DOI:
10.1039/C4RA00201F
(Paper)
RSC Adv., 2014,
4, 14527-14537
Ring-opening polymerization of cyclic esters initiated by zirconium, titanium and yttrium complexes†
Received
2nd September 2013
, Accepted 3rd February 2014
First published on 3rd February 2014
Abstract
A series of dinuclear zirconium [LZr(OiPr)2]2[(μ-OiPr)2] (1–3) as well as mononuclear titanium [LTi(OiPr)2] (4), yttrium [LYCl] (5) and zirconium [LZr(OiPr)2] (6–7) salen-type complexes (L = dianionic [ONNO]-tetradentate ancillary ligand) have been prepared and characterized. Dinuclear zirconium salen complexes [LZr(OiPr)2]2[(μ-OiPr)2] (1–3) have shown effective activity toward the ring opening polymerization of lactides and ε-caprolactone. However, mononuclear titanium, yttrium and zirconium complexes 4–7 are almost inactive for the ROP of L-lactide. Solvent-free bulk polymerization of L-lactide initiated by dinuclear zirconium complex 2 with a conversion >90% can be achieved within 10 min, yielding high molecular weight polylactide with low polydispersity index. Kinetic studies reveal the ROP of L-lactide initiated by dinuclear zirconium complex 3 has a first-order dependency on [LA].
Introduction
Due to their unique biocompatible and biodegradable properties, polyesters such as polylactides (PLAs) and poly(ε-caprolactone) (PCL) have been intensively used in many applications, ranging from packing to biomedical devices.1 Polyesters can be prepared by direct polycondensation of acids or by ring-opening polymerization (ROP) of cyclic esters using a suitable homogeneous catalyst.2 The most common and effective method for preparation of polyesters is ring-opening polymerization of lactides (LAs) and lactones using various metal complexes as catalysts/initiators. Over the past decades, numerous metal complexes supported by a variety of ligands have been used as catalysts/initiators for the ROP of cyclic esters.3–8 Most of them show efficient activity in the ROP of cyclic esters in toluene or CH2Cl2. However, for environmental and biomedical purposes, the most desirable features of catalysts for lactides’ polymerization are the compounds with high activity, low toxicity and the ability to produce high molecular weight polymers with low polydispersity in the absence of any organic solvent.
Because of their diverse forms and ease of preparation, many Schiff-base supported metal complexes have been widely used as catalysts in many applications.9 Geometrically more rigid salen-type ligands are also readily prepared and their metal complexes are widely used in many applications such as organic syntheses,10 and olefin polymerization.11 Recently, metal salen complexes have been widely used in ring-opening polymerization. For instance, aluminum salen complexes have shown highly stereocatalytic activity toward the ROP of rac-lactide.12 Group 4 metal salen complexes are also reported to be active in the ROP of cyclic esters. Recently, it has been reported that the activities of group 4 metal complexes in lactide polymerization are dramatically affected by the identity of the metal and the substituents of the ligands.13 We report herein the preparation of a series of zirconium, titanium and yttrium salen-type complexes and their activities toward the ROP of lactides and ε-caprolactones.
Experimental section
General methods and materials
All manipulations were carried out under a dry nitrogen atmosphere. Solvents were dried by refluxing for at least 24 h over sodium/benzophenone (hexane, toluene, and tetrahydrofuran (THF)), phosphorus pentaoxide (CH2Cl2), or calcium hydride (benzyl alcohol). Deuterated solvents (Aldrich, Merck) were dried over molecular sieves. L-Lactide was purchased from the Bio Invigor Corporation and recrystallized from a toluene solution prior to use. Other regents were purchased from Aldrich or Acros and used without further purification.
Measurement
1H and 13C NMR spectra were recorded on a Varian Unity Inova-600 (600 MHz for 1H and 150 MHz for 13C) or a Varian Mercury-400 (400 MHz for 1H and 100 MHz for 13C) spectrometer with chemical shifts given in ppm from the internal TMS, toluene, or center line of CHCl3. The GPC measurements were performed on a Jasco PU-2080 HPLC pump system equipped with a Jasco RI-2031 Plus detector using THF (HPLC grade) as an eluent. The chromatographic column was a Jordi 15022 column (250 mm) and the calibration curve was made by polystyrene standards to calculate Mn (GPC). 3,5-Bis(α,α-dimethylbenzyl)-2-hydroxybenzaldehyde and N,N′-3,5-bis(α,α-dimethylbenzyl)-2-hydroxysalicylidene-2,2-dimethyl-1,3-diamine (L1H) were prepared according to the method described in the literature.14
Preparation of N,N′-3,5-bis(α,α-dimethylbenzyl)-2-hydroxysalicylidene-1,2-phenylenediamine (L2H2)
L2H2 was prepared according to the procedure of L1H. Yield: 3.57 g (86%).1H NMR (CDCl3, 400 MHz, ppm): δ 13.21 (s, OH, 2H), 8.18 (s, N
CH, 2H), 7.30–6.98 (m, Ar–H, 28H), 3.66 (s, CH2C(CH3)2CH2, 4H), 1.68 (s, CH3, 24H). 13C NMR (CDCl3, 100 MHz, ppm): δ 164.5 (ArC
N), 157.9 (ArCOH), 150.6, 150.3 (ArCC(CH3)2), 142.4 (ArCN–C), 139.8, 136.4 (ArC-cumyl), 130.2, 128.6, 128.0, 127.7, 127.1, 126.7, 125.7, 125.6, 125.0 (Ar–C), 118.4 (ArCC
N), 42.4, 42.4, 30.8, 29.3 (C(CH3)2) Anal. calcd for C52H56N2O2: C, 84.28; H, 7.62; N, 3.78%. Found: C, 84.40; H, 7.31; N, 3.47%.
Preparation of N,N′-3,5-bis(α,α-dimethylbenzyl)-2-hydroxysalicylidene-1,2-ethylenediamine (L3H2)
L3H2 was prepared according to the procedure of L1H. Yield: 3.30 g (85%). 1H NMR (CDCl3, 400 MHz, ppm): δ 12.96 (s, OH, 2H), 8.42 (s, N
CH, 2H), 7.30–7.10 (m, Ar–H, 28H), 1.68 (s, CH3, 24H). 13C NMR (CDCl3, 100 MHz, ppm): δ 166.8 (ArC
N), 157.6 (ArCOH), 150.6, 150.5 (ArC–C(CH3)2), 139.3, 135.9 (ArC-cumyl), 129.0, 127.9, 127.7, 126.6, 125.5, 124.9 (Ar–C), 117.8 (ArCC
N), 59.3 (NCH2), 42.4, 42.0, 30.8, 29.3 (C(CH3)2). Anal. calcd for C56H56N2O2: C, 85.24; H, 7.15; N, 3.55%. Found: C, 85.40; H, 6.91; N, 3.07%.
General procedures for the preparation of [LZr(OiPr)2]2[(μ-OiPr)2]
A solution of Zr(OiPr)4·iPrOH (4.00 mmol) in toluene (25 mL) was cooled to −78 °C and a solution of LH2 (2.00 mmol) in toluene (25 mL) was then added slowly. After LH2 was added, the mixture was stirred at 25 °C for 24 h. Volatile materials were removed under vacuum and the residue was recrystallized from toluene.
[L1Zr(OiPr)2]2[(μ-OiPr)2] (1)
Yield: 1.61 g (61%). 1H NMR (CDCl3, 600 MHz, ppm): δ 7.74 (s, N
CH, 1H), 7.25–7.13 (m, Ar–H, 12H), 7.04–6.92 (m, Ar–H, 12H), 4.42 (m, OCH(CH3)2), 4.06 (sept, OCH(CH3)2, 2H, J = 6.0 Hz), 2.87 (d, CH2C(CH3)2CH2, 4H, J = 9.6 Hz), 1.74–1.61 (m, C(CH3)2Ph, 12H), 1.29–1.17 (m, C(CH3)2, 12H), 0.92 (s, C(CH3)2, 3H), 0.85–0.76 (d, C(CH3)2, 6H), 0.50 (s, C(CH3)2, 3H). 13C NMR (CDCl3, 150 MHz, ppm): δ 166.3 (ArC
N), 151.1, 151.0 (ArC–O), 138.6, 137.8, 136.7, 131.6, 129.0, 128.2, 127.8, 127.6, 127.1, 126.7, 126.0, 125.3, 125.2, 124.2, 121.88 (Ar–C), 72.42, 71.9, 70.8, 70.6, 70.2, 69.0 (C(CH3)2), 42.4, 42.3, 42.2 (C(CH3)2Ph), 36.8, 30.8, 30.7 (C(CH3)2), 27.0, 26.8, 26.5, 26.4, 26.2, 26.1, 25.5, 24.3, 21.4 (C(CH3)2). Calc. for C74H96N2O8Zr2: C, 66.52; H, 7.80; N, 2.13%. Found: C, 66.13; H, 8.70; N, 2.70%.
[L2Zr(OiPr)2]2[(μ-OiPr)2](2)
Yield: 1.77 g (67%). 1H NMR (CDCl3, 600 MHz, ppm): δ 7.92 (s, N
CH, 1H), 7.83 (s, N
CH, 1H), 7.24–7.22 (m, Ar–H, 5H), 7.18–7.10 (m, Ar–H, 10H), 7.07–7.06 (m, Ar–H, 3H), 6.93–6.92 (m, Ar–H, 3H), 6.89–6.88 (m, Ar–H, 2H), 6.83–6.82 (m, Ar–H, 1H), 6.79 (Ar–H, 1H), 6.63 (Ar–H, 1H), 4.53 (sept, OCH(CH3)2, 1H, J = 6.0 Hz), 4.41 (sept, OCH(CH3)2, 2H, J = 6.0 Hz), 4.11 (sept, OCH(CH3)2, 2H, J = 6.0 Hz), 3.47 (sept, OCH(CH3)2, 2H, J = 6.0 Hz), 1.88 (s, C(CH3)2, 3H), 1.62 (s, C(CH3)2, 9H), 1.46 (s, C(CH3)2, 6H), 1.24–1.19 (m, C(CH3)2, 18H), 1.10 (s, C(CH3)2, 6H), 0.95 (d, C(CH3)2, 3H, J = 6.0 Hz), 0.83 (d, C(CH3)2, 3H, J = 6.0 Hz), 0.75 (m, C(CH3)2, 3H, J = 6.0 Hz), 0.31 (m, C(CH3)2, 3H, J = 6.0 Hz), 13C NMR (CDCl3, 150 MHz, ppm): δ 169.5 (ArC
N), 163.4, 162.9, 161.1 (ArC–O), 151.0, 150.9, 150.4, 147.8, 146.5, 138.0, 137.64, 137.2, 136.5, 136.4, 134.0, 131.3, 131.0, 129.0, 128.2, 127.8, 127.7, 127.5, 127.4, 126.6, 126.5, 126.4, 126.0, 125.4, 125.2, 124.6, 122.2, 122.1, 120.0 (Ar–C), 71.7, 71.1, 70.4, 69.0, 68.7 (C(CH3)2), 42.6, 42.18, 41.82 (C(CH3)2), 31.9, 30.7, 30.5, 28.6, 28.0 (C(CH3)2), 26.9, 26.8, 26.7, 26.1, 24.2, 23.2 (C(CH3)2). Calc. for C74H96N2O8Zr2: C, 67.13; H, 7.31; N, 2.12%. Found: C, 66.47; H, 8.26; N, 2.29%.
[L3Zr(OiPr)2]2[(μ-OiPr)2] (3)
Yield: 1.61 g (63%). 1H NMR (CDCl3, 600 MHz, ppm): δ 7.84 (s, N
CH, 2H), 7.33 (Ar–H, 2H), 7.26–7.21 (m, Ar–H, 7H), 7.17–7.12 (m, Ar–H, 7H), 7.04–7.02 (m, Ar–H, 4H), 6.93 (Ar–H, 4H), 4.45 (sept, OCH(CH3)2, 2H, J = 6.0 Hz), 4.10 (d, CH2CH2, 2H, J = 12 Hz), 3.91 (sept, OCH(CH3)2, 2H, J = 6.0 Hz), 3.83 (sept, OCH(CH3)2, 2H, J = 6 Hz), 3.48 (d, CH2CH2, 2H, J = 1.8 Hz), 2.00 (s, C(CH3)2, 6H), 1.67 (s, C(CH3)2, 9H), 1.49 (s, C(CH3)2, 3H), 1.24 (m, C(CH3)2, 12H), 0.99 (d, C(CH3)2, 6H, J = 6.6 Hz), 0.78 (d, C(CH3)2, 6H, J = 6.0 Hz), 0.66 (d, C(CH3)2, 6H, J = 6.6 Hz), 0.62 (m, C(CH3)2, 6H). 13C NMR (CDCl3, 150 MHz, ppm): δ = 167.4 (ArC
N), 160.9 (ArCO), 151.5, 151.3, 137.9, 136.9, 131.6, 130.3, 129.0, 128.2, 127.8, 127.6, 126.7, 126.1, 125.8, 125.3, 125.2, 124.6, 121.6 (Ar–C), 70.8, 70.6, 69.7 (C(CH3)2), 63.6 (CH2), 42.5 (C(CH3)2Ph), 33.4, 30.9, 30.8, 26.7 (C(CH3)2), 26.6, 26.5, 26.4, 26.0, 24.6, 24.3 (C(CH3)2). Anal. calcd for C70H96N2O8Zr2: C, 66.00; H, 7.44; N, 2.20%. Found: C, 66.58; H, 7.58; N, 1.89%.
Preparation of [L3Ti(OiPr)2] (4)
A solution of L3H2 (1.48 g, 2.00 mmol) in THF (25 mL) was added slowly into a solution of Ti(OiPr)4 (0.57 g, 2.00 mmol) in THF (25 mL). The mixture was then refluxed under a N2 atmosphere for 18 h and cooled to 0 °C. Hexane (25 mL) was added to the solution yielding a yellow solid. The solid was collected by filtration. Yield: 1.03 g (57%). 1H NMR (CDCl3, 600 MHz, ppm): δ 8.11 (s, N
CH, 2H), 7.32–7.02 (m, Ar–H, 24H), 3.76 (s, CH2CH2, 4H), 3.58 (m, OCH(CH3)2, 2H), 1.72 (s, PhC(CH3)2, 24H), 0.36 (m, OCH(CH3)2, 12H). 13C NMR (CDCl3, 150 MHz, ppm): δ 163.4, 163.1 (ArC
N), 151.1, 151.0 (ArC–O), 137.0, 136.8, 132.1, 129.6, 127.8, 127.6, 126.7, 126.0, 125.4, 124.6, 121.7 (Ar–C), 72.4 (C(CH3)2), 58.5, 42.7, 42.2 (C(CH3)2Ph), 30.8, 29.7 (C(CH3)2), 25.5 (OCH(CH3)2). Anal. calcd for C58H68N2O4Ti: C, 76.97; H, 7.57; N, 3.10%. Found: C, 77.12; H, 6.89; N, 2.99%.
Preparation of [L2Na2]
Sodium bis(trimethylsilyl)amide (0.81 g, 4.40 mmol) in THF (25 mL) was added slowly to an ice cold solution of N,N′-3,5-bis(α,α-dimethylbenzyl)-2-hydroxysalicylidene-1,2-benzenediamine (L2H2) (1.54 g, 2.00 mmol) in THF (25 mL). The mixture was stirred at 25 °C for 3 h and hexane (30 mL) was added yielding a yellow solid. The solid was filtered and dried under vacuum. Yield: 1.18 g (71%).
[L2YCl] (5)
A mixture of [L2Na2] (0.83 g, 1.00 mmol) and YCl3 (0.195 g, 1.00 mmol) in ice cold THF (25 mL) was stirred for 12 h during which the temperature was raised to 25 °C. The mixture was then filtered and the filtrate was dried under vacuum. 1H NMR (CDCl3, 400 MHz, ppm): δ 8.35 (s, N
CHAr, 2H), 7.34–7.13 (m, ArH, 30H), 1.77 (s, C(CH3)2, 6H), 1.67 (s, C(CH3)2, 12H), 1.39 (s, C(CH3)2, 6H), 13C NMR (CDCl3, 100 MHz, ppm): δ 165.7 (ArC
N), 163.6 (ArCO), 151.6, 150.9 (ArCC(CH3)2), 144.9 (ArCN
C), 138.5 (ArC-cumyl), 136.6 (ArC-cumyl), 131.4, 129.0, 128.2, 128.0, 127.9, 127.4, 126.7, 126.2, 125.8, 125.4, 125.3, 124.8, 121.9 (Ar–C), 117.9 (ArCC
N), 43.0, 42.2 (C(CH3)2), 32.2, 30.8 (C(CH3)2).
Synthesis of L4H2
A mixture of 4-benzoyl-3-methyl-1-phenyl-pyrazol-5(4H)-one (5.56 g, 20.0 mmol) and 2,2-dimethylpropane-1,3-diamine (1.2 mL, 10.0 mmol) was stirred in refluxing ethanol (30 mL) for 24 h. While stirring, a yellow precipitate was observed. The mixture was cooled to 25 °C and the resulting precipitate was collected by filtration and the solid was dried under vacuum. Yield: 5.16 (83%). 1H NMR (CDCl3, 400 MHz, ppm): δ = 11.38 (s, OH, 2H), 7.99 (Ar–H, 2H), 7.46 (m, Ar–H, 6H), 7.40 (t, Ar–H, 4H), 7.22 (m, Ar–H, 4H), 7.15 (t, Ar–H, 2H), 3.02 (d, J = 6 Hz, NCH2C(CH3)2, 4H), 1.45 (s, CH3C
N, 6H), 1.06 (s, C(CH3)2, 6H), 13C NMR (CDCl3, 100 MHz, ppm): δ 166.0 (
CNH), 165.6 (C
O), 147.7, 139.0, 130.8, 130.5, 129.1, 128.7, 127.4, 124.3, 119.2 (Ar–C), 100.0 (C
CC), 51.9 (NHCH2), 35.9 (C(CH3)2), 23.4 (C(CH3)2), 15.3 (CCH3).
Synthesis of [L4Zr(OiPr)2] (6)
A mixture of L4H2 (1.0 mmol, 0.622 g) and Zr(OiPr)2.iPrOH (1.5 mmol, 0.58 g) was stirred in toluene (25 mL) at 60 °C for 12 h and was then cooled to 25 °C. The volatile materials were removed under vacuum giving a white powder. The solid was washed with hexane (30 mL) and then filtered. The resulting precipitate was then dried in vacuo to give a white solid. Yield: 0.55 g (67%). 1H NMR (−40 °C, CDCl3, ppm): δ 8.02 (2H, Ar–H), 7.69 (2H, Ar–H), 7.56–7.44 (m. 6H, Ar–H), 7.37 (t, 2H, Ar–H), 7.30–7.18 (m, 5H, Ar–H), 6.86–6.79 (m, 3H, Ar–H), 4.50 (sept, J = 6 Hz, CH(CH3)2, 1H), 4.16 (sept, J = 6 Hz, CH(CH3)2 1H), 4.08 (d, J = 12 Hz, CH2C(CH3)2, 1H), 3.74 (d, J = 12.8 Hz, CH2C(CH3)2, 1H), 3.47 (d, J = 12 Hz, CH2C(CH3)2, 1H), 3.27 (d, J = 12.8 Hz, CH2C(CH3)2, 1H), 1.38 (s, 3H, C(CH3)2), 1.32 (s, 3H, C(CH3)2), 1.33–1.29 (m, 6H, OC(CH3)3), 0.95–0.89 (two doublet, J1 = 6.0 Hz, J2 = 7.2 Hz, OC(CH3)3, 6H), 0.75 (s, 3H, CH3C
N), 0.61 (s, 3H, CH3C
N). Anal. calcd for C45H50N6O4Zr: C, 65.11; H, 6.07; N, 10.12%. Found: C, 65.78; H, 6.05; N, 9.91%.
Syntheses L5H2
A mixture of 4-benzoyl-1-(4-chlorophenyl)-3-methyl-pyrazol-5(4H)-one (6.24 g, 20.0 mmol) and 2,2-dimethylpropane-1,3-diamine (1.2 mL, 10.0 mmol) was stirred in refluxing ethanol (30 mL) for 24 h. While stirring, a yellow precipitate was observed. The mixture was cooled to 25 °C and the resulting precipitate was collected by filtration and the solid was dried under vacuum. Yield: 4.97 (72%). 1H NMR (CDCl3, 400 MHz, ppm): δ 11.30 (s, OH, 2H), 7.96 (Ar–H, 4H), 7.48 (m, Ar–H, 6H), 7.35 (m, Ar–H, 4H), 7.20 (m, Ar–H, 4H), 3.03 (d, J = 5.6 Hz, NCH2C(CH3)2, 4H), 1.42 (s, CH3C
N, 6H), 1.05 (s, C(CH3)2, 6H). 13C NMR (CDCl3, 100 MHz, ppm): δ 166.0 (
CNH), 165.7 (C
O), 148.0, 137.6, 130.7, 130.6, 129.2, 129.1, 128.7, 127.4, 120.1 (ArC), 99.9 (C
CC), 52.0 (NHCH2), 35.9 (C(CH3)2), 23.4 (C(CH3)2), 15.3 (CCH3). Anal. calcd for C39H36Cl2N6O2: C, 67.72; H, 5.25; N, 12.15%. Found: C, 67.16; H, 4.61; N, 11.50%.
Synthesis of [L5Zr(OiPr)2] (7)
A mixture of L5H2 (1.0 mmol, 0.692 g) and Zr(OiPr)2.iPrOH (1.5 mmol, 0.58 g) was stirred in toluene (25 mL) at 60 °C for 12 h and was then cooled to 25 °C. The volatile materials were removed under vacuum giving a white powder. The solid was washed with hexane (30 mL) and then filtered. The resulting precipitate was then dried in vacuo to give a white solid. Yield: 0.67 g (75%). 1H NMR (−40 °C, CDCl3, 400 MHz, ppm): δ 7.95 (Ar–H, 2H), 7.56–7.46 (m, Ar–H, 8H), 7.24–7.20 (m, Ar–H, 6H), 6.85 (Ar–H, 2H), 4.47 (sept, J = 6 Hz, CH(CH3)2, 1H), 4.12 (sept, J = 5.2 Hz, CH(CH3)2, 1H), 4.04 (d, J = 12 Hz, CH2C(CH3)2, 1H), 3.75 (d, J = 13.6 Hz, CH2C(CH3)2, 1H), 3.51 (d, J = 12 Hz, CH2C(CH3)2, 1H), 3.29 (d, J = 13.6 Hz, CH2C(CH3)2, 1H), 1.37 (s, C(CH3)2, 3H), 1.35 (s, C(CH3)2, 3H), 1.33–1.30 (m, OCH(CH3)2, 6H), 0.90–0.86 (m, OCH(CH3)2, 6H), 0.78 (s, CH3C
N, 3H), 0.62 (s, CH3C
N, 3H). Anal. calcd for C45H48Cl2N6O4Zr: C, 60.12; H, 5.38; N, 9.35%. Found: C, 59.94; H, 5.20; N, 8.97%.
Typical general procedures for polymerization of ε-caprolactone
A typical polymerization procedure was exemplified by the synthesis of PCL-50 (the number 50 indicates the designed [LA]0/[complex]0) initiated by 1 at room temperature. The conversion of polymerization was analyzed by 1H NMR spectroscopic studies. Complex 1 (0.066 g, 0.05 mmol) was added to a solution of ε-caprolactone (0.28 mL, 2.5 mmol) in toluene (5.0 mL). The mixture was stirred at 80 °C for 5 min and was then quenched by the addition of isopropyl alcohol (IPA) (1.0 mL). The polymer was precipitated by pouring the above mixture into n-hexane (20 mL) to give a white solid. Yield: 0.46 g (80%).
Typical general procedures for polymerization of lactides
A typical polymerization procedure was exemplified by the synthesis of PLLA-50 (the number 50 indicates the designed [LA]0/[complex]0) initiated by 1 at room temperature. The conversion of polymerization was analyzed by 1H NMR spectroscopic studies. Complex 1 (0.066 g, 0.05 mmol) was added to a solution of L-lactide (0.36 g, 2.5 mmol) in toluene (5.0 mL). The mixture was stirred at 100 °C for 10 min and was then quenched by the addition of IPA (1.0 mL). The polymer was precipitated by pouring the above mixture into n-hexane (20 mL) to give a white crystalline solid. Yield: 0.62 g (85%).
Kinetic studies of the polymerization of lactides
Procedures for the kinetic studies were exemplified by the synthesis of PLLA-50 (the number 50 indicates the designed [LA]0/[complex]0) initiated by 3 at room temperature. The conversion of polymerization was analyzed by 1H NMR spectroscopic studies. Complex 3 ([3]0 = 1, 2, 3, 4 mM) was added to a solution of L-lactide (0.05 M) in toluene (1.0 mL). The mixture was stirred at 100 °C for 5 min. The conversion yield of PLLA was monitored after every minute by 1H NMR spectroscopic studies.
X-ray crystallographic studies
Single crystals suitable for X-ray single crystal structure determination were obtained from slowly cooling a toluene solution (complex 3) or from a toluene/hexane solution (complex 6). Suitable crystals were immersed with FOMBLIN®Y under a nitrogen atmosphere and mounted on an Oxford Gemini S diffractometer. Intensity data were collected in 1350 frames with increasing ω (width of 0.3° per frame). The absorption correction was based on the symmetry equivalent reflections using SADABS program.15 The space group determination was based on a check of the Laue symmetry and systematic absences, and was confirmed using the structure solution. The structure was solved by direct methods using a SHELXTL package.16 All non-H atoms were located from successive Fourier maps and hydrogen atoms were refined using a riding model. Anisotropic thermal parameters were used for all non-H atoms, and fixed isotropic parameters were used for the H atoms.
Results and discussion
Synthesis and structural characterization
The reactions of a series of sterically bulky salen ligands (L1H2-L3H2) with two equiv. of [Zr(OiPr)4·HOiPr] yield dinuclear zirconium complexes [L1Zr(OiPr)2]2[(μ-OiPr)2] (1), [L2Zr(OiPr)2]2[(μ-OiPr)2] (2) and [L3Zr(OiPr)2]2[(μ-OiPr)2] (3), respectively (Scheme 1). It is worth noting that no mononuclear species [LZr(OiPr)2] was observed even though the reaction of L1H2-L3H2 with a stoichiometric amount of Zr(OiPr)4·HOiPr was carried out. Instead, only bimetallic zirconium complexes 1–3 were obtained. However, L3H2 reacts with one equiv. of Ti(OiPr)4 giving a mononuclear titanium complex [L2Ti(OiPr)2] (4). Furthermore, treatment of L2H2 with a stoichiometric amount of Na[N(SiMe3)2] in tetrahydrofuran (THF), followed by the addition of YCl3 produces a mononuclear yttrium complex [L2YCl] (5). The reaction of 4-benzoyl-3-methyl-1-(4-R-phenyl-pyrazol-5(4H)-one (R = H or Cl)) with 2,2-dimethylpropane-1,3-diamine produces less sterically bulky salen-like ligands L4H2 and L5H2, respectively. Further reaction of L4H2 and L5H2 with a stoichiometric amount of [Zr(OiPr)4·HOiPr] gives mononuclear zirconium complexes [L4Zr(OiPr)2] (6) and [L5Zr(OiPr)2] (7), respectively (Scheme 2). All of these complexes have been characterized according to the integration ratio of protons in the salen-type ligand and isopropoxyl group from 1H NMR spectra as well as elemental analysis. The molecular structures of complexes 3 and 6 were further verified by X-ray structural studies. It is interesting to note that variable temperature dependent 1H NMR spectra (Fig. 1) of 6 reveal two inequivalent –OiPr groups at low temperature with two multiplets for methine protons (4.56 and 4.46 ppm). All of the four methylene protons are also inequivalent as four resonance doublets (4.11, 3.83, 3.50, and 3.29 ppm) were observed. However, they merge into one peak each for both methine (4.42 ppm) and methylene (3.58 ppm) protons at temperature >60 °C indicating fluxionality of this complex.
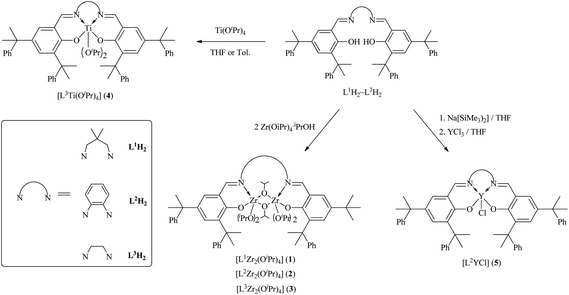 |
| Scheme 1 Preparation of complexes 1–5. | |
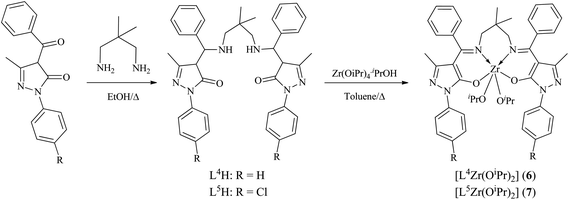 |
| Scheme 2 Preparation of complexes 6–7. | |
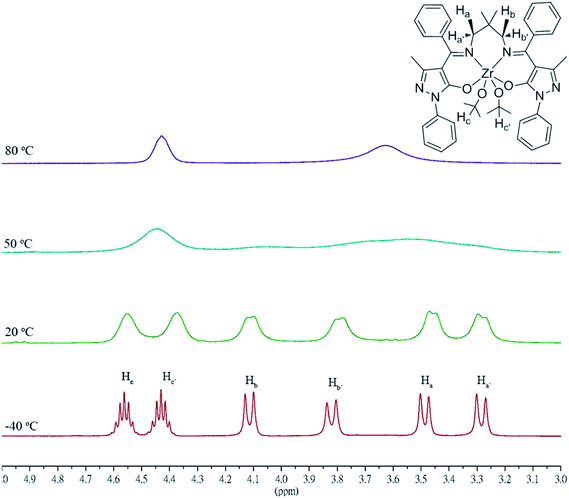 |
| Fig. 1 Variable temperature dependent 1H NMR spectra of 6. | |
Crystals of [L3Zr(OiPr)2]2[(μ-OiPr)2] (3) were obtained from slow cooling a toluene solution. The Oak Ridge Thermal Ellipsoid Plot (ORTEP) of the solid state molecular structure of 1 is shown in Fig. 2. The X-ray structure of 3 indicates that the complex is dinuclear in the solid state, resembling the zirconium salen complexes reported by Chakraborty et al.17 Each Zr center adopts a distorted octahedral geometry bridging through two –OiPr moieties. The two N centers from the salen ligand are coordinated to two different Zr centers. All bond lengths and angles are in agreement with similar zirconium complexes reported in the literature.17,18 Crystals suitable for X-ray structure studies of compound [L4Zr(OiPr)2] (6) were obtained from slow diffusion of hexane in a toluene solution and the solid state structure of complex 6 is shown in Fig. 3. The solid structure indicates that complex 6 is mononuclear with a distorted octahedral Zr center and two –OiPr moieties are inequivalent, which is consistent with the result observed in the solution.
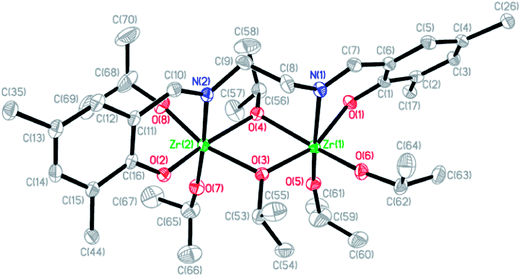 |
| Fig. 2 Molecular structure of 3. Thermal ellipsoids are drawn at the 50% probability level. The methyl carbon of the tert-butyl groups and all H atoms are omitted for clarity. Selected bond lengths (Å) and angles (deg.): Zr1–O1 2.067(2), Zr1–O3 2.146(2), Zr1–O4 2.205(2), Zr1–O5 1.936(2), Zr1–O6 1.930(2), Zr1–N1 2.422(2), Zr2–O2 2.068(2), Zr2–O3 2.200(2), Zr2–O4 2.148(2), Zr2–O7 1.925(2), Zr2–O8 1.937(2), Zr2–N2 2.427(2); O1–Zr1–O3 158.2(1), O4–Zr1–O6 161.8(3), N1–Zr1–O5 171.9(1), O2–Zr2–O4 157.4(1), O3–Zr2–O8 163.0(1), N2–Zr2–O7 171.6(1). | |
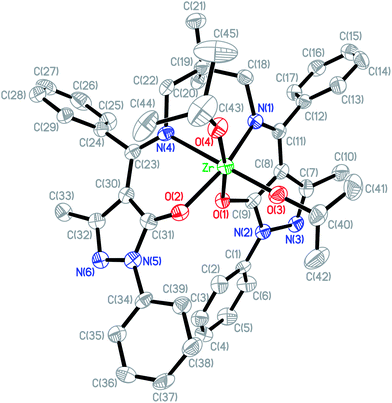 |
| Fig. 3 Molecular structure of 6. Thermal ellipsoids are drawn at the 50% probability level and H atoms omitted for clarity. Selected bond lengths (Å) and angles (deg.): Zr–O1 2.187(4), Zr–O2 2.088(4), Zr–O3 1.930(4), Zr–O4 1.932(4), Zr–N1 2.272(5), Zr–N4 2.374(5); O1–Zr–O4 168.7(2), O2–Zr–N1 153.7(2), O3–Zr–N4 172.9(2), C40–O3–Zr 175.6(4), C43–O4–Zr 159.7(5). | |
Ring-opening polymerization of lactides
Ring-opening polymerization of L-lactide (L-LA) initiated by complexes 1–7 was systematically studied as shown in Table 1. Experimental results reveal that dinuclear zirconium complex 1 is an effective initiator for the ROP of L-lactide in toluene at 100 °C. The conversion can reach over 90% within 10 min in the [LA]0/[complex]0 ratio ranging from 50 to 300 (Table 1, entries 1–5). The linear relationship between Mn versus [LA]0/[complex]0 and low polydispersity indexes (PDIs) indicate a good controlled manner and “living” character for the polymerization process. The 1H NMR spectrum of PLLA-50 reveals that the polymer chain is end-capped with an isopropyl ester and a hydroxyl group (Fig. 4). Based on these results, it is believed that the polymerization proceeds via the “coordination–insertion” mechanism and there are only two –OiPr groups that are active in the polymerization. Furthermore, complex 1 is also active toward unpurified L-lactide (entry 6). Complex 2 shows similar activity to that of complex 1 with the process occuring in a controlled manner (entries 7–11). However, the activity of 3 is somewhat lower than that of 1 and 2 with a conversion of L-LA of 72% within 10 min (entry 12). The conversion can reach >90% within 15 min (entries 13–16). In contrast, the mononuclear titanium complex 4 and yttrium complex 5 are inactive towards the ROP of L-lactide (entries 17–18) with the conversion <10% after several hours. It is also worth noting that the activity of mononuclear pyrazolonato zirconium complexes 6–7 in the ROP of L-LA is also low with the conversion <1% within 12 min. Complexes 1–3 are also active in the polymerization of rac-lactide in toluene with the conversion >90% within 10 min (Table 2, entries 1–3). However, the selectivity is poor with Pr = 0.64 for 1 and Pr = 0.62 for 2, which are somewhat higher than that of 3 (Pr = 0.55). For environmental protection purposes, metal complexes that can polymerize L-lactide in the absence of solvent are of great interest. It is interesting to note that complex 2 showed great activity in the solvent-free bulk polymerization of L-lactide with the conversion >90% within 15 min, yielding a polymer with high molecular weight (Mn = 5600–95
000) and low PDI (PDI < 1.40) (Table 3).
Table 1 Ring opening polymerization of L-lactidea
Entry |
Complex |
[LA]0/[complex]0 |
Time (min) |
Conv.b (%) |
Mnb (NMR) |
Mnc (obsd) |
PDId |
Reaction conditions: 100 °C, toluene (5.0 mL), [complex]0 = 5.0 mM. Obtained from the 1H NMR analysis. Obtained from GPC analysis and calibrated by polystyrene standard. Values in parentheses are the values obtained from GPC times 0.58.19 Obtained from GPC. Use unpurified L-Lactide. 80 °C. |
1 |
1 |
50/1 |
10 |
94 |
1100 |
3000 (1700) |
1.14 |
2 |
1 |
100/1 |
10 |
91 |
2600 |
5000 (2900) |
1.14 |
3 |
1 |
150/1 |
10 |
93 |
4100 |
7700 (4300) |
1.20 |
4 |
1 |
200/1 |
10 |
92 |
6300 |
11 000 (6400) |
1.20 |
5 |
1 |
300/1 |
10 |
93 |
9000 |
16 000 (9300) |
1.17 |
6e |
1 |
50/1 |
10 |
93 |
1000 |
2600 (1500) |
1.15 |
7 |
2 |
50/1 |
10 |
91 |
1900 |
3600 (2100) |
1.19 |
8 |
2 |
100/1 |
10 |
94 |
3500 |
6500 (3800) |
1.18 |
9 |
2 |
150/1 |
10 |
93 |
4700 |
8700 (5100) |
1.17 |
10 |
2 |
200/1 |
10 |
93 |
6300 |
10 800 (6200) |
1.21 |
11 |
2 |
300/1 |
10 |
92 |
8900 |
16 000 (9300) |
1.13 |
12 |
3 |
100/1 |
10 |
72 |
2900 |
5600 (3200) |
1.14 |
13 |
3 |
100/1 |
15 |
91 |
4000 |
7400 (4300) |
1.13 |
14 |
3 |
150/1 |
15 |
93 |
7000 |
12 000 (7000) |
1.18 |
15 |
3 |
200/1 |
15 |
92 |
8100 |
15 200 (8800) |
1.10 |
16 |
3 |
300/1 |
15 |
96 |
11 400 |
20 000 (12 000) |
1.19 |
17 |
4 |
100/1 |
420 |
<1% |
— |
— |
— |
18f |
5 |
100/1 |
96 |
<1% |
— |
— |
— |
19 |
6 |
100/1 |
10 |
<1% |
— |
— |
— |
20 |
7 |
100/1 |
10 |
<1% |
— |
— |
— |
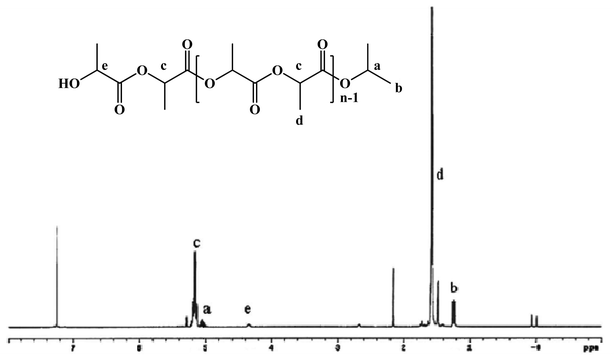 |
| Fig. 4 1H NMR spectrum of PLLA-50 (50 indicates [LA]0/[1]0 = 50). | |
Table 2 Ring-opening polymerization of rac-LAa
Entry |
Complex |
Conv.b (%) |
Solvent |
Mnb (NMR) |
Mnc (obsd) |
PDId |
Pre |
Reaction conditions: [rac-LA]0/[complex]0 = 100/1, 100 °C, solvent (5.0 mL), [complex]0 = 5 mM for 10 min. Obtained from the 1H NMR analysis. Obtained from GPC analysis and calibrated by polystyrene standard. Values in parentheses are the values obtained from GPC times 0.58.19 Obtained from GPC. Pr is the probability of racemic linkages between monomer units and is determined from the methine region of the homonuclear decoupled 1H NMR spectrum: [mmm] = [2(1 − Pr)2 + Pr(1 − Pr)]/2; [mrm] = [Pr2 + Pr(1 − Pr)]/2; [mmr] = [rmm] = [Pr(1 − Pr)]/2; [rmr] = Pr2/2. Reaction conditions: 80 °C, 50 min. Reaction conditions: 50 °C, 24 h. |
1 |
1 |
93 |
Toluene |
3200 |
6800 (3900) |
1.23 |
0.64 |
2 |
2 |
90 |
Toluene |
3000 |
6000 (3500) |
1.17 |
0.62 |
3 |
3 |
92 |
Toluene |
3000 |
6100 (3500) |
1.21 |
0.55 |
4f |
1 |
91 |
Toluene |
3100 |
6200 (3600) |
1.19 |
0.65 |
5f |
1 |
82 |
THF |
2800 |
5400 (3100) |
1.24 |
0.64 |
6g |
1 |
93 |
Toluene |
2700 |
6100 (3500) |
1.23 |
0.54 |
Table 3 Bulk polymerization of L-lactide initiated by complex 2a
Entry |
[LA]0/[2] |
Time (min) |
Conv.b (%) |
Mnb (NMR.) |
Mnc (obsd) |
PDId |
Reaction conditions: 140 °C, [complex]0 = 5.0 mM. Obtained from the 1H NMR analysis. Obtained from GPC analysis and calibrated by polystyrene standard. Values in parentheses are the values obtained from GPC times 0.58.19 Obtained from GPC. |
1 |
100/1 |
10 |
91 |
5000 |
9700 (5600) |
1.37 |
2 |
200/1 |
10 |
93 |
18 000 |
19 000 (11 300) |
1.32 |
3 |
1000/1 |
10 |
93 |
45 900 |
80 600 (46 700) |
1.39 |
4 |
6000/1 |
15 |
95 |
94 100 |
164 000 (95 000) |
1.31 |
Ring-opening polymerization of ε-caprolactone
Ring-opening polymerization (ROP) of ε-caprolactone (ε-CL) initiated by complexes 1–7 was also studied (Table 4). Experimental results indicate that the dinuclear complex 1 is highly active in the polymerization of ε-CL with the conversion >90% within 5 min at 80 °C (entries 1–4). However, it requires 15 min to reach 86% conversion when the temperature is decreased to 60 °C (entry 5). The activity of complex 1 in ε-CL polymerization (80 °C, 5 min) is higher than the activity in LA polymerization (100 °C, 10 min). The plot of Mn versus ([M]0/[complex]0) exhibits a linear relationship for ROP of ε-CL, indicating the “living” character of the polymerization process. Similarly, the activity of 2 (entry 6) in ε-CL polymerization is comparable with that of 1, and the activity of 3 (entry 7) is somewhat lower that of 1 and 2. Unlike in the ROP of LA, mononuclear titanium and yttrium complexes are also active initiators in ROP of ε-CL, with the conversion of 73% for 4 (entry 9, Table 4) in 420 min and 86% for 5 in 32 min (entry 10, Table 4).
Table 4 Ring opening polymerization of ε-caprolactone (ε-CL)a
Entry |
Complex |
[ε-CL]0/[complex] |
Time (min) |
Conv.b (%) |
Mnb (NMR.) |
Mnc (obsd) |
PDId |
Reaction conditions: 80 °C, toluene (5.0 mL), [complex]0 = 5 mM. Obtained from the 1H NMR analysis. Obtained from GPC analysis and calibrated by polystyrene standard. Values in parentheses are the values obtained from GPC times 0.56.19 Obtained from GPC. Reaction conditions: 60 °C. |
1 |
1 |
50/1 |
5 |
94 |
3700 |
6900 (3900) |
1.14 |
2 |
1 |
100/1 |
5 |
94 |
5600 |
10 800 (6000) |
1.16 |
3 |
1 |
150/1 |
5 |
95 |
7100 |
12 900 (7200) |
1.15 |
4 |
1 |
200/1 |
5 |
95 |
7500 |
14 300 (8000) |
1.15 |
5e |
1 |
200/1 |
15 |
87 |
6600 |
12 500 (7000) |
1.15 |
6 |
2 |
100/1 |
5 |
95 |
5500 |
10 500 (5900) |
1.10 |
7 |
3 |
100/1 |
20 |
88 |
5700 |
11 000 (6200) |
1.21 |
8 |
4 |
100/1 |
90 |
6 |
— |
— |
— |
9 |
4 |
100/1 |
420 |
73 |
7000 |
12 900 (7200) |
1.38 |
10 |
5 |
100/1 |
32 |
86 |
— |
35 700 (20 000) |
1.18 |
Kinetic studies of the ring-opening polymerization of L-lactide
The kinetic studies of complex 3 were performed in toluene at 100 °C. Conversion of L-lactide with time in the presence of different concentration of complex 3 ([3]0 = 1, 2, 3, 4 mM) was monitored by 1H NMR spectroscopic studies. The plots of ln([LA]0/[LA]t) versus time were linear indicating the polymerization rate has a first-order dependency on L-lactide (Fig. 5a). Thus, the rate law of polymerization can be written as −d[LA]/dt = kobs[LA], where kobs = kapp[3]x. The reaction order of [3] can be obtained by the plotting of ln
kobs versus ln[3]. The slope reveals that the polymerization rate is −d[LA]/dt = kapp[LA][3]1.5, where the rate constant kapp = 4.95 mol−2 L2 s−1 based on the intercept of the fitted line in Fig. 5b.
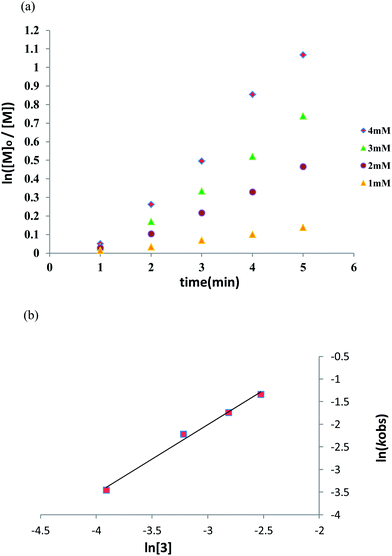 |
| Fig. 5 (a) ln([M]0/[M]) versus time plot, demonstrating the first-order dependence for L-lactide polymerizations catalyzed by complex 3 in toluene at 100 °C with different concentrations of 3 ([3]0 = 1, 2, 3, 4 mM in toluene at 100 °C, [LA]0 = 0.05 M). (b) Linear plot of ln kobs versus ln[3] for the polymerization of L-lactide (toluene, 100 °C, [LA]0 = 0.05 M). | |
Proposed mechanism for the polymerization of L-lactide
Based on the molecular structure and kinetic study results of zirconium complex 3, it is believed that the polymerization proceeds by the dissociation of the bridging isopropoxide and the coordination of L-lactide giving intermediate A (Scheme 3). Following the insertion of Zr-OiPr into the L-lactide, intermediate B is obtained.
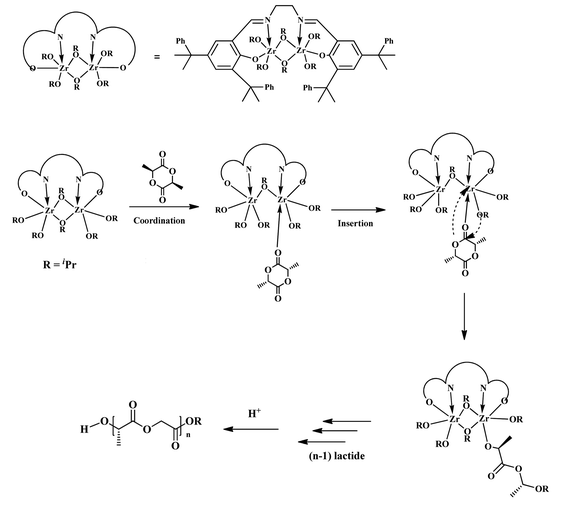 |
| Scheme 3 Proposed mechanism for the ROP of L-lactide initiated by complex 3. | |
Conclusion
A series of dinuclear zirconium complexes and mononuclear titanium, yttrium and zirconium complexes supporting salen-type ligands have been prepared and characterized. Dinuclear zirconium salen complexes [LZr(OiPr)2]2[(μ-OiPr)2] (1–3) have shown effective activity toward the ring opening polymerization of lactides and ε-caprolactone. Bulk polymerization of L-lactide initiated by 2 with high molecular weight PLLA (Mn(obsd) = 95
000 g mol−1 after correction) can be achieved within 15 min.
Acknowledgements
Financial support from the National Science Council of the Republic of China is gratefully appreciated. (NSC-101-2113-M-005-010-MY3).
References
-
(a) M. Vert, S. M. Li, G. Spenlehauer and P. Guerin, J. Mater. Sci.: Mater. Med., 1992, 3, 432–446 CrossRef CAS;
(b) W. C. Puelacher, D. Mooney, R. Langer, J. Upton, J. P. Vacanti and C. A. Vacanti, Biomaterials, 1994, 15, 774–778 CrossRef CAS PubMed;
(c) E. Chiellini and R. Solaro, Adv. Mater., 1996, 8, 305–313 CrossRef CAS;
(d) B. Jeong, Y. H. Bae, D. S. Lee and S. W. Kim, Nature, 1997, 388, 860–862 CrossRef CAS PubMed;
(e) R. A. Gross and B. Kalra, Science, 2002, 297, 803–807 CrossRef CAS PubMed;
(f) R. E. Drumright, P. R. Gruber and D. E. Henton, Adv. Mater., 2000, 12, 1841–1846 CrossRef CAS;
(g) R. Auras, B. Harte and S. Selke, Macromol. Biosci., 2004, 4, 835–864 CrossRef CAS PubMed.
-
(a) A. P. Gupta and V. Kumar, Eur. Polym. J., 2007, 43(10), 4053 CrossRef CAS;
(b) S. Inkinen, M. Hakkarainen, A. C. Albertsson and A. Sodergard, Biomacromolecules, 2011, 12, 523 CrossRef CAS PubMed.
- For reviews:
(a) B. J. O'Keefe, M. A. Hillmyer and W. B. Tolman, J. Chem. Soc., Dalton Trans., 2001, 2215–2224 RSC;
(b) D. A. Atwood and M. J. Harvey, Chem. Rev., 2001, 101, 37–52 CrossRef CAS PubMed;
(c) O. Dechy-Cabaret, B. Martin-Vaca and D. Bourissou, Chem. Rev., 2004, 104, 6147–6176 CrossRef CAS PubMed;
(d) J. Wu, T.-L. Yu, C.-T. Chen and C.-C. Lin, Coord. Chem. Rev., 2006, 250, 602–626 CrossRef CAS;
(e) K. M. Stridsberg, M. Ryner and A.-C. Albertsson, Adv. Polym. Sci., 2002, 157, 41–52 CrossRef CAS;
(f) R. H. Platel, L. M. Hodgson and C. K. Williams, Polym. Rev., 2008, 48, 11–63 CrossRef CAS;
(g) C. Wheaton, P. Hayes and B. Ireland, Dalton Trans., 2009,(25), 4832–4846 RSC;
(h) D. Samuel, N. Mickael, K. Evgeny and J.-F. Carpentier, Coord. Chem. Rev., 2013, 257, 1869–1886 CrossRef.
- Examples of group 1 and 2 metals:
(a) B.-T. Ko and C.-C. Lin, J. Am. Chem. Soc., 2001, 123, 7973–7977 CrossRef CAS PubMed;
(b) C. A. Huang and C. T. Chen, Dalton Trans., 2007, 5561–5566 RSC;
(c) H.-Y. Chen, J. Zhang, C.-C. Lin, J. H. Reibenspies and S. A. Miller, Green Chem., 2007, 9, 1038 RSC;
(d) W. Clegg, M. G. Davidson, D. V. Graham, G. Griffen, M. D. Jones, A. R. Kennedy, C. T. O'Hara, L. Russo and C. M. Thomson, Dalton Trans., 2008, 1295–1301 RSC;
(e) Y. Huang, Y.-H. Tsai, W.-C. Hung, C.-S. Lin, W. Wang, J.-H. Huang, S. Dutta and C.-C. Lin, Inorg. Chem., 2010, 49, 9416–9425 CrossRef CAS PubMed;
(f) B. Calvo, M. G. Davidson and D. García-Vivó, Inorg. Chem., 2011, 50, 3589–3595 CrossRef CAS PubMed;
(g) X. Xu, X. Pan, S. Tang, X. Lv, L. Li, J. Wu and X. Zhao, Inorg. Chem. Commun., 2013, 29, 89–93 CrossRef CAS;
(h) R. K. Dean, A. M. Reckling, H. Chen, L. N. Dawe, C. M. Schneider and C. M. Kozak, Dalton Trans., 2013, 42, 3504–3520 RSC;
(i) M. H. Chisholm, N. W. Eilerts, J. C. Huffman, S. S. Iyer, M. Pacold and K. Phomphrai, J. Am. Chem. Soc., 2000, 122, 11845–11854 CrossRef CAS;
(j) B. M. Chamberlain, M. Cheng, D. R. Moore, T. M. Ovitt, E. Lobkovsky and G. W. Coates, J. Am. Chem. Soc., 2001, 123, 3229–3238 CrossRef CAS PubMed;
(k) M. S. Hill and P. B. Hitchcock, Chem. Commun., 2003, 1758–1759 RSC;
(l) M. Westerhausen, M. Gärtner, R. Fischer and J. Langer, Angew. Chem., Int. Ed., 2007, 46, 1950–1956 CrossRef CAS PubMed;
(m) J. D. Smith, Angew. Chem., Int. Ed., 2009, 48, 6597–6599 CrossRef CAS PubMed;
(n) S. Harder, Chem. Rev., 2010, 110, 3852–3876 CrossRef CAS PubMed;
(o) R. A. Collins, J. Unruangsri and P. Mountford, Dalton Trans., 2013, 42, 759–769 RSC;
(p) M.-W. Hsiao and C.-C. Lin, Dalton Trans., 2013, 42, 2041–2051 RSC;
(q) H.-J. Chuang, H.-L. Chen, J.-L. Ye, Z.-Y. Chen, P.-L. Huang, T.-T. Liao, T.-E. Tsai and C.-C. Lin, J. Polym. Sci., Part A: Polym. Chem., 2013, 51, 696–707 CrossRef CAS.
- For group 3 metals:
(a) W. M. Stevels, M. J. K. Ankone, P. J. Dijkstra and J. Feijen, Macromolecules, 1996, 29, 3332–3333 CrossRef CAS;
(b) B. M. Chamberlain, Y. Sun, J. R. Hagadorn, E. W. Hemmesch, V. G. Young, Jr, M. Pink, M. A. Hillmyer and W. B. Tolman, Macromolecules, 1999, 32, 2400–2402 CrossRef CAS;
(c) T. M. Ovitt and G. W. Coates, J. Am. Chem. Soc., 1999, 121, 4072–4073 CrossRef CAS;
(d) A. Amgoune, C. M. Thomas, T. Roisnel and J.-F. Carpentier, Chem. – Eur. J., 2005, 12, 169–179 CrossRef PubMed;
(e) C. Bakewell, T. P. Cao, N. Long, X. F. Le Goff, A. Auffrant and C. K. Williams, J. Am. Chem. Soc., 2012, 134, 20577–20580 CrossRef CAS PubMed;
(f) M. Mazzeo, R. Tramontano, M. Lamberti, A. Pilone, S. Milione and C. Pellecchia, Dalton Trans., 2013, 42, 9338–9351 RSC;
(g) J. Qiu, M. Lu, Y. Yao, Y. Zhang, Y. Wang and Q. Shen, Dalton Trans., 2013, 42, 10179–10189 RSC.
- For group 4 metals:
(a) Y. Kim, P. N. Kapoor and J. G. Verkade, Inorg. Chem., 2002, 41, 4834 CrossRef CAS PubMed;
(b) B. Lian, L. Toupet and J.-F. Carpentier, Chem. – Eur. J., 2004, 10, 4301–4307 CrossRef CAS PubMed;
(c) A. J. Chmura, M. G. Davidson, M. D. Jones, M. D. Lunn and M. F. Mahon, Dalton Trans., 2006, 887–889 RSC;
(d) N. Marquet, E. Kirillov, T. Roisnel, A. Razavi and J.-F. Carpentier, Organometallics, 2009, 28, 606–620 CrossRef CAS;
(e) A. L. Zelikoff, J. Kopilov, I. Goldberg, G. W. Coates and M. Kol, Chem. Commun., 2009, 6804–6806 RSC;
(f) B. J. Jeffery, E. L. Whitelaw, D. Garcia-Vivo, J. A. Stewart, M. F. Mahon, M. G. Davidson and M. D. Jones, Chem. Commun., 2011, 47, 12328–12330 RSC;
(g) E. Kirillov, N. Marquet, M. Bader, A. Razavi, V. Belia, F. Hampel, T. Roisnel, J. A. Gladysz and J.-F. Carpentier, Organometallics, 2011, 30, 263–272 CrossRef CAS;
(h) T. K. Saha, V. Ramkumar and D. Chakraborty, Inorg. Chem., 2011, 50, 2720–2722 CrossRef CAS PubMed;
(i) C. Romain, B. Heinrich, S. B. Laponnaz and S. Dagorne, Chem. Commun., 2012, 48, 2213–2215 RSC;
(j) L.-C. Liang, S.-T. Lin, C.-C. Chien and M.-T. Chen, Dalton Trans., 2013, 42, 9286–9293 RSC.
- For group 13 metals:
(a) B.-T. Ko and C.-C. Lin, Macromolecules, 1999, 32, 8296 CrossRef CAS;
(b) M. H. Chisholm, C.-C. Lin, J. C. Gallucci and B.-T. Ko, Dalton Trans., 2003, 406 RSC;
(c) R. C. Yu, C. H. Hung, J. H. Huang, H. Y. Lee and J. T. Chen, Inorg. Chem., 2002, 41, 6450 CrossRef CAS PubMed;
(d) A. Alaaeddine, T. Roisnel, C. M. Thomas and J.-F. Carpentier, Adv. Synth. Catal., 2008, 350 Search PubMed;
(e) M. P. F. Pepels, M. Bouyahyi, A. Heise and R. Duchateau, Macromolecules, 2013, 46, 4324–4334 CrossRef CAS;
(f) V. Balasanthiran, M. H. Chisholm, C. B. Durr and J. C. Gallucci, Dalton Trans., 2013, 42, 11234–11241 RSC.
- For other metals:
(a) B. J. O'Keefe, M. A. Hillmyer and W. B. Tolman, Dalton Trans., 2001, 2215–2224 RSC;
(b) E. Barnea, D. Moradove, J.-C. Berthet, M. Ephritikhine and M. S. Eisen, Organometallics, 2006, 25, 320 CrossRef CAS;
(c) E. Rabinovich, S. Aharonovich, M. Botoshansky and M. S. Eisen, Dalton Trans., 2010, 39, 6667–6676 RSC;
(d) P. J. Dijkstra, H. Du and J. Feijen, Polym. Chem., 2011, 2, 520–527 RSC;
(e) C. E. Hayes, Y. Sarazin, M. J. Katz, J.-F. Carpentier and D. B. Leznoff, Organometallics, 2013, 32, 1183–1192 CrossRef CAS.
-
(a) J. F. Larrow and E. N. Jacobsen, Top. Organomet. Chem., 2004, 6, 123–152 CAS;
(b) N. S. Venkataramanan, G. Kuppuraj and S. Rajagopai, Coord. Chem. Rev., 2005, 249, 1249 CrossRef CAS.
-
(a) S. R. Doctrow, K. Huffman, C. B. Marcus, G. Tocco, E. Malfroy, C. A. Adinolfi, H. Kruk, K. Baker, N. Lazarowych, J. Mascarenhas and B. Malfroy, J. Med. Chem., 2002, 45, 4549–4558 CrossRef CAS PubMed;
(b) K. Pamin, G. Pozzi, E. Tabor, W. Bukowski and J. Połtowicz, Catal. Commun., 2013, 39, 102–105 CrossRef CAS;
(c) K. Balaraman, R. Vasanthan and V. Kesavan, Tetrahedron, 2013, 69, 6162–6169 CrossRef CAS;
(d) Y. Noritake, N. Umezawa, N. Kato and T. Higuchi, Inorg. Chem., 2013, 52, 3653–3662 CrossRef CAS PubMed.
-
(a) T. R. Younkin, E. F. Connor, J. I. Henderson, S. K. Friedrich, R. H. Grubbs and D. A. Bansleben, Science, 2000, 287, 460–462 CrossRef CAS PubMed;
(b) E. Y. Tshuva, I. Goldberg and M. Kol, J. Am. Chem. Soc., 2000, 122, 10706–10707 CrossRef CAS;
(c) J. Huang, B. Lian, L. Yong and Y. Qian, Inorg. Chem. Commun., 2001, 4, 392–394 CrossRef CAS;
(d) V. Busico, R. Cipullo, N. Friederich, S. Ronca and M. Toguru, Macromolecules, 2003, 36, 3806–3808 CrossRef CAS;
(e) V. Busico, R. Cipullo, N. Friederich, S. Ronca, G. Talarico, M. Toguru and B. Wang, Macromolecules, 2004, 37, 8201–8203 CrossRef CAS;
(f) A. Yeori, I. Goldberg, M. Szuster and M. Kol, J. Am. Chem. Soc., 2006, 128, 13062–13063 CrossRef CAS PubMed;
(g) J.-L. Huang, B. Lian, L. Yong and Y.-L. Qian, Chin. J. Chem., 2004, 22, 577–584 CrossRef CAS;
(h) M. Strianese, M. Lamberti, M. Mazzeo, C. Tedesco and C. Pellecchia, J. Mol. Catal. A: Chem., 2006, 258, 284–291 CrossRef CAS;
(i) M. Bialek and A. Pietruszka, J. Polym. Sci., Part A: Polym. Chem., 2009, 47(23), 6693–6703 CrossRef CAS.
-
(a) C. P. Radano, G. L. Baker and M. R. Smith, J. Am. Chem. Soc., 2000, 122, 1552–1553 CrossRef CAS;
(b) Z. Zhong, P. J. Digkstra and J. Feijen, Angew. Chem., Int. Ed., 2002, 41, 4510–4513 CrossRef CAS;
(c) P. Hormnirun, E. L. Marshall, V. C. Gibson, A. J. P. White and D. J. Williams, J. Am. Chem. Soc., 2004, 126, 2688–2689 CrossRef CAS PubMed;
(d) P. Hormnirun, E. L. Marshall, V. C. Gibson, R. I. Pugh and A. White, Proc. Natl. Acad. Sci. U. S. A., 2006, 103, 15343–15348 CrossRef CAS PubMed;
(e) N. Nomura, R. Ishii, Y. Yamamoto and T. Kondo, Chem. – Eur. J., 2007, 13, 4433–4451 CrossRef CAS PubMed;
(f) X. Pang, H. Du, X. Chen, X. Wang and X. Jing, Chem. – Eur. J., 2008, 14, 3126–3136 CrossRef CAS PubMed;
(g) H. Du, A. H. Velders, P. J. Dijkstra, J. Sun, Z. Zhong, X. Chen and J. Feijen, Chem. – Eur. J., 2009, 15, 9836–9845 CrossRef CAS PubMed;
(h) H.-L. Chen, S. Dutta, P.-Y. Huang and C.-C. Lin, Organometallics, 2012, 31, 2016–2025 CrossRef CAS.
- S. Gendler, S. Segal, I. Goldberg, Z. Goldschmidt and M. Kol, Inorg. Chem., 2006, 45, 4783–4790 CrossRef CAS PubMed.
- H.-J. Chuang, Y.-C. Su, B. T. Ko and C.-C. Lin, Inorg. Chem. Commun., 2012, 18, 38–42 CrossRef CAS.
- G. M. Sheldrick, SADABS: Siemens Area Detector Absorption Correction Softwares, University of Gottingen, Gottingen, Germany, 1998 Search PubMed.
- G. M. Sheldrick, SHELXTL-Plus, NT Crystallographic System, release 5.1; Bruker Analytical X-ray Systems, Madison, WI, 1998 Search PubMed.
- T. K. Saha, V. Ramkumar and D. Chakraborty, Inorg. Chem., 2011, 50, 2720–2722 CrossRef CAS PubMed.
-
(a) S. Gendler, S. Segal, I. Goldberg, Z. Gold Jones, M. D. Lunn, M. F. Mahon, A. F. Johnson, P. Khunkamchoo, S. L. Roberts and S. S. F. Wong, Macromolecules, 2006, 39, 7250–7257 CrossRef;
(b) A. Stopper, J. Okuda and M. Kol, Macromolecules, 2012, 45, 698–704 CrossRef CAS.
- Values in parenthesis is Mn(GPC) times 0.58 for polylactide and 0.56 for ε-caprolactone
(a) J. Baran, A. Duda, A. Kowalski, R. Szymanski and S. Penczek, Macromol. Rapid Commun., 1997, 18, 325–333 CrossRef CAS;
(b) T. Biela, A. Duda and S. Penczek, Macromol. Symp., 2002, 183, 1–10 CrossRef CAS;
(c) M. Save, M. Schappacher and A. Soum, Macromol. Chem. Phys., 2002, 203, 889–899 CrossRef CAS.
Footnote |
† Electronic supplementary information (ESI) available: Variable 1H NMR spectra of complex 6 ranging from −40 °C to 80 °C are also available. CCDC 958124 and 958125. For ESI and crystallographic data in CIF or other electronic format see DOI: 10.1039/c4ra00201f |
|
This journal is © The Royal Society of Chemistry 2014 |
Click here to see how this site uses Cookies. View our privacy policy here.