DOI:
10.1039/C4RA01718H
(Paper)
RSC Adv., 2014,
4, 22470-22475
Facile and green synthesis of graphene
Received
27th February 2014
, Accepted 29th April 2014
First published on 29th April 2014
Abstract
Herein, we report a simple, facile, green and cost effective strategy for the synthesis of graphene using naturally available anti-oxidants such as carotenoids present in vegetable (carrot, sweet potato, etc.) extracts. In this work, we have employed carrot extract to reduce graphene oxide to reduced graphene oxide. A red shift (in the λmax from 230 nm to 270 nm) during the course of the reduction of GO clearly indicates the effective restoration of the sp2 graphitic carbons. In addition, we have also noticed the colour change of the reaction mixture from yellowish brown to black after 1 hour, thereby indicating the reduction of GO to reduced graphene oxide (Ct-RGO). Further, an increase in the D/G ratio value of GO from 0.979 to 1.198 after the complete reduction indicated the effective restoration of the in plane sp2 domains in the Ct-RGO. The morphology and conductivities of the Ct-RGO are characterized by several characterization techniques such as UV, FT-IR, Raman, XRD, XPS, SEM, TEM, AFM and EIS. The green synthesis reported in this work is expected to yield a biocompatible graphene material suitable for futuristic biological applications.
Introduction
Graphene is a two-dimensional carbon material that finds substantial applications in the areas of nanoelectronic devices, biosensors, energy storage devices, solar cells, drug delivery systems and optical devices.1–4 The profound application of graphene is due to its excellent mechanical strength and electronic and thermal properties.5 In the literature, several strategies have been reported to synthesize graphene such as epitaxial growth,5–7 cutting carbon nanotubes,8,9 direct sonication,10,11 chemical vapour deposition,12–14 micro mechanical exploitation,15–17 chemical route,18 template directed method19 and thermal strategy.20 However, all these methods have their own advantages and disadvantages. Common problems encountered during the synthesis of graphene are the preparation of a stable dispersion of biocompatible graphene and the scalability of the existing methods. Though it is possible to scale-up the chemical reduction methods, a major drawback of all these methods is that they employ extremely hazardous and toxic agents such as hydrazine, dimethyl hydrazine, sodium borohydride, etc. In order to overcome or eliminate the toxicity associated with the reduced graphene oxide (RGO) obtained using the above methods, we herein report a novel strategy to obtain eco-friendly and biocompatible graphene by a greener route. There are reports available in the literature regarding the eco-friendly conversion of GO to RGO using non-toxic reducing agents such as vitamin C,21 reducing sugars22 and bovine serum albumin.23 Though these existing strategies are successful in eliminating the toxic effects, the RGO obtained using these reducing agents is highly agglomerated.21–27 In order to overcome this issue, we developed a green and non-toxic route to reduce GO to RGO using carotenoids available in vegetable extracts.
Carotenoids react with highly oxidizing species such as singlet oxygen, hydroxyl radical, superoxide, hydrogen peroxide, organic hydroperoxides and peroxy radicals generated in biological systems by any one of the following three distinct pathways, viz. electron transfer, hydrogen abstraction and radical addition.28 Examples of carotenoids include lycopene, lutein, β-carotene and 3-epilutein. The structures of these carotenoids are shown below.
Of the various carotenoids mentioned above, lycopene is a highly unsaturated straight chain hydrocarbon with a total of 13 double bonds, out of which 11 are conjugated. This unique nature of the lycopene molecule makes it a potential anti-oxidant among other carotenoids. In vitro studies revealed that the antioxidant property of lycopene is 2 times that of β-carotene, 10 times that of α-tocopherol, 100 times that of vitamin E and 125 times that of glutathione towards singlet oxygen quenching ability.29,30 A combination of two or more carotenoids was found to be more effective than a single carotenoid in its action towards antioxidant properties. This synergistic effect was more pronounced when lycopene or lutein was present in the mixture.31 As a result, several reactive oxygen species can be effectively reduced by carotenoids, and the resultant products are physiologically friendly, relatively stable and inert. Carrot is a vegetable that contains abundant antioxidants such as β-carotene, lutein and lycopene.28,31,32 Herein, we discuss a simple, facile, green and cost effective strategy to reduce GO into reduced graphene oxide (Ct-RGO) by using carrot extract.
Experimental section
Materials
Graphite powder ≤20 µm was purchased from Sigma-Aldrich. Analytical Grade KMnO4, NaNO3, H2SO4, HCl, NaOH and H2O2 were all purchased from Alfa Aesar and used as purchased. Fresh carrots were purchased from a local vegetable market.
Methods and instruments employed
UV-Visible spectra were recorded using a Perkin Elmer Scan Lambda 650, UV-Visible spectrophotometer. FT-IR spectra were recorded using a Tensor 27 FT-IR spectrophotometer (Bruker optics) between 4000 and 400 nm−1 on transmittance mode. Raman spectra were obtained using a Renishaw Raman system 2000 model that employs a 514 nm Ar+ laser source. AFM images were recorded in the tapping mode at ambient conditions using an Agilent Technologies 5500 model AFM instrument. SEM images were obtained using a Tescan SEM VEGA 3 XM, and TEM analysis were performed using a Tecnai G2 20 with an accelerating voltage of 220 kV (FEI make). Electrochemical impedance spectra were recorded using a PGSTAT 302N model potentiostat.
Results and discussion
Preparation of GO
GO was obtained by Hummers method.33 Briefly, 10 g of graphite and 5 g of NaNO3 were added into a round bottom flask. To this mixture, 250 mL of conc. H2SO4 was added drop wise and stirred for 30 min. The reaction mixture was then cooled to 20 °C. Following this, 30 g of KMnO4 was gradually added to this reaction mixture with vigorous stirring. The temperature of the mixture was increased to 35 °C and maintained at 35 °C for 30 minutes. Following this, 450 mL of DI water was added in aliquots to the mixture and the temperature was increased to 70 °C. After 20 minutes, 1.4 L of hot water and an aqueous solution of 25 mL of 30% H2O2 were all added. This resulted in the colour change of the mixture from yellow to dark brown, and this suspension was repeatedly washed with dil. HCl and water and dried at 50 °C under vacuum for 72 h. This resulted in the formation of a dark brown solid.
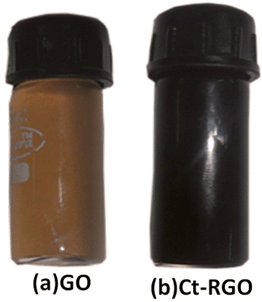 |
| Fig. 1 Photograph of (a) graphene oxide (GO) and (b) carrot reduced graphene oxide (Ct-RGO) in de-ionized water. | |
Preparation of carrot extract
Initially, the fresh carrots as purchased were washed in cold water three times. Next, carrot skins were peeled and the carrots were chopped into pieces. Then 20 g of carrot slices were mixed with 100 mL of de-ionized water, boiled at 100 °C for 50 min and filtered through Whatman 40 filter paper. The filtrate thus obtained contains a mixture of carotenoids namely β-carotene, lutein, and lycopene, which will act as antioxidants (reducing agents) for the reduction of GO (Scheme 1).
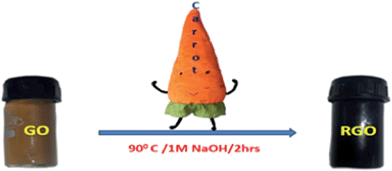 |
| Scheme 1 Schematic representing the reduction of GO by carrot. | |
Synthesis of reduced graphene oxide [ct-RGO]-a green approach
Initially, 2 mg of GO was mixed with 2 mL of de-ionized water (1 mg mL−1) and sonicated for 1 h. About 20 mL of this GO suspension was added to a flask containing 200 mL of 1 M NaOH and 100 mL of the carrot extract. The mixture was then stirred for 1 h at 90 °C. As the reaction proceeded, the yellowish brown colour mixture slowly turned to dark black within 1 h (Fig. 1). The mixture was kept under the same condition for an additional 1 h to allow the GO to be reduced completely. Finally, the mixture was cooled down to room temperature, repeatedly washed with de-ionized water to remove any impurities (if present) from the product and then dried in a vacuum.
Surface characterization
Initially, the UV-Visible spectra of GO and Ct-RGO were obtained to confirm the complete reduction of GO to RGO (Fig. 2). The results indicated that the value of λmax had shifted from 230 nm (at t = 0 min) to 270 nm (when t = 60 min), thereby confirming the effective reduction of the GO by carrot extract. However, we did not see any further shift in the λmax value after 60 min, which further confirms that the total reduction occurred within 1 h. The observed behaviour is in good agreement with the Marino et al. report that the maximum red shift value can be used as the yardstick to predict the performance of the reducing agent.27 Moreover, Ct-RGO displayed absorption at the same wavelength as that of graphene obtained by the reduction of hydrazine. In addition, the absorption wavelength is higher than that of the green reduced graphene oxide,22 which suggests that carrots are an efficient and effective reducing agent for the reduction of GO.
Next, FT-IR spectroscopy was used to investigate the extent of GO reduction (see Fig. 3). From Fig. 3, it is evident that GO had its characteristic bands at 1734 cm−1, 1179 cm−1, 1070 and 1011 cm−1, and 1629 cm−1 corresponding to COOH, –COOR, C–O–C, and C
C stretching, respectively. After the reduction of GO by carrot, the FT-IR spectra of Ct-RGO exhibited bands at 1094 cm−1 and 1641 cm−1 corresponding to aromatic (C
C stretching) and ether linkage (C–O–C stretching). The disappearance of bands between 1179 cm−1 and 1734 cm−1 indicated that the reduction resulted in the significant removal of –COOH and –COOR moieties. Further, the appearance of a new band at 1538 cm−1 is attributed to the restoration of aromatic rings. Upon comparison, it is evident that carrot extract is an effective reducing agent to convert GO to RGO.
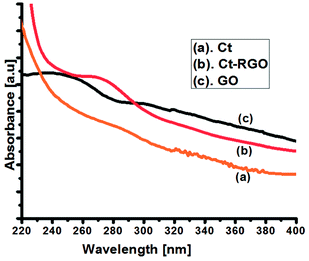 |
| Fig. 2 UV-Visible spectra of (a) carrot extract, (b) Ct-RGO and (c) GO. | |
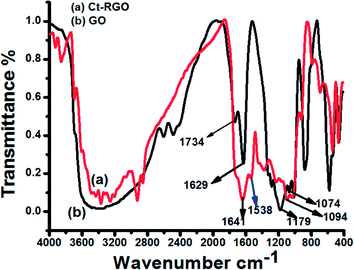 |
| Fig. 3 FT-IR spectra of (a) Ct-RGO and (b) GO. | |
Raman spectroscopy of GO and Ct-RGO was then performed and is shown in Fig. 4. As evident from Fig. 4, both GO and Ct-RGO showed the characteristic peaks at 1336 cm−1 and 1593 cm−1, corresponding to D and G bands, respectively. Further, the D/G ratio was calculated as 0.979 for GO and 1.198 for Ct-RGO. The increase in the D/G ratio in the Ct-RGO is due to the restoration of the sp2 network and the formation of the unrepaired defects after the removal of plenty of oxygen functionalities.30 A significant increase in the D/G ratio from 0.979 to 1.198 clearly indicated the reduction of GO by carrot extract. It is evident that the increase in the D/G ratio is an indication of the effective reduction of GO by carrot extract. Further, the appearance of a sharp 2D band in the Ct-RGO indicated the complete reduction of GO to RGO. Further evidence to support the formation of RGO was the appearance of the characteristic peak at 26.5°, which is absent in GO (see Fig. 5).
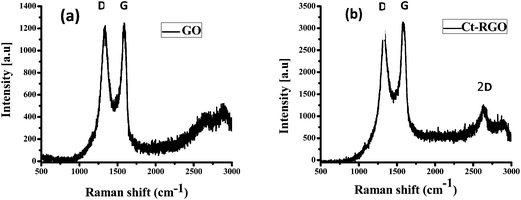 |
| Fig. 4 Raman spectra of (a) GO and (b) Ct-RGO. | |
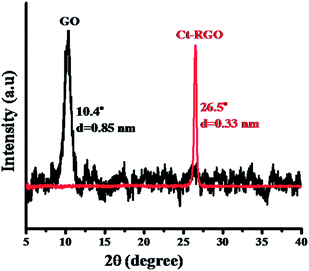 |
| Fig. 5 X-ray diffraction patterns of GO and RGO. | |
Fig. 6(a) is a representative image of GO in which we noticed a clear layer-by-layer stacking of GO. On the other hand, Fig. 6(b) shows an SEM image of Ct-RGO, in which we can clearly see the isolation of the graphene layers.
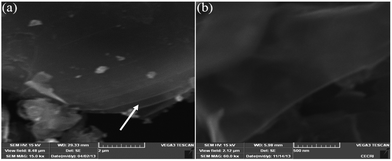 |
| Fig. 6 SEM images of (a) GO and (b) Ct-RGO. | |
Fig. 7(a) shows the SAED patterns of the Ct-RGO, showing only one set of hexagonal diffraction patterns and a single crystalline lattice structure, which is in agreement with the Wang et al. report.19 Fig. 7(b) is a TEM image of Ct-RGO, indicating a typical silky, transparent and rippled morphology due to its high aspect ratio. Following this a more detailed C/O ratio analysis was performed using X-ray photoelectron spectroscopy. In this analysis, we witnessed a C/O ratio of 5.38 in GO whereas the C/O ratio in RGO was tremendously increased to 18.8 (see Fig. 8). A tremendous increase in this C/O ratio is due to the effective decrease in the oxygen content as a result of successful reduction of carboxyl and ketonic groups by the anti-oxidants present in the juice.
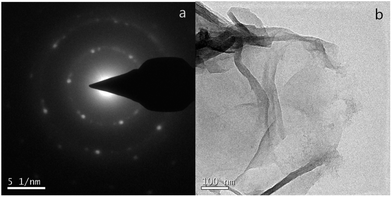 |
| Fig. 7 (a) SAED pattern of Ct-RGO and (b) TEM image of Ct-RGO. | |
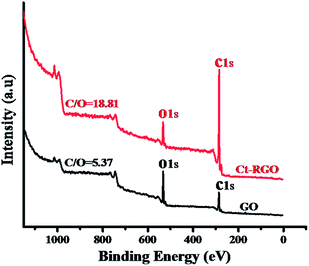 |
| Fig. 8 XPS of GO and ct-RGO. | |
AFM measurements were then conducted to calculate the thickness of the GO and Ct-RGO. Based on our experiments, we have calculated the thickness of GO and Ct-RGO to be 1.1 nm and 0.8 nm, respectively (Fig. 9). Following this, electrochemical impedance spectroscopy (EIS) was conducted at GC, GC/GO and GC/Ct-RGO in 10 mM K4[Fe(CN)6]/K3[Fe(CN)6] in 0.1 M KCl, and the corresponding Nyquist plots were constructed (Fig. 10). From the Nyquist plots, it is evident that the semicircle of the GO is greater than that of GC, thereby indicating the difficulty experienced by the interfacial charge transfer due to the semi-conducting property of GO. On the other hand, the absence of a semicircular portion in the Nyquist plot corresponding to Ct-RGO indicates that the rapid electron transfer between the [Fe(CN)6]3−/4− and the electrode is facilitated. From the comparison, it is evident that the conductivity of the Ct-RGO is greater than that of GO, and this is due to the restoration of the graphitic nature (i.e. sp2 bonds) in Ct-RGO.
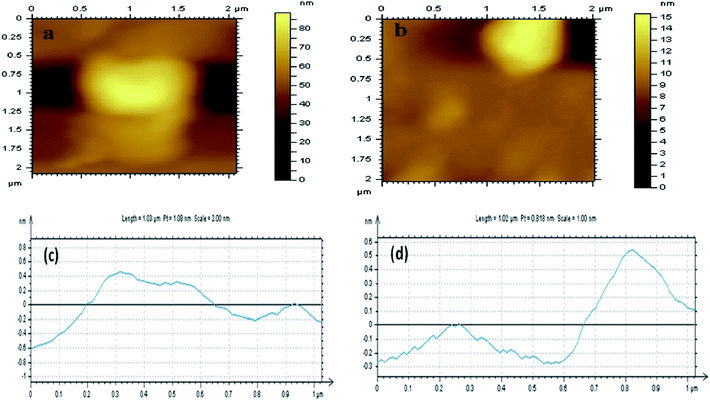 |
| Fig. 9 AFM images of (a) GO and (b) Ct-RGO and surface profiles of (c) GO and (d) Ct-RGO. | |
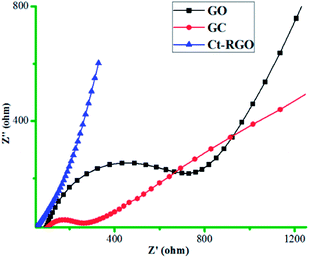 |
| Fig. 10 Electrochemical impedance spectra (EIS) of bare GC, GC/GO and GC/Ct-RGO in 1 : 1 ratio of 10 mM K4[Fe(CN)6]/K3[Fe(CN)6] in 0.1 M KCl. | |
Conclusion
In summary, for the first time we report a green, simple, facile and cost effective strategy for direct reduction of GO by a vegetable extract. A stable dispersion of graphene can be readily prepared by the reduction of GO by carrot extract. Ct-RGO obtained in this work is expected to be a biocompatible product and will find several potential applications in various fields such as drug delivery, biocomposites and biosensors.
Acknowledgements
CSR Vusa thanks UGC for JRF support and CIF CSIR-CECRI for characterization facilities. SB thanks network project no. CSC0134, and SA thanks OLP0088 for financial assistance.
References
- S. Stankovich, D. A. Dikin, G. H. B. Dommett, K. M. Kohlhaas, E. J. Zimney, E. A. Stach, R. D. Piner, S. T. Nguyen and R. S. Ruoff, Nature, 2006, 442, 282 CrossRef CAS PubMed.
- D. A. Dikin, S. Stankovich, E. J. Zimney, R. D. Piner, G. H. B. Dommett, G. Evmenenko, S. T. Nguyen and R. S. Ruoff, Nature, 2007, 448, 457 CrossRef CAS PubMed.
- X. Wang, L. Zhi and K. Mullen, Nano Lett., 2007, 8, 323 CrossRef PubMed.
- L. J. Cote, F. Kim and J. Huang, J. Am. Chem. Soc., 2008, 131, 1043 CrossRef PubMed.
- C. Berger, Z. Song, X. Li, X. Wu, N. Brown, C. c. Naud, D. Mayou, T. Li, J. Hass, A. N. Marchenkov, E. H. Conrad, P. N. First and W. A. de Heer, Science, 2006, 312, 1191 CrossRef CAS PubMed.
- P. W. Sutter, J.-I. Flege and E. A. Sutter, Nat. Mater., 2008, 7, 406 CrossRef CAS PubMed.
- Y. Pan, H. Zhang, D. Shi, J. Sun, S. Du, F. Liu and H.-j. Gao, Adv. Mater., 2009, 21, 2777 CrossRef CAS.
- L. Jiao, L. Zhang, X. Wang, G. Diankov and H. Dai, Nature, 2009, 458, 877 CrossRef CAS PubMed.
- D. V. Kosynkin, A. L. Higginbotham, A. Sinitskii, J. R. Lomeda, A. Dimiev, B. K. Price and J. M. Tour, Nature, 2009, 458, 872 CrossRef CAS PubMed.
- Y. Hernandez, V. Nicolosi, M. Lotya, F. M. Blighe, Z. Sun, S. De, I. T. McGovern, B. Holland, M. Byrne, Y. K. Gun'Ko, J. J. Boland, P. Niraj, G. Duesberg, S. Krishnamurthy, R. Goodhue, J. Hutchison, V. Scardaci, A. C. Ferrari and J. N. Coleman, Nat. Nanotechnol., 2008, 3, 563 CrossRef CAS PubMed.
- M. Lotya, Y. Hernandez, P. J. King, R. J. Smith, V. Nicolosi, L. S. Karlsson, F. M. Blighe, S. De, Z. Wang, I. T. McGovern, G. S. Duesberg and J. N. Coleman, J. Am. Chem. Soc., 2009, 131, 3611 CrossRef CAS PubMed.
- M. Eizenberg and J. M. Blakely, Surf. Sci., 1979, 82, 228 CrossRef CAS.
- T. Aizawa, R. Souda, S. Otani, Y. Ishizawa and C. Oshima, Phys. Rev. Lett., 1990, 64, 768 CrossRef CAS.
- K. S. Kim, Y. Zhao, H. Jang, S. Y. Lee, J. M. Kim, K. S. Kim, J.-H. Ahn, P. Kim, J.-Y. Choi and B. H. Hong, Nature, 2009, 457, 706 CrossRef CAS PubMed.
- K. S. Novoselov, A. K. Geim, S. V. Morozov, D. Jiang, Y. Zhang, S. V. Dubonos, I. V. Grigorieva and A. A. Firsov, Science, 2004, 306, 666 CrossRef CAS PubMed.
- L. Xuekun, Y. Minfeng, H. Hui and S. R. Rodney, Nanotechnology, 1999, 10, 269 CrossRef.
- K. S. Novoselov, D. Jiang, F. Schedin, T. J. Booth, V. V. Khotkevich, S. V. Morozov and A. K. Geim, Proc. Natl. Acad. Sci. U. S. A., 2005, 102, 10451 CrossRef CAS PubMed.
- S. Park and R. S. Ruoff, Nat. Nanotechnol., 2009, 4, 217 CrossRef CAS PubMed.
- H. Wang, D. Zhang, T. Yan, X. Wen, L. Shi and J. Zhang, J. Mater. Chem., 2012, 22, 23745 RSC.
- H. Wang, D. Zhang, T. Yan, X. Wen, J. Zhang, L. Shi and Q. Zhong, J. Mater. Chem. A, 2013, 1, 11778 CAS.
- J. Gao, F. Liu, Y. Liu, N. Ma, Z. Wang and X. Zhang, Chem. Mater., 2010, 22, 2213 CrossRef CAS.
- C. Zhu, S. Guo, Y. Fang and S. Dong, ACS Nano, 2010, 4, 2429 CrossRef CAS PubMed.
- J. Liu, S. Fu, B. Yuan, Y. Li and Z. Deng, J. Am. Chem. Soc., 2010, 132, 7279 CrossRef CAS PubMed.
- V. Dua, S. P. Surwade, S. Ammu, S. R. Agnihotra, S. Jain, K. E. Roberts, S. Park, R. S. Ruoff and S. K. Manohar, Angew. Chem., Int. Ed., 2010, 49, 2154 CrossRef CAS PubMed.
- M. Fang, J. Long, W. Zhao, L. Wang and G. Chen, Langmuir, 2010, 26, 16771 CrossRef CAS PubMed.
- J. Zhang, H. Yang, G. Shen, P. Cheng, J. Zhang and S. Guo, Chem. Commun., 2010, 46, 1112 RSC.
- M. J. Fernández-Merino, L. Guardia, J. I. Paredes, S. Villar-Rodil, P. Solís-Fernández, A. Martínez-Alonso and J. M. D. Tascón, J. Phys. Chem. C, 2010, 114, 6426 Search PubMed.
- N. I. Krinsky, J. T. Landrum and R. A. Bone, Annu. Rev. Nutr., 2003, 23, 171 CrossRef CAS PubMed.
- M. Carail and C. C. Veyrat, Pure Appl. Chem., 2006, 78, 1493 CrossRef CAS.
- L. G. Rao, E. Guns and A. V. Rao, Lycopene: Its role in human health and disease, Calcium Research Laboratory, St. Michael's Hospital and Department of Medicine, University of Toronto, Toronto, Ontario, Canada, 2003 Search PubMed.
- D. Heber and Q.-Y. Lu, Exp. Biol. Med., 2002, 227, 920 CAS.
- G. W. Burton and K. U. Ingold, Science, 1984, 224, 569 CAS.
- W. S. Hummers and R. E. Offeman, J. Am. Chem. Soc., 1958, 80, 1339 CrossRef CAS.
|
This journal is © The Royal Society of Chemistry 2014 |