DOI:
10.1039/C3RA46715E
(Paper)
RSC Adv., 2014,
4, 13533-13545
Synthesis and discovery of andrographolide derivatives as non-steroidal farnesoid X receptor (FXR) antagonists†
Received
15th November 2013
, Accepted 28th January 2014
First published on 28th January 2014
Abstract
Based upon the discovery of the natural compound andrographolide (1) as a non-steroidal farnesoid X receptor (FXR) antagonist, a series of andrographolide derivatives were designed and synthesized accordingly. Our primary SAR studies demonstrated that 14-phenoxy andrographolide scaffold is an excellent structural pharmacophore for FXR antagonists. Remarkably, 14β-compounds of 12b, 12f and 10g were found to be the most potent FXR antagonists in this work. Structural docking discovered that the phenoxy substitution at the 14-position and the modification at 3,19-positions altered the putative binding positions of small FXR ligands, resulting in their FXR antagonistic activity discrepancy.
Introduction
Nuclear receptors are classified as ligand-activated transcription factors that regulate the expression of specific target genes and are involved in several physiological functions including reproduction, development, and metabolism.1 Farnesoid X receptor (FXR), also known as bile acid receptor (BAR) or NR1H4, is a nuclear receptor that is encoded by the NR1H4 gene in humans and expressed at high levels in the liver, intestine and other cholesterol-rich tissues.1,2 Cholic acid (CA), chenodeoxycholic acid (CDCA), and other bile acids are natural ligands2–5 for FXR (Fig. 1).
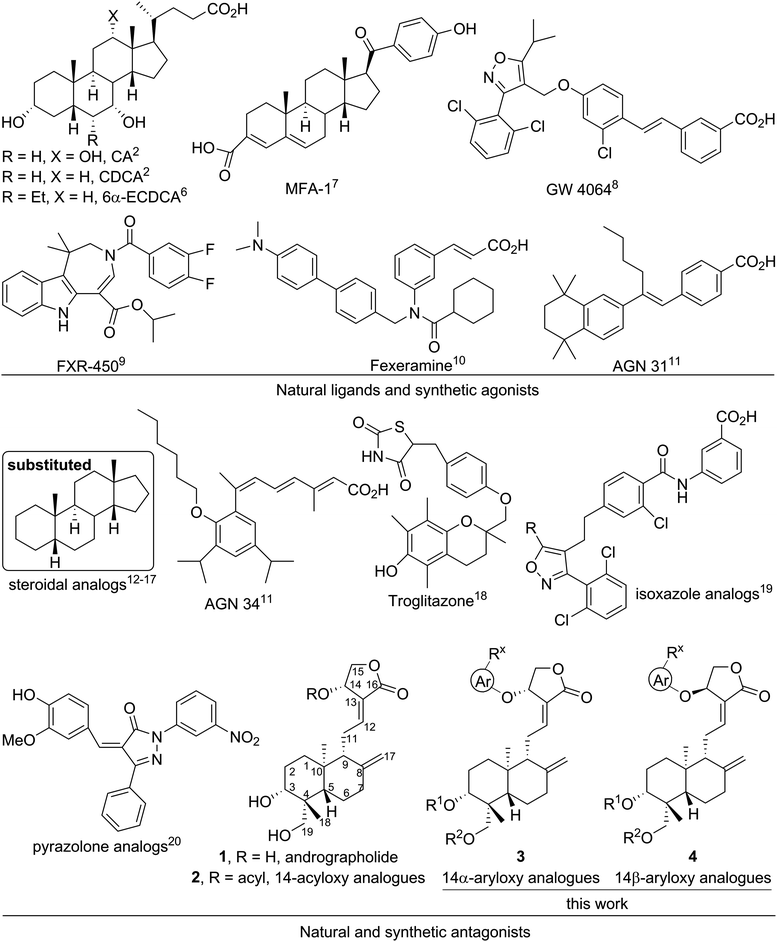 |
| Fig. 1 Natural and synthetic known ligands for FXR and general structures of andrographolide and their derivatives. | |
After activated by bile acid ligand/s,2–5 FXR translocates to the cell nucleus, where FXR recruits retinoid X receptor (RXR) to form a heterodimer and then binds to hormone response elements on DNA, which up- or down-regulates the expression of certain genes.1,2 The down-regulation of CYP7A1 (ref. 21) (cholesterol 7α-hydroxylase) of the rate-limiting enzyme in bile acid synthesis from cholesterol is one of the primary functions of FXR activation, resulting in a negative feedback in which synthesis of bile acids is inhibited if the cellular levels of bile acids are high.1–5 It is reported that FXR has an active role in regulating cholesterol, lipoprotein, and glucose metabolism and its association with liver disease,22,23 liver regeneration24 and tumorigenesis,25 suggesting that FXR represents an attractive pharmacological target.26,27
Currently, most studies are focused on FXR agonists (Fig. 1).6–11 Because it is possible that FXR over-regulation causes some diseases and an unnatural FXR agonist diverts the normal pathway to result in potentially undesirable side effects,25–28 it is necessary to develop FXR antagonists as FXR modulators.
The known FXR antagonists12–20 (Fig. 1) are mainly derived from a steroidal scaffold and a finite number of other structural skeletons have been reported in recent years. Thus, seeking potent, structurally diverse and non-steroidal FXR antagonists should be an important step forward in this field. The utilization of natural products as a source of structural or functional diversity for the design and synthesis of novel molecules has been an important aspect of new drug design.29,30 So as to increase structural diversity of FXR antagonists, we launched a project initiated by the high-throughput screening of the natural compound libraries from a series of Traditional Chinese Medicines (TCMs) based on FXR luciferase activity assay.31 Andrographolide (1, Fig. 1),32,33 a diterpene ester from Andrographis panniculata (Burm.f.) Nees, was identified as a weak FXR antagonist (IC50 = 9.7 μM, Fig. 2).
Andrographolide (1) is the representative active ingredient of Andrographis panniculata (Burm.f.) Nees and it works as a broad therapeutic agent.34,35 Although 1 has a molecular weight of 350 Da, 3 hydrogen bond donors, 5 hydrogen bond acceptors and 2.1186 of the calculated Log
P (Table S1†, entry 23), which conforms to the “Rule of Five”, its poor water-solubility and also relatively low lipo-solubility, weak potency and inadequate therapeutic efficacy restrict its further application. To improve its physiochemical properties and pharmaceutical features, numerous andrographolide derivatives have been reported from time to time, especially preparation of 14-acyloxy andrographolide derivatives36–40 (2, Fig. 1). Herein, we describe the synthesis and SAR studies of 14-aryloxy andrographolide derivatives (3 and 4, Fig. 1), leading to the identification of a series of 14-phenoxy andrographolide derivatives as more potent FXR antagonists than the lead andrographolide (1).41
Results and discussion
In our preliminary study, andrographolide (1) was found to be a weak FXR antagonist (IC50 = 9.7 μM) in the concentration-dependent mode (Fig. 2),31 this result spurred us on to discover more potent FXR antagonists on the basis of andrographolide derivatives. As 14-acyloxy andrographolide esters (2, Fig. 1) were effective in some reported studies for other assays,36–38 we were interested in investigating 14-modified andrographolide derivatives as FXR novel antagonists.
In an initial exploration step, some simple derivatives of andrographolide were studied (Scheme 1). Starting from 1, 3,19-acetonylidene protection was conducted in DCM and catalyzed by PPTS yielding 5 as reported.36–38 Inversion of 14α-OH of 5 into 14β-OAc of 6 was achieved by Mitsunobu reaction under normal conditions. 14-Epimeric andrographolide (8) or its 14β-acetylated derivative (7) was generated in MeOH/H2O (4/1) with TsOH·H2O by partial (at 20 °C) or complete (40 °C) hydrolysis of 6, respectively. Finally, 14β-3,19-acetonylidene derivative (9) was made by the same preparation conditions as 5.
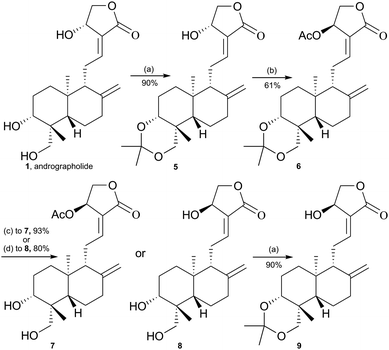 |
| Scheme 1 Reagents and conditions: (a) anhydrous DCM, 2,2-dimethoxypropane, PPTS, 40 °C; (b) anhydrous THF; 5 (1.0 eq.), anhydrous HOAc (1.5 eq.), DIAD (1.5 eq.), PPh3 (1.5 eq.), 0 °C to room temperature; (c) MeOH/H2O (4/1), TsOH·H2O, 20 °C; (d) MeOH/H2O (4/1), TsOH·H2O, 40 °C. | |
FXR antagonistic results of the synthesized compounds were measured by the luciferase reporter assay31 and shown in Table 1 (entries 23–28). The antagonistic potencies at 5.0 μM are depicted in Fig. 2. Compared to 1, 3,19-isopropylidene andrographolide (5) slightly improved the FXR antagonistic activity. 14β-Epimeric andrographolide (8) was a much weaker FXR antagonist than 1 and 14β-acetylated andrographolide (7) became inactive to FXR. Unlike the relationship between 1 and 5, 3,19-isopropylidene 14β-andrographolide (9) was indeed a weaker FXR antagonist than 8. 14β-Acetylated 3,19-isopropylidene andrographolide (6) is more active than 14β-acetylated 3,9-diol analogue 7 in antagonizing FXR. By comparison of 14β-andrographolide (8) with its modified derivatives (6, 7, 9), it was discovered that 3,19-isopropylidene modification alone (9) almost did not change the antagonistic activity and 14-acetylation modification alone (7) decreased the antagonistic activity, but simultaneous modifications (6) of 3,19-isopropylidene and 14-acetylation enhanced somewhat the antagonistic activity. It was inferred from these preliminary data (Fig. 2 and Table 1, entries 23–28) that the optimal combination of 3,19- and 14-modifications including 14α- or 14β-configuration is possibly helpful for the FXR antagonistic activity.
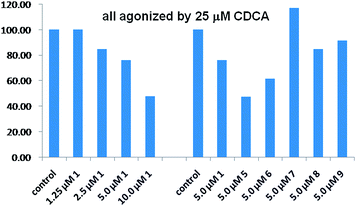 |
| Fig. 2 The FXR antagonistic activities of compounds 1 and 5–9. Control (100%) is the activity of FXR in the presence of 25.0 μM CDCA. | |
Considering that aryloxy ether is chemically more stable than the acyloxy ester, we are interested in 14-aryloxy ether analogues of andrographolide (Fig. 1) in our exploration. Incrementing the structural diversity is the important superiority of 14-aryloxy ether by the utilizing of varied aromatic skeletons (e.g. phenyl, naphthyl, quinolinyl, pyridinyl, etc.) and the incorporation of different substituents into aromatic scaffolds. In this work, 14-phenoxy andrographolide derivatives (Ar = Ph, Fig. 1, series 3 and 4) were firstly studied.
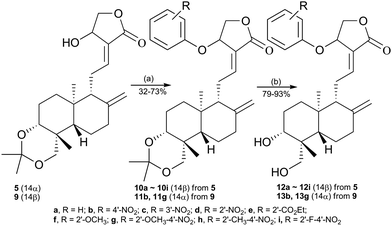 |
| Scheme 2 Reagents and conditions: (a) anhydrous THF; 5 or 9 (1.0 eq.), phenol (1.5 eq.), DIAD (1.5 eq.), PPh3 (1.5 eq.), 0 °C to room temperature; (b) MeOH/H2O (4/1), TsOH·H2O, 20 °C. | |
As shown in Scheme 2, 14α-compound 5 or its 14β-epimer 9 were used as starting materials for the synthesis of 14β- or 14α-phenoxy andrographolide derivatives, respectively. The key synthetic approach to 14-phenoxy andrographolide derivatives is the Mitsunobu reaction. Diverse phenols were used to prepare two series of 14β-phenoxy (10) and 14α-phenoxy (11) compounds. The reaction was performed in general from 0 °C to room temperature in anhydrous THF and afforded 10 or 11 in mild to moderate isolated yield depending on the distinct phenol property. Removal of the protective group of 3,19-acetonylidene from 10 or 11 by TsOH·H2O in MeOH/H2O (4/1) at 20 °C, the series of compounds 12 or 13 were obtained in high yields, respectively. It is worthwhile noting that α-isomers of 11 and 13 were generally less stable than their corresponding β-isomers of 10 and 12.
In order to confirm the stereochemistry of C-14 in these series, the single crystal of 10b was cropped from petroleum ether/ethyl acetate (5/4) and its crystal structure was determined as shown in Fig. 3a by X-ray crystallography, which is in accordance with our expected structure. The crystal packing diagram (Fig. 3b) of 10b demonstrated that the molecule 10b is stabilized and linked by the intermolecular hydrogen bonds of C(9)–H(β)⋯O(4′-NO2) and C(14)–H(α)⋯O(lactone)–C(15).
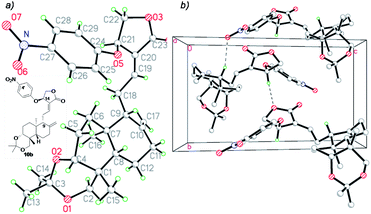 |
| Fig. 3 Molecular and crystal structures (a) and crystal packing diagram (b) of 10b. ESI.† | |
The calculated data of “Lipinski's Rule of Five” of 1 and these synthesized compounds 5–9 and 10–13 are included in Table S1.† Even some of these compounds do not conform very well to the “Rule of Five”; they have the potential to be optimized and developed into drug-like candidates in the future. FXR antagonistic activity and the cellular toxicity of 1 and these synthesized compounds 5–9, and 10–13 are listed in Table 1.
At first, 14β-phenoxy andrographolide derivative 10a bearing the 3,19-acetonylidene group was found to be inactive (Table 1, entry 1); however, its hydrolyzed product of 3,19-diol 12a (Table 1, entry 2) exhibited as a moderate FXR antagonist (IC50 = 5.7 μM), is better than our lead 1. Then, we moved to incorporate substituted groups into the phenyl ring at the 14-position to investigate the structure–activity relationship (SAR). 4′-Nitrophenoxy compounds of 10b with 3,19-acetonylidene group and 12b of 3,19-diol derivative were designed and tested. Compound 10b (IC50 = 8.6 μM; Table 1, entry 3) is less potent than 12a but it is much more active than its corresponding counterpart 10a as a FXR antagonist; notably, the IC50 value of 12b reached 0.55 μM (Table 1, entry 4) in the FXR antagonistic activity, indicating that the 14-(4′-nitrophenoxy) group is an important pharmacophore (10a < 10b and 12a < 12b). In addition, it seems that the 3,19-acetonylidene group decreases the FXR antagonistic activity (10a < 12a and 10b < 12b) for these compounds.
Table 1 FXR antagonistic activity of synthesized compounds 1, 5–9, 10–13
Entry |
Cmpda |
R |
14-Isomer |
IC50b |
CC50c |
See Scheme 2. FXR-transfected 293T cells were agonized by 25.0 μM CDCA in all experiments and the vehicle control was set as 100%, “NA” means “not active”. The 293T cells were used to test compound's cellular toxicity, “—” represents “not detected”. |
1 |
10a |
H |
β |
NA |
— |
2 |
12a |
H |
β |
5.7 |
>15.0 |
3 |
10b |
4′-NO2 |
β |
8.6 |
>15.0 |
4 |
12b |
4′-NO2 |
β |
0.55 |
>15.0 |
5 |
10c |
3′-NO2 |
β |
NA |
— |
6 |
12c |
3′-NO2 |
β |
NA |
— |
7 |
10d |
2′-NO2 |
β |
3.0 |
8.5 |
8 |
12d |
2′-NO2 |
β |
NA |
— |
9 |
10e |
2′-CO2Et |
β |
2.9 |
12.3 |
10 |
12e |
2′-CO2Et |
β |
3.5 |
>15.0 |
11 |
10f |
2′-OCH3 |
β |
2.4 |
>15.0 |
12 |
12f |
2′-OCH3 |
β |
0.98 |
>15.0 |
13 |
10g |
2′-OCH3-4′-NO2 |
β |
2.0 |
>15.0 |
14 |
12g |
2′-OCH3-4′-NO2 |
β |
11.9 |
>15.0 |
15 |
10h |
2′-CH3-4′-NO2 |
β |
6.3 |
>15.0 |
16 |
12h |
2′-CH3-4′-NO2 |
β |
NA |
— |
17 |
10i |
2′-F-4′-NO2 |
β |
3.9 |
>15.0 |
18 |
12i |
2′-F-4′-NO2 |
β |
NA |
— |
19 |
11b |
4′-NO2 |
α |
15.1 |
>15.0 |
20 |
13b |
4′-NO2 |
α |
16.0 |
>15.0 |
21 |
11g |
2′-OCH3-4′-NO2 |
α |
2.5 |
12.0 |
22 |
13g |
2′-OCH3-4′-NO2 |
α |
7.1 |
>15.0 |
23 |
1, Andrographolide |
α |
9.7 |
>15.0 |
24 |
5 |
α |
9.0 |
>15.0 |
25 |
6 |
β |
9.3 |
>15.0 |
26 |
7 |
β |
NA |
— |
27 |
8 |
β |
>15.0 |
— |
28 |
9 |
β |
>15.0 |
— |
After the above, 3′- and 2′-nitrophenoxy andrographolide derivatives 10c and 12c, 10d and 12d, respectively, were designed to investigate the effects of different nitro positions on the FXR antagonistic activity in comparison to 4′-nitrophenoxy analogues 10b and 12b. Among these, 10c and 12c of 3′-nitrophenoxy analogues, and 12d of the 2′-nitrophenoxy analogue did not express FXR antagonistic activity (Table 1, entries 5, 6 and 8); nevertheless, 10d of the 2′-nitrophenoxy analogue with 3,19-acetonylidene (Table 1, entry 7) showed a good FXR antagonistic activity (IC50 = 3.0 μM) but it possessed an obvious cellular toxicity (CC50 = 8.5 μM). Based upon these results, we further explored other substitution at the 2′-position of the 14-phenoxy group. Compound 10e with an electron-withdrawing group of 2′-carboxylic ester (Table 1, entry 9) exhibited similar FXR antagonistic activity (IC50 = 2.9 μM) and cellular toxicity (CC50 = 12.3 μM) to its 2′-nitro counterpart 10d. 2′-Carboxylic ester 3,19-diol compound 12e (Table 1, entry 10), which is different from its inactive 2′-nitro-3,19-diol counterpart 12d, acted as a good FXR antagonist (IC50 = 3.5 μM) but did not show any obvious cellular toxicity. Interestingly, an electron-donating group of 2′-methoxy-substituted compound 10f with 3,19-acetonylidene exhibited similar FXR antagonistic activity (IC50 = 2.4 μM, Table 1, entry 11) to 10d and 10e containing 2′-electron-withdrawing substitutions. Moreover, 2′-methoxy-3,19-diol 12f was very active (IC50 = 0.98 μM, Table 1, entry 12) and its relatively low cellular toxicity was detected. Summarized from the above-mentioned results, it is concluded that the mono substitution at 2′-position is very flexible to the electron-donating group and the electron-withdrawing group for the FXR antagonistic activity, especially for 10 series compounds with the 3,19-acetonylidene group.
Inspired by these promising results of 12b and 12f bearing 4′-nitro and 2′-methoxy groups at 14-phenoxy, respectively, 2′-methoxy-4′-nitro-andrographolide derivatives 10g and 12g were logically designed (Fig. 4) and tested for their FXR antagonistic activity (Table 1 and Fig. 4). We envisioned that the “integration” of 2′-methoxy and 4′-nitro groups should retain or increase the antagonistic activity. Compared to its mother analogues 10b (4′-nitro) and 10f (2′-methoxy) with single substitution at 14-phenoxy, the dual substituted 2′-OMe-4′-NO2 derivative 10g with 3,19-acetonylidene (IC50 = 2.0 μM, Fig. 4 and Table 1, entry 13) was a more potent FXR antagonist than 10b (IC50 = 8.6 μM) and 10f (IC50 = 2.4 μM) as expected. However, it was surprising that 3,19-diol 12g (IC50 = 11.9 μM) with dual substitutions at 14-phenoxy by 2′-methoxy group and 4′-nitro group (Fig. 4 and Table 1, entry 14) showed a much weaker FXR antagonistic activity than 10g and its corresponding mother 3,19-diol analogues 12b and 12f, suggesting that the “integration” of 2′-methoxy and 4′-nitro groups affects the binding ability of these small molecules to FXR. More importantly, these data reveal that there are synergistic effects between substitutions at 14-phenoxy and 3,19-modifications on the antagonistic activities to the receptor FXR.
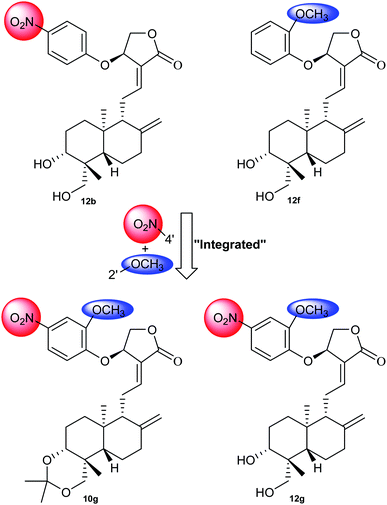 |
| Fig. 4 Design of 10g and 12g from 12b and 12f. | |
As part of exploring the effects of 14-phenoxy substitutions on the FXR antagonistic activity, substituents of the methyl group as a weak electron-donating group and fluoro group as an electron-withdrawing group were introduced at the 2′-position into the 14-(4′-nitro)-phenoxy group, respectively. The assay data disclosed that 2′-methyl-4′-nitro derivative 10h was a moderate FXR antagonist (IC50 = 6.3 μM, Table 1, entry 15) and its diol derivative 12h lost its FXR antagonistic activity (Table 1, entry 16); meanwhile, introduction of 2′-fluoro at 14-(4′-nitro)-phenoxy made 10i bearing 3,19-acetonylidene (IC50 = 3.9 μM, Table 1, entry 17) slightly more active than its 2′-methyl counterpart 10h but its diol analogues 12i (Table 1, entry 18) had a lack of FXR antagonistic activity as the 2′-methyl counterpart diol 12h.
Taking into consideration that 1 is a 14α-isomer, we subsequently investigated 14α-(4′-nitro-phenoxy) and 14α-(2′-methoxy-4′-nitro-phenoxy) andrographolide analogues (Scheme 2 and Table 1, entries 19–22) since their corresponding 14β-isomers 12b and 12g are very potent FXR antagonists. Contrary to their 14β-isomers of 10b and 12b, two 14α-(4′-nitro)-phenoxy analogues of 11b (IC50 = 15.1 μM, Table 1, entry 19) and 13b (IC50 = 16.0 μM, Table 1, entry 20) were unfortunately weaker FXR antagonists than 1 (IC50 = 9.7 μM). 11g (IC50 = 2.5 μM, Table 1, entry 21) of 3,19-acetonylidene-14α-(2′-methoxy-4′-nitro)-phenoxy derivative showed a similar FXR antagonistic activity to its 14β-counterpart 10g (IC50 = 2.0 μM) but 11g exhibited a cellular toxicity (CC50 = 12.0 μM); meanwhile, its corresponding diol 13g (IC50 = 7.1 μM, Table 1, entry 22) was a moderate FXR antagonist. Thus, these data together with their decreased stability suggest that at the current stage, 14α-isomer analogues should not be considered further as FXR antagonists.
To decipher the structural basis for the above SARs, we compared the putative binding positions of compounds 1, 5, 6, 10b, 12b, 10g and 12g by means of molecular docking. The docking model was generated from the chain A of 3DCT42 from the same view using Discovery Studio 3.5 and the modelling results are shown in Fig. 5 and 6.
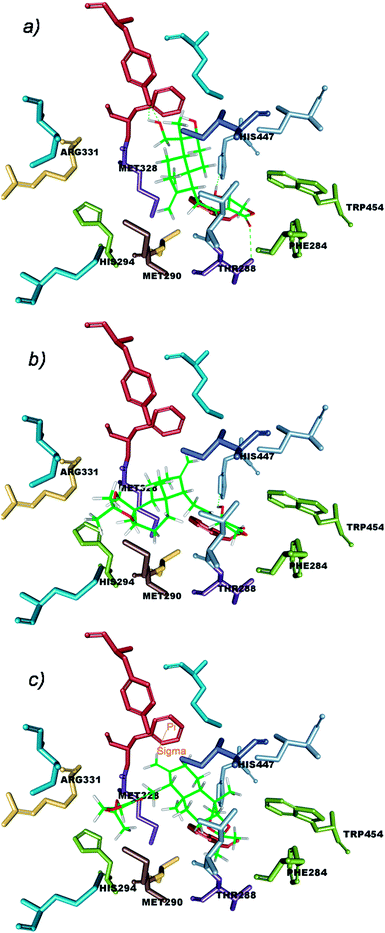 |
| Fig. 5 Three-dimensional (3D) interaction diagrams of predicted binding positions of 1 (a), 5 (b) and 6 (c) in FXR active sites. Critical amino acid residues of the binding pocket are labelled. The green dash line denotes hydrogen bond between ligand and amino acid residue atoms of FXR protein. | |
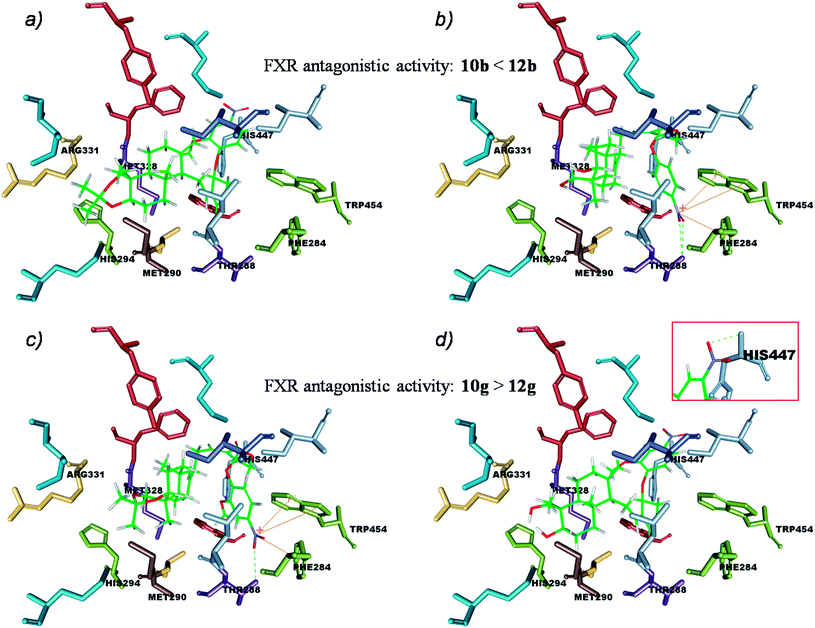 |
| Fig. 6 Three-dimensional (3D) interaction diagrams of predicted binding positions of 10b (a), 12b (b), 10g (c) and 12g (d) in FXR active site. Critical amino acid residues of the binding pocket are labelled. The green dash line denotes hydrogen bonding between ligand and amino acid residue atoms of FXR protein, while the orange line denotes π–π interaction from the ligand to amino acid aromatic ring residue of the FXR protein. The inset (in red rectangle) of the hydrogen bond in 12g (d) was viewed from a different visual angle. | |
As shown in Fig. 5, even FXR antagonistic activities of 1, 5 and 6 are similar, the putative binding positions of 1 (Fig. 5a), 5 (Fig. 5b) and 6 (Fig. 5c) in NS3 appear to be quite different, in which their orientation of decalin rings are distinctive, the bindings of lactone rings in 1 and 5 are similar but different from that in 6. The putative binding position of 1 can be transformed into 5's by making a rotation of 180° along the bond of C9–C11 or C11–C12 or 6's by a rotation of 90° clockwise and then 180° along the bond of C9–C11 or C11–C12. These simulation results suggest that the spatial volume of the binding pocket in NS3 is large enough for different occupation modes which are dependent on the interaction between a specific ligand with the binding site. This means that optimal structural tuning will benefit binding potency.
Further, the predictive binding of the most potent compound of 3,19-diol 12b is in good agreement with the large spatial volume binding pocket (Fig. 6b), in which there are two hydrogen bonds between the 4′-nitro group and the hydroxyl group of the Thr 288 residue and three π–π interactions of the 4′-nitro group with aromatic rings of Trp454 and Phe284, leading to the tight binding between 12b and FXR (Fig. 6b). However, due to the relatively large and rigid 6-membered ring formed by 3,19-acetonylidene protection as indicated in Fig. 6a, compound 10b, whose binding position is close to 5 but not 1 or 6, was obstructed by the putative “gate” established by 4 amino acid residues of Met290, His294, Met328 and Arg331, which lets compound 10b not fully enter the binding pocket, resulting in the loss of hydrogen bond and π–π interaction in the binding of 10b to FXR. These molecular binding positions of 10b and 12b are in good agreement with their FXR antagonistic activities (10b < 12b). Moreover, by comparison with the predictive binding positions of 10b and 12b, the orientations of 14β-(4′-nitro)-phenoxy moiety and the lactone ring of 10b are reciprocally exchanged, suggesting the importance of their orientations for binding.
Since compounds 10g and 12g expressed big differences in their FXR antagonistic activities (10g > 12g) and their SAR is totally distinct from that of 10b and 12b (10b < 12b), we were interested in understanding how 2′-methoxy group affects the binding positions of 10g and 12g. Interestingly and notably, the predictive binding position of 10g (Fig. 6c) bearing 3,19-acetonylidene protective group is different from that of its corresponding counterpart 10b (Fig. 6a) but is quite similar to that of the diol 12b (Fig. 6b); meanwhile, the predictive binding position of the diol 12g (Fig. 6d) is not similar to that of the diol 12b (Fig. 6b) but is almost identical to that of 3,19-acetonylidene protected compound 10b (Fig. 6a), these observations gave a good explanation for the above-mentioned FXR antagonistic relationship of 10b < 12b vs. 10g > 12g (Fig. 6 and Table 1, entries 1, 2, 13 and 14). Except for one more hydrogen bond in the binding pose of 12b as shown in Fig. 6b, the interaction of 10g with FXR (Fig. 6c), which is stabilized by one hydrogen bond and three π–π interactions, is similar to 12b. Because 3,19-acetonylidene protected molecule 10g was partially blocked by the “gate” (Fig. 6c), the binding pose of 10g is much more flat than 12b in order to reach the same binding site as 12b. These results demonstrated that the 2′-methoxy group plays an important role in the binding of these small molecules to FXR.
These modelling results are interesting in that the putative binding positions of 10b (14β) and 12g (14β), and the putative orientations of decalin rings of 12b (14β) and 10g (14β) are similar to those of 5 (14α) but not of 6 (14β), respectively.
Conclusions
In summary, starting from the readily available natural compound of andrographolide (1), a series of andrographolide derivatives by the introduction of various substituted phenoxy groups to andrographolide at 14-position were straightforwardly synthesized with inversion of the 14-configuration by the Mitsunobu reaction. Our primary SAR studies revealed that 14-phenoxy andrographolide scaffold is an excellent structural moiety for FXR antagonists. Structural tuning of the substitutions at 14-phenoxy, modifications at 3,19-positions and 14-configurations could be an efficient way to discover 14-phenoxy andrographolide derivatives as effective FXR antagonists with low cellular toxicity. Remarkably, 14β-compounds of 12b, 12f and 10g were found to be the most potent FXR antagonists in this work. Lesser stability and relatively weaker potency with somewhat cellular toxicity of α-isomers restricted their application in our current data. The structural docking unveiled that the structural features of a small FXR ligand affects its binding position, resulting in its FXR antagonistic discrepancy. It should be mentioned that these compounds have the potential to be optimized and developed into drug-like candidates in the future even compounds 6, 10b–h, 11b, 11g and 12h have too high C Log P values (>5.0) to obey the Lipinski rule of 5. Taken together, the data strongly supports that andrographolide backbone combined with phenoxy substitution at the 14-position provides an excellent scaffold for FXR antagonists.
Experimental
Materials and equipment
Unless otherwise noted, materials were obtained from commercial suppliers and used without further purification. Melting points were measured using an YRT-3 melting point apparatus (Shanghai, China) and were uncorrected. 1H and 13C NMR spectra were recorded on a Bruker AV-400 spectrometer at 400 and 100 MHz, respectively, in CDCl3, CD3OD, DMSO-d6, etc. as indicated. Coupling constants (J) are expressed in Hertz (Hz). Chemical shifts (δ) of NMR are reported in parts per million (ppm) units relative to the solvent. The low or high resolution of ESIMS (in the positive ion or negative ion acquisition mode) was recorded on an Agilent 1200 HPLC-MSD mass spectrometer or Applied Biosystems Q-STAR Elite ESI-LC-MS/MS mass spectrometer, respectively.
Preparation of 3,19-acetonylidene andrographolide (5)36–38
To the solution of 10 g (28.5 mmol) of andrographolide (1) and 24 ml (196 mmol) of 2,2-dimethoxypropane in 20.0 ml of anhydrous dichloromethane, 0.72 g (2.9 mmol) of PPTS was added and the reaction mixture was heated at 40 °C. The reaction was monitored by TLC and then treated with ethyl acetate and sat. NaHCO3 after the reaction was complete. The organic phase was washed with brine, dried over anhydrous Na2SO4, and then filtered organic solution was evaporated to dryness. The residue was purified by silica gel column chromatography (petroleum ether/ethyl acetate 1/1) to afford a white solid 10.05 g (90.2%); mp 187–192 °C. 1H NMR (400 MHz, DMSO-d6) δ 6.63 (t, J = 6.4 Hz, 1H), 5.74 (d, J = 6.0 Hz, 1H), 4.93 (t, J = 5.8 Hz, 1H), 4.86 (s, 1H), 4.69 (s, 1H), 4.41 (dd, J = 10.0, 6.0 Hz, 1H), 4.04 (dd, J = 9.8, 1.8 Hz, 1H), 3.89 (d, J = 11.6 Hz, 1H), 3.42 (dd, J = 9.2, 3.6 Hz, 1H), 3.12 (d, J = 11.6 Hz, 1H), 2.54–2.50 (m, 1H), 2.40–1.88 (m, 3H), 1.78–1.63 (m. 3H), 1.34 (s, 3H), 1.26 (s, 3H), 1.34–1.15 (m, 3H), 1.14 (s, 3H), 0.88 (s, 3H).
Preparation of 3,19-acetonylidene-14β-acetoxy-andrographolide (6)
Under N2 atmosphere, 1.0 mmol of compound 5, 1.5 mmol of PPh3, 1.5 mmol of acetic acid were dissolved in 10.0 ml of anhydrous THF. The solution was cooled to 0 °C and then treated with 1.5 mmol of DIAD in 2.0 ml of anhydrous THF. The reaction was stirred overnight at room temperature after being stirred at 0 °C for 1 h. After distilling off the volatile solvents, the residue was dissolved in ethyl acetate and washed with brine about 5 times and dried over anhydrous Na2SO4. The filtered organic solution was evaporated to dryness and the residue was purified by silica gel column chromatography (petroleum ether–ethyl acetate 1
:
1) to give 6 (61%) as a white solid; mp 140–142 °C. 1H NMR (400 MHz, C6D6) δ 7.00 (dd, J = 7.1, 1.8 Hz, 1H), 5.93 (dd, J = 5.6, 2.5 Hz, 1H), 4.89–4.84 (m, 1H), 4.56 (dd, J = 11.3, 6.2 Hz, 1H), 4.41 (d, J = 1.7 Hz, 1H), 4.22 (dd, J = 11.3, 1.9 Hz, 1H), 3.94 (d, J = 11.6 Hz, 1H), 3.49 (dd, J = 8.4, 3.9 Hz, 1H), 3.16 (d, J = 11.6 Hz, 1H), 2.55–2.31 (m, 3H), 2.10 (s, 3H), 1.98 (dd, J = 12.2, 6.4 Hz, 2H), 1.91–1.83 (m, 1H), 1.83–1.64 (m, 3H), 1.39 (s, 3H), 1.35 (s, 3H), 1.28 (tdd, J = 12.5, 8.2, 4.6 Hz, 3H), 1.18 (s, 3H), 0.94 (s, 3H); 13C NMR (101 MHz, C6D6) δ 168.7, 161.3, 152.0, 146.9, 142.5, 126.4, 123.9, 115.3, 108.3, 80.3, 77.3, 71.8, 70.3, 64.0, 55.7, 55.2, 42.8, 39.0, 37.7, 36.9, 28.1, 25.8, 23.7, 22.7, 15.1; ESI-HRMS: m/z 455.2397 [M + Na]+, calcd for C25H36NaO6, 455.2410.
Preparation of 14β-acetoxy-andrographolide (7)
0.5 mmol of compound 6 was dissolved in 4 ml of methanol and then treated with 0.05 mmol of TsOH at 20 °C for 30 min. Diluted by ethyl acetate and washed with sat. NaHCO3, brine, the organic phase was dried over anhydrous Na2SO4, filtered, evaporated by a rotavap to dryness. Compound 7 was purified by silica gel column chromatography (petroleum ether–ethyl acetate 10
:
7) (white solid, 93%, mp 163–165 °C). 1H NMR (400 MHz, CDCl3) δ 6.98 (dd, J = 7.0, 1.8 Hz, 1H), 5.91 (dt, J = 6.0, 1.8 Hz, 1H), 4.85 (q, J = 1.3 Hz, 1H), 4.54 (dd, J = 11.3, 6.1 Hz, 1H), 4.36 (d, J = 1.7 Hz, 1H), 4.20 (ddd, J = 25.9, 11.0, 1.8 Hz, 2H), 3.46 (dt, J = 10.2, 4.6 Hz, 1H), 3.36–3.26 (m, 1H), 3.00–2.89 (m, 2H), 2.53–2.26 (m, 3H), 2.11 (s, 3H), 1.96 (td, J = 12.5, 5.0 Hz, 1H), 1.89–1.75 (m, 4H), 1.75–1.68 (m, 1H), 1.36–1.12 (m, 6H), 0.66 (s, 3H); 13C NMR (101 MHz, CDCl3) δ 170.5, 169.1, 150.6, 146.8, 123.9, 108.4, 80.4, 71.7, 68.1, 64.1, 55.7, 55.2, 42.9, 39.0, 37.7, 36.9, 28.2, 28.2, 25.6, 23.7, 22.7, 20.8, 15.2; ESI-HRMS: m/z 415.2067 [M + Na]+, calcd for C22H32NaO6, 415.2097.
Preparation of 14β-andrographolide (8)
0.5 mmol of compound 6 was dissolved in 4 ml of methanol and then treated with 0.05 mmol of TsOH at 40–50 °C for 4 h. Diluted by ethyl acetate and washed with sat. NaHCO3, brine, the organic phase was dried over anhydrous Na2SO4, filtered, evaporated by a rotavap to dryness. Compound 8 was purified by silica gel column chromatography (dichloromethane–ethyl acetate 1
:
2) (white solid, 80%, mp 200–202 °C). 1H NMR (400 MHz, DMSO-d6) δ 6.61 (ddd, J = 7.9, 6.1, 1.8 Hz, 1H), 5.64 (d, J = 6.1 Hz, 1H), 5.06 (d, J = 4.8 Hz, 1H), 4.94 (t, J = 6.1 Hz, 1H), 4.79 (d, J = 1.8 Hz, 1H), 4.45–4.36 (m, 2H), 4.12 (dd, J = 7.6, 2.8 Hz, 1H), 4.02 (dd, J = 9.9, 2.2 Hz, 1H), 3.84 (dd, J = 10.9, 2.9 Hz, 1H), 3.24 (ddd, J = 14.8, 9.9, 6.1 Hz, 2H), 2.63–2.53 (m, 1H), 2.43–2.26 (m, 2H), 1.92 (t, J = 12.2 Hz, 2H), 1.79–1.59 (m, 4H), 1.43–1.15 (m, 3H), 1.08 (s, 3H), 0.66 (s, 3H); 13C NMR (101 MHz, C6D6) δ 175.2, 153.3, 151.8, 134.5, 113.0, 83.8, 79.6, 70.2, 68.0, 60.7, 59.8, 47.6, 44.1, 42.9, 41.8, 33.2, 29.8, 29.3, 28.4, 20.2; ESI-HRMS: m/z 373.1981 [M + Na]+, calcd for C20H30NaO5, 373.1991.
Preparation of 3,19-acetonylidene-14β-andrographolide (9)
The same procedure was used as the preparation of compound 5. The compound 9 was purified by silica gel column with petroleum ether/ethyl acetate 10/7 as a white solid (90%), mp 167–168 °C. 1H NMR (400 MHz, CDCl3) δ 6.95 (ddd, J = 8.0, 6.5, 1.8 Hz, 1H), 5.08 (t, J = 6.5 Hz, 1H), 4.87 (t, J = 1.5 Hz, 1H), 4.47 (dd, J = 10.5, 6.1 Hz, 1H), 4.43 (q, J = 1.3 Hz, 1H), 4.25 (dd, J = 10.5, 2.1 Hz, 1H), 3.96 (d, J = 11.6 Hz, 1H), 3.50 (dd, J = 8.6, 3.6 Hz, 1H), 3.18 (d, J = 11.6 Hz, 1H), 2.72–2.61 (m, 1H), 2.54–2.44 (m, 1H), 2.41 (dt, J = 13.3, 2.9 Hz, 1H), 2.07 (d, J = 6.8 Hz, 1H), 2.05–1.89 (m, 3H), 1.85–1.70 (m, 3H), 1.41 (s, 3H), 1.37 (s, 3H), 1.36–1.24 (m, 3H), 1.21 (s, 3H), 0.96 (s, 3H); 13C NMR (101 MHz, DMSO) δ 169.8, 147.8, 146.3, 129.2, 108.1, 98.2, 75.8, 74.2, 64.8, 62.8, 55.2, 51.5, 38.2, 37.2, 37.1, 33.8, 27.4, 25.8, 25.2, 24.8, 24.6, 22.7, 15.8; ESI-HRMS: m/z 413.2302 [M + Na]+, calcd for C23H34NaO5, 413.2304.
Preparation of the series compounds 10a–10i, 11b and 11g
The same procedure was used as the preparation of 3,19-acetonylidene 14β-acetoxy andrographolide (6). Generally, the purification was conducted by silica gel column chromatography with petroleum ether–ethyl acetate from 3
:
1 to 1
:
1.
3,19-Acetonylidene-14β-phenoxy-andrographolide (10a)
From 5 in 51% yield, white solid, mp 153–155 °C. 1H NMR (400 MHz, CDCl3) δ (ppm) 7.39–7.28 (m, 2H), 7.13 (td, J = 7.4, 1.8 Hz, 1H), 7.05 (tt, J = 7.5, 1.0 Hz, 1H), 6.88–6.80 (m, 2H), 5.53 (d, J = 5.7 Hz, 1H), 4.87 (d, J = 1.6 Hz, 1H), 4.60 (dd, J = 10.7, 5.8 Hz, 1H), 4.46 (s, 1H), 4.40 (dd, J = 10.7, 2.0 Hz, 1H), 3.91 (d, J = 11.6 Hz, 1H), 3.42 (dd, J = 8.3, 3.9 Hz, 1H), 3.14 (d, J = 11.5 Hz, 1H), 2.57–2.46 (m, 1H), 2.45–2.26 (m, 2H), 1.95 (dd, J = 27.6, 12.1 Hz, 2H), 1.88–1.77 (m, 1H), 1.75–1.67 (m, 1H), 1.64 (dd, J = 11.8, 6.0 Hz, 1H), 1.51 (dd, J = 8.0, 5.4 Hz, 1H), 1.37 (s, 3H), 1.34 (s, 3H), 1.31–1.19 (m, 3H), 1.17 (s, 3H), 0.87 (s, 3H); 13C NMR (101 MHz, C6D6) δ 168.8, 157.0, 149.6, 148.1, 130.1, 126.0, 122.2, 115.9, 108.0, 99.4, 75.4, 71.5, 70.5, 64.2, 55.8, 51.3, 38.5, 38.3, 37.8, 33.7, 26.7, 26.1, 25.7, 25.3, 24.8, 23.3, 16.6; ESI-HRMS: m/z 489.2658 [M + Na]+, calcd for C29H38NaO5, 489.2617.
3,19-Acetonylidene-14β-(4′-nitro-phenoxy)-andrographolide (10b)
From 5 in 52% yield, white solid, mp 179–181 °C. 1H NMR (400 MHz, CDCl3) δ 8.31–8.22 (m, 2H), 7.19 (ddd, J = 8.0, 6.7, 1.7 Hz, 1H), 6.97–6.88 (m, 2H), 5.69–5.62 (m, 1H), 4.89 (dd, J = 1.8, 1.0 Hz, 1H), 4.67 (dd, J = 10.9, 5.8 Hz, 1H), 4.45 (d, J = 1.5 Hz, 1H), 4.37 (dd, J = 10.9, 1.9 Hz, 1H), 3.88 (d, J = 11.6 Hz, 1H), 3.44 (dd, J = 7.8, 3.8 Hz, 1H), 3.14 (d, J = 11.5 Hz, 1H), 2.54 (ddd, J = 15.9, 7.8, 2.5 Hz, 1H), 2.47–2.31 (m, 2H), 2.04–1.89 (m, 2H), 1.85–1.75 (m, 1H), 1.75–1.68 (m, 1H), 1.68–1.59 (m, 1H), 1.51 (td, J = 8.0, 4.2 Hz, 1H), 1.35 (s, 3H), 1.33 (s, 3H), 1.31–1.21 (m, 3H), 1.16 (s, 3H), 0.89 (s, 3H); 13C NMR (101 MHz, CDCl3) δ 168.68, 161.28, 151.98, 147.27, 142.53, 126.35, 123.93, 115.16, 108.26, 99.37, 75.42, 71.78, 70.26, 64.05, 55.82, 51.66, 38.50, 38.10, 37.58, 33.85, 26.47, 26.01, 25.94, 25.10, 24.43, 23.17, 16.44; ESI-HRMS: m/z 534.2473 [M + Na]+, calcd for C29H37NNaO7, 534.2468.
3,19-Acetonylidene-14β-(3′-nitro-phenoxy)-andrographolide (10c)
From 5 in 32% yield, white solid, mp 163–165 °C. 1H NMR (400 MHz, C6D6) δ 7.51 (d, J = 7.1 Hz, 1H), 7.31 (d, J = 2.1 Hz, 1H), 7.23–7.16 (m, 1H), 6.62 (t, J = 8.2 Hz, 1H), 6.52 (dd, J = 8.3, 1.8 Hz, 1H), 4.85 (s, 1H), 4.62 (d, J = 5.1 Hz, 1H), 4.43 (s, 1H), 3.77 (d, J = 11.6 Hz, 1H), 3.67–3.54 (m, 2H), 3.41 (dd, J = 7.1, 3.5 Hz, 1H), 3.06 (d, J = 11.5 Hz, 1H), 2.12 (ddd, J = 22.8, 17.7, 9.7 Hz, 3H), 1.68 (dt, J = 13.0, 6.6 Hz, 2H), 1.53–1.36 (m, 2H), 1.39 (s, 3H), 1.35–1.25 (m, 2H), 1.34 (s, 3H), 1.07 (s, 3H), 0.99 (td, J = 13.0, 3.8 Hz, 1H), 0.94–0.80 (m, 2H), 0.84 (s, 3H); 13C NMR (101 MHz, C6D6) δ 168.1, 157.1, 150.2, 149.5, 147.8, 130.4, 124.9, 122.2, 116.8, 109.2, 108.0, 99.5, 74.9, 71.8, 69.8, 64.2, 55.6, 51.0, 38.4, 38.2, 37.6, 33.5, 26.2, 25.9, 25.8, 25.1, 24.4, 23.1, 16.6; ESI-HRMS: m/z 534.2463 [M + Na]+, calcd for C29H37NNaO7, 534.2468.
3,19-Acetonylidene-14β-(2′-nitro-phenoxy)-andrographolide (10d)
From 5 in 73% yield, white solid, mp 146–148 °C. 1H NMR (400 MHz, C6D6) δ 7.27 (dd, J = 8.0, 1.7 Hz, 1H), 7.21 (ddd, J = 8.1, 6.3, 1.9 Hz, 1H), 6.77–6.65 (m, 1H), 6.34 (td, J = 7.8, 1.1 Hz, 1H), 6.06–5.92 (m, 1H), 4.93–4.80 (m, 2H), 4.38 (t, J = 2.8 Hz, 1H), 3.82 (d, J = 11.5 Hz, 1H), 3.60 (qd, J = 10.8, 4.2 Hz, 2H), 3.45 (dd, J = 7.7, 3.3 Hz, 1H), 3.08 (d, J = 11.5 Hz, 1H), 2.40–2.29 (m, 1H), 2.26–2.11 (m, 2H), 1.86–1.67 (m, 2H), 1.62–1.47 (m, 3H), 1.42 (s, 3H), 1.36 (s, 3H), 1.36–1.31 (m, 1H), 1.12 (s, 3H), 1.05–0.91 (m, 3H), 0.87 (s, 3H); 13C NMR (101 MHz, C6D6) δ 168.0, 151.6, 149.3, 148.4, 141.6, 133.2, 127.7, 125.8, 124.3, 121.7, 115.8, 107.8, 99.3, 75.4, 73.3, 69.6, 64.1, 55.7, 51.0, 38.4, 38.1, 37.6, 33.6, 26.7, 25.9, 25.2, 24.7, 23.2, 16.5; ESI-HRMS: m/z 534.2467 [M + Na]+, calcd for C29H37NNaO7, 534.2468.
Ethyl 3,19-acetonylidene-14β-(2′-carboxy-phenoxy)-andrographolide (10e)
From 5 in 78% yield, white solid, mp 168–170 °C. 1H NMR (400 MHz, C6D6) δ 7.80 (dd, J = 7.7, 1.8 Hz, 1H), 7.24–7.18 (m, 1H), 6.98–6.92 (m, 1H), 6.69 (td, J = 7.6, 0.9 Hz, 1H), 6.28 (d, J = 8.2 Hz, 1H), 5.04 (d, J = 5.4 Hz, 1H), 4.87–4.81 (m, 1H), 4.44 (s, 1H), 4.12 (qq, J = 10.9, 7.1 Hz, 2H), 4.01 (dd, J = 10.6, 1.4 Hz, 1H), 3.82 (d, J = 11.5 Hz, 1H), 3.68 (dd, J = 10.6, 5.5 Hz, 1H), 3.42 (dd, J = 7.6, 3.7 Hz, 1H), 3.08 (d, J = 11.5 Hz, 1H), 2.24–2.05 (m, 3H), 1.73 (dq, J = 13.4, 6.8 Hz, 2H), 1.59 (d, J = 8.3 Hz, 1H), 1.53–1.43 (m, 1H), 1.43–1.31 (m, 2H), 1.41 (s, 3H), 1.37 (s, 3H), 1.11 (s, 3H), 1.04 (t, J = 7.1 Hz, 3H), 1.01–0.89 (m, 3H), 0.85 (s, 3H); 13C NMR (101 MHz, C6D6) δ 168.7, 165.7, 156.0, 149.7, 148.2, 132.9, 132.2, 127.8, 125.8, 123.9, 122.2, 116.6, 107.9, 99.3, 75.3, 73.2, 70.3, 64.1, 61.0, 55.8, 51.0, 38.4, 38.2, 37.6, 33.5, 26.6, 25.91, 25.87, 25.2, 24.6, 23.2, 16.5, 14.1; ESI-HRMS: m/z 561.2829 [M + Na]+, calcd for C32H42NaO7, 561.2828.
3,19-Acetonylidene-14β-(2′-methoxy-phenoxy)-andrographolide (10f)
From 5 in 60% yield, white solid, mp 130–131 °C. 1H NMR (400 MHz, C6D6) δ 7.13–7.10 (m, 1H), 6.80 (td, J = 7.8, 1.7 Hz, 1H), 6.65 (td, J = 7.7, 1.5 Hz, 1H), 6.58 (dd, J = 7.9, 1.6 Hz, 1H), 6.47 (dd, J = 8.1, 1.3 Hz, 1H), 5.18 (d, J = 5.4 Hz, 1H), 4.82 (d, J = 1.2 Hz, 1H), 4.42 (s, 1H), 4.15 (dd, J = 10.6, 1.5 Hz, 1H), 3.83 (d, J = 11.5 Hz, 1H), 3.65 (dd, J = 10.6, 5.5 Hz, 1H), 3.41 (dd, J = 7.7, 3.7 Hz, 1H), 3.29 (s, 3H), 3.09 (d, J = 11.5 Hz, 1H), 2.26–2.04 (m, 3H), 1.83–1.62 (m, 2H), 1.54–1.28 (m, 10H), 1.08 (s, 3H), 1.05–0.82 (m, 6H); 13C NMR (101 MHz, C6D6) δ 169.08, 151.78, 149.42, 148.27, 145.92, 128.15, 128.03, 127.91, 127.79, 127.67, 126.39, 124.00, 121.11, 120.16, 112.54, 107.85, 99.30, 75.39, 73.44, 70.56, 64.14, 55.72, 55.11, 51.10, 38.34, 38.12, 37.66, 33.56, 26.57, 26.00, 25.64, 25.29, 24.74, 23.24, 16.46; ESI-HRMS: m/z 519.2715 [M + Na]+, calcd for C30H40NaO6, 519.2723.
3,19-Acetonylidene-14β-(2′-methoxy-4′-nitro-phenoxy)-andrographolide (10g)
From 5 in 51% yield, pale yellowish solid, mp 129–131 °C. 1H NMR (400 MHz, C6D6) δ 7.56 (dd, J = 8.8, 2.6 Hz, 1H), 7.46 (d, J = 2.6 Hz, 1H), 7.20–7.15 (m, 1H), 5.95 (d, J = 8.8 Hz, 1H), 4.88 (d, J = 5.5 Hz, 1H), 4.83 (d, J = 1.5 Hz, 1H), 4.41–4.37 (m, 1H), 3.82–3.74 (m, 2H), 3.60 (dd, J = 10.8, 5.7 Hz, 1H), 3.41 (dd, J = 7.3, 3.6 Hz, 1H), 3.08 (s, 1H), 3.05 (s, 3H), 2.19–2.10 (m, 3H), 1.77–1.60 (m, 2H), 1.52–1.44 (m, 1H), 1.44–1.36 (m, 1H), 1.40 (s, 3H), 1.36 (s, 3H), 1.34–1.25 (m, 2H), 1.06 (s, 3H), 1.03–0.93 (m, 1H), 0.94–0.80 (m, 3H), 0.85 (s, 3H); 13C NMR (101 MHz, C6D6) δ 168.1, 150.8, 150.5, 150.3, 148.1, 143.2, 124.9, 116.9, 115.0, 107.7, 107.1, 99.4, 74.8, 72.9, 69.7, 64.0, 55.4, 55.1, 50.7, 38.2, 38.1, 37.4, 33.2, 26.1, 25.8, 25.6, 25.0, 24.3, 23.0, 16.4; ESI-HRMS: m/z 564.2584 [M + Na]+, calcd for C30H39NNaO8, 564.2573.
3,19-Acetonylidene-14β-(2′-methyl-4′-nitro-phenoxy)-andrographolide (10h)
From 5 in 61% yield, white solid, mp 168–170 °C. 1H NMR (400 MHz, C6D6) δ 8.13 (d, J = 7.7 Hz, 2H), 7.19 (td, J = 7.2, 1.7 Hz, 1H), 6.79–6.70 (m, 1H), 5.67 (d, J = 5.6 Hz, 1H), 4.91–4.84 (m, 1H), 4.67 (dd, J = 10.9, 5.7 Hz, 1H), 4.43 (d, J = 1.8 Hz, 1H), 4.35 (dd, J = 11.0, 1.7 Hz, 1H), 3.87 (d, J = 11.6 Hz, 1H), 3.43 (dd, J = 7.7, 3.7 Hz, 1H), 3.13 (d, J = 11.6 Hz, 1H), 2.51 (ddd, J = 16.2, 7.4, 2.6 Hz, 1H), 2.45–2.34 (m, 2H), 2.27 (s, 3H), 1.96 (ddd, J = 27.0, 12.4, 6.9 Hz, 2H), 1.83–1.66 (m, 2H), 1.65–1.44 (m, 3H), 1.34 (s, 3H), 1.33 (s, 3H), 1.31–1.17 (m, 3H), 1.15 (s, 3H), 0.89 (s, 3H); 13C NMR (101 MHz, C6D6) δ 162.6, 153.7, 145.2, 142.7, 136.9, 123.1, 121.4, 119.4, 117.9, 105.4, 102.5, 94.2, 69.1, 66.5, 64.3, 58.8, 50.5, 45.4, 32.9, 32.2, 27.9, 20.5, 19.6, 18.7, 17.7, 11.4, 10.5; ESI-HRMS: m/z 548.2636 [M + Na]+, calcd for C30H39NNaO7, 548.2624.
3,19-Acetonylidene-14β-(2′-fluoro-4′-nitro-phenoxy)-andrographolide (10i)
From 5 in 72% yield, white solid, mp 114–117 °C. 1H NMR (400 MHz, C6D6) δ 7.56 (dd, J = 10.6, 2.6 Hz, 1H), 7.47 (ddd, J = 9.1, 2.7, 1.5 Hz, 1H), 7.23–7.18 (m, 1H), 5.75 (td, J = 8.4, 3.3 Hz, 1H), 4.84 (d, J = 1.8 Hz, 1H), 4.70 (d, J = 5.1 Hz, 1H), 4.39 (d, J = 1.7 Hz, 1H), 3.77 (d, J = 11.6 Hz, 1H), 3.63–3.51 (m, 2H), 3.43 (dd, J = 7.0, 3.5 Hz, 1H), 3.05 (d, J = 11.5 Hz, 1H), 2.26–2.08 (m, 3H), 1.79–1.60 (m, 2H), 1.55–1.43 (m, 2H), 1.39 (s, 3H), 1.34 (s, 3H), 1.36–1.27 (m, 2H), 1.05 (s, 3H), 1.01–0.85 (m, 3H), 0.89 (s, 3H); 13C NMR (101 MHz, C6D6) δ 167.9, 167.7, 153.1, 151.5, 151.4, 150.6, 149.7, 149.6, 148.17, 142.3, 142.3, 124.2, 120.6, 115.0, 113.0, 112.8, 107.8, 99.5, 74.7, 73.4, 69.5, 64.2, 55.5, 50.7, 38.3, 38.3, 37.5, 33.2, 26.0, 25.8 (2C), 25.0, 24.2, 23.1, 16.7; ESI-HRMS: m/z 552.2418 [M + Na]+, calcd for C29H36FNNaO7, 552.2374.
3,19-Acetonylidene-14α-(4′-nitro-phenoxy)-andrographolide (11b)
From 9 in 56% yield, white solid, mp 149–152 °C. 1H NMR (400 MHz, Benzene-d6) δ 7.80–7.74 (m, 2H), 6.39 (dd, J = 9.5, 2.4 Hz, 2H), 6.11 (dt, J = 4.7, 1.9 Hz, 1H), 5.49 (s, 1H), 5.20 (dt, J = 10.7, 1.8 Hz, 1H), 5.17–5.13 (m, 1H), 3.86 (d, J = 11.5 Hz, 1H), 3.68 (dd, J = 3.3, 2.0 Hz, 2H), 3.50 (dd, J = 7.1, 3.4 Hz, 1H), 3.09 (d, J = 11.5 Hz, 1H), 2.36–2.20 (m, 2H), 2.09–2.03 (m, 1H), 2.02–1.92 (m, 1H), 1.85 (dd, J = 14.6, 10.6 Hz, 1H), 1.81–1.63 (m, 3H), 1.51 (dd, J = 12.6, 6.7 Hz, 1H), 1.43 (s, 3H), 1.41 (s, 3H), 1.33 (ddt, J = 6.9, 5.1, 2.9 Hz, 1H), 1.12–0.97 (m, 2H), 1.03 (s, 3H), 1.02 (s, 3H); 13C NMR (101 MHz, C6D6) δ 171.5, 162.4, 147.2, 145.7, 142.2, 134.0, 125.9, 115.0, 109.1, 99.5, 75.0, 73.0, 69.9, 64.3, 52.3, 51.4, 38.4, 38.3, 38.2, 33.9, 30.9, 26.2, 25.8, 25.2, 24.6, 23.4, 17.0; ESI-HRMS: m/z 534.2500 [M + Na]+, calcd for C29H37NNaO7, 534.2468.
3,19-Acetonylidene-14α-(2′-methoxy-4′-nitro-phenoxy)-andrographolide (11g)
From 9 in 50% yield, white solid, mp 121–123 °C. 1H NMR (400 MHz, C6D6) δ 7.56 (dd, J = 8.8, 2.4 Hz, 1H), 7.45 (d, J = 2.5 Hz, 1H), 7.14–7.10 (m, 1H), 6.07–5.99 (m, 1H), 4.90 (s, 1H), 4.82 (s, 1H), 4.61 (s, 1H), 3.81 (dd, J = 13.3, 6.7 Hz, 2H), 3.71 (dd, J = 10.8, 5.9 Hz, 1H), 3.46 (dd, J = 7.5, 3.7 Hz, 1H), 3.07 (t, J = 6.6 Hz, 4H), 2.41–2.24 (m, 1H), 2.22–2.05 (m, 2H), 1.85 (td, J = 13.6, 6.4 Hz, 1H), 1.79–1.67 (m, 1H), 1.62–1.52 (m, 1H), 1.52–1.28 (m, 9H), 1.06 (d, J = 16.4 Hz, 3H), 1.04–0.89 (m, 3H), 0.84–0.74 (m, 3H); 13C NMR (101 MHz, C6D6) δ 168.17, 151.11, 150.60, 147.17, 143.51, 124.80, 116.98, 115.61, 109.33, 107.40, 99.44, 75.17, 73.37, 69.90, 64.12, 55.82, 55.21, 51.25, 38.16, 38.04, 37.64, 34.06, 26.32, 26.01, 25.32, 25.20, 24.69, 23.16, 16.39; ESI-HRMS: m/z 564.2569 [M + Na]+, calcd for C30H39NNaO8, 564.2573.
Preparation of the series compounds 12a–12i, 13b and 13g
The same procedure was used as the preparation of compound 7. Generally, the purification was conducted by silica gel column chromatography with petroleum ether–ethyl acetate from 2
:
1 to 1
:
1.
14β-Phenoxy-andrographolide (12a)
From 10a in 86% yield, white solid, mp 164–166 °C. 1H NMR (400 MHz, CDCl3) δ 7.40–7.29 (m, 2H), 7.14–7.01 (m, 2H), 6.89–6.79 (m, 2H), 5.55–5.48 (m, 1H), 4.85 (dd, J = 1.9, 1.0 Hz, 1H), 4.60 (dd, J = 10.7, 5.9 Hz, 1H), 4.44–4.36 (m, 2H), 4.17–4.08 (m, 1H), 3.40 (dd, J = 11.5, 4.3 Hz, 1H), 3.29 (d, J = 10.9 Hz, 1H), 2.54–2.44 (m, 1H), 2.44–2.36 (m, 1H), 2.33–2.23 (m, 1H), 2.16 (d, J = 26.3 Hz, 3H), 1.97 (td, J = 12.7, 10.7, 6.2 Hz, 1H), 1.92–1.85 (m, 1H), 1.85–1.70 (m, 2H), 1.70–1.55 (m, 2H), 1.33–1.16 (m, 5H), 1.11 (td, J = 13.5, 3.7 Hz, 1H), 0.58 (s, 3H); 13C NMR (101 MHz, C6D6) δ 169.5, 156.4, 150.8, 147.1, 130.0, 125.0, 122.3, 115.7, 108.2, 80.4, 71.3, 71.0, 64.1, 55.9, 55.1, 42.8, 39.0, 37.7, 36.8, 28.1, 25.6, 23.7, 22.7, 15.1; HRMS (ESI): m/z 449.2342 [M + Na]+, calcd for C26H34NaO5, 449.2304.
14β-(4′-Nitro-phenoxy)-andrographolide (12b)
From 10b in 89% yield, white solid, mp 184–186 °C. 1H NMR (400 MHz, CDCl3) δ 8.32–8.23 (m, 2H), 7.18–7.11 (m, 1H), 6.93 (d, J = 9.2 Hz, 2H), 5.63 (d, J = 5.7 Hz, 1H), 4.87 (s, 1H), 4.67 (dd, J = 10.9, 5.8 Hz, 1H), 4.43–4.32 (m, 2H), 4.13 (d, J = 11.2 Hz, 1H), 3.42 (dd, J = 11.6, 4.3 Hz, 1H), 3.29 (d, J = 11.0 Hz, 1H), 2.50 (dd, J = 7.0, 2.7 Hz, 1H), 2.46–2.37 (m, 1H), 2.32 (dd, J = 10.7, 7.1 Hz, 1H), 2.05 (s, 3H), 1.97 (dd, J = 12.7, 5.2 Hz, 1H), 1.90 (d, J = 10.5 Hz, 1H), 1.87–1.70 (m, 2H), 1.70–1.63 (m, 1H), 1.60 (dd, J = 13.0, 3.5 Hz, 1H), 1.34–1.18 (m, 5H), 1.13 (td, J = 13.2, 3.8 Hz, 1H), 0.60 (s, 3H); 13C NMR (101 MHz, CDCl3) δ 168.7, 161.3, 152.0, 146.9, 142.5, 126.4, 123.9, 115.3, 108.3, 80.3, 77.3, 71.8, 70.3, 64.0, 55.7, 55.2, 42.8, 39.0, 37.7, 36.9, 28.1, 25.8, 23.7, 22.7, 15.1; HRMS (ESI): m/z 494.2148 [M + Na]+, calcd for C26H33NNaO7, 494.2155.
14β-(3′-nitro-phenoxy)-andrographolide (12c)
From 10c in 92% yield, white solid, mp 144–146 °C. 1H NMR (400 MHz, CDCl3) δ 7.94 (ddd, J = 8.2, 2.0, 0.9 Hz, 1H), 7.69 (t, J = 2.3 Hz, 1H), 7.54 (t, J = 8.2 Hz, 1H), 7.20 (ddd, J = 8.3, 2.6, 0.9 Hz, 1H), 7.14 (td, J = 7.1, 1.7 Hz, 1H), 5.62 (dt, J = 5.6, 1.7 Hz, 1H), 4.87 (q, J = 1.3 Hz, 1H), 4.67 (dd, J = 10.9, 5.7 Hz, 1H), 4.45–4.35 (m, 2H), 4.12 (d, J = 11.2 Hz, 1H), 3.41 (ddd, J = 11.7, 4.5, 1.3 Hz, 1H), 3.29 (dd, J = 11.2, 1.3 Hz, 1H), 2.51 (ddd, J = 16.1, 7.2, 3.0 Hz, 1H), 2.46–2.38 (m, 1H), 2.37–2.23 (m, 4H), 1.98 (td, J = 12.6, 4.9 Hz, 1H), 1.93–1.87 (m, 1H), 1.87–1.79 (m, 1H), 1.79–1.69 (m, 1H), 1.62 (ddt, J = 24.2, 13.1, 3.8 Hz, 2H), 1.34–1.18 (m, 5H), 1.12 (td, J = 13.2, 4.1 Hz, 1H), 0.60 (s, 3H); 13C NMR (101 MHz, CDCl3) δ 168.9, 157.0, 151.7, 149.4, 146.9, 130.9, 124.2, 122.4, 117.3, 109.7, 108.3, 80.3, 71.9, 70.4, 64.1, 55.8, 55.2, 42.8, 39.00, 37.7, 36.9, 28.1, 25.7, 23.7, 22.7, 15.1; HRMS (ESI): m/z 494.2156 [M + Na]+, calcd for C26H33NNaO7, 494.2155.
14β-(2′-nitro-phenoxy)-andrographolide (12d)
From 10d in 86% yield, white solid, mp 167–168 °C. 1H NMR (400 MHz, CDCl3) δ 7.89 (dd, J = 8.1, 1.6 Hz, 1H), 7.62–7.55 (m, 1H), 7.18 (t, J = 7.7 Hz, 1H), 7.15–7.10 (m, 1H), 6.92 (d, J = 8.3 Hz, 1H), 5.64 (d, J = 5.6 Hz, 1H), 4.83 (s, 1H), 4.66 (dd, J = 10.9, 6.0 Hz, 1H), 4.44 (dd, J = 10.9, 2.0 Hz, 1H), 4.31 (s, 1H), 4.13 (d, J = 11.2 Hz, 1H), 3.43 (dd, J = 11.5, 4.6 Hz, 1H), 3.28 (d, J = 11.1 Hz, 1H), 2.56–2.45 (m, 1H), 2.38 (dd, J = 13.2, 3.5 Hz, 1H), 2.26 (ddd, J = 16.5, 11.2, 7.8 Hz, 1H), 2.09 (s, 3H), 1.98 (t, J = 12.3 Hz, 1H), 1.89 (d, J = 10.4 Hz, 1H), 1.85–1.78 (m, 1H), 1.78–1.63 (m, 2H), 1.59 (dt, J = 13.0, 3.3 Hz, 1H), 1.24 (s, 5H), 1.10 (td, J = 13.2, 3.9 Hz, 1H), 0.58 (s, 3H); 13C NMR (101 MHz, CDCl3) δ 168.7, 152.6, 149.4, 147.3, 141.1, 134.1, 126.3, 123.7, 122.3, 115.9, 108.1, 80.3, 73.1, 70.3, 64.1, 55.8, 54.9, 42.8, 38.9, 37.6, 36.5, 28.1, 25.8, 23.7, 22.6, 15.1; HRMS (ESI): m/z 494.2158 [M + Na]+, calcd for C26H33NNaO7, 494.2155.
Ethyl 14β-(2′-carboxy-phenoxy)-andrographolide (12e)
From 10e in 88% yield, white solid, mp 181–182 °C. 1H NMR (400 MHz, CDCl3) δ 7.88 (dd, J = 7.8, 1.7 Hz, 1H), 7.56–7.46 (m, 1H), 7.20–7.11 (m, 1H), 7.04 (ddd, J = 7.7, 6.0, 1.6 Hz, 1H), 6.91 (d, J = 8.3 Hz, 1H), 5.61 (d, J = 5.3 Hz, 1H), 4.83 (d, J = 1.9 Hz, 1H), 4.63–4.48 (m, 2H), 4.41–4.26 (m, 3H), 4.14 (d, J = 11.1 Hz, 1H), 3.41 (dt, J = 11.7, 2.8 Hz, 1H), 3.29 (d, J = 10.9 Hz, 1H), 2.47–2.25 (m, 4H), 2.17 (ddd, J = 16.4, 11.2, 8.2 Hz, 1H), 1.96 (td, J = 12.6, 5.2 Hz, 1H), 1.88–1.78 (m, 2H), 1.78–1.61 (m, 2H), 1.36 (t, J = 7.1 Hz, 3H), 1.32–1.16 (m, 5H), 1.04 (td, J = 13.3, 3.9 Hz, 1H), 0.56 (s, 3H); 13C NMR (101 MHz, CDCl3) δ 169.5, 165.7, 155.8, 150.9, 147.1, 133.4, 132.3, 124.9, 123.2, 122.6, 117.0, 108.2, 80.3, 73.4, 71.0, 64.1, 61.2, 55.8, 54.9, 42.8, 38.8, 37.6, 36.6, 28.1, 25.6, 23.7, 22.6, 15.1, 14.3; HRMS (ESI): m/z 521.2517 [M + Na]+, calcd for C29H39NaO7, 521.2515.
14β-(2′-methoxy-phenoxy)-andrographolide (12f)
From 10f in 93% yield, white solid, mp 176–178 °C. 1H NMR (400 MHz, CDCl3) δ 7.13–7.02 (m, 1H), 7.01–6.83 (m, 4H), 5.58 (t, J = 13.3 Hz, 1H), 4.82 (t, J = 7.3 Hz, 1H), 4.55–4.40 (m, 2H), 4.32 (dd, J = 7.6, 6.7 Hz, 1H), 4.13 (t, J = 8.9 Hz, 1H), 3.92–3.81 (m, 3H), 3.47–3.35 (m, 1H), 3.30 (d, J = 11.1 Hz, 1H), 2.42–2.33 (m, 1H), 2.32–2.21 (m, 2H), 2.02–1.66 (m, 5H), 1.56 (dt, J = 13.3, 3.6 Hz, 1H), 1.31–1.02 (m, 6H), 0.61 (dd, J = 18.6, 2.5 Hz, 3H); 13C NMR (101 MHz, CDCl3) δ 169.77, 151.35, 150.68, 147.23, 145.29, 125.46, 124.33, 121.09, 119.97, 112.35, 108.12, 80.45, 73.42, 71.20, 64.14, 55.80, 55.69, 55.03, 42.87, 38.88, 37.69, 36.64, 28.22, 25.55, 23.73, 22.70, 15.10; HRMS (ESI): m/z 479.2408 [M + Na]+, calcd for C27H36NaO6, 479.2410.
14β-(2′-methoxy-4′-nitro-phenoxy)-andrographolide (12g)
From 10g in 87% yield, pale yellowish solid, mp 151–153 °C. 1H NMR (400 MHz, CDCl3) δ 8.13 (d, J = 7.1 Hz, 2H), 7.16 (q, J = 7.0, 6.5 Hz, 1H), 6.75 (d, J = 9.6 Hz, 1H), 5.64 (d, J = 5.4 Hz, 1H), 4.86 (s, 1H), 4.67 (dd, J = 10.9, 5.7 Hz, 1H), 4.39–4.32 (m, 2H), 4.12 (d, J = 11.1 Hz, 1H), 3.39 (dd, J = 11.7, 4.3 Hz, 1H), 3.29 (d, J = 11.2 Hz, 1H), 2.54–2.38 (m, 2H), 2.31 (d, J = 32.5 Hz, 5H), 2.11 (s, 3H), 1.97 (dt, J = 13.0, 6.4 Hz, 1H), 1.92–1.77 (m, 2H), 1.78–1.68 (m, 1H), 1.68–1.60 (m, 1H), 1.57 (dd, J = 12.9, 3.3 Hz, 1H), 1.34–1.15 (m, 5H), 1.08 (td, J = 13.4, 3.6 Hz, 1H), 0.59 (s, 3H); 13C NMR (101 MHz, CDCl3) δ 169.0, 151.8, 151.0, 150.6, 147.1, 143.3, 124.4, 117.4, 116.0, 108.2, 107.5, 80.4, 73.3, 70.6, 64.0, 56.4, 55.7, 55.1, 42.8, 38.9, 37.6, 36.6, 28.2, 25.7, 23.7, 22.8, 15.1; HRMS (ESI): m/z 524.2254 [M + Na]+, calcd for C27H35NNaO8, 524.2260.
14β-(2′-methyl-4′-nitro-phenoxy)-andrographolide (12h)
From 10h in 87% yield, white solid, mp 115–117 °C. 1H NMR (400 MHz, CDCl3) δ 8.13 (d, J = 7.7 Hz, 2H), 7.19 (td, J = 7.2, 1.7 Hz, 1H), 6.79–6.70 (m, 1H), 5.67 (d, J = 5.6 Hz, 1H), 4.91–4.84 (m, 1H), 4.67 (dd, J = 10.9, 5.7 Hz, 1H), 4.43 (d, J = 1.8 Hz, 1H), 4.35 (dd, J = 11.0, 1.7 Hz, 1H), 3.87 (d, J = 11.6 Hz, 1H), 3.43 (dd, J = 7.7, 3.7 Hz, 1H), 3.13 (d, J = 11.6 Hz, 1H), 2.51 (ddd, J = 16.2, 7.4, 2.6 Hz, 1H), 2.45–2.34 (m, 2H), 2.27 (s, 3H), 1.96 (ddd, J = 27.0, 12.4, 6.9 Hz, 2H), 1.83–1.66 (m, 2H), 1.65–1.44 (m, 3H), 1.33 (d, J = 6.2 Hz, 6H), 1.31–1.17 (m, 3H), 1.15 (s, 3H), 0.89 (s, 3H); 13C NMR (101 MHz, CDCl3) δ 168.7, 152.6, 149.3, 147.2, 141.0, 134.1, 126.2, 123.6, 122.3, 115.8, 108.0, 80.3, 73.1, 70.3, 64.1, 55.7, 54.8, 42.7, 38.8, 37.6, 36.5, 28.1, 25.8, 23.6, 22.6, 15.1; HRMS (ESI): m/z 508.2305 [M + Na]+, calcd for C27H35NNaO7, 508.2311.
14β-(2′-fluoro-4′-nitro-phenoxy)-andrographolide (12i)
From 10i in 91% yield, white solid, mp 162–165 °C. 1H NMR (400 MHz, CD3OD) δ 8.20–8.12 (m, 2H), 7.35 (t, J = 8.4 Hz, 1H), 7.14–7.05 (m, 1H), 6.04–5.98 (m, 1H), 4.91 (s, 1H), 4.73 (dd, J = 11.2, 5.5 Hz, 1H), 4.57 (s, 1H), 4.46 (dd, J = 11.2, 1.4 Hz, 1H), 4.03 (d, J = 11.1 Hz, 1H), 3.33 (d, J = 1.2 Hz, 1H), 3.22 (dd, J = 11.8, 4.1 Hz, 1H), 2.53 (ddd, J = 15.3, 8.0, 3.2 Hz, 1H), 2.46–2.35 (m, 2H), 2.08–1.93 (m, 2H), 1.84 (ddt, J = 12.5, 4.6, 2.3 Hz, 1H), 1.74–1.47 (m, 3H), 1.34 (qd, J = 12.8, 4.1 Hz, 1H), 1.23 (dd, J = 12.9, 2.3 Hz, 1H), 1.18 (s, 3H), 1.11–0.99 (m, 1H), 0.65 (s, 3H); 13C NMR (101 MHz, CD3OD) δ 171.0, 154.4, 153.0, 151.9, 151.8, 151.7, 148.9, 143.4, 143.3, 126.1, 122.2, 122.2, 116.7, 113.8, 113.6, 108.5, 80.8, 74.6, 72.2, 64.9, 57.7, 56.3, 43.6, 40.2, 38.9, 37.9, 28.9, 26.8, 25.2, 23.4, 15.4; HRMS (ESI): m/z 512.2099 [M + Na]+, calcd for C26H32FNNaO7, 512.2061.
14α-(4′-nitro-phenoxy)-andrographolide (13b)
From 11b in 82% yield, white solid, mp 163–166 °C. 1H NMR (400 MHz, C6D6) δ 8.18–8.13 (m, 2H), 7.25 (m, 1H), 6.91–6.86 (m, 2H), 5.21 (d, J = 10.4 Hz, 1H), 5.00 (d, J = 6.9 Hz, 2H), 4.84–4.79 (m, 2H), 4.18 (d, J = 11.1 Hz, 1H), 3.54–3.46 (m, 1H), 3.32 (d, J = 11.1 Hz, 1H), 2.48 (s, 1H), 2.43–2.35 (m, 1H), 2.13–2.02 (m, 1H), 1.96–1.76 (m, 6H), 1.43–1.14 (m, 6H), 1.25 (s, 3H), 1.23 (s, 3H), 0.66 (s, 3H); 13C NMR (101 MHz, C6D6) δ 171.54, 162.43, 147.22, 145.74, 142.21, 134.02, 125.95, 115.01, 109.07, 99.53, 74.96, 72.97, 69.92, 64.28, 52.29, 51.37, 38.36, 38.27, 38.16, 33.92, 30.89, 26.20, 25.85, 25.16, 24.63, 23.45, 16.98; HRMS (ESI): m/z 494.2185 [M + Na]+, calcd for C29H37NNaO7, 494.2155.
14α-(2′-methoxy-4′-nitro-phenoxy)-andrographolide (13g)
From 13g in 79% yield, white solid, mp 155–159 °C. 1H NMR (400 MHz, CD3OD) δ 7.93 (dd, J = 8.8, 2.6 Hz, 1H), 7.90 (d, J = 2.6 Hz, 1H), 7.14 (d, J = 8.8 Hz, 1H), 7.05 (dd, J = 9.7, 3.6 Hz, 1H), 5.85 (d, J = 5.7 Hz, 1H), 4.89 (s, 1H), 4.71 (dd, J = 10.9, 5.8 Hz, 1H), 4.65 (s, 1H), 4.44 (dd, J = 10.9, 1.8 Hz, 1H), 4.08 (d, J = 11.1 Hz, 1H), 3.96 (s, 3H), 3.36 (t, J = 5.4 Hz, 2H), 2.56–2.38 (m, 3H), 2.08–1.93 (m, 2H), 1.85 (dd, J = 12.8, 2.5 Hz, 1H), 1.78–1.58 (m, 3H), 1.36 (ddd, J = 25.0, 12.5, 4.0 Hz, 1H), 1.30–1.15 (m, 5H), 0.64 (s, 3H); 13C NMR (101 MHz, CD3OD) δ 171.45, 153.12, 152.55, 151.94, 148.73, 144.44, 126.18, 118.28, 116.58, 109.47, 108.45, 80.89, 74.76, 72.59, 64.92, 57.18, 56.87, 56.30, 43.66, 39.85, 38.88, 38.16, 28.97, 26.37, 25.18, 23.36, 15.44; HRMS (ESI): m/z: 524.2253 [M + Na]+, calcd for C27H35NNaO8, 524.2260.
General information for assay
Every compound was dissolved in DMSO and diluted with PBS into a stock solution before use. The final concentration of DMSO is 0.1% for all wells. Every experiment was performed in triplicate. Cell culture: 293T cells were maintained in MEM media with 10% fetal bovine serum (FBS), 100 U ml−1 penicillin and streptomycin and 1 mM non-essential amino acid under humidified air containing 5% CO2 at 37 °C.
Transient transfection and FXR luciferase reporter assay31
293T cells were plated in 96-well plates at 2.5 × 104 per well. After cells attached, 5.0 ng per well pCMV-GAL4-DBD-hFXR-LBD expression vector, 25.0 ng per well pFRLuciferase and 5.0 ng per well Renilla–Luciferase reporter plasmids were transiently transfected into cells using 0.25 μL per well lipofectamine (Invitrogen). After transfection for 18 h, cells were treated with an FXR natural agonist chenodeoxycholic acid (CDCA) at 25 μM in fresh MEM containing 0.5% charcoal-stripped FBS. Then, the synthesized compound was added in gradient concentrations in an antagonist mode. Luciferase activity was measured after an additional 24 h using Dual-Luciferase Reporter Assay System (Promega). The relative antagonistic activity was defined as the ratio of pFRLuciferase activity/Renilla–Luciferase activity and the activity of FXR in the presence of 25.0 μM of CDCA was set as 100%.
Cell viability assay for CC50
293T cells were plated in 96-well plates at 2.5 × 104 per well. After being cultured overnight, the cells were exposed to fresh medium containing different concentrations of synthesized compound. After culturing for an additional 24 h, cell viability was determined by 3-(4,5-dimethylthiazol-2-yl)-2,5-diphenyltetrazolium bromide (MTT) assay.
The molecular docking of compounds 10b, 12b, 10g and 12g
The Molecular docking was performed using the Lamarckian Genetic Algorithm as implemented in Autodock 4.2. The crystal structure of the target protein was retrieved from the Protein Data Bank (PDB entry: 3DCT42). The docking grids (binding site) were prepared as 60 × 60 × 60 points with a grid spacing of 0.375 Å and centred on the original ligand of the crystal structure. The set of parameters was listed as the following: the size of the population was 150 and the number of energy evaluations was set to 1.0 × 107 as the run terminates. For clustering the conformations, the root mean square deviation tolerance was 2.0. Two hundred independent docking runs were carried out for every ligand. ALL other parameters were set to default. Analysis of the results was performed using AutoDockTools and the docked structures were viewed using Discovery Studio 3.5 Client.
Acknowledgements
Authors thank Dr Qian Li for his help of the molecular docking.
Notes and references
- B. M. Forman, E. Goode, J. Chen, A. E. Oro, D. J. Bradley, T. Perlmann, D. J. Noonan, L. T. Burka, T. McMorris, W. W. Lamph, E. M. Evans and C. Weinberger, Cell, 1995, 81, 687 CrossRef CAS.
- P. Lefebvre, B. Cariou, F. Lien, F. Kuipers and B. Staels, Physiol. Rev., 2009, 89, 147 CrossRef CAS PubMed.
- H. Wang, J. Chen, K. Hollister, L. C. Sowers and B. M. Forman, Mol. Cell, 1999, 3, 543 CrossRef CAS.
- M. Makishima, A. Y. Okamoto, J. J. Repa, H. Tu, R. M. Learned, A. Luk, M. V. Hull, K. D. Lustig, D. J. Mangelsdorf and B. Shan, Science, 1999, 284, 1362 CrossRef CAS.
- D. J. Parks, S. G. Blanchard, R. K. Bledsoe, G. Chandra, T. G. Consler, S. A. Kliewer, J. B. Stimmel, T. M. Willson, A. M. Zavacki, D. D. Moore and J. M. Lehmann, Science, 1999, 284, 1365 CrossRef CAS.
- R. Pellicciari, S. Fiorucci, E. Camaioni, C. Clerici, G. Costantino, P. R. Maloney, A. Morelli, D. J. Parks and T. M. Willson, J. Med. Chem., 2002, 45, 3569 CrossRef CAS PubMed.
- S. M. Soisson, G. Parthasarathy, A. D. Adams, S. Sahoo, A. Sitlani, C. Sparrow, J. Cui and J. W. Becker, Proc. Natl. Acad. Sci. U. S. A., 2008, 105, 5337 CrossRef CAS PubMed.
- P. R. Maloney, D. J. Parks, C. D. Haffner, A. M. Fivush, G. Chandra, K. D. Plunket, K. L. Creech, L. B. Moore, J. G. Wilson, M. C. Lewis, S. A. Jones and T. M. Willson, J. Med. Chem., 2000, 43, 2971 CrossRef CAS.
- M. J. Evans, P. E. Mahaney, L. Borges-Marcucci, K. Lai, S. Wang, J. A. Krueger, S. Gardell, C. Huard, R. Martinez, G. P. Vlasuk and D. C. Harnish, Am. J. Physiol.: Gastrointest. Liver Physiol., 2009, 296, G543 CrossRef CAS PubMed.
- K. C. Nicolaou, R. M. Evans, A. J. Roecker, R. Hughes, M. Downes and J. A. Pfefferkorn, Org. Biomol. Chem., 2003, 1, 908 CAS.
- I. Dussault, R. Beard, M. Lin, K. Hollister, J. Chen, J.-H. Xiao, R. Chandraratna and B. M. Forman, J. Biol. Chem., 2003, 278, 7027 CrossRef CAS PubMed.
- N. L. Urizar, A. B. Liverman, D. T. Dodds, F. V. Silva, P. Ordentlich, Y. Yan, F. J. Gonzalez, R. A. Heyman, D. J. Mangelsdorf and D. D. Moore, Science, 2002, 296, 1703 CrossRef CAS PubMed.
- A. K. Verma, P. Khemaria, J. Gupta, D. P. Singh, B. S. Joshi, R. Roy, A. K. Mishra and R. Pratap, ARKIVOC, 2010, 2010, 1 CrossRef.
- V. Sepe, G. Bifulco, B. Renga, C. D'Amore, S. Fiorucci and A. Zampella, J. Med. Chem., 2011, 54, 1314 CrossRef CAS PubMed.
- J. Yu, J. L. Lo, L. Huang, A. Zhao, E. Metzger, A. Adams, P. T. Meinke, S. D. Wright and J. Cui, J. Biol. Chem., 2002, 277, 31441 CrossRef CAS PubMed.
- S. J. Nam, H. Ko, M. Shin, J. Ham, J. Chin, Y. Kim, H. Kim, K. Shin, H. Choi and H. Kang, Bioorg. Med. Chem. Lett., 2006, 16, 5398 CrossRef CAS PubMed.
- B. A. Carter, O. A. Taylor, D. R. Prendergast, T. L. Zimmerman, R. Von Furstenberg, D. D. Moore and S. J. Karpen, Pediatr. Res., 2007, 62, 301 CrossRef CAS PubMed.
- R. Kaimal, X. Song, B. Yan, R. King and R. Deng, J. Pharmacol. Exp. Ther., 2009, 330, 125 CrossRef CAS PubMed.
- M. Kainuma, M. Makishima, Y. Hashimoto and H. Miyachi, Bioorg. Med. Chem., 2007, 15, 2587 CrossRef CAS PubMed.
- H. Huang, Y. Yu, Z. Gao, Y. Zhang, C. Li, X. Xu, H. Jin, W. Yan, R. Ma, J. Zhu, X. Shen, H. Jiang, L. Chen and J. Li, J. Med. Chem., 2012, 55, 7037 CrossRef CAS PubMed.
- J. Y. Chiang, R. Kimmel, C. Weinberger and D. Stroup, J. Biol. Chem., 2000, 275, 10918 CrossRef CAS PubMed.
- P. A. Edwards, H. R. Kast and A. M. Anisfeld, J. Lipid Res., 2002, 43, 2 CAS.
- N. Y. Kalaany and D. J. Mangelsdorf, Annu. Rev. Physiol., 2006, 68, 159 CrossRef CAS PubMed.
- W. Huang, K. Ma, J. Zhang, M. Qatanani, J. Cuvillier, J. Liu, B. Dong, X. Huang and D. Moore, Science, 2006, 312, 233 CrossRef CAS PubMed.
- F. Yang, X. Huang, T. Yi, Y. Yen, D. D. Moore and W. Huang, Cancer Res., 2007, 67, 863 CrossRef CAS PubMed.
- S. Fiorucci, A. Mencarelli, E. Distrutti, G. Palladino and S. Cipriani, Curr. Med. Chem., 2010, 17, 139 CrossRef CAS.
- S. Fiorucci, S. Cipriani, F. Baldelli and A. Mencarelli, Prog. Lipid Res., 2010, 49, 171 CrossRef CAS PubMed.
- S. Fiorucci, S. Cipriani, A. Mencarelli, F. Baldelli, G. Bifulco and A. Zampella, Mini-Rev. Med. Chem., 2011, 11, 753 CrossRef CAS.
- T. A. M. Gulder and B. S. Moore, Curr. Opin. Microbiol., 2009, 12, 252 CrossRef CAS PubMed.
- K.-H. Lee, J. Nat. Prod., 2010, 73, 500 CrossRef CAS PubMed.
- W. Liu and C.-W. Wong, Phytother. Res., 2010, 24, 369 CrossRef CAS PubMed.
- M. K. Gorter, Recl. Trav. Chim. Pays-Bas Belg., 1911, 30, 151 CrossRef.
- M. P. Cava, W. R. Chan, L. J. Haynes, L. F. Johnson and B. Weinstein, Tetrahedron, 1962, 18, 397 CrossRef CAS.
- T. Zhang, Zhongyaocai, 2000, 23, 366 CAS.
- X. Liu, Y. Wang and G. Li, Zhongyaocai, 2003, 26, 135 Search PubMed.
- G.-Z. Liu, H.-W. Xu, K. Sun, J. Wang and H.-M. Liu, J. Org. Chem., 2008, 28, 201 CAS , and references therein.
- B. Das, C. Chowdhury, D. Kumar, R. Sen, R. Roy, P. Das and M. Chatterjee, Bioorg. Med. Chem. Lett., 2010, 20, 6947 CrossRef CAS PubMed.
- Z. Wang, P. Yu, G. Zhang, L. Xu, D. Wang, L. Wang, X. Zeng and Y. Wang, Bioorg. Med. Chem., 2010, 18, 4269 CrossRef CAS PubMed.
- J. C. Lim, T. K. Chan, D. S. Ng, S. R. Sagineedu, J. Stanslas and W. S. Wong, Clin. Exp. Pharmacol. Physiol., 2012, 39, 300 CrossRef CAS PubMed , and references therein.
- B. Zhou, D. Zhang and X. Wu, Mini-Rev. Med. Chem., 2013, 13, 298 CAS , and references therein.
- Some of compounds were published in the patent CN103224492 A 20130731 by G.-C. Zhou, C.-W. Wong, Z. Liu, D. Sheng, X. Nie and D. Wang.
- A. Akwabi-Ameyaw, J. Y. Bass, R. D. Caldwell, J. A. Caravella, L. Chen, K. L. Creech, D. N. Deaton, S. A. Jones, I. Kaldor, Y. Liu, K. P. Madauss, H. B. Marr, R. B. McFadyen, A. B. Miller, F. N. Iii, D. J. Parks, P. K. Spearing, D. Todd, S. P. Williams and G. B. Wisely, Bioorg. Med. Chem. Lett., 2008, 18, 4339 CrossRef CAS PubMed.
Footnotes |
† Electronic supplementary information (ESI) available: Table S1, 1H NMR and 13C NMR spectra of compounds 5–13. CCDC 960845. For ESI and crystallographic data in CIF or other electronic format see DOI: 10.1039/c3ra46715e |
‡ Current address: Department of Pharmaceutical and Chemical Engineering, Taizhou Polytechnic College, Taizhou 225300, Jiangsu, PR China. |
|
This journal is © The Royal Society of Chemistry 2014 |
Click here to see how this site uses Cookies. View our privacy policy here.