, M. Tateno, M. Koizumi, M. Takase, M. Iyoda, N. Kobayashi and Y. Kunugi, J. Mater. Chem., 2011, 21, 14959–14966 RSC;
(b) M. Fujii, T. Nishinaga and M. Iyoda, Tetrahedron Lett., 2009, 50, 555–558 CrossRef CAS PubMed.
-
(a) A. Dhanabalan, K. J. van Duren, P. A. van Hal, D. L. J. van Dongen and R. A. J. Janssen, Adv. Funct. Mater., 2001, 11, 255–262 CrossRef CAS;
(b) C. J. Brabec, C. Winder, N. S. Sariciftci, J. C. Hummelen, A. Dhanabalan, P. A. van Hal and R. A. J. Janssen, Adv. Funct. Mater., 2002, 12, 709–712 CrossRef CAS;
(c) Y. Liu, M. A. Summers, C. Edder, J. M. J. Fréchet and M. D. McGehee, Adv. Mater., 2005, 17, 2960–2964 CrossRef CAS PubMed.
- G. G. Abashev, A. Y. Bushueva and E. V. Shklyaeva, Chem. Heterocycl. Compd., 2011, 47, 103–110 CrossRef.
-
(a) G. Wegner, Z. Naturforsch., B, 1969, 24, 824–832 CAS;
(b) G. Wegner, Makromol. Chem., 1971, 145, 85–90 CrossRef CAS PubMed.
- M. J. Cohen, A. F. Garito, A. J. Heeger, A. G. MacDiarmid, C. M. Mikulski, M. S. Saran and J. Kleppinger, J. Am. Chem. Soc., 1976, 98, 3844–3848 CrossRef CAS.
-
(a) H. Meng, D. F. Perepichka and F. Wudl, Angew. Chem., Int. Ed., 2003, 42, 658–661 CrossRef CAS PubMed;
(b) H. Meng, D. F. Perepichka, M. Bendikov, F. Wudl, G. Z. Pan, W. Yu, W. Dong and S. Brown, J. Am. Chem. Soc., 2003, 125, 15151–15162 CrossRef CAS PubMed;
(c) H. J. Spencer, R. Berridge, D. J. Crouch, S. P. Wright, M. Giles, I. Mcculloch, S. J. Coles, M. B. Hurtsthouse and P. J. Skabara, J. Mater. Chem., 2003, 13, 2075–2077 RSC;
(d) A. Patra, Y. H. Wijsboom, S. S. Zade, M. Li, Y. Sheynin, G. Leitus and M. Bendikov, J. Am. Chem. Soc., 2008, 130, 6734–6736 CrossRef CAS PubMed;
(e) M. Lepeltier, J. Hiltz, T. Lockwood, F. Bélanger-Gariépy and D. F. Perepichka, J. Mater. Chem., 2009, 19, 5167–5174 RSC;
(f) A. Patra, Y. H. Wijsboom, G. Leitus and M. Bendikov, Chem. Mater., 2011, 23, 896–906 CrossRef CAS.
- J. P. Albarella and N.-H. Lin, US Pat. 5021586, 1991.
- L. I. Belen'kii, G. P. Gromova and V. I. Smirnov, Chem. Heterocycl.
Compd., 2008, 44, 1092–1111 CrossRef CAS PubMed.
-
(a) V. Tamilavan, P. Sakthivel, Y. Li, M. Song, C.-H. Kim, S.-H. Jin and M. H. Hyun, J. Polym. Sci., Part A: Polym. Chem., 2010, 48, 3169–3177 CrossRef CAS PubMed;
(b) F. B. Koyuncu, E. Sefer, S. Koyuncu and E. Ozdemir, Polymer, 2011, 52, 5772–5779 CrossRef CAS PubMed;
(c) V. Tamilavan, N. Cho, C. Kim, J. Ko and M. H. Hyun, Tetrahedron, 2012, 68, 5890–5897 CrossRef CAS PubMed.
-
(a) N. A. Lengkeek, J. M. Harrowfield and G. A. Koutsantonis, Synth. Met., 2010, 160, 72–75 CrossRef CAS PubMed;
(b) S. Noureen, S. Caramori, A. Monari, X. Assfeld, R. Argazzi, C. A. Bignozzi, M. Beley and P. C. Gros, Dalton Trans., 2012, 41, 4833–4844 RSC.
-
(a) R. E. Niziurski-Mann, C. Scordilis-Kelley, T. L. Liu, M. P. Cava and R. T. Carlin, J. Am. Chem. Soc., 1993, 115, 887–891 CrossRef CAS;
(b) J. P. Parakka, A. P. Chacko, D. E. Nikles, P. Wang, S. Hasegawa, Y. Maruyama, R. M. Metzger and M. P. Cava, Macromolecules, 1996, 29, 1928–1933 CrossRef CAS;
(c) M. Kozaki, J. P. Parakka and M. P. Cava, J. Org. Chem., 1996, 61, 3657–3661 CrossRef CAS PubMed;
(d) T. V. Hughes and M. P. Cava, J. Org. Chem., 1999, 64, 313–315 CrossRef CAS;
(e) K.-S. Kim, M.-S. Kang, H. Ma and A. K.-Y. Jen, Chem. Mater., 2004, 16, 5058–5062 CrossRef CAS;
(f) Y.-S. Yen, Y.-C. Hsu, J. T. Lin, C.-W. Chang, C.-P. Hsu and D.-J. Yin, J. Phys. Chem. C, 2008, 112, 12557–12567 CrossRef CAS;
(g) T. Nishinaga, M. Tateno, M. Fujii, W. Fujita, M. Takase and M. Iyoda, Org. Lett., 2010, 12, 5374–5377 CrossRef CAS PubMed;
(h) C. Kaiser, M. Behl, M. Schroeter, K. Kratz and A. Lendlein, React. Funct. Polym., 2012, 72, 533–541 CrossRef CAS PubMed.
- M. J. Frisch, G. W. Trucks, H. B. Schlegel, G. E. Scuseria, M. A. Robb, J. R. Cheeseman, G. Scalmani, V. Barone, B. Mennucci, G. A. Petersson, H. Nakatsuji, M. Caricato, X. Li, H. P. Hratchian, A. F. Izmaylov, J. Bloino, G. Zheng, J. L. Sonnenberg, M. Hada, M. Ehara, K. Toyota, R. Fukuda, J. Hasegawa, M. Ishida, T. Nakajima, Y. Honda, O. Kitao, H. Nakai, T. Vreven, J. A. Montgomery, Jr, J. E. Peralta, F. Ogliaro, M. Bearpark, J. J. Heyd, E. Brothers, K. N. Kudin, V. N. Staroverov, R. Kobayashi, J. Normand, K. Raghavachari, A. Rendell, J. C. Burant, S. S. Iyengar, J. Tomasi, M. Cossi, N. Rega, J. M. Millam, M. Klene, J. E. Knox, J. B. Cross, V. Bakken, C. Adamo, J. Jaramillo, R. Gomperts, R. E. Stratmann, O. Yazyev, A. J. Austin, R. Cammi, C. Pomelli, J. W. Ochterski, R. L. Martin, K. Morokuma, V. G. Zakrzewski, G. A. Voth, P. Salvador, J. J. Dannenberg, S. Dapprich, A. D. Daniels, O. Farkas, J. B. Foresman, J. V. Ortiz, J. Cioslowski and D. J. Fox, Gaussian 09, Revision C.01, Gaussian Inc., Wallingford CT, 2010 Search PubMed.
- M. Cossi, V. Barone, R. Cammi and J. Tomasi, Chem. Phys. Lett., 1996, 255, 327–335 CrossRef CAS and references therein.
- Bruker SADABS V2008-1, Bruker AXS, Madison, WI, USA, 2008 Search PubMed.
- G. M. Sheldrick, SHELX 97 Program for Crystal Structure Determination, University of Göttingen, Göttingen, Germany, 1997 Search PubMed.
- P. E. Just, K. I. Chane-Ching and P. C. Lacaze, Tetrahedron, 2002, 58, 3467–3472 CrossRef CAS.
Footnote |
† Electronic supplementary information (ESI) available: 1H and 13C NMR of all compounds, absolute energies and coordinates of DFT calculation. Crystal structure information of 2. CCDC 935851. For ESI and crystallographic data in CIF or other electronic format see DOI: 10.1039/c4ra01993h |
|
This journal is © The Royal Society of Chemistry 2014 |
Click here to see how this site uses Cookies. View our privacy policy here.