DOI:
10.1039/C3RA47813K
(Communication)
RSC Adv., 2014,
4, 11152-11158
Pd-catalyzed ligand-free Suzuki reaction of β-substituted allylic halides with arylboronic acids in water†
Received
20th December 2013
, Accepted 11th February 2014
First published on 11th February 2014
Abstract
The catalyst system consisting of Pd(TFA)2 and KOH allows for a wide range of β-substituted allylic halides to react efficiently with various arylboronic acids in neat water under ligand-free conditions, affording the allylated arenes in high yields with broad functional group tolerance and up to 7.4 × 105 TON and 15
416 h−1 TOF.
As one of the most powerful protocols for the construction of C–C bonds in organic synthesis, the Pd-catalyzed Suzuki reaction has been extensively studied with great success during the last several decades.1 Recently there has been much research interest in developing catalyst systems capable of allowing this cross coupling reaction to be performed in water. The attractiveness of water lies in the fact that it is cheap, clean, non-toxic, non-flammable, non-explosive and non-carcinogenic, and its high heat capacity can help prevent exothermic reactions from overheating on a large scale. Obviously, carrying out organic reactions in water would lead to substantial environmental, economical and safety gains.2 As a result, a number of catalytic Suzuki reactions have proved to be feasible in water,3 and strategies developed to overcome the major concern about water solubility of catalysts and substrates include the use of water-soluble ligands,4 phase-transfer catalysis,5 organic co-solvents,6 microwave or ultrasound.7 Of particular note is that recent developments have enabled the aqueous Suzuki reactions to proceed smoothly in neat water under ligand-free conditions without calling for any phase-transfer catalyst, co-solvent or degassing.8
Allylated arenes are valuable reagents for the construction of useful molecules,9 and they are also important structural motifs present in many biologically active natural products.10 Therefore, a significant amount of effort has been focused on the efficient preparation of these compounds, and many methods are now available in the literature.11 Among these known methods, the Pd-catalyzed Suzuki reaction of allylic halides,12,13 esters14 and alcohols15 with organoboronic compounds has received increasing attention due to its simplicity, tolerance of various functional groups and the easy availability of reagents, and it is notable that this kind of transformation could benefit considerably from the use of aqueous solvents as the reaction media.13e,h,k–n,14j,m,15c More recently, Scrivanti and Ueda disclosed that allylic bromides could efficiently react with aryl- and vinylboronic acids under transition-metal-free conditions to give the corresponding products in high yields.16 Nevertheless, despite the exciting progress, those reported examples mainly dealt with the cross-coupling reactions of γ-substituted allylic substrates, and less attention has been paid to the reactions involving β-substituted allylic derivatives, with only several reports available in the literature.13k–n As part of our continuing effort on the development of catalysis in water,17 herein, we report a highly efficient palladium-catalyzed ligand-free Suzuki reaction of β-substituted allylic bromides and chlorides with aryl- and vinylboronic acids in neat water without requiring any organic solvent, surfactant or inert gas protection, in which excellent group compatibility and up to 7.4 × 105 TON and 15
416 h−1 TOF were observed.
Preliminary screening for optimal reaction conditions was performed with (3-bromoprop-1-en-2-yl)benzene (1a) and 4-methoxyphenylboronic acid (2a) as the model substrates. Following the previous reports by Scrivanti and Ueda,16 we first tried the coupling reaction of 1a and 2a without employing any transition-metal catalyst. However, no reaction occurred using either Scrivanti's or Ueda's protocol (Table 1, entries 1 and 2). Obviously, in this case the use of transition-metal catalyst is required. Considering a number of attractive advantages of using water as a solvent for organic reactions,2,3 we therefore chose water as the reaction medium for subsequent study. In the presence of catalytic amount of Pd(OAc)2 (0.01 mol%), we carried out the coupling reaction with NEt3 as the base in pure water, and a 18% yield of the expected product 3aa was achieved (Table 1, entry 3). However, employing Pd2(dba)3, PdCl2 or Pd/C led to lower yields (Table 1, entries 4–6). Pd(PPh3)4 afforded a 40% yield (Table 1, entry 7), and the best yield of 68% was obtained in the case of Pd(TFA)2 (Table 1, entry 8). In order to improve the reaction efficiency, we then explored the performance of other bases. Clearly, the reaction was sensitive to the choice of base (Table 1, entries 9–19). It is notable that HNiPr2, reported to be highly effective in Pd-catalyzed Suzuki reaction of aryl halides with aryl boronic acids in neat water,8a gave a good yield of 85% (Table 1, entry 10), and KOH resulted in the best reactivity with a nearly quantitative yield (Table 1, entry 19). As expected, a control experiment indicated that no reaction took place in the absence of palladium catalyst (Table 1, entry 20). Recent studies disclosed that TEMPO (2,2,6,6-tetramethyl-1-piperidinyloxy) and HQ (hydroquinone) could greatly facilitate the Pd-catalyzed cross coupling reactions,18 but only reduced reactivity was observed in our case (Table 1, entries 21 and 22). Lower temperature disfavored the reaction as the yield of 3aa dropped to 75% at 70 °C (Table 1, entry 23). The protocols developed respectively by Liu,8a Bora8b and Hirao,8c reported to be highly effective for the Suzuki reaction of aryl halides in neat water, were also examined (Table 1, entries 24–26). Only Liu's protocol proved equally effective (Table 1, entry 26), but the high catalyst loading made it less attractive. Remarkably, the catalyst loading could be decreased to 0.001 mol% without compromising catalytic activity (Table 1, entry 27). It is worth noting that at an even lower loading of 0.0001 mol% (S/C = 1
000
000) of the catalyst, a good yield of 74% was still obtained albeit over a longer reaction time of 48 h (Table 1, entry 28), corresponding to 7.4 × 105 TON and 15
416 h−1 TOF. It should be pointed out that the homocoupling reaction of 2a and isomerization of the product were not detected in all cases. Under the same reaction conditions, the reaction of 2a with 2-phenylprop-2-en-1-ol or 2-phenylallyl acetate was attempted, but we did not observe any product. Noteworthy is that all the reactions were performed smoothly in air without an inert atmosphere.
Table 1 Screening conditions for Pd-catalyzed Suzuki reaction of 1a and 2aa
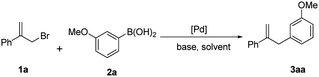
|
Entry |
Solvent |
[Pd] |
Base |
Time (h) |
Yieldb (%) |
Reaction conditions: 1a (1.0 mmol), 2a (1.2 mmol), catalyst (0.01 mol%), base (1.2 mmol), solvent (3.0 mL), 90 °C. nd: not detected. Isolated yield. 1.5 eq. of Cs2CO3 were added. 0.1 eq. of TEMPO was added. 0.1 eq. of HQ was added. Reaction temperature 60 °C. 1a (1.0 mmol), 2a (1.0 mmol), Pd/C (0.3 mol%), base (3.0 mmol), H2O (10.0 mL), 25 °C. 1a (0.50 mmol), 2a (0.55 mmol), PdCl2 (2 mol%), base (1.5 mmol), Na2SO4 (8 mol%), H2O (4.0 mL), 25 °C. 1a (0.50 mmol), 2a (0.75 mmol), Pd(OAc)2 (0.25 mol%), base (1.0 mmol), H2O (1.0 mL), 100 °C. S/C = 100 000. S/C = 1 000 000. |
1 |
Toluene |
None |
K2CO3 |
3 |
nd |
2c |
CH2Cl2–H2O |
None |
Cs2CO3 |
18 |
nd |
3 |
H2O |
Pd(OAc)2 |
NEt3 |
3 |
18 |
4 |
H2O |
Pd2(dba)3 |
NEt3 |
3 |
8 |
5 |
H2O |
PdCl2 |
NEt3 |
3 |
16 |
6 |
H2O |
Pd/C |
NEt3 |
3 |
11 |
7 |
H2O |
Pd(PPh3)4 |
NEt3 |
3 |
40 |
8 |
H2O |
Pd(TFA)2 |
NEt3 |
3 |
68 |
9 |
H2O |
Pd(TFA)2 |
HNEt2 |
3 |
16 |
10 |
H2O |
Pd(TFA)2 |
HNiPr2 |
3 |
85 |
11 |
H2O |
Pd(TFA)2 |
Cy2NMe |
3 |
9 |
12 |
H2O |
Pd(TFA)2 |
NaOAc |
3 |
28 |
13 |
H2O |
Pd(TFA)2 |
NaOH |
3 |
55 |
14 |
H2O |
Pd(TFA)2 |
K2CO3 |
3 |
37 |
15 |
H2O |
Pd(TFA)2 |
KF |
3 |
51 |
16 |
H2O |
Pd(TFA)2 |
Cs2CO3 |
3 |
26 |
17 |
H2O |
Pd(TFA)2 |
Na2CO3 |
3 |
34 |
18 |
H2O |
Pd(TFA)2 |
K3PO4 |
3 |
58 |
19 |
H2O |
Pd(TFA)2 |
KOH |
3 |
95 |
20 |
H2O |
None |
KOH |
3 |
nd |
21d |
H2O |
Pd(TFA)2 |
KOH |
3 |
73 |
22e |
H2O |
Pd(TFA)2 |
KOH |
3 |
60 |
23f |
H2O |
Pd(TFA)2 |
KOH |
3 |
75 |
24g |
H2O |
Pd/C |
K2CO3 |
3 |
nd |
25h |
H2O |
PdCl2 |
K2CO3 |
3 |
nd |
26i |
H2O |
Pd(OAc)2 |
HNiPr2 |
3 |
92 |
27j |
H2O |
Pd(TFA)2 |
KOH |
12 |
86 |
28k |
H2O |
Pd(TFA)2 |
KOH |
48 |
74 |
Having established the optimized reaction conditions, we then investigated the reaction of 1a with other organoboronic acids. As can be seen from Table 2, a range of arylboronic acids reacted smoothly with 1a in neat water in the presence of 0.01 mol% Pd(TFA)2 to produce the expected 2,3-diarypropenes in good to excellent yields in 3 h (Table 2, entries 1–20). It was found that the reaction was highly tolerant of various functional groups with different electronic and steric properties. Notably, the sensitive halogen substituent (Br and Cl) in the aromatic ring of arylboronic acids could well survive under the current conditions, affording the corresponding products in high yields (Table 2, entries 8–11). Interestingly, the presence of a hydroxyl group on the aryl ring of arylboronic acid did not affect the reaction, and the expected product 3am could be obtained in 90% yield (Table 2, entry 12). Steric hindrance seems to have little influence on the reaction efficiency as evidenced by the fact that arylboronic acids bearing substituents at the 2-position showed good reactivity (Table 2, entries 4, 5, 9, 11, 19). The reactions involving heteroarylboronic and alkenylboronic acids were also highly efficient, delivering the corresponding products in 83–93% yields (Table 2, entries 21–25). To showcase the practicability of this process, we tried the cross coupling of 5 g of 1a with 2a (30.61 mmol) in the presence of 0.01 mol% Pd(TFA)2 in water. The reaction afforded the desired product 3aa in 91% isolated yield in 8 h.
Table 2 Pd-catalyzed Suzuki reaction of 1a with organoboronic acids in watera
The reaction of 1a with other organoboronic derivatives under the optimized reaction conditions was subsequently investigated. The arylboronic ester, 4,4,5,5-tetramethyl-2-phenyl-1,3,2-dioxaborolane, underwent smooth allylation in water, affording the expected product 3ab in 78% yield (Scheme 1, (1)). A better yield of 88% was achieved with potassium phenyl trifluoroborate as the coupling partner (Scheme 1, (2)).
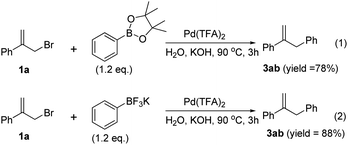 |
| Scheme 1 Allylation of other organoboronic derivatives. | |
We then turned our attention to the coupling reactions of other β-arylated allylic halides with organoboronic acids, and the results are summarized in Table 3. Under the same conditions as described above, it was found that various allylic bromides with different substituents on the aryl ring could undergo successful coupling reaction with a range of organoboronic acids, and the presence of functional groups in both coupling partners did not affect the transformation (Table 3, entries 1–25). For example, the allylic bromide 1g bearing a sensitive bromine substituent on the aryl ring could be efficiently transformed into the target products with high yields, leaving the sp2 C–Br bond intact (Table 3, entries 17 and 18). The effectiveness of the current catalyst system was further demonstrated in the arylation of allylic chloride. When (3-chloroprop-1-en-2-yl)benzene (1k) was employed, the coupling reaction proceeded effectively, affording the target compound 3aa and 3aq in 91% and 89% yield, respectively (Table 3, entries 26 and 27). The β-alkylated allylic halide 1l also cross-coupled with aryl and vinyl boronic acids to give the expected products in good yields (Table 3, entries 28–31).
Table 3 Pd-catalyzed Suzuki reaction of β-arylated allylic halides with organoboronic acids in watera
To expand the scope of our catalyst system further, the coupling reactions of other types of β-substituted allylic halide with organoboronic acids were studied. However no reaction was observed in the coupling reaction of 1m with organoboronic acids under the same reaction conditions. This problem could be resolved simply by increasing the S/C ratio to 1000, which allowed the smooth allylation of 2b, 2q and 2x to give good results (Scheme 2).
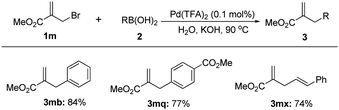 |
| Scheme 2 The reactions of substrate 1m with organoboronic acids. | |
We also explored the reaction of the di-bromo-substituted substrate 1n with arylboronic acids (Scheme 3). It was found that the double arylated products 3ba and 3nq were isolated in good yields, and the vinyl bromide could not be tolerated. Similar observation has been reported by Ikegami and co-workers in the aqueous Suzuki reaction catalyzed by assembled Pd complex.13l
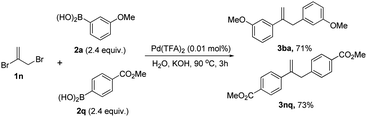 |
| Scheme 3 Arylation of the di-bromo-substituted substrate 1n. | |
Conclusions
In summary, we have demonstrated that in the presence of catalytic amount of Pd(TFA)2 the allylation of various organoboronic acids with different β-substituted allylic halides could proceed efficiently in neat water without using any ligand and organic solvent. Key to the success of the reaction is the choice of base and catalyst precursor. The reaction was found to be compatible with a wide range of functional groups in both coupling partners, and could be performed in air under a catalyst loading as low as 0.00001 mol% with TON up to up to 7.4 × 105 and TOF up to 15
416 h−1. Unlike most of the older ligand-free water based methods, our method did not require the addition of surfactants, phase-transfer agents or co-solvents or using microwave or ultrasound. The present catalysis offers an efficient, green and practical new method for accessing allylated arenes.
Experimental section
General procedure for allylation of arylboronic acid in water
A mixture of allylic halide 1 (1.0 mmol), arylboronic acid 2 (1.20 mmol), KOH (84.17 mg, 1.5 mmol), palladium trifluoroacetate (0.033 mg, 0.0001 mmol) in water (3.0 mL) in a round-bottom flask was stirred and heated at 90 °C for 3 h. Then the flask was removed from the oil bath and cooled to room temperature. Water (5.0 mL) was added, and the mixture was extracted with CH2Cl2 (3 × 5.0 mL). The combined organic extracts were dried over anhydrous Na2SO4, concentrated in vacuo. The residue was then purified via flash chromatography on silica gel using a mixture of ethyl acetate and hexane to give the pure product.
Acknowledgements
Financial support from the National Natural Science Foundation of China (21072225, 21172258, 21372258 and 91127039) is greatly acknowledged.
Notes and references
-
(a) N. Miyaura and A. Suzuki, Chem. Rev., 1995, 95, 2457–2483 CrossRef CAS;
(b) A. Suzuki, J. Organomet. Chem., 1999, 576, 147–168 CrossRef CAS;
(c) S. Kotha, K. Lahiri and D. Kashinath, Tetrahedron, 2002, 58, 9633–9695 CrossRef CAS;
(d) F. Bellina, A. Carpita and R. Rossi, Synthesis, 2004, 2419–2440 CAS;
(e) K. C. Nicolaou, P. G. Bulger and D. Sarlah, Angew. Chem., Int. Ed., 2005, 44, 4442–4489 CrossRef CAS PubMed;
(f) N. T. S. Phan, M. Van Der Sluys and C. W. Jones, Adv. Synth. Catal., 2006, 348, 609–679 CrossRef CAS;
(g) R. Martin and S. L. Buchwald, Acc. Chem. Res., 2008, 41, 1461–1473 CrossRef CAS PubMed;
(h) F. Alonso, I. P. Beletskaya and M. Yus, Tetrahedron, 2008, 64, 3047–3101 CrossRef CAS PubMed;
(i) R. Rossi, F. Bellina and M. Lessi, Tetrahedron, 2011, 67, 6969–7025 CrossRef CAS PubMed.
-
(a) W. J. W. Watson, Green Chem., 2012, 14, 251–259 RSC;
(b) M. Lamblin, L. Nassar-Hardy, J.-C. Hierso, E. Fouquet and F.-X. Felpin, Adv. Synth. Catal., 2010, 352, 33–79 CrossRef CAS;
(c) C.-J. Li, Chem. Rev., 2005, 105, 3095–3165 CrossRef CAS PubMed;
(d) C.-J. Li, Acc. Chem. Res., 2002, 35, 533–538 CrossRef CAS PubMed.
- V. Polshettiwar, A. Decottignies, C. Len and A. Fihri, ChemSusChem, 2010, 3, 502–522 CrossRef CAS PubMed.
-
(a) M. A. Kinzhalov, K. V. Luzyanin, V. P. Boyarskiy, M. Haukka and V. Yu. Kukushkin, Organometallics, 2013, 32, 5212–5223 CrossRef CAS;
(b) N. Liu, C. Liu and Z. Jin, Green Chem., 2012, 14, 592–597 RSC;
(c) A. Fihri, D. Luart, C. Len, A. Solhy, C. Chevrinb and V. Polshettiwar, Dalton Trans., 2011, 40, 3116–3121 RSC;
(d) C. A. Fleckensteinab and H. Plenio, Chem. Soc. Rev., 2010, 39, 694–711 RSC;
(e) K. H. Shaughnessy, Chem. Rev., 2009, 109, 643–710 CrossRef CAS PubMed;
(f) C. Fleckenstein, S. Roy, S. Leuthauber and H. Plenio, Chem. Commun., 2007, 2870–2872 RSC;
(g) C. A. Fleckenstein and H. Plenio, Green Chem., 2007, 9, 1287–1291 RSC;
(h) R. Huang and K. H. Shaughnessy, Organometallics, 2006, 25, 4105–4112 CrossRef CAS.
-
(a) C. Rohlich, A. S. Wirth and K. Kohler, Chem. –Eur. J., 2012, 18, 15485–15494 CrossRef PubMed;
(b) S.-L. Mao, Y. Sun, G.-A. Yu, C. Zhao, Z.-J. Han, J. Yuan, X. Zhu, Q. Yang and S.-H. Liu, Org. Biomol. Chem., 2012, 10, 9410–9417 RSC;
(c) S. S. Soomro, C. Röhlich and K. Köhler, Adv. Synth. Catal., 2011, 353, 767–775 CrossRef CAS;
(d) M. J. Jin and D. H. Lee, Angew. Chem., Int. Ed., 2010, 49, 1119–1122 CrossRef CAS PubMed;
(e) B. Basu, K. Biswas, S. Kundu and S. Ghosh, Green Chem., 2010, 12, 1734–1738 RSC;
(f) B. H. Lipshutz, T. B. Petersen and A. R. Abela, Org. Lett., 2008, 10, 1333–1336 CrossRef CAS PubMed.
-
(a) J.-P. Wan, C. Wang, R. Zhou and Y. Liu, RSC Adv., 2012, 2, 8789–8792 RSC;
(b) C. Liu, Q. Ni, F. Bao and J. Qiu, Green Chem., 2011, 13, 1260–1266 RSC;
(c) F. Li and T. S. A. Hor, Adv. Synth. Catal., 2008, 350, 2391–2400 CrossRef CAS;
(d) L. R. Moore, E. C. Western, R. Craciun, J. M. Spruell, D. A. Dixon, K. P. O'Halloran and K. H. Shaughnessy, Organometallics, 2008, 27, 576–593 CrossRef CAS;
(e) P. Capek, R. Pohl and M. Hocek, Org. Biomol. Chem., 2006, 4, 2278–2284 RSC;
(f) L. Liu, Y. Zhang and Y. Wang, J. Org. Chem., 2005, 70, 6122–6125 CrossRef CAS PubMed.
-
(a) A. L. F. de Souza, L. C. da Silva, B. L. Oliveira and O. A. C. Antunes, Tetrahedron Lett., 2008, 49, 3895–3989 CrossRef CAS PubMed;
(b) J. Zhang, F. Yang, G. Ren, T. C. Mak, M. Song and Y. Wu, Ultrason. Sonochem., 2008, 15, 115–118 CrossRef CAS PubMed;
(c) V. Polackova, M. Hutka and S. Toma, Ultrason. Sonochem., 2005, 12, 99–102 CrossRef CAS PubMed;
(d) A. Kirschning, W. Solodenko, U. Schön, J. Messinger and A. Glinschert, Synlett, 2004, 1699–1702 CrossRef PubMed.
-
(a) C. Liu, Y. Zhang, N. Liu and J. Qiu, Green Chem., 2012, 14, 2999–3003 RSC;
(b) M. Mondal and U. Bora, Green Chem., 2012, 14, 1873–1876 RSC;
(c) H. Sakurai, T. Tsukuda and T. Hirao, J. Org. Chem., 2002, 67, 2721–2722 CrossRef CAS PubMed;
(d) N. A. Bumagin and V. V. Bykov, Tetrahedron, 1997, 53, 14437–14450 CrossRef CAS;
(e) N. A. Bumagin, V. V. Bykov and I. P. Beletskaya, Izv. Akad. Nauk SSSR, Ser. Khim., 1989, 2394–2401 CAS.
- For selected examples, see:
(a) J. Camacho-Bunquin, M. J. Ferguson and J. M. Stryker, J. Am. Chem. Soc., 2013, 135, 5537–5540 CrossRef CAS PubMed;
(b) C. Qin, W. Zhou, F. Chen, Y. Ou and N. Jiao, Angew. Chem., Int. Ed., 2011, 50, 12595–12599 CrossRef CAS PubMed;
(c) H. Chen, H. Jiang, C. Cai, J. Dong and W. Fu, Org. Lett., 2011, 13, 992–994 CrossRef CAS PubMed;
(d) B. Zupancic, B. Mohar and M. Stephan, Org. Lett., 2010, 12, 3022–3025 CrossRef CAS PubMed;
(e) B. Kramer and S. R. Waldvogel, Angew. Chem., Int. Ed., 2004, 43, 2446–2449 CrossRef CAS PubMed;
(f) A. Scarso, M. Colladon, P. Sgarbossa, C. Santo, R. A. Michelin and G. Strukul, Organometallics, 2010, 29, 1487–1497 CrossRef CAS;
(g) M. A. Brown and M. A. Kerr, Tetrahedron Lett., 2001, 42, 983–985 CrossRef CAS;
(h) A. Fürstner, O. R. Thiel and G. Blanda, Org. Lett., 2000, 2, 3731–3734 CrossRef PubMed.
- For selected examples, see:
(a) J. L. Farmer, H.
N. Hunter and M. G. Organ, J. Am. Chem. Soc., 2012, 134, 17470–17473 CrossRef CAS PubMed;
(b) H. Ohmiya, Y. Makida, D. Li, M. Tanabe and M. Sawamura, J. Am. Chem. Soc., 2010, 132, 879–889 CrossRef CAS PubMed;
(c) G. Ni, Q.-J. Zhang, Z.-F. Zheng, R.-Y. Chen and D.-Q. Yu, J. Nat. Prod., 2009, 72, 966–968 CrossRef CAS PubMed;
(d) A. Demotie, I. J. S. Fairlamb, F.-J. Lu, N. J. Shaw, P. A. Spencer and J. Southgate, Bioorg. Med. Chem. Lett., 2004, 14, 2883–2887 CrossRef CAS PubMed;
(e) B. M. Trost, O. R. Thiel and H.-C. Tsui, J. Am. Chem. Soc., 2002, 124, 11616–11617 CrossRef CAS PubMed;
(f) B. M. Trost and F. D. Toste, J. Am. Chem. Soc., 2000, 122, 11262–11263 CrossRef CAS.
- For some recent examples, see:
(a) H. Wang, N. Schroder and F. Glorius, Angew. Chem., Int. Ed., 2013, 52, 5386–5389 CrossRef CAS PubMed;
(b) S. Wang, Q. Qian and H. Gong, Org. Lett., 2012, 14, 3352–3355 CrossRef CAS PubMed;
(c) B. J. Stokes, S. M. Opra and M. S. Sigman, J. Am. Chem. Soc., 2012, 134, 11408–11411 CrossRef CAS PubMed;
(d) L. L. Anka-Lufford, M. R. Prinsell and D. J. Weix, J. Org. Chem., 2012, 77, 9989–10000 CrossRef CAS PubMed;
(e) B. Yao, Y. Liu, M.-K. Meng, J.-H. Li, R.-Y. Tang, X.-G. Zhang and C.-L. Deng, Adv. Synth. Catal., 2012, 354, 1069–1076 CrossRef CAS;
(f) J. Wang, Z. Cui, Y. Zhang, H. Li, L.-M. Wu and Z. Liu, Org. Biomol. Chem., 2011, 9, 663–666 RSC;
(g) J. Cvengros, J. Schütte, N. Schlorer, J. Neudorfl and H.-G. Schmalz, Angew. Chem., Int. Ed., 2009, 48, 6148–6151 CrossRef CAS PubMed;
(h) S. E. Denmark and N. S. Werner, J. Am. Chem. Soc., 2008, 130, 16382–16393 CrossRef CAS PubMed;
(i) S. Sebelius, V. J. Olsson, O. A. Wallner and K. J. Szabo, J. Am. Chem. Soc., 2006, 128, 8150–8151 CrossRef CAS PubMed;
(j) S. Hayashi, K. Hirano, H. Yorimitsu and K. Oshima, J. Am. Chem. Soc., 2006, 128, 2210–2211 CrossRef CAS PubMed;
(k) B. Mariampillai, C. Herse and M. Lautens, Org. Lett., 2005, 7, 4745–4747 CrossRef CAS PubMed.
- F. C. Pigge, Synthesis, 2010, 1745–1762 CrossRef CAS PubMed.
-
(a) L. Chiummiento, M. Funicello, P. Lupattelli and F. Tramutola, Org. Lett., 2012, 14, 3928–3931 CrossRef CAS PubMed;
(b) J. F. Civicos, D. A. Alonso and C. Najera, Adv. Synth. Catal., 2011, 353, 1683–1687 CrossRef CAS;
(c) R. Ghosh, N. N. Adarsh and A. Sarkar, J. Org. Chem., 2010, 75, 5320–5322 CrossRef CAS PubMed;
(d) B. Crociani, S. Antonaroli, M. Burattini, P. Paolib and P. Rossi, Dalton Trans., 2010, 39, 3665–3672 RSC;
(e) E. Alacid and C. Najera, J. Organomet. Chem., 2009, 694, 1658–1665 CrossRef CAS PubMed;
(f) D. C. Gerbino, S. D. Mandolesi, H.-G. Schmalz and J. C. Podesta, Eur. J. Org. Chem., 2009, 3964–3972 CrossRef CAS;
(g) D. Srimani and A. Sarkar, Tetrahedron Lett., 2008, 49, 6304–6307 CrossRef CAS PubMed;
(h) G. W. Kabalka, E. Dadusha and M. Al-Masumb, Tetrahedron Lett., 2006, 47, 7459–7461 CrossRef CAS PubMed;
(i) C. Bonini, L. Chiummiento, M. De Bonis and M. Funicello, Tetrahedron: Asymmetry, 2006, 17, 2919–2924 CrossRef CAS PubMed;
(j) R. Singh, M. S. Viciu, N. Kramareva, O. Navarro and S. P. Nolan, Org. Lett., 2005, 7, 1829–1832 CrossRef CAS PubMed;
(k) C. Najera, J. Gil-Molto and S. Karlstrom, Adv. Synth. Catal., 2004, 346, 1798–1811 CrossRef CAS;
(l) Y. M. A. Yamada, K. Takeda, H. Takahashi and S. Ikegami, J. Org. Chem., 2003, 68, 7733–7741 CrossRef CAS PubMed;
(m) D. A. Alonso, C. Najera and M. C. Pacheco, J. Org. Chem., 2002, 67, 5588–5594 CrossRef CAS PubMed;
(n) L. Botella and C. Najera, J. Organomet. Chem., 2002, 663, 46–57 CrossRef CAS;
(o) M. Moreno-Manas, R. Pleixats and S. Villarroya, Organometallics, 2001, 20, 4524–4528 CrossRef CAS;
(p) M. Moreno-Maiias, F. Pajuelo and R. Pleixats, J. Org. Chem., 1995, 60, 2396–2397 CrossRef;
(q) N. A. Bumagin, I. G. Bumagina, A. N. Kashin and I. P. Beletskaya, Dokl. Akad. Nauk SSSR, 1981, 261, 1141–1144 CAS.
-
(a) Y. M. A. Yamada, S. M. Sarkar and Y. Uozumi, J. Am. Chem. Soc., 2012, 134, 3190–3198 CrossRef CAS PubMed;
(b) S. M. Sarkar, Y. Uozumi and Y. M. A. Yamada, Angew. Chem., Int. Ed., 2011, 50, 9437–9441 CrossRef CAS PubMed;
(c) Y. Makida, H. Ohmiya and M. Sawamura, Chem. –Asian J., 2011, 6, 410–414 CrossRef CAS PubMed;
(d) D. Li, T. Tanaka, H. Ohmiya and M. Sawamura, Org. Lett., 2010, 12, 3344–3347 CrossRef CAS PubMed;
(e) H. Ohmiya, N. Yokokawa and M. Sawamura, Org. Lett., 2010, 12, 2438–2440 CrossRef CAS PubMed;
(f) H. Ohmiya, Y. Makida, D. Li, M. Tanabe and M. Sawamura, J. Am. Chem. Soc., 2010, 132, 879–889 CrossRef CAS PubMed;
(g) V. Maslak, Z. Tokic-Vujosevic and R. N. Saicic, Tetrahedron Lett., 2009, 50, 1858–1860 CrossRef CAS PubMed;
(h) Y. M. A. Yamada, T. Watanabe, K. Toriib and Y. Uozumi, Chem. Commun., 2009, 5594–5596 RSC;
(i) H. Ohmiya, Y. Makida, T. Tanaka and M. Sawamura, J. Am. Chem. Soc., 2008, 130, 17276–17277 CrossRef CAS PubMed;
(j) T. Mino, K. Kajiwara, Y. Shirae, M. Sakamoto and T. Fujita, Synlett, 2008, 2711–2715 CrossRef PubMed;
(k) G. W. Kabalka and M. Al-Masum, Org. Lett., 2006, 8, 11–13 CrossRef CAS PubMed;
(l) D. Bouyssi, V. Gerusz and G. Balme, Eur. J. Org. Chem., 2002, 2445–2448 CrossRef CAS;
(m) Y. Uozumi, H. Danjo and T. Hayashi, J. Org. Chem., 1999, 64, 3384–3388 CrossRef CAS.
-
(a) M. L. Kantam, K. B. S. Kumar and B. Sreedhar, J. Org. Chem., 2008, 73, 320–322 CrossRef CAS PubMed;
(b) H. Tsukamoto, T. Uchiyama, T. Suzuki and Y. Kondo, Org. Biomol. Chem., 2008, 6, 3005–3013 RSC;
(c) K. Manabe, K. Nakada, N. Aoyama and S. Kobayashi, Adv. Synth. Catal., 2005, 347, 1499–1503 CrossRef CAS;
(d) Y. Kayaki, T. Koda and T. Ikariya, Eur. J. Org. Chem., 2004, 4989–4993 CrossRef CAS;
(e) H. Tsukamoto, M. Sato and Y. Kondo, Chem. Commun., 2004, 1200–1201 RSC.
-
(a) A. Scrivanti, V. Beghetto, M. Bertoldini and U. Matteoli, Eur. J. Org. Chem., 2012, 264–268 CrossRef CAS;
(b) M. Ueda, K. Nishimura, R. Kashima and I. Ryu, Synlett, 2012, 1085–1089 CrossRef CAS PubMed.
-
(a) Z. Jiang, L. Zhang, C. Dong, X. Su, H. Li, W. Tang, L. Xu and Q. Fan, RSC Adv., 2013, 3, 1025–1028 RSC;
(b) Y. Deng, Z. Jiang, M. Yao, D. Xu, L. Zhang, H. Li, W. Tang and L. Xu, Adv. Synth. Catal., 2012, 354, 899–907 CrossRef CAS;
(c) J. Tan, W. Tang, Y. Sun, Z. Jiang, F. Chen, L. Xu, Q. Fan and J. Xiao, Tetrahedron, 2011, 67, 6206–6213 CrossRef CAS PubMed.
-
(a) T. Lu, C. Xue and F. Luo, Tetrahedron Lett., 2003, 44, 1587–1590 CrossRef CAS;
(b) M. Lemhadri, H. Doucet and M. Santelli, Synlett, 2006, 2935–2940 CAS.
Footnote |
† Electronic supplementary information (ESI) available. See DOI: 10.1039/c3ra47813k |
|
This journal is © The Royal Society of Chemistry 2014 |
Click here to see how this site uses Cookies. View our privacy policy here.