DOI:
10.1039/C3RA46095A
(Paper)
RSC Adv., 2014,
4, 5316-5321
Nanoaggregates of benzothiazole-based amidine-coupled chemosensors: a chemosensor for Ag+ and the resultant complex as a secondary sensor for Cl−†
Received
24th October 2013
, Accepted 16th December 2013
First published on 16th December 2013
Abstract
Nanoaggregates of benzothiazole-based amidines were prepared for study as sensors in aqueous medium. Sensor N2 was found to behave as a selective primary sensor for Ag+, and the subsequent complex N2·Ag+ acted as a secondary sensor for Cl− in aqueous medium through a cation displacement mechanism. The cation displacement mechanism was further supported by a CV titration of complex N2·Ag+ with Cl−.
Introduction
Designing chemosensors that include benzothiazole and benzimidazole structures for in vivo recognition of analytes is a dynamic area of research.1 A number of dipodal or tripodal sensors based on benzothiazole and benzimidazole moieties with different substituents have been developed for sensing numerous analytes via UV-vis and fluorescence spectroscopy.2
Silver ion has strong tissue healing and antimicrobial properties against a wide range of bacteria, for which it has been used for hundreds of years.3 Silver also plays a major role in different industrial fields such as photography imaging, soldering production, electrical and electronics, automobiles, and as a catalyst in oxidation reactions.4 Ionic silver in small amounts is nontoxic; however, an excess of silver has harmful effects on the environment and on the human body.5 Examples of the biological effects of silver include a cytotoxic effect on pollen (the male gametophyte of higher plants),6 induction of apoptosis and necrosis in THP-1,7 inhibition of mitochondrial electron transport by the disappearance of metallothionein in human bronchial epithelial cells,8 cytotoxic effects on fibroblasts and endothelial cells,9 and reduction of the glycolytic rate to 20–30 percent of normal, which leads to reduced lactate formation and increased pyruvate formation.10 Chloride ion also plays crucial roles in various cellular functions including regulation of the transport system in animals.11 Thus, developing sensors for silver and chloride ion is quite important. Numerous sensors for quantitative and qualitative detection of silver and chloride ion in organic solvents or semi-aqueous media are available.12–14 However, their detection in pure aqueous medium would have a number of additional benefits.15
As progress in our ongoing research, we report the synthesis of benzothiazole-based amidine-coupled compounds for naked eye primary sensing of cations and secondary sensing of anions based on a cation displacement assay. The cation displacement assay involves decomplexation of the cation from the metal complex in the presence of a particular anion, which restores the original photophysical properties of the receptor.16 Unlike the recognition of anions with a receptor fabricated with purely hydrogen bond donor sites, anion recognition with cation displacement is rarely diminished in aqueous medium.17 To address the sensor activity in aqueous medium, the sensors were processed into nanoaggregates in an aqueous solvent system. Nanoaggregates have a wide range of applications in biology and chemistry, and they have recently been studied for modulation of the photophysical properties of organic receptors for sensor applications.18
Results and discussions
Compounds 1 and 2 were synthesized by the condensation of N,N-dimethylformamide dimethyl acetal with 2-aminobenzothiazole or 6-nitro-2-aminobenzothiazole in boiling MeOH. Compound 3 was synthesized by reduction of compound 2 with an excess of NaBH4 in MeOH (Scheme 1).
 |
| Scheme 1 Structures of compounds 1–3. | |
The absorption profiles of sensors 1–3 were measured in methanol (Fig. S1†). Sensor 1 exhibited a weak absorption band at 300 nm. Sensors 2 and 3 displayed absorptions at 345 and 346 nm, respectively, with a bathochromic shift due to the nitro group.19 In order to investigate the properties of sensors 2 and 3 in aqueous medium, the sensors were processed into organic nanoparticles through a bottom up technique. This process involved the time regulated injection of a solution of sensor 2 or 3 in MeOH into deionized water. The nanoaggregated materials are represented as N2 and N3, respectively. The formation of organic nanoparticles led to a bathochromic shift of absorption maxima relative to sensor 2 or 3 (Fig. S1†). Imines are frequently found to be unstable in water. Hence, we recorded time dependent UV-vis spectra of sensors N2 and N3, which demonstrated that both compounds were stable for 6 days. The nanoaggregates were measured with a particle size analyzer based on dynamic light scattering (DLS) to have a size of ∼30 nm (Fig. S2†).
The effect of pH on sensors N2 and N3 was investigated by UV-vis spectroscopy of solutions of sensors N2 or N3 in aqueous medium with varying pH as shown in Fig. S3.† Sensors N2 and N3 were stable in the pH 4–12 range, as the absorption profiles remained almost unaltered in this range. In highly acidic pH, sensors N2 and N3 appeared to decompose since at a pH below 4, the absorption peak at 355 nm diminished. The effect of pH on the photophysical properties of the sensors was avoided by performing further studies in a HEPES buffer solution.
The sensing ability of sensor N2 towards different metal ions was studied through UV-vis absorption spectroscopy. Changes in the UV-vis absorption spectra of the solution of sensor N2 in HEPES buffered aqueous solution upon the addition of nitrate salts of a wide range of cations including Na+, K+, Ba2+, Sr2+, Ca2+, Mg2+, Mn2+, Cr3+, Fe3+, Co2+, Ni2+, Cu2+, Zn2+, Pb2+, Ag+, Hg2+, and Cd2+ were investigated. Sensor N2 selectively binds Ag+ with a bathochromic shift in the absorption spectra from 355 nm to 410 nm with color changes from straw yellow to dark yellow color upon subsequent addition of AgNO3 (Fig. 1A). Sensor 2 has an absorption band at 345 nm due to the intramolecular charge transfer (ICT) prevailed in benzothiazole moiety. This band was red shifted by the formation of organic nanoparticles, N2, due to the change in the conformation from isolated twisted to planar, resulting in the stacking of molecules.20 Upon the addition of Ag+ ion, absorption spectra of N2 was further shifted toward higher wavelength with λmax = 410 nm (bathochromic shift) due to the modulation of ICT as a result of binding of Ag+ ion to sensor N2. Sensor N2 has a narrow size distribution (Fig. S2†) whereas N2·Ag+ has a wide range of particle size (Fig. S4†). Upon addition of Ag+, the particle size increased up to 300 nm with average particle size of 200 nm. This can be explained with the fact that the Ag+ coordination leads to enhance the particle size by coordinating with the binding sites available on different nanoaggregates. To analyze the role of binding sites, the binding behavior of sensor N3 was also examined for metal ion binding. Sensor N3 exhibited the same selective binding of Ag+ as sensor N2 (Fig. 1B), which indicated that there was a negligible effect of changing the sp2 N atom to an sp3 N atom on the binding ability of the sensor. Fluorescence responses of sensors N2 and N3 were also examined, resulting in no selectivity toward any metal ions (Fig. S5†).
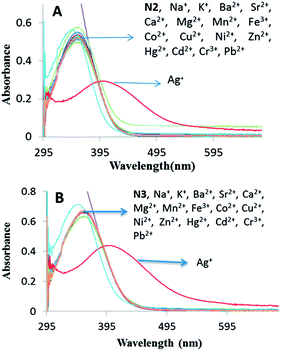 |
| Fig. 1 Changes in the UV-vis spectra of a 20 μM solution of (A) sensor N2 and (B) sensor N3 in HEPES buffered aqueous solution (20 mM, pH = 7.0) in the presence of different metal nitrate salts (200 μM). | |
Fig. 2 displays the titration profiles of sensors N2 and N3 with incrementally increased concentrations of Ag+. Gradual addition of Ag+ to sensors N2 and N3 resulted in the gradual decrease in absorbance at 355 nm and increase in absorbance at 410 nm with an isosbestic point at 385 nm, inducing a significant ratiometric sensing.
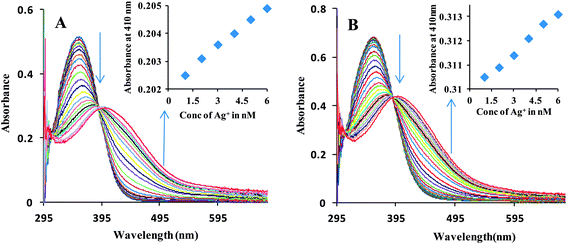 |
| Fig. 2 Changes in the UV-vis absorption spectra of (A) sensor N2 and (B) sensor N3 (20 μM) in HEPES buffered aqueous solution (20 mM, pH = 7.0) with increasing concentrations of Ag+ (0–200 μM). | |
The selectivity of sensors N2 and N3 for Ag+ was evaluated by performing competitive experiments of sensors N2 and N3 with Ag+ in the presence of other cations. Sensor N2 was found to selectively bind Ag+ over all other cations as the UV-vis absorption spectra of sensor N2 with Ag+ remained unaltered in the presence of other tested cations (Fig. 3A). However, sensor N3 displayed interference with a number of cations as illustrated by the changes in absorbance in Fig. 3B. The stoichiometry of complex 2·Ag+ was determined to be 1
:
1 with a Job's plot.21 The binding constants were calculated by Benesi–Hildebrand plots to be 3.08 (±0.1) × 103 M−1 for complex N2·Ag+ and 4.30 (±0.2) × 103 M−1 for complex N3·Ag+, respectively (Fig. S6†).22 The limits of Ag+ detection with sensors N2 and N3 were found to be 1.26 nM and 1.31 nM, respectively.23
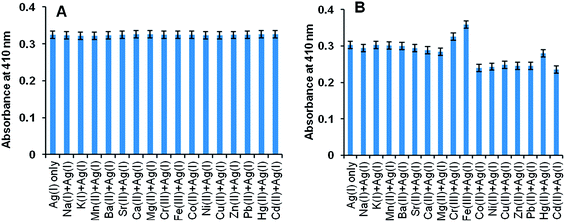 |
| Fig. 3 Bar diagram showing the absorbance of (A) sensor N2 and (B) sensor N3 (20 μM) binding to Ag+ (200 μM) in the presence of other cations (200 μM) in HEPES aqueous solution (20 mM, pH = 7.0) at 410 nm. | |
Mechanistically, the nature of the binding in the receptor–ion interaction was investigated with a 1H NMR titration experiment. The series of 1H NMR spectra of sensor 2 upon addition of increasing amounts of silver nitrate (0.5 equiv.) in D2O are shown in Fig. 4. Upon incremental addition of 0.5 equivalents of silver nitrate, a shift in the 1H NMR signals of the Schiff base proton from 8.24 to 8.32 ppm was observed. Furthermore, two singlets at 3.33 and 3.35 ppm due to –N(CH3)2 merged and shifted to a singlet at 3.72 ppm (Fig. 4). These observations support the vital involvement of the fabricated binding sites of sensor 2 in its binding of Ag+ ion.
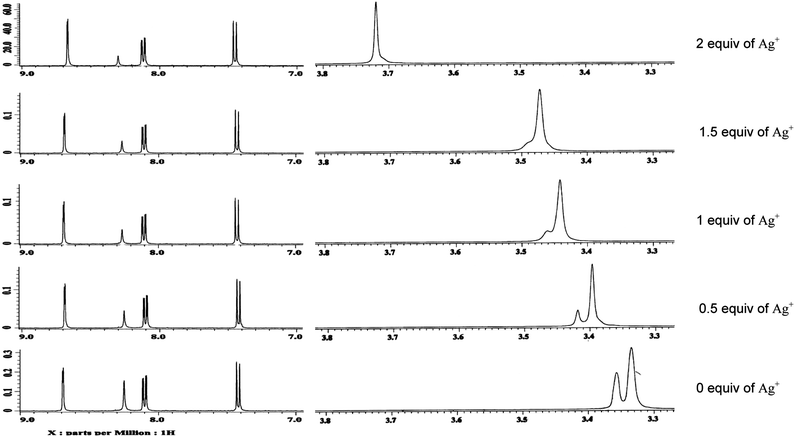 |
| Fig. 4 Family of 1H NMR spectra showing changes in signals of sensor 2 (10 mM) upon complexation with Ag+ in a DMSO-d6/D2O (95 : 5, v/v) solvent system. | |
It is known that Ag+ binds strongly with halides. This property of Ag+ encouraged us to examine the halide sensing behavior of N2·Ag+ complex. UV-vis spectra of a solution of complex N2·Ag+ in HEPES buffered aqueous solution was recorded in the presence of tetrabutylammonium salt of various halides. Complex N2·Ag+ showed a selective affinity for Cl− over other halides (Fig. 5). The addition of Cl− to complex N2·Ag+ resulted in displacement of Ag+ from the complex, as indicated by the shift of the UV-vis absorption maxima from 410 nm to 355 nm. When Cl− ions were added to a solution of N2·Ag+, the size of the nanoaggregates was decreased up to 60 nm (average particle size) (Fig. S7†). It is only possible, if Cl− ions expel out Ag+ ions from the coordination sphere of N2 and thus, those nanoaggregates, which were assembled due to Ag+ coordination, are now separated from each other.
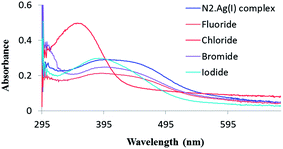 |
| Fig. 5 Changes in UV-vis spectra of complex N2·Ag+ (20 μM) in HEPES buffered aqueous solution (20 mM, pH = 7.0) in the presence of tetrabutylammonium halide salts (200 μM). | |
Stepwise changes in the UV-vis absorption spectra of complex N2·Ag+ were observed in the titration with Cl−. Upon slowly increasing the concentration of Cl− in a solution of complex N2·Ag+ in HEPES buffered aqueous solution, the absorbance at 410 nm decreased and the absorbance at 355 nm slowly increased (Fig. 6). Careful analysis of Fig. 2 and 6 revealed that the addition of Cl− to the complex generated the original spectrum of uncomplexed sensor N2, which indicates a cation displacement mechanism is operating for the sensing of chloride. However, upon addition of lower equivalents of Cl−, the absorption signal of complex N2·Ag+ did not match the titration performed with sensor N2 and Ag+. This led to the conclusion that upon addition of fewer equivalents of Cl−, an anion displacement mechanism is operating, which upon further titration shifted to cation displacement. This supposition was confirmed by the formation of AgCl precipitates, which generated broadening of the base line in UV-vis absorption spectra. From the UV-vis titration curve of complex N2·Ag+ with Cl− (Fig. 6), it was apparent that upon initial addition of Cl−, there was a decline in the absorbance at 410 nm and no increase at 355 nm. This result indicates that Cl− initially displaces the nitrate counter ion from the metal complex. Ag+ remains in the coordination cavity with up to 5 equivalents of Cl− in this counter ion displacement mechanism. However, upon further addition of Cl−, Ag+ is removed from the coordination cavity, resulting in precipitation of AgCl following cation displacement. The detection limit for analysis of Cl− by complex N2·Ag+ was found to be 3.89 nM (Fig. 6).22 Binding constant was calculated to be 6.9 (±0.3) × 103 M−1 for complex N2·Ag+ and Cl− using Benesi–Hildebrand plot (Fig. S8†).21 Selective binding of Cl− with complex N2·Ag+ was confirmed by performing competitive experiments of complex N2·Ag+ with Cl− in the presence of other halides (Fig. S9†).
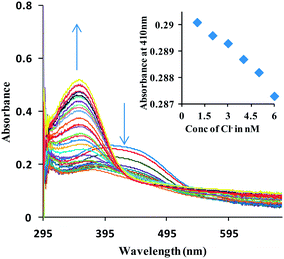 |
| Fig. 6 Changes in the UV-vis absorption spectra of complex N2·Ag+ (20 μM) with increasing concentrations of Cl− (0–200 μM) in HEPES buffered aqueous solution (20 mM, pH = 7.0). | |
Sensor N2 was inactive in the chosen potential range as there was no oxidation or reduction peaks in its CV profile (Fig. 7). After adding Ag+ (200 μM) to a buffered solution of sensor N2 (20 μM), an oxidation peak at −0.25 V and a reduction peak at 0.35 V arose in the CV profile, which suggests that the binding of Ag+ to sensor N2 alters its electrochemical structure and leads to generation of oxidation and reduction peaks. Treatment of complex N2·Ag+ with increasing concentrations of Cl− resulted in the disappearance of the oxidation and reduction peaks and reversion to the CV profile of sensor N2, which further confirms the cation displacement mechanism.
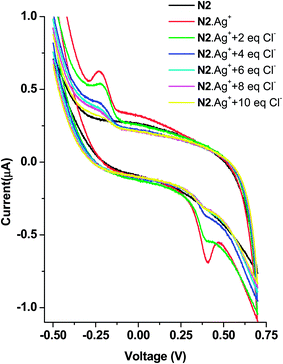 |
| Fig. 7 CV profiles of sensor N2, complex N2·Ag+, and complex N2·Ag+ + Cl− in HEPES-buffered aqueous solution (20 mM, pH = 7.0). | |
Conclusions
In conclusion, nanoaggregates N1–N3 were prepared, and their sensor activity was studied. The size of the nanoaggregates was established through dynamic light scattering measurements. Nanoaggregates of sensor N2 were found to be selective for Ag+, and the complex N2·Ag+ was selective for Cl− through a cation displacement mechanism. CV titration of complex N2·Ag+ with Cl− confirmed the cation displacement mechanism by the reemergence of the sensor N2 profile.
Experimental section
Compound 1 was prepared according to a literature method.24
N,N-Dimethyl-N′-(6-nitrobenzo[d]thiazol-2-yl)formamidine (2)
A solution of 6-nitro-2-aminobenzothiazole (1.95 g, 10 mmol) and N,N-dimethylformamide dimethyl acetal (1.31 g, 11 mmol) in MeOH was refluxed for 13 h. After completion of the reaction, the solvent was evaporated under reduced pressure, and the product was separated by silica gel column chromatography using 6% chloroform in hexane as eluent to obtain compound 2 (2.10 g, 85%) as a pale yellow solid; mp 92–94 °C; IR (MeOH): 1612 (s), 1470 (s), 1431 (w), 1323 (m), 1091 (m), 751 (m) cm−1; 1H NMR (400 MHz, CDCl3) δ 8.69 (d, 1H, J = 2.5 Hz, ArH), 8.24 (s, 1H, –HC
N), 8.00 (dd, 1H, J = 2.4 and 9.2 Hz, ArH), 7.42 (d, 1H, J = 8.9 Hz, ArH), 3.35 (s, 3H, N(CH3)2), 3.33 (s, 3H, N(CH3)2); 13C NMR (75 MHz, CDCl3) δ 171.1, 157.6, 150.5, 140.9, 131.2, 121.4, 116.7, 116.4, 30.3. Anal. calcd for C10H10N4O2S: C, 47.99; H, 4.03; N, 22.39; found C, 47.89; H, 3.94; N, 22.32.
N-[(Dimethylamino)methyl]-6-nitrobenzo[d]thiazol-2-amine (3)
NaBH4 (950 mg, 25 mmol) was added in portions to a solution of amidine 2 (625 mg, 2.5 mmol) in MeOH (20 mL). After 12 h, the reaction was quenched with ice/water, concentrated under vacuum to remove MeOH, and extracted with diethyl ether (3 × 50 mL). The combined organic layers were washed with brine, dried over anhydrous Na2SO4, filtered, and evaporated under reduced pressure. The crude oily residue was purified by silica gel column chromatography using 2% chloroform in hexane as eluent to give compound 3 (504 mg, 80%) as a pale yellow solid; mp 90–91 °C; IR (MeOH): 1615 (s), 1465 (s), 1423 (w), 1331 (m), 1099 (m), 749 (m) cm−1; 1H NMR (400 MHz, CDCl3) δ 8.01 (s, 1H, ArH), 7.66 (dd, 1H, J = 2.3 and 8.6 Hz, ArH), 7.41 (s, 1H, NH), 6.97 (d, 1H, J = 8.7 Hz, ArH), 2.81 (s, 2H, CH2) 2.13 (s, 3H, N(CH3)2), 2.12 (s, 3H, N(CH3)2); 13C NMR (75 MHz, CDCl3) δ 171.2, 160.1, 151.8, 141.1, 131.9, 121.6, 116.7, 72.2, 30.4. Anal. calcd for C10H12N4O2S: C, 47.61; H, 4.79; N, 22.21; found C, 47.53; H, 4.71; N, 22.15.
Synthesis of complex 2·Ag+
The silver complex of compound 2 was synthesized by reaction of a 1 M solution of AgCl in DMSO (2 mL) and compound 2 (5 mg in DMSO) in methanol. The silver complex 2.Ag+ was characterized by spectroscopic analysis. MS (ESI): m/z (M + Ag+) 357.2. Anal. calcd for C10H10AgN4O2S: C, 33.54; H, 2.81; N, 15.64; found C, 33.43; H, 2.75; N, 15.58.
Preparation of nanoparticles of compounds 1–3
Nanoparticles of compounds 1–3 were prepared by slowly injecting 2 mL (5 mM in methanol) solution of each compound under ultrasonic irradiation to 500 mL of water. Compounds immediately aggregated to form a dispersion of nanoaggregates in water with a concentration of 20 μM.
Acknowledgements
This work was supported by the Korean and Indian Governments for the India–Korea Joint Program of Cooperation in Science & Technology (NRF-2011-0027710) and by the CSIR project (project no.: 01(2417)/10/EMR-II). PS would like to thank UGC India for her research fellowship.
References
-
(a) C. I. C. Esteves, M. M. M. Raposo and S. P. G. Costa, Tetrahedron, 2010, 66, 7479 CrossRef CAS PubMed;
(b) V. S. Patil, V. Padalkar, A. B. Tathe and N. Sekar, Dyes Pigm., 2013, 98, 507 CrossRef CAS PubMed;
(c) F. Wang, S. W. Nam, Z. Guo, S. Park and J. Yoon, Sens. Actuators, B, 2012, 161, 948 CrossRef CAS PubMed;
(d) H. Wang, G. Chen, X. Xu, H. Chen and S. Ji, Dyes Pigm., 2010, 86, 238 CrossRef CAS PubMed;
(e) A. K. Mahapatra, J. Roy, S. K. Manna, S. Kundu, P. Sahoo, S. K. Mukhopadhyay and A. Banik, J. Photochem. Photobiol., A, 2012, 240, 26 CrossRef CAS PubMed.
-
(a) D. Y. Lee, N. Singh, M. J. Kim and D. O. Jang, Org. Lett., 2011, 13, 3024 CrossRef CAS PubMed;
(b) N. Singh and D. O. Jang, Org. Lett., 2007, 9, 1991 CrossRef CAS PubMed;
(c) M. J. Kim, K. Kaur, N. Singh and D. O. Jang, Tetrahedron, 2012, 68, 5429 CrossRef CAS PubMed;
(d) H. J. Jung, N. Singh, D. Y. Lee and D. O. Jang, Tetrahedron Lett., 2009, 50, 5555 CrossRef CAS PubMed;
(e) H. J. Jung, N. Singh and D. O. Jang, Tetrahedron Lett., 2008, 49, 2960 CrossRef CAS PubMed;
(f) N. Singh, G. W. Lee and D. O. Jang, Tetrahedron, 2008, 64, 1482 CrossRef CAS PubMed;
(g) K. S. Moon, N. Singh, G. W. Lee and D. O. Jang, Tetrahedron, 2007, 63, 9106 CrossRef CAS PubMed;
(h) C. Kar, A. Basu and G. Das, Tetrahedron Lett., 2012, 53, 4754 CrossRef CAS PubMed;
(i) D. Y. Lee, N. Singh and D. O. Jang, Tetrahedron Lett., 2010, 51, 1103 CrossRef CAS PubMed;
(j) S. Kumar, S. Arora, P. Singh and S. Kumar, RSC Adv., 2012, 2, 9969 RSC.
-
(a) J. Wu, S. Hou, D. Ren and P. T. Mather, Biomacromolecules, 2009, 10, 2686 CrossRef CAS PubMed;
(b) T. Hrkac, C. Röhl, R. Podschun, V. Zaporojtchenko, T. Strunskus, H. Papavlassopoulos, D. Garbe-Schönberg and F. Faupel, Mater. Sci. Eng., C, 2013, 33, 2367 CrossRef CAS PubMed;
(c) L. Lin, H. Cui, G. Zeng, M. Chen, H. Zhang, M. Xu, X. Shen, C. Bortolini and M. Dong, J. Mater. Chem. B, 2013, 1, 2719 RSC;
(d) X. Wang, Y. Dai, J. L. Zou, L. Y. Meng, S. Ishikawa, S. Li, M. Abuobeidah and H. G. Fu, RSC Adv., 2013, 3, 11751 RSC;
(e) D. R. Monteiro, L. F. Gorup, A. S. Takamiya, A. C. Ruvollo-Filho, E. R. D. Camargo and D. B. Barbosa, Int. J. Antimicrob. Agents, 2009, 34, 103 CrossRef CAS PubMed.
- J. Park, W. Choi, K. Jang and S. Na, Biosens. Bioelectron., 2013, 41, 471 CrossRef CAS PubMed.
-
(a) J. Y. Roh, S. J. Sim, J. Yi, K. Park, K. H. Chung, D. Y. Ryu and J. Choi, Environ. Sci. Technol., 2009, 43, 3933 CrossRef CAS;
(b) E. A. Zatulovskiy, A. N. Skvortsov, P. Rusconi, E. U. Ilyechova, P. S. Babich, N. V. Tsymbalenko, M. Broggini and L. V. Puchkova, J. Inorg. Biochem., 2012, 116, 88 CrossRef CAS PubMed.
- A. Speranza, R. Crinelli, V. Scoccianti, A. R. Taddei, M. Iacobucci, P. Bhattacharyae and P. C. Ke, Environ. Pollut., 2013, 179, 258 CrossRef CAS PubMed.
- R. Foldbjerg, P. Olesen, M. Hougaard, D. A. Dang, H. J. Hoffmann and H. Autrup, Toxicol. Lett., 2009, 190, 156 CrossRef CAS PubMed.
- T. Miyayama, Y. Arai, N. Suzuki and S. Hirano, Toxicology, 2013, 305, 20 CrossRef CAS PubMed.
- E. Hidalgo and C. Domínguez, Toxicol. Lett., 1998, 98, 169 CrossRef CAS.
- S. Baar, Burns, 1975, 2, 58 CrossRef.
- N. Sperelakis, Cell Physiology Source Book: A Molecular Approach, Ohio, USA, 3rd edn, 2001, pp. 301–318 Search PubMed.
- For sensing Ag+ in organic solvent:
(a) H. Wang, L. Xue, Y. Qian and H. Jiang, Org. Lett., 2010, 12, 292 CrossRef CAS PubMed;
(b) H. Lin, M. E. Cinar and M. Schmittel, Dalton Trans., 2010, 39, 5130 RSC;
(c) M. Kandaz, O. Güney and F. B. Senkal, Polyhedron, 2009, 28, 3110 CrossRef CAS PubMed;
(d) D. Ray, E. Iyer, S. Siva, K. K. Sadhu and P. K. Bharadwaj, Dalton Trans., 2009, 5683 RSC;
(e) H. Hung, C. Cheng, Y. Wang, Y. Chen and W. Chung, Eur. J. Org. Chem., 2009, 6360 CrossRef CAS;
(f) R. Joseph, B. Ramanujam, A. Acharya and C. P. Rao, J. Org. Chem., 2009, 74, 8181 CrossRef CAS PubMed;
(g) L. Liu, D. Zhang, G. Zhang, J. Xiang and D. Zhu, Org. Lett., 2008, 10, 2271 CrossRef CAS PubMed;
(h) C. Huang, X. Peng, Z. Lin, J. Fan, A. Ren and D. Sun, Sens. Actuators, B, 2008, 133, 113 CrossRef CAS PubMed;
(i) M. Shamsipur, K. Alizadeh, M. Hosseini, C. Caltagirone and V. Lippolis, Sens. Actuators, B, 2006, 113, 892 CrossRef CAS PubMed;
(j) A. Coskun and E. U. Akkaya, J. Am. Chem. Soc., 2005, 127, 10464 CrossRef CAS PubMed;
(k) S. Kim, J. Lee, S. Lee, M. Lim, S. Lee, W. Sim and J. S. Kim, J. Org. Chem., 2004, 69, 2877 CrossRef CAS PubMed;
(l) H. Tong, L. X. Wang, X. B. Ting and F. Wang, Macromolecules, 2002, 35, 7169 CrossRef CAS;
(m) J. Kang, M. Choi, E. Y. Lee and J. Yoon, J. Org. Chem., 2002, 67, 4384 CrossRef CAS PubMed;
(n) J. Parker and T. E. Glass, J. Org. Chem., 2001, 66, 6505 CrossRef PubMed;
(o) K. Rurack, M. Kollmannsberger, U. ReschGenger and J. Daub, J. Am. Chem. Soc., 2000, 122, 968 CrossRef CAS.
- For sensing Ag+ in semi aqueous conditions:
(a) Y. Fu, L. Mu, X. Zeng, J.-L. Zhao, C. Redshaw, X.-L. Ni and T. Yamato, Dalton Trans., 2013, 42, 3552 RSC;
(b) H. Zheng, M. Yan, X.-X. Fan, D. Sun, S.-Y. Yang, L.-J. Yang, J.-D. Li and Y.-B. Jiang, Chem. Commun., 2012, 2243 RSC;
(c) L. Xu, Y. Xu, W. Zhu, C. Yang, L. Han and X. Qian, Dalton Trans., 2012, 41, 7212 RSC;
(d) F. Wang, R. Nandhakumar, J. H. Moon, K. M. Kim, J. Y. Lee and J. Yoon, Inorg. Chem., 2011, 50, 2240 CrossRef CAS PubMed;
(e) C. Lin, C. Yu, Y. Lin and W. Tseng, Anal. Chem., 2010, 82, 6830 CrossRef CAS PubMed;
(f) Z. Xu, S. Zheng, J. Yoon and D. R. Spring, Analyst, 2010, 135, 2554 RSC;
(g) C. Park, J. Lee, E. Kang, J. Lee and S. Lee, Tetrahedron Lett., 2009, 50, 671 CrossRef CAS PubMed;
(h) K. Swamy, H. Kim, J. Soh, Y. Kim, S. Kim and J. Yoon, Chem. Commun., 2009, 1234 RSC;
(i) A. Chatterjee, M. Santra, N. Won, S. Kim, J. Kim, S. Kim and K. H. Ahn, J. Am. Chem. Soc., 2009, 131, 2040 CrossRef CAS PubMed;
(j) S. Lyoshi, M. Taki and Y. Yamamoto, Inorg. Chem., 2008, 47, 3946 CrossRef PubMed;
(k) M. Schmittel and H. Lin, Inorg. Chem., 2007, 46, 9139 CrossRef CAS PubMed;
(l) R. Yang, W. Chan, A. Lee, P. Xia, H. Zhang and K. Li, J. Am. Chem. Soc., 2003, 125, 2884 CrossRef CAS PubMed.
- For sensing Ag+ in aqueous conditions: H. Wang, L. Xue and H. Jiang, Org. Lett., 2011, 13, 3844 CrossRef CAS PubMed.
- For sensing Cl−:
(a) T. Pinter, C. Simhadri and F. Hof, J. Org. Chem., 2013, 78, 4642 CrossRef CAS PubMed;
(b) A. Kaur, H. Sharma, S. Kaur, N. Singh and N. Kaur, RSC Adv., 2013, 3, 6160 RSC;
(c) C. Caltagirone, C. Bazzicalupi, A. Bencini, F. Isaia, G. Alessandra and V. Lippolis, Supramol. Chem., 2012, 24, 95 CrossRef CAS;
(d) C. Do-Thanh, N. Khanal, Z. Lu, S. Cramer, D. Jenkins and M. Best, Tetrahedron, 2012, 68, 1669 CrossRef CAS PubMed;
(e) B. Wu, C. Jia, X. Wang, S. Li, X. Huang and X.-J. Yang, Org. Lett., 2012, 14, 684 CrossRef CAS PubMed;
(f) M. Wenzel, J. R. Hiscock and P. A. Gale, Chem. Soc. Rev., 2012, 41, 480 RSC;
(g) C. J. E. Haynes and P. A. Gale, Chem. Commun., 2011, 47, 8203 RSC;
(h) P. R. Brotherhood and A. P. Davis, Chem. Soc. Rev., 2010, 39, 3633 RSC;
(i) J. T. Davis, O. Okunola and R. Quesada, Chem. Soc. Rev., 2010, 39, 3843 RSC;
(j) G. W. Gokel and I. A. Carasel, Chem. Soc. Rev., 2007, 36, 378 RSC;
(k) B. Schazmann, N. Alhashimy and D. Diamond, J. Am. Chem. Soc., 2006, 128, 8607 CrossRef CAS PubMed.
- P. Saluja, N. Kaur, N. Singh and D. O. Jang, Tetrahedron Lett., 2012, 53, 3292 CrossRef CAS PubMed.
-
(a) H. Wang, L. Xue and H. Jiang, Org. Lett., 2011, 13, 3844 CrossRef CAS PubMed;
(b) Z. Xu, J. Pan, D. R. Spring, J. Cui and J. Yoon, Tetrahedron, 2010, 66, 1678 CrossRef CAS PubMed.
-
(a) X. Zhang, X. Zhang, B. Yang, S. Wang, M. Liu, Y. Zhang, L. Tao and Y. Wei, RSC Adv., 2013, 3, 9633 RSC;
(b) A. M. Smith, H. Duan, A. M. Mohs and S. Nie, Adv. Drug Delivery Rev., 2008, 60, 1226 CrossRef CAS PubMed;
(c) X. Zhang, S. Wang, L. Xu, Y. Ji, L. Feng, L. Tao, S. Li and Y. Wei, Nanoscale, 2012, 4, 5581 RSC.
- M. A. R. Ahamed and A. R. Burkanudeen, J. Inorg. Organomet. Polym. Mater., 2012, 22, 1046 CAS.
-
(a) P. Khullar, V. Singh, A. Mahal, H. Kumar, G. Kaur and M. S. Bakshi, J. Phys. Chem. B, 2013, 117, 3028 CrossRef CAS PubMed;
(b) P. Khullar, A. Mahal, T. S. Banipal, G. Kaur and M. S. Bakshi, Langmuir, 2010, 26, 11363 CrossRef CAS PubMed;
(c) B.-K. An, S.-K. Kwon, S.-D. Jung and S. Y. Park, J. Am. Chem. Soc., 2002, 124, 14410 CrossRef CAS PubMed.
- P. Job, Ann. Chim., 1928, 9, 113 CAS.
- H. Benesi and H. Hildebrand, J. Am. Chem. Soc., 1949, 71, 2703 CrossRef CAS.
- M. Shortreed, R. Kopelman, M. Kuhn and B. Hoyland, Anal. Chem., 1996, 68, 1414 CrossRef CAS.
- C. Landreau, D. Deniaud, M. Evain, A. Reliquet and J. C. Meslin, J. Chem. Soc., Perkin Trans. 1, 2002, 741 RSC.
Footnote |
† Electronic supplementary information (ESI) available: Additional spectroscopic data are available as supplementary data. See DOI: 10.1039/c3ra46095a |
|
This journal is © The Royal Society of Chemistry 2014 |