DOI:
10.1039/C3RA48071B
(Communication)
RSC Adv., 2014,
4, 12482-12485
Rh2(OAc)4-catalyzed 2,3-migration of β-ferrocenecarboxyl α-diazocarbonyl compounds: an efficient synthesis of ferrocene-containing α,β-unsaturated esters†
Received
31st December 2013
, Accepted 17th February 2014
First published on 17th February 2014
Abstract
A series of β-ferrocenecarboxyl α-diazocarbonyl compounds were prepared by the reaction of ferrocenoyl chloride with β-hydroxyl α-diazocarbonyl compounds in the presence of pyridine. The diazo decomposition of these ferrocene-containing diazocarbonyl compounds with Rh2(OAc)4 resulted in 2,3-ferrocenecarboxyl migration to give ferrocene-containing α,β-unsaturated esters in high yields.
Introduction
Since its discovery more than sixty years ago, ferrocene has acquired significant attention1 as a consequence of its unique physical and chemical properties which allow for its application in many areas such as asymmetric catalysis,2 materials science,3 and bioorganometallic chemistry.4 Among these, the field where ferrocene importance is continuously increasing is bioorganometallic chemistry.5 Although ferrocene itself is usually considered to be a nontoxic, biologically inert compound, many novel ferrocene derivatives exhibit significant anticancer, antiparasitic, antibacterial, antifungal, and other biological activities.4,5 Undoubtedly, the design motif and synthesis strategy in ferrocene-based architectures play the most important role to deliver the targeted property. Due to their increasing applications in biology, materials science, and chemistry, there has been an ever increasing demand for the synthesis of novel ferrocenes bearing various functional groups through simple and efficient approaches.
On the other hand, transition-metal-catalyzed diazo decomposition of various substituted diazocarbonyl compounds is a class of important transformation in organic chemistry that has been widely investigated for its intriguing mechanistic features and extensive applications in natural products synthesis.6 In these diazo decomposition reactions, 1,2-migration is sometimes the dominating pathway, which can compete with the typical reactions of diazo compounds, such as cyclopropanations, X–H (X = C, O, S, N, etc.) insertions and ylide formations.6 Among the transition-metal-catalyzed 1,2-migration reactions of α-diazo carbonyl compounds, the 1,2-hydride migration is usually predominant,7 but 1,2-aryl, 1,2-alkyl, 1,2-vinyl, 1,2-acetylenyl and 1,2-thio group migrations are also reported.8 Besides, 1,2-acetoxy group migration of Rh(II)–carbene was also described by Ganem and co-workers in 1981;9 it is conceived that the 1,2-migration of acetoxy group may proceed through a three-centered or five-centered transition state, where the lone pair electrons of the alkoxy or carbonyl oxygen of the acetoxy group interacts with the electron-deficient Rh(II)–carbene center (Scheme 1). It should be noted that it is actually a 2,3-migration reaction if 1,2-acetoxy group migration proceeds through a five-centered transition state. Wang and co-workers have demonstrated a 2,3-migration reaction of Rh(II)–carbene subtly through installation of an amido group onto the β-position of the α-diazo carbonyl compounds.10 Inspired by this report, and also as an extension of our interest in the ferrocene and diazo chemistry,11 we conceived that an α-diazo carbonyl compound bearing a ferrocenecarboxyl group on the β-position may also undergo a similar acyloxy migration in a Rh(II)-catalyzed reaction to give a ferrocene-containing α,β-unsaturated ester. Moreover, if the reaction occurs as predicted, the migration should proceed through a five-centered transition state because of the steric effect of the bulky ferrocene group. In this paper, we wish to report the Rh2(OAc)4 catalyzed decomposition of β-ferrocenecarboxyl α-diazocarbonyl compounds. The results show an efficient 2,3-ferrocenecarboxyl migration leading to the formation of ferrocene-containing α,β-unsaturated esters.
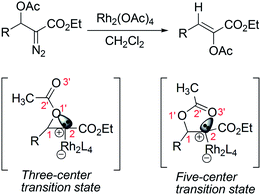 |
| Scheme 1 Possible transition state of 1,2-acetoxy migration. | |
Results and discussion
At the outset of this investigation, we first converted the β-hydroxyl group in diazo compound 2 into β-ferrocenecarboxyl group to afford β-ferrocenecarboxyl substituted diazo compounds 3a–j (Scheme 2). The β-hydroxy α-diazo esters 2 were prepared by tert-BuOK-catalyzed condensation of ethyl diazoacetate (EDA) with aldehyde under solvent-free conditions recently developed by our group with the yields ranging from 69% to 92%.12 Then ferrocenoyl chloride in anhydrous CH2Cl2 was added dropwise to a solution of β-hydroxy α-diazo ester 2 with pyridine in anhydrous CH2Cl2 at 0 °C. The esterification reaction was completed within 3 h, and the β-ferrocenecarboxyl substituted α-diazo carbonyl compounds 3a–j were obtained in moderate to good yields (Table 1).
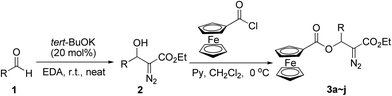 |
| Scheme 2 Synthesis of β-ferrocenecarboxyl α-diazocarbonyl compounds. | |
Table 1 Reaction of β-hydroxy α-diazo esters with ferrocenoyl chloridea
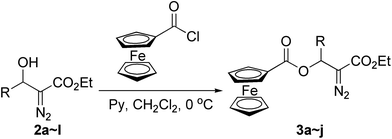
|
Entry |
R |
Time (h) |
Yieldb (%) |
Reactions were carried out with 1.0 mmol of 2, 1.1 mmol of ferrocenoyl chloride, and 2.0 mmol of pyridine at 0 °C. Yield of isolated product after chromatography. No β-ferrocenecarboxyl substituted α-diazo ester product was detected. |
1 |
2a, C6H5 |
2 |
3a, 56 |
2 |
2b, p-ClC6H4 |
2 |
3b, 55 |
3 |
2c, p-BrC6H4 |
2 |
3c, 57 |
4 |
2d, o-ClC6H4 |
2.5 |
3d, 70 |
5 |
2e, p-CH3C6H4 |
1 |
3e, 65 |
6 |
2f, p-NO2C6H4 |
3 |
3f, 66 |
7 |
2g, o-NO2C6H4 |
3 |
3g, 61 |
8 |
2h, H |
1.5 |
3h, 70 |
9 |
2i, CH3 |
1.5 |
3i, 60 |
10 |
2j, CH3CH2CH2 |
1.5 |
3j, 50 |
11 |
2k, p-CH3OC6H4 |
3 |
ndc |
12 |
2l, 2-furyl |
3 |
ndc |
It is worthwhile to note that the reaction should be quenched with saturated NaHCO3 at 0 °C in order to avoid the oxidation of ferrocene as well as the decomposition of the diazo compound under acidic conditions. Most aromatic or aliphatic substituents could be tolerated, and the reaction gave moderate to good yields of the products 3a–j (Table 1, entries 1–10). However, when R was a p-methoxyphenyl or 2-furyl group, we were disappointed to find that the reaction gave a complex mixture and no desired β-ferrocenecarboxyl substituted α-diazo ester product was detected (Table 1, entries 11 and 12). Moreover, the pure ferrocene-containing diazo compounds 3a–j obtained above are relatively stable and can be kept at 0 °C for several days without any change.
With the β-ferrocenecarboxyl substituted α-diazo esters in hand, we then proceeded to investigate their diazo decomposition behavior using Rh2(OAc)4 as catalyst. First, diazo compounds 3a–g with an aromatic ring on the β-position were investigated. The diazo decomposition proceeded smoothly in the presence of 0.1 mol% of Rh2(OAc)4 in anhydrous CH2Cl2 at room temperature to afford the stereoisomeric mixture of products 4a–g in excellent total yields (Table 2, entries 1–7). It was found that substrates with a halogen or electron-donating substituent on the phenyl ring, such as methyl group, gave slightly lower stereoselectivities (Table 2, entries 1–5), while substrates with a phenyl ring bearing a strong electron-withdrawing group generally gave slightly higher stereoselectivities (Table 2, entries 6 and 7). More interestingly, when R was an aliphatic group, the reaction gave only one isomer whose absolute configuration was not identified from the 1H NMR spectra (Table 2, entries 9 and 10). Moreover, the stereochemistry of the E product was further confirmed by X-ray structure of 4Eb (Fig. 1).13
Table 2 Rh(II)-catalyzed reaction of diazo compounds 3a–j
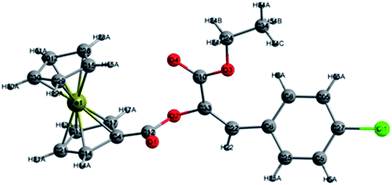 |
| Fig. 1 X-ray structure of 4Eb. | |
Based on the experimental results above and the X-ray structure of 4Eb as well as previous understanding of this type of reactions,9,10 we conceived that this reaction was performed through a facile 2,3-ferrocenecarboxyl migration of the Rh(II)–carbene. Considering the steric effect of the bulky ferrocene group, the 2,3-migration in this Rh(II)-catalyzed reaction is considered to be the favorable conformation of the five-centered transition state as depicted in Scheme 3. The formation of three-centered transition state may be highly unfavorable due to the steric interaction of the phenyl ring with the ferrocene skeleton. Thus, the current study supports the five-centered transition state that was proposed by Ganem and Wang for 1,2-acetoxy and 2,3-trifluoroacetamido migration.
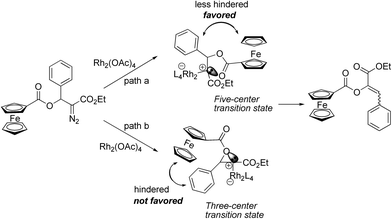 |
| Scheme 3 Possible pathway for Rh2(OAc)4-catalyzed migration reaction of β-ferrocenecarboxyl α-diazocarbonyl compound. | |
Conclusions
In conclusion, we have observed an efficient 2,3-ferrocenecarboxyl migration leading to the formation of ferrocene-containing α,β-unsaturated esters under mild reaction conditions. Mechanistically, the reaction is thought to proceed through a five-centered transition state. Moreover, this reaction may find synthetic application as a new entry to these novel ferrocene derivatives.
Experimental
General procedure for the preparation of β-ferrocenecarboxyl substituted diazo compounds 3
In a dried three-necked round-bottom flask, ferrocenoyl chloride (1.1 mmol) in 2 mL of anhydrous CH2Cl2 was added dropwise to a solution of β-hydroxy α-diazo ester 2 (1.0 mmol) with pyridine (2.0 mmol) in 5 mL of anhydrous CH2Cl2 at 0 °C under N2. The resulting mixture was stirred at 0 °C and the progress of the reaction was monitored by TLC. After completion of the esterification reaction, saturated NaHCO3 was added at 0 °C. the organic phase was dried using Na2SO4, and solvent was removed under reduced pressure. Next, the residue was subjected to chromatography on silica gel to afford the products 3a–j.
General procedure for the Rh2(OAc)4-catalyzed reaction of 3a–j
In a dried three-necked round-bottom flask, Rh2(OAc)4 (0.1 mol%) was dissolved into 5 mL of anhydrous CH2Cl2. A solution of diazo substrates 3a–j in anhydrous CH2Cl2 was added dropwise at 0 °C over the course of 30 min. The reaction mixture was stirred until TLC analysis indicated the complete disappearance of the diazo compound. The solvent was then removed under reduced pressure, and the crude residue was purified by column chromatography to give the corresponding 2,3-migration products.
Acknowledgements
This project is generously supported by National Natural Science Foundation of China (no. 21262023, 21102068 and 20902044) and the Program for Young Talents of Science and Technology in Universities of Inner Mongolia Autonomous Region (NJYT-12-B03).
Notes and references
- For recent monographs and review, see:
(a) A. Togni and T. Hayashi, Ferrocenes: Homogeneous Catalysis, Organic Synthesis, Material Science, VCH, New York, 1995 Search PubMed;
(b) N. J. Long, Metallocenes: An Introduction to Sandwich Complexes, Blackwell Science, Oxford, U.K., 1998 Search PubMed;
(c) P. Štĕpnička, Ferrocenes: Ligands, Materials and Biomolecules, Wiley-VCH, Chichester, U.K., 2008 Search PubMed;
(d) K. Kowalski, Coord. Chem. Rev., 2010, 254, 1895 CrossRef CAS PubMed;
(e) P. Molina, A. Tárraga and M. Alfonso, Eur. J. Org. Chem., 2011, 4505 CrossRef CAS.
- For related monograph and reviews, see:
(a) L. Dai and X. Hou, Chiral Ferrocenes in Asymmetric Catalysis: Synthesis and Applications, Wiley-VCH, Weinheim, 2010 Search PubMed;
(b) C. J. Richards and A. J. Locke, Tetrahedron: Asymmetry, 1998, 9, 2377 CrossRef CAS;
(c) L. Dai, T. Tu, S. You, W. Deng and X. Hou, Acc. Chem. Res., 2003, 36, 659 CrossRef CAS PubMed;
(d) O. B. Sutclie and M. R. Bryce, Tetrahedron: Asymmetry, 2003, 14, 2297 CrossRef;
(e) R. C. J. Atkinson, V. C. Gibson and N. J. Long, Chem. Soc. Rev., 2004, 33, 313 RSC;
(f) A. Moyano and R. Rios, Synlett, 2009, 1863 CrossRef CAS PubMed.
- For related monograph and reviews, see:
(a) S. Barlow and S. R. Marder, in Functional Organic Materials, ed. T. J. J. Muller and U. H. F. Bunz, Wiley-VCH, Weinheim, 2007, pp. 393–437 Search PubMed;
(b) S. A. Hudson and P. M. Maitlis, Chem. Rev., 1993, 93, 861 CrossRef CAS;
(c) A. S. Abd-El-Aziz and E. K. Todd, Coord. Chem. Rev., 2003, 246, 3 CrossRef CAS;
(d) B. Fabre, Acc. Chem. Res., 2010, 43, 1509 CrossRef CAS PubMed.
- For related monographs and reviews, see:
(a) G. Jaouen, Bioorganometallics: Biomolecules, Labeling, Medicine, Wiley-VCH, New York, 2006 Search PubMed;
(b) G. Jaouen and N. Metzler-Nolte, Medicinal Organometallic Chemistry, Springer, Berlin, 2010, Topics in Organometallic Chemistry 32 Search PubMed;
(c) D. R. Van Staveren and N. Metzler-Nolte, Chem. Rev., 2004, 104, 5931 CrossRef CAS PubMed;
(d) M. F. R. Fouda, M. M. Abd-Elzaher, R. A. Abdelsamaia and A. A. Labib, Appl. Organomet. Chem., 2007, 21, 613 CrossRef CAS;
(e) G. Gasser, I. Ott and N. Metzler-Nolte, J. Med. Chem., 2011, 54, 3 CrossRef CAS PubMed;
(f) C. Ornelas, New J. Chem., 2011, 35, 1973 RSC;
(g) M. Patra and G. Gasser, ChemBioChem, 2012, 13, 1232 CrossRef CAS PubMed;
(h) M. Patra, G. Gasser and N. Metzler-Nolte, Dalton Trans., 2012, 41, 6350 RSC.
-
(a) O. Buriez, J. M. Heldt, E. Labbe, A. Vessieres, G. Jaouen and C. Amatore, Chem.–Eur. J., 2008, 14, 8195 CrossRef CAS PubMed;
(b) D. Hamels, P. M. Dansette, E. A. Hillard, S. Top, A. Vessières, P. Herson, G. Jaouen and D. Mansuy, Angew. Chem., Int. Ed., 2009, 48, 9124 CrossRef CAS PubMed;
(c) M. Patra, G. Gasser, M. Wenzel, K. Merz, J. E. Bandow and N. Metzler-Nolte, Organometallics, 2010, 29, 4312 CrossRef CAS;
(d) S. Martić, M. Labib, P. O. Shipman and H. B. Kraatz, Dalton Trans., 2011, 40, 7264 RSC;
(e) A. I. Mufula, B. A. Aderibigbe, E. W. Neuse and H. E. Mukaya, J. Inorg. Organomet. Polym. Mater., 2012, 22, 423 CrossRef CAS;
(f) J. Amin, I. S. Chuckowree, M. Wang, G. J. Tizzard, S. J. Coles and J. Spencer, Organometallics, 2013, 32, 5818 CrossRef CAS;
(g) K. Kowalski, J. Skiba, L. Oehninger, I. Ott, J. Solecka, A. Rajnisz and B. Therrien, Organometallics, 2013, 32, 5766 CrossRef CAS.
- For comprehensive reviews, see:
(a) M. P. Doyle, M. A. McKervey and T. Ye, Modern Catalytic Methods for Organic Synthesis with Diazo Compounds, Wiley, New York, NJ, USA, 1998 Search PubMed;
(b) T. Ye and M. A. McKervey, Chem. Rev., 1994, 94, 1091 CrossRef CAS;
(c) H. M. L. Davies and R. E. J. Beckwith, Chem. Rev., 2003, 103, 2861 CrossRef CAS PubMed;
(d) P. M. P. Gois and C. A. M. Afonso, Eur. J. Org. Chem., 2004, 3773 CrossRef CAS;
(e) Z. Zhang and J. Wang, Tetrahedron, 2008, 64, 6577 CrossRef CAS PubMed.
-
(a) R. Pellicciari, R. Fringuelli, P. Ceccherelli and E. Sisani, J. Chem. Soc., Chem. Commun., 1979, 959 RSC;
(b) N. Ikota, N. Takamura, S. D. Young and B. Ganem, Tetrahedron Lett., 1981, 22, 4163 CrossRef CAS;
(c) K. L. Hoffmann and M. Regitz, Tetrahedron Lett., 1983, 24, 5355 CrossRef CAS;
(d) T. Hudlicky, H. F. Olivo, M. G. Natchus, E. F. Umpierrez, E. Pandolfi and C. Volonterio, J. Org. Chem., 1990, 55, 4767 CrossRef CAS;
(e) D. F. Taber and R. S. Hoerrner, J. Org. Chem., 1992, 57, 441 CrossRef CAS;
(f) M. Ohno, M. Itoh, M. Umeda, R. Furuta, K. Kondo and S. Eguchi, J. Am. Chem. Soc., 1996, 118, 7075 CrossRef CAS;
(g) F. Sarabia García, G. M. Pedraza Cebrián, A. Heras López and F. J. López Herrera, Tetrahedron, 1998, 54, 6867 CrossRef;
(h) V. K. Aggarwal, C. G. Sheldon, G. J. Macdonald and W. P. Martin, J. Am. Chem. Soc., 2002, 124, 10300 CrossRef CAS PubMed;
(i) Z. Zhang, W. Shi, J. Zhang, B. Zhang, B. Liu, Y. Liu, B. Fan, F. Xiao, F. Xu and J. Wang, Chem. – Asian J., 2010, 5, 1112 CrossRef CAS PubMed.
-
(a) K. Nagao, M. Chiba and S. W. Kim, Synthesis, 1983, 197 CrossRef CAS;
(b) A. Padwa, S. F. Hornbuckle, Z. Zhang and L. Zhi, J. Org. Chem., 1990, 55, 5297 CrossRef CAS;
(c) T. Ye and M. A. McKervey, Tetrahedron, 1992, 48, 8007 CrossRef CAS;
(d) S. Kanemasa, T. Kanai, T. Araki and E. Wada, Tetrahedron Lett., 1999, 40, 5055 CrossRef CAS;
(e) M. Vitale, T. Lecourt, C. G. Sheldon and V. K. Aggarwal, J. Am. Chem. Soc., 2006, 128, 2524 CrossRef CAS PubMed;
(f) S. Chen, Y. Zhao and J. Wang, Synthesis, 2006, 1705 CAS;
(g) H. Xu, W. Zhang, D. Shu, J. B. Werness and W. Tang, Angew. Chem., Int. Ed., 2008, 47, 8933 CrossRef CAS PubMed;
(h) F. Xu, W. Shi and J. Wang, J. Org. Chem., 2005, 70, 4191 CrossRef CAS PubMed;
(i) W. Shi, F. Xiao and J. Wang, J. Org. Chem., 2005, 70, 4318 CrossRef CAS PubMed.
- N. Ikota, N. Takamura, S. D. Young and B. Ganem, Tetrahedron Lett., 1981, 22, 4163 CrossRef CAS.
- F. Xu, S. Zhang, X. Wu, Y. Liu, W. Shi and J. Wang, Org. Lett., 2006, 8, 3207 CrossRef CAS PubMed.
-
(a) S. Chen, L. Zhang, Y. Du and B. Li, Synlett, 2012, 23, 943 CrossRef CAS PubMed;
(b) Q. Xiao, B. Wang, L. Tian, Y. Yang, J. Ma, Y. Zhang, S. Chen and J. Wang, Angew. Chem., Int. Ed., 2013, 52, 9305 CrossRef CAS PubMed.
- S. Chen, F. Yuan, H. Zhao and B. Li, RSC Adv., 2013, 3, 12616 RSC.
- ESI.†.
Footnote |
† Electronic supplementary information (ESI) available. CCDC 883909. For ESI and crystallographic data in CIF or other electronic format see DOI: 10.1039/c3ra48071b |
|
This journal is © The Royal Society of Chemistry 2014 |
Click here to see how this site uses Cookies. View our privacy policy here.