DOI:
10.1039/C3RA45658G
(Paper)
RSC Adv., 2014,
4, 3368-3382
Synthesis of α-D-Glcp-(1→3)-α-D-Galf-(1→2)-α-L-Rhap constituent of the CPS of Streptococcus pneumoniae 22F. Effect of 3-O-substitution in 1,2-cis α-D-galactofuranosylation†
Received
11th October 2013
, Accepted 15th November 2013
First published on 19th November 2013
Abstract
The synthesis of the trisaccharide α-D-Glcp-(1→3)-α-D-Galf-(1→2)-L-Rhap (3) constituent of Streptococcus pneumonia 22F was achieved with complete diastereoselectivity. This is the first example of a synthesis of an internal α-D-Galf containing oligosaccharide of a pathogen microorganism. Allyl α-D-galactofuranoside, used as novel precursor of the internal Galf, allowed the introduction of an orthogonal group at O-3. The trichloroacetimidate method was used for the construction of 1,2-cis-α-D-galactofuranosyl linkage. The influence of the 3-O-substituent (PMB, Bz, PFBz, PMBz, TIPS) was evaluated in benzylated galactofuranosyl trichloroacetimidate donors in terms of yield and selectivity of α-D-Galf-(1→2)-α-L-Rhap product as well as donor rearrangement by-product. Complete stereoselectivity was observed with all protecting groups used in the Galf donor, but the 3-O-benzoyl substitution gave the best yield. Protective groups were also evaluated in the rhamnoside acceptor, benzyl substitution was a requirement for complete stereoselectivity.
Introduction
Carbohydrates play important roles in biology1 and the need for well-defined oligosaccharides for understanding those roles turned the synthesis of oligosaccharides into a major issue.2,3 The glycosylation reaction is pivotal in this matter.4–7 Among the difficulties in the construction of glycopyranosyl linkages, 1,2-cis-β-mannopyranosides as well as β-rhamnopyranosides and α-2-deoxyglycopyranosides are the most difficult to prepare stereoselectively.8 A β-mannopyranosyl equivalent in furanoses is the β-Araf8 which has been studied over the last years8–12 mainly due to its presence in a motif of the Mycobacterium tuberculosis arabinogalactan and lipoarabinomannan.13–15 However, studies in stereoselective furanosylation are more limited compared to pyranosides.4 L-Arabinose shows the same stereochemistry at C-2, C-3 and C-4 as D-galactose; however, the synthesis of 1,2-cis α-D-Galf linkage has been less studied. This xenobiotic unit has been found in several pathogenic bacteria such as Streptococcus pneumonia 22F,16 Escherichia coli O16717,18 and O85,19 Salmonella enterica O5320 and O17,19 Pragia fontium,21 and pathogenic fungi such us Paracoccidioides brasiliensis,22 and other ones.23–26 For that reason, the stereoselective methods for the synthesis of this unit would provide tools for learning about its glycobiology which has not been studied yet. However, there is no general method for α-D-galactofuranosylation as a single diastereomer.23,24 Complete stereoselectivity was first achieved by the use of the tetrabenzylated carboxylbenzyl galactofuranoside system when activated at low temperature with triflic anhydride in the synthesis of agelagalastatin for the construction of the terminal α-D-Galf-(1→2)-D-Galf linkage.27 The tetrabenzyl protection of this donor was essential for complete α-stereoselectivity over a 2-OH mannosyl acceptor.28 The 2,3-anhydrosugar methodology was also proposed for α-D-galactofuranosylation29 and the remarkable synthesis of pentasaccharide repeating unit of varianose containing internal α-D-Galf was achieved.30 However, while the glycosylation reaction was completely stereoselective, the subsequent epoxide opening was not. The intramolecular aglycon delivery (IAD) strategy was also developed for the synthesis of a 1,2-cis-α-D-fucofuranoside, a 6-deoxy analogue of α-D-galactofuranoside.31
Due to the success of conformationally restricted arabinofuranosyl donors for 1,2-cis-glycosylation, conformationally restricted 3,5-O-di-tert-butylsilylene-D-galactofuranosyl trichloroacetimidate donors have been evaluated for α-galactofuranosylation. In contrast to the arabinose counterpart, stereoselectivities have not been improved compared to the flexible 2,3,5,6-tetra-O-benzylated imidate analog when used CH2Cl2 as solvent, and surprisingly, highest α/β ratios were obtained in ethereal participating solvents.32
Recently, in connection with the use of tetrabenzylated donors for α-D-galactofuranosylation, high diastereomeric ratio (α/β ratio >9) with moderate yields was described by Lemieux-type halide ion-catalyzed glycosylation in the preparation of a α-D-Galf glycolipid analog.33 By the use of O-(2,3,5,6-tetra-O-benzyl-β-D-galactofuranosyl) trichloroacetimidate (1)34,35 as donor, disaccharide and trisaccharide alditols isolated from glycoproteins of Clostridium thermocellum and Bacteroides cellulosolvens were synthesized.35 In addition, the terminal α-D-Galf-(1→2)-L-Rha linkage in the hexasaccharide repeating unit of a rhizobacteria was also synthesized using donor 1 in 7
:
1 α/β ratio.36
The influence of the solvent has been evaluated in glycosylation reactions of trichloroacetimidate 1 with several acceptors. Interestingly, high stereoselectivity (α/β 10
:
1) has been observed in the construction of the α-D-Galf-(1→2)-L-Rha linkage using allyl 3,4-di-O-benzyl-α-L-rhamnopyranoside (2) as acceptor at −78 °C in CH2Cl2 with TMSOTf as catalyst, whereas same conditions in acetonitrile gave the β configuration as a single diasteromer.37
By taking advantage of the above result we envisioned the synthesis of the trisaccharide constituent of Streptococcus pneumoniae 22F capsular polysaccharide (CPS)16 (Fig. 1), α-D-Glcp-(1→3)-α-D-Galf-(1→2)-L-Rhap (3).
 |
| Fig. 1 Capsular polysaccharide of Streptococcus pneumoniae 22F. The target trisaccharide 3 is presented in blue. | |
S. pneumoniae is the causative agent of a variety of diseases, including bacterial sepsis, pneumonia, meningitis and otitis media, particularly affecting the elderly and young children.38,39 To date, 93 capsular serotypes have been identified, each differing in chemical structure of the capsular polysaccharide and immunogenicity.40 The polysaccharide vaccine Pneumovax (PPV-23) is not effective in children, and this was overcome by the conjugated vaccine Prevnar (PCV-7) of limited serotype coverage introduced in 2000. A dramatic decrease on invasive pneumococcal disease in children was observed,41 together with the emerge of serotypes not included in the latter one (19A, 6C, and 22F and serogroup 15).42 Up today, the development of decavalent conjugate vaccine (Synflorix) and tridecavalent conjugate vaccine (Prevnar-13) covers against more S. pneumoniae serotypes;43 however 22F serotype is not included. A recent study shows that the introduction of PCV7 resulted in an increase in non-PCV7 serotypes meningitis in England and Wales, including some not covered by the 13-valent vaccine, such as serotypes 22F and 33F.44 Pre-clinical evaluation of a 15-valent pneumococcal conjugate vaccine, which includes the 22F serotype has been performed.45
The isolation and purification of CPS could also contain bacterial contaminants.46 Synthetic well-defined oligosaccharide structures constitute valuable tools to study the immune response after conjugation.47 Many oligosaccharides constituents of various serotypes of S. pneumoniae have been previously synthesized, and some synthetic oligosaccharides-conjugated protein have been explored as potential vaccines.48 In this sense, the trisaccharide 3 constituent of S. pneumoniae 22F is an interesting target that includes also the immunogenic α-D-Galf. Kamerling et al. have reported the only example of the synthesis of an oligosaccharide belonging to this serotype, β-L-Rhap-(1→4)-α-D-Glcp-(1→3)-α-D-Galf as 3-aminopropylglycoside.49 In this case, the β-L-Rhap-(1→4)-D-Glcp disaccharide moiety was condensed to a galactofuranosyl derivative which was prepared from ethyl 1-thio-α-D-galactofuranoside,50 and the aminopropyl glycoside was obtained in moderate yield and selectivity by in situ formation of the galactofuranoside bromide.
Our target, trisaccharide 3 has the additional challenge of synthesizing an internal α-D-Galf present in its chemical structure. Moreover, these synthetic efforts would allow us to investigate the influence of the 3-O-substituent in the α-D-galactofuranosyl donor.
Results and discussion
Based in the high diastereoselectivities obtained in the construction of the α-D-Galf-(1→2)-L-Rha linkage by the use of tetra-O-benzylated galactofuranosyl imidate 1 as donor,37 the construction of trisaccharide 3 was envisioned from the reducing end to the non-reducing end (Scheme 1). Retrosynthetic analysis indicated the stereoselective introduction of a 1,2-cis α-D-Glcp unit on the galactofuranosyl 3-OH of disaccharide 4, and this could be performed by the use of 2,3,4,6-tetra-O-benzyl-D-glucopyranosyl trichloroacetimidate (5)51,52 as donor. To the best of our knowledge, the only report on the construction of this linkage α-D-Glcp-(1→3)-α-D-Galf was described by Kamerling et al.49 Disaccharide 4 could be synthesized by glycosylation of known allyl 3,4-di-O-benzyl-α-L-rhamnopyranoside53 (2) with the galactofuranosyl trichloroacetimidate donor 6 carrying an orthogonal group at O-3. The p-methoxybenzyl (PMB) group was selected first as a closely related analogue of the benzyl group. Moreover, the influence of this substitution in the glycosylation reaction could be also a matter of study. On the other hand, the allyl moiety of rhamnoside acceptor 2 would allow functionalization of the trisaccharide for further conjugation to an immunogenic carrier protein.
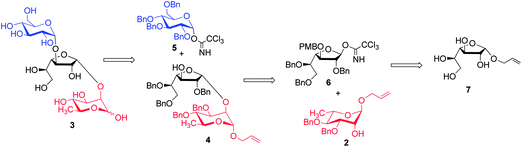 |
| Scheme 1 Retrosynthesis of trisaccharide 3. | |
The synthesis of internal Galf-containing oligosaccharides implies a first choice of the galactofuranosyl precursors.23–25,54,55 In this case, the trichloroacetimidate method of glycosylation was the option for stereoselective galactofuranosylation over the rhamnosyl acceptor 2. For that reason, and based in our previous experience in anomeric O-alkylation,32,37,54 allyl α-D-galactofuranoside56 (7) was chosen as a convenient starting material. Selective deprotection of the allyl group would allow further activation of the anomeric center.
Synthesis of the galactofuranosyl donor 6
Starting from 7, the 5- and 6-O positions were protected by reaction with dimethoxypropane in acetone catalyzed by p-toluensulfonic acid to give the 5,6-O-isopropylidene derivative 8 in 96% yield (Scheme 2). The next step was the selective protection on OH-2 of 8 by a silylether, and triisopropylsilyl group (TIPS) was selected in order to avoid silyl migration or deprotection in the following benzylation step.57 Treatment of 8 with 1.3 equiv. of triisopropylsilylchloride in DMF and imidazol gave the 2-O-TIPS derivative 9 in 85% yield as the only product. The regioselectivity was confirmed by the correlation of OH-3 (δ 2.02) with H-3 (δ 4.13) in the 1H NMR and COSY spectra, and could be attributed to the nucleophilicity of OH-2 increased by intramolecular hydrogen bond with the α-anomeric oxygen.31,54 Same regioselectivity was observed in the α-benzyl analog of 8 by treatment with tert-butyldiphenylsilyl chloride (1.25 equiv.) to yield the 2-O-derivative as the only product.54 However, silylation with TBDPCl of the 1-β-thiotolyl analog of 8 gave a separable mixture of 2-O- and 3-O-derivatives in 5
:
1 ratio,58 whereas silylation with TBDMCl of the β 2′-(benzyloxycarbonyl)benzyl (BCB) analog of 8 gave the corresponding 2-O- and 3-O-derivatives in 10
:
1 ratio.27 In this sense, allyl or benzyl α-galactofuranoside are important D-Galf precursors because complete regioselectivity protection could be achieved.
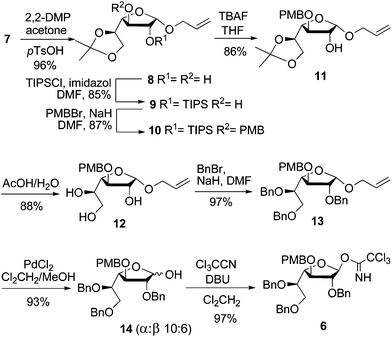 |
| Scheme 2 Synthesis of donor 6. | |
The p-methoxybenzylation was performed by addition of NaH over a solution of 9 in DMF and followed by a rapid addition of p-methoxybenzyl bromide to give 10 in 92% yield, as confirmed by downfield shift (6.8 ppm) of the C-3 in the 13C NMR spectrum compared to the same signal in 9. With the aim of benzylate the O-2, O-5, and O-6 positions of the galactofuranosyl precursor, deprotection of silyl group followed by isopropylidene hydrolysis was performed based on their straightforward purification of the intermediate. Treatment of 2-O-TIPS derivative 10 with TBAF in THF gave 11 (86%). Hydrolysis of isopropylidene group of 11 was performed by treatment with 66% v/v AcOH in H2O at 60 °C and carefully quenching with pyridine until pH 6 to afford 12 in 88% yield. The 13C NMR spectrum showed that the signal of C-3 appeared downfield shifted at 82.8 ppm and correlated (HSQC) with the H-3 at δ 4.06 confirming the position of the PMB group. Standard benzylation of 12 with benzyl bromide and NaH in DMF gave 13 in 97% yield as a single product. In order to activate the anomeric center via the trichloroacetimidate method, the allyl group was removed by treatment with PdCl2 in a mixture of CH2Cl2–MeOH protected from light to give 14. The H-1β appeared as a broad singlet (δ 5.41) whereas the H-1α appeared as a doublet of doublet (δ 5.26) with the typical J1,2 of 4.5 Hz and a second coupling constant due to H-1 and OH-1 coupling. The α/β ratio of the anomeric mixture was established as 5
:
3 as indicated by integration of the anomeric protons. In the 13C NMR spectrum, the C-1α and C-1β appeared at 96.2 and 100.9 ppm, respectively. Treatment of 14 with trichloroacetonitrile in dichloromethane with DBU as a base gave the β-trichloroacetimidate 6 as the only isomer in 97% yield, as indicated by the broad singlet of the only anomeric H-1 signal at δ 6.37 in 1H NMR spectrum. The 1H and 13C NMR spectra of 6 were almost similar to those from the fully benzylated analogue 1.35
Once donor 6 was at hand, the next step was the evaluation of rhamnosyl acceptor 2 using the glycosylation conditions that favored the α product in α/β 10
:
1 ratio. A direct procedure was employed, to a mixture of acceptor 2 and trichloroacetimidate 6 in CH2Cl2 at −78 °C, TMSOTf (0.3 equiv.) as catalyst was added (Method A).37 Surprisingly, the target α-disaccharide was not detected even as a minor product. Instead, the β-product of glycosylation lacking the PMB group, allyl 2,5,6-tri-O-benzyl-β-D-galactofuranosyl-(1→2)-3,4-di-O-benzyl-α-L-rhamnopyranoside (15) was isolated (24%, Scheme 3). The β configuration of 15 was confirmed by the small J1,2 (1.4 Hz) that appeared in the corresponding 1H NMR spectrum as well as the resonance for C-1′ at δ 107.8 in the 13C NMR spectrum. The Chapman-like rearrangement by-product without the PMB group, 2,5,6-tri-O-benzyl-N-trichloroacetyl-α-D-galactofuranosylamine (16) was also obtained in 36% yield. A third compound was detected in 18% yield and was identified as 2,5,6-tri-O-benzyl-β-D-galactofuranosyl-(1→3)-2,5,6-tri-O-benzyl-N-trichloroacetyl-α-D-galactofuranosylamine (17, Scheme 3). Compound 17 is a glycosylation product of transposition by-product 16 as acceptor with imidate 6 with or without PMB as donor.
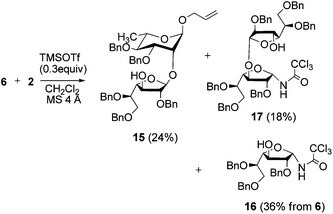 |
| Scheme 3 Glycosylation of 2 with 6 using Method A. | |
In summary, PMB group did not resist TMSOTf as Lewis acid. For that reason, the catalyst employed was reduced to 0.05 equiv. (Method B, Table 1, entry 1) and, in this case, the desire α-disaccharide allyl 2,5,6-tri-O-benzyl-3-O-(4-methoxybenzyl)-α-D-galactofuranosyl-(1→2)-3,4-di-O-benzyl-α-L-rhamnopyranoside (18) was obtained as the only glycosylation product in 41% yield, as indicated by the H-1 signal in the 1H NMR spectrum which appeared as a doublet with J1,2 4.2 Hz characteristic of the 1,2-cis configuration. Neither β-disaccharide nor other glycosylation products without the PMB group were detected at all. In contrast, the use of higher amount of TMSOTf (Method A) afforded only β-disaccharides but without PMB group. A question arises whether the absence of a PMB group or the presence of the OH in the donor could influence on the stereoselectivity of glycosylation. The transposition by-product 2,5,6-tri-O-benzyl-3-O-(4-methoxybenzyl)-N-trichloroacetyl-α-D-galactofuranosylamine (19) was also isolated from the reaction mixture in 42% yield with α-configuration confirmed by NOESY experiment (Table 1, entry 1). The 1H NMR spectrum of 19 was similar to that from the fully benzylated analogue.35,37 Lowering the amount of the TMSOTf avoided the removal of the PMB group; however, the yield of the glycosylation was moderate with high amount of transposition by-product (Table 1, entry 1). Highly armed donors and poor nucleophilic acceptors usually favor the rearrangements of the imidate, which are avoided employing the inverse procedure of glycosylation, i.e. addition of the donor to an acceptor/catalyst solution.59,60 Thus, slow addition of the donor 6 to a mixture containing acceptor 2, TMSOTf (0.05 equiv.) and molecular sieves at −78 °C (Method C), afforded 18 in better yield (51%) reducing the amount of the by-product 19 to 18% (Table 1, entry 2). Once again, no β-glycosylation product was detected.
Table 1 Glycosylation of acceptors 2 and 20 with imidates 6, 24–27. (B) Direct method (0.05 equiv. TMSOTf). (C) Inverse method (0.05 equiv. TMSOTf)
Influence of the protecting groups in rhamnoside acceptor
Previous results indicated that best selectivities on α-D-galactofuranosylation employing tetrabenzylated imidate 1 were obtained with benzoylated mannopyranosides over benzylated analogues.37 Moreover, acyl-protective groups on glycosyl acceptors were essential for the 1,2-cis β-stereoselectivity using tri-O-benzyl carboxylbenzyl D-arabinofuranoside as donor.61 Our next step was the evaluation of the protecting group pattern in rhamnoside acceptor, by replacing the benzyl groups of 2 by benzoyl groups. Therefore, with the use of the inverse procedure (Method C), donor 6 reacted with allyl 3,4-di-O-benzoyl-α-L-rhamnopyranoside62 (20) and, in this case, the α-product 21α was obtained instead in 36% yield (Table 1, entry 3). The β-glycosylation product 21β was also isolated in 11% yield. The by-product 19 was also obtained in 21% yield. The α/β ratio of glycosylation products was 3.3
:
1 in contrast to the complete selectivity toward the α anomer obtained with benzylated rhamnoside 2.
Construction of the α-D-Glcp-(1→3)-α-D-Galf linkage
Having determined the benzylated rhamnosyl acceptor 2 for complete α-selectivity, our attention was focused on the construction of the α-D-Glcp-(1→3)-α-D-Galf linkage. Kamerling et al. built this linkage by glycosylation of α-D-Galf acceptor with disaccharide imidate of L-Rhap-(1→4)-D-Glcp by a direct method of glycosylation employing ethyl ether as solvent at −30 °C, with 45% yield.49 Considering the α-configuration of D-Galf in the trisaccharide 3, we used allyl 2,5,6-tri-O-benzyl-α-D-galactofuranoside (22) as simple model, which was obtained quantitatively by treatment of 13 with TFA in CH2Cl2 at 0 °C (Scheme 5). Ethyl ether was employed as the solvent required for α-glucopyranosylation60 with TMSOTf (0.3 equiv.) as catalyst, at −78 °C using the inverse procedure by slow addition of glucopyranosyl donor 5 to the reaction mixture containing 22 (Scheme 4). Under these conditions, the desired α-disaccharide allyl 2,3,4,6-tetra-O-benzyl-α-D-glucopyranosyl-(1→3)-2,5,6-tri-O-benzyl-α-D-galactofuranoside (23) was obtained as a single diastereomer in very good yield (91%). In the 1H NMR spectrum, the H-1′ appeared as a doublet (δ 5.17) with J1,2 3.5 Hz indicating the α-configuration of the new glycosidic linkage. In the 13C NMR spectrum, the anomeric region showed two signals at 98.3 and 95.8 ppm corresponding to α-D-Galf C-1′ and α-D-Glcp C-1, respectively.
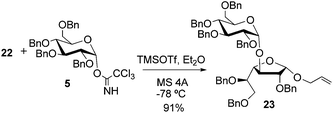 |
| Scheme 4 Glycosylation of acceptor 22 with imidate 5. | |
Influence of the O-3 substitution of the donor in α-D-Galf-(1→2)-L-Rhap linkage construction
At this point, the synthesis of α-D-Galf-(1→2)-L-Rhap was completely stereoselective but still the yield was moderate (51%). For that reason, we decided to study the influence of the substituent at O-3 of the galactofuranosyl imidate donor to improve the yield maintaining the high stereoselectivity. The 3-OH position should be protected by an orthogonal group to the benzyl and allyl groups present in the galactofuranosyl and rhamnosyl derivatives, as discussed in the retrosynthetic analysis (Scheme 1). The first protecting group of choice was the electron withdrawing benzoyl group, which would disarm the donor 24 (Scheme 5) comparing to the PMB analogue 6. On the other hand, electronic effects or long distance assistance or remote participation63–65 could provide control on the stereoselectivity of the reaction favoring the α-anomer (Fig. 2). Pentafluorobenzoyl group (PFBz) was also elected as more electron withdrawing group than benzoyl, which would limit the remote participation because of the lesser electronic density of the carbonyl, but would exert a marked electronic effect by disarming the donor 25 during the glycosylation reaction.66,67 A lesser withdrawing group than benzoyl as 4-methoxybenzoyl group (PMBz) was also chosen in 26 and, in this case, it would favor the remote participation due to higher electronic density at the carbonyl oxygen.68 In order to complete the scope of protecting groups, we decide to evaluate an electron donor group such a silyl group, and in contrast to the benzyl and PMB group, the bulky TIPS in 27 would also exert a steric hindrance over the β-face.
 |
| Scheme 5 Synthesis of donors 24–27. Conditions: (a) (i) BzCl, C5H5N for 28; (ii) PFBzCl, C5H5N for 29; (iii) PMBzCl, C5H5N for 30; (iv) TIPSCl, imidazol, DMF for 31. | |
 |
| Fig. 2 Dioxocarbenium ion as possible intermediate in remote participation. | |
Donors 24–27 were synthesized from 22 as indicated in Scheme 5 (see ESI†). In all cases, only the β anomer was obtained. Glycosylation reactions were evaluated using benzylated rhamnosyl acceptor 2. The inverse procedure of glycosylation using 0.05 equiv. of TMSOTf was employed (Method C) since the best yield with complete diastereoselectivity was obtained with 3-O-PMB donor 6. The results are indicated in Table 1 (entries 4–7). The best result was obtained with benzoylated 24. In this case, the corresponding disaccharide 36 was obtained with excellent yield (92%) with complete diastereoselectivity. Interestingly, the transposition by-product trichloroacetamide 37 was detected in very low yield (3%). PFBz derivative 25 gave the α-disaccharide 38 as the only glycosylation product, but the yield lowered to 75% with no transposition by-product detected. On the other hand, the PMBz derivative 26 gave the corresponding disaccharide 39 in good yield (67%) but, unfortunately, the trichloroacetamide by-product 40 was obtained in 28% yield from imidate 26. The use of TIPS donor 27 afforded the disaccharide 41 with complete diastereoselectivity as well, and a yield (57%) comparable to that obtained with the PMB donor 6. Unexpectedly, the 2,5,6-tri-O-benzyl-3-O-triisopropylsilyl-α-D-galactofuranosyl-(1→1)-2,5,6-tri-O-benzyl-3-O-triisopropylsilyl-α-D-galactofuranoside (42, Fig. 3) was obtained with 16% yield together with a small amount of the trichloroacetamide 43 (3%). Interestingly, 42 exhibited both linkages as 1,2-cis α. Neither α,β nor β,β analogues were detected. A question arises about the stereochemistry of this by-product. If any hydrolysis of the imidate 27 were occurred first, a mixture of α,β anomeric acceptors 35 would have been expected, with the β anomer as a major isomer to give some β-glycosylation product, which is absent. However, the α-anomer could be produced kinetically, or could be more nucleophilic to give only the α,α product. These aspects remain unclear.
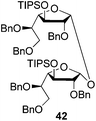 |
| Fig. 3 Structure of by-product 42. | |
Focusing on the influence of the protecting groups, only the α-anomer has been detected in all cases, all glycosylation were complete stereoselective. However, the better yields were obtained with acyl groups protection, being the benzoyl group the most effective (92%). Long distance participation cannot be discarded nor confirmed as the more electron-rich carbonyl oxygen of the PMBz group did not increase the yield of glycosylation compared to that of the Bz analogue. However, the acyl protection is related to the yield of the transposition by-product. Whereas no transposition by-product was detected with the less armed PFBz donor 25, this by-product was increased to 28% using PMBz donor 26.
Synthesis of trisaccharide 3
A complete diastereoselective α-galactofuranosylation was achieved with excellent yield, removal of benzoyl group of 36 with sodium methoxide afforded disaccharide 4 in 74% yield (Scheme 6). Glycosylation of 4 with glucopyranosyl donor 5, using the inverse procedure of glycosylation (Method D), proved for the simple model 23, gave trisaccharide 44 with complete diastereoselectivity and quantitative yields. The signals of 1H NMR spectrum were fully assigned by the use of COSY and HSQC experiments. In the 13C NMR spectrum, the C-1′ of the α-D-Galf moiety appeared at 98.5 ppm. The C-1′′ of the α-D-Glcp moiety appeared at 95.4 ppm similar to that observed for simple model 23, indicating the α-configuration for the new glycosidic linkage. On the other hand, the C-1 and C-6 of the Rha moiety appeared at 96.8 and 17.9 ppm, respectively. In the 1H NMR spectrum, the H-1′′ of the new glycosidic linkage appeared superimposed to the quartet of the allyl moiety, however a doublet with J1,2 of 3.5 Hz could be distinguished confirming the α-configuration.
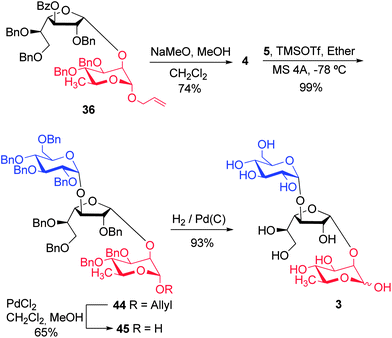 |
| Scheme 6 Synthesis of trisaccharide 3. | |
Trisaccharide 44 was further deprotected. Treatment with PdCl2 in CH2Cl2–methanol gave the anomeric free trisaccharide 45 in 10
:
7 α/β anomeric mixture as indicated by the integration of the H-1′ of the D-Galf moiety of the α and β anomers at δ 5.25 and 5.27, respectively in the 1H NMR spectrum. Hydrogenolysis of 45 with H2 and Pd(C) gave the target fully unprotected trisaccharide 3. The anomeric hydrogens appeared well resolved as shown in the 1H NMR spectrum which could be assigned through COSY and HSQC experiments.
Conclusions
The synthesis of the novel trisaccharide α-D-Glcp-(1→3)-α-D-Galf-(1→2)-L-Rhap (3) constituent of the capsular polysaccharide of Streptococcus pneumonia 22F was performed with complete diastereoselectivity. The robust trichloroacetimidate method was employed for the glycosylation steps. Allyl α-D-galactofuranoside, synthesized in one step from galactose, was used as novel precursor of the internal Galf for the first time, allowing the introduction of an orthogonal group at O-3. From the analysis on the influence of the 3-O-substituent (PMB, Bz, PFBz, PMBz, TIPS) in benzylated galactofuranosyl donors in order to built the α-D-Galf-(1→2)-α-L-Rhap linkage, we can conclude that all galactofuranosyl donors gave complete stereoselectivity with acceptor 2; however, the substitution pattern had a strong effect on glycosylation yields. Excellent yield was obtained employing 3-O-benzoyl galactofuranosyl donor. Although remote participation effect is not conclusive, 3-O-acyl galactofuranosyl donors gave better yields of glycosylation products with lesser amount of trichloroacetamide by-products. A stabilization of the oxonium ion by the 3-O-benzoyl group could be involved. On the other hand, benzyl protection on rhamnoside acceptor was a requirement for complete stereoselectivity. Moreover, a high yield and complete stereoselective construction of α-D-Glcp-(1→3)-α-D-Galf linkage was achieved by the use of the inverse procedure of glycosylation at low temperature (−78 °C) in ethyl ether as solvent.
In summary, the present work has brought new insight to the 1,2-cis-galactofuranosylation field as well as allowed the synthesis of 3, which is the first example of a synthesis of an internal 1,2-cis α-D-Galf-containing oligosaccharide of a pathogen microorganism. Moreover, allyl glycoside 44 or anomeric free 45 could be useful precursors of oligosaccharides conjugates for immunogenic studies and vaccine developments.
Experimental
General
TLC was performed on 0.2 mm silica gel 60 F254 aluminium supported plates and developed in the solvent system indicated. When TEA was added to the solvent system, TLC plate required a pretreatment with the elution solvent to reduce its acidic nature thereof. Detection was effected by exposure to UV light or by spraying with 10% (v/v) sulfuric acid in EtOH and charring. Column chromatography was performed on silica gel 60 (230–400 mesh). Melting points are uncorrected. NMR spectra were recorded with a Bruker AVANCE II 500 spectrometer at 500 MHz (1H) and 125.8 MHz (13C) or with a Bruker AC 200 at 200 MHz (1H) and 50.3 MHz (13C). Chemical shifts are given relative to the signal of internal acetone standard at 2.22 and 30.89 ppm for 1H NMR and 13C NMR spectra, respectively when recorded in D2O. 1H and 13C assignments were supported by 2D COSY, and HSQC experiments. NOESY experiment was performed when indicated. High resolution mass spectra (HRMS) were recorded on a BRUKER micrOTOF-Q II spectrometer. Optical rotations were measured with a Perkin-Elmer 343 polarimeter with a path length of 1 dm at 25 °C. Sugars were dried under vacuum (2 × 10−3 mbar) for 6 hs at room temperature.
Allyl 5,6-O-isopropylidene-α-D-galactofuranoside (8). To a solution of dried allyl α-D-galactofuranoside56 (7, 1.77 g, 8.19 mmol) in 5
:
1 acetone-2,2 dimethoxypropane (20.4 mL) cooled to 0 °C, was added p-toluensulfonic acid (5 mg). After stirring for 30 min at room temperature, the mixture was neutralized with concentrated ammonia, and concentrated under reduced pressure. Purification of the residue by silica gel column chromatography (3
:
2 hexane–EtOAc) gave 8 (2.01 g, 96%) as a colorless syrup which crystallized from 10
:
1 hexane–EtOAc: mp 58–59 °C; Rf 0.51 (1
:
1 hexane–EtOAc); [α]D +85.8 (c 1.1, CHCl3); 1H NMR (CDCl3, 500 MHz) δ 5.90 (dddd, 1H, J = 17.0, 10.5, 6.0, 5.7 Hz, CH
CH2), 5.29 (dd, 1H, J = 17.0, 1.4 Hz, HC
CHaH), 5.21 (d, 1H, J = 10.5 Hz, HC
CHbH), 5.00 (d, 1H, J = 4.4 Hz, H-1), 4.33 (dd, 1H, J = 12.8, 5.7 Hz, OCHaH-CH
), 4.21 (apparent q, 1H, J = 6.6 Hz, H-5), 4.10–4.04 (m, 3H, OCHbH–CH
, H-2, H-3), 4.03 (dd, 1H, J = 8.4, 6.7 Hz, H-6a), 3.93 (dd, 1H, J = 8.4, 7.0 Hz, H-6b), 3.85 (t, 1H, J = 6.7 Hz, H-4), 2.57 (d, 1H, J = 9.8 Hz, OH-3), 2.50 (d, 1H, J = 3.00 Hz, OH-2); 1.45, 1.38 (2s, 6H, (CH3)2C)); 13C NMR (CDCl3, 125 MHz) δ 133.6 (CH
CH2), 117.8 (CH
CH2), 109.7 ((CH3)2C), 99.9 (C-1), 81.4 (C-4), 78.1 (C-2), 77.3 (C-3), 76.6 (C-5), 68.8 (OCH2–CH
), 64.9 (C-6); 26.5, 25.3 ((CH3)2C); HRMS (ESI) calcd for (M + Na) C12H20O6Na: 283.1152. Found: 283.1153.
Allyl 5,6-O-isopropylidene-2-O-triisopropylsilyl-α-D-galactofuranoside (9). To a stirred solution of dry 8 (1.01 g, 3.88 mmol) and imidazol (669 mg, 9.83 mmol) in anh DMF (10 mL), cooled to 0 °C, triisopropylsilyl chloride (1.07 mL, 5.04 mmol) was slowly added. After stirring for 18 h at room temperature, the mixture was diluted with CH2Cl2 (50 mL), washed with water (5 × 60 mL), dried (Na2SO4), filtered and concentrated under reduced pressure. Purification of the residue on silica gel column chromatography (15
:
1 hexane–EtOAc) gave 9 (1.38 g, 85%) as needles (mp 41–42 °C); Rf 0.61 (2
:
1 hexane–EtOAc); [α]D +69.7 (c 0.9, CHCl3); 1H NMR (CDCl3, 500 MHz) δ 5.91 (dddd, 1H, J = 17.2, 10.4, 6.1, 5.4 Hz, CH
CH2), 5.30 (dq, 1H, J = 17.2, 1.6 Hz, HC
CHaH), 5.16 (apparent dq, 1H, J = 10.4, 1.4 Hz, HC
CHbH), 4.85 (d, 1H, J = 4.0 Hz, H-1), 4.28 (ddt, 1H, J = 12.7, 5.4, 1.4 Hz, OCHaH-CH
),4.21 (apparent q, 1H, J = 7.0 Hz, H-5), 4.18 (dd, 1H, J = 7.8, 4.2 Hz, H-2), 4.15 (dt, 1H, J = 7.4, 4.0 Hz, H-3), 4.01 (dd, 1H, J = 8.5, 6.6 Hz, H-6a), 3.99 (dt, 1H, J = 12.7, 6.1, 1.4 Hz, OCHbH-CH
), 3.92 (dd, 1H, J = 8.5, 7.0 Hz, H-6b), 3.82 (t, 1H, J = 7.2 Hz, H-4), 2.02 (d, 1H, J = 4.0 Hz, OH-3), 1.45, 1.37 (2s, 6H, (CH3)2C), 1.16–1.04 (m, 21H, (CH3)2CH)3Si); 13C NMR (CDCl3, 125 MHz) δ 134.1 (CH
CH2), 117.3 (CH
CH2), 109.6 ((CH3)2C), 100.8 (C-1), 81.3 (C-4), 78.6 (C-2), 78.1 (C-5), 76.4 (C-3), 68.5 (OCH2–CH
), 64.9 (C-6); 26.5, 25.2 ((CH3)2C), 17.8 ((CH3)2CH)3Si, 12.2 ((CH3)2CH)3Si; HRMS (ESI) calcd for (M + Na) C21H40O6SiNa: 439.2486. Found: 439.2488.
Allyl 5,6-O-isopropylidene-3-O-(4-methoxybenzyl)-2-O-triisopropylsilyl-α-D-galactofuranoside (10). To a solution of dry 9 (1.30 g, 3.13 mmol) in anh DMF (15 mL) cooled to 0 °C, was added NaH (dispersion in oil 60%, 175 mg, 4.38 mmol) under argon atmosphere. p-Methoxybenzyl bromide (0.63 mL, 4.38 mmol) was added immediately after to avoid silyl migration. After stirring for 1.5 h at 0 °C, the mixture was diluted with CH2Cl2 (120 mL), sequentially washed with brine (4 × 120 mL), H2O (1× 120 mL), dried (Na2SO4), filtered and concentrated. Silica gel column chromatography (toluene; then 40
:
1 toluene–EtOAc) of the residue afforded 10 (1.57 g, 92%) as a colorless syrup: Rf 0.45 (10
:
1 toluene–EtOAc); [α]D +38.4 (c 1.3, CHCl3); 1H NMR (CDCl3, 500 MHz) δ 7.24 (d, 2H, J = 8.7 Hz, PMBn), 6.88 (d, 2H, J = 8.7 Hz, PMBn), 5.94 (dddd, 1H, J = 17.2, 10.4, 6.1, 5.6 Hz, CH
CH2), 5.30 (dq, 1H, J = 17.2, 1.6 Hz, HC
CHaH), 5.17 (dq, 1H, J = 10.4, 1.3 Hz, HC
CHbH), 4.86 (d, 1H, J = 4.3 Hz, H-1); 4.77, 4.51 (2d, 2H, J = 11.0 Hz, pMPhCH2), 4.40 (dd, 1H, J = 4.3, 7.1 Hz, H-2), 4.31 (ddt, 1H, J = 12.5, 5.6, 1.4 Hz, OCHaH-CH
), 4.13 (dt, 1H, J = 6.4, 7.7 Hz, H-5), 3.99 (t, 1H, J = 6.8 Hz, H-3), 3.97 (ddt, 1H, J = 12.5, 6.1, 1.4 Hz, OCHbH-CH
), 3.85 (dd, 1H, J = 7.9, 6.6 Hz, H-4), 3.80 (s, 3H, OCH3), 3.78 (dd, 1H, J = 8.2, 6.4 Hz, H-6a), 3.70 (dd, 1H, J = 8.2, 7.8 Hz, H-6b), 1.41, 1.34 (2s, 6H, (CH3)2C), 1.12–1.10 (m, 21H, (CH3)2CH)3Si); 13C NMR (CDCl3, 125 MHz) δ 159.3 (PMBn), 134.1 (CH
CH2); 129.9, 129.7 (PMBn); 117.4 (CH
CH2), 113.8 (PMBn), 109.4 ((CH3)2C), 101.3 (C-1), 83.2, 81.0, 79.1, 78.4, 72.6 (PMBn), 68.5 (OCH2–CH
), 65.2 (C-6), 55.3 (OCH3); 26.7, 25.5 ((CH3)2C); 18.0, 17.9 ((CH3)2CH)3Si); 12.4 ((CH3)2CH)3Si); HRMS (ESI) calcd for (M + Na) C29H48O7SiNa: 559.3062. Found: 559.3076.
Allyl 5,6-O-isopropylidene-3-O-(4-methoxybenzyl)-α-D-galactofuranoside (11). A freshly prepared solution of TBAF (1.93 g, 7.38 mmol) in anhyd THF (38 mL) cooled to 0 °C was added to 10 (1.99 g, 3.71 mmol) with stirring. After 1 h, TLC examination showed disappearance of the starting material and the solution was diluted with CH2Cl2 (150 mL), washed with H2O (4 × 100 mL), dried (Na2SO4), filtered and concentrated. The residue was purified by silica gel column chromatography (20
:
1 and then 6
:
1 hexane–EtOAc) to afford syrupy 11 (1.21 g, 86%). Rf 0.34 (2
:
1 hexane–EtOAc); [α]D +24.0 (c 1.0, CHCl3); 1H NMR (CDCl3, 500 MHz) δ 7.28 (d, 2H, J = 8.8 Hz, PMBn), 6.88 (d, 2H, J = 8.8 Hz, PMBn), 5.91 (dddd, 1H, J = 17.2, 10.4, 6.2, 5.4 Hz, CH
CH2), 5.29 (dq, 1H, J = 17.2, 1.6 Hz, HC
CHaH), 5.21 (dq, 1H, J = 10.4, 1.3 Hz, HC
CHbH), 5.03 (d, 1H, J = 4.8 Hz, H-1), 4.79, 4.56 (2d, 2H, J = 11.4 Hz, pMPhCH2), 4.35 (ddt, 1H, J = 12.8, 5.4, 1.4 Hz, OCHaH–CH
), 4.27 (dd, 1H, J = 6.2, 4.8 Hz, H-2), 4.09 (dt, 1H, J = 7.5, 6.4 Hz, H-5), 4.08 (ddt, 1H, J = 12.8, 6.2, 1.4 Hz, OCHbH–CH
), 3.87 (dd, 1H, J = 7.3, 6.4 Hz, H-4), 3.84 (dd, 1H, J = 8.3, 6.4 Hz, H-6a), 3.81 (s, 3H, OCH3), 3.77 (t, 1H, J = 6.3 Hz, H-3), 3.75 (dd, 1H, J = 8.3, 7.5 Hz, H-6b), 2.64 (bs, 1H, OH); 1.42, 1.36 (2s, 6H, (CH3)2C); 13C NMR (CDCl3, 125 MHz) δ 159.3 (PMBn), 133.6 (CH
CH2); 129.7, 129.6 (PMBn); 117.8 (CH
CH2), 113.8 (PMBn), 100.8 (C-1), 83.0 (C-3), 81.9 (C-4), 78.12 (C-5), 78.07 (C-2), 71.3 (PMBn), 68.9 (OCH2–CH
), 65.1 (C-6), 55.2 (OCH3); 26.6, 25.5 ((CH3)2C); HRMS (ESI) calcd for (M + Na) C20H28O7Na: 403.1727. Found: 403.1739.
Allyl 3-O-(4-methoxybenzyl)-α-D-galactofuranoside (12). A solution of 11 (1.21 g, 3.18 mmol) in 66% v/v AcOH in H2O (23 mL) was warmed to 60 °C with stirring. After 30 min, TLC examination revealed disappearance of starting material, and the solution was cooled to 0 °C. Pyridine (21 mL) was slowly added until pH = 6 to avoid p-methoxybenzyl removal. The mixture was concentrated and the residue was co-evaporated with toluene (5 × 5 mL). Column chromatography of the residue (6
:
4 hexane–EtOAc) afforded 12 (940 mg, 88%) as a colorless syrup which crystallized from 1
:
1 hexane–EtOAc: mp 74–75 °C; Rf 0.52 (EtOAc); [α]D +35.4 (c 0.9, CHCl3); 1H NMR (CDCl3, 500 MHz) δ 7.28 (d, 2H, J = 8.7 Hz, PMBn), 6.88 (d, 2H, J = 8.7 Hz, PMBn), 5.92 (dddd, 1H, J = 17.2, 10.4, 6.1, 5.7 Hz, CH
CH2), 5.32 (dq, 1H, J = 17.2, 1.5 Hz, HC
CHaH), 5.26 (dq, 1H, J = 10.4, 1.3 Hz, HC
CHbH), 5.03 (d, 1H, J = 4.9 Hz, H-1); 4.78, 4.56 (2d, 2H, J = 11.3 Hz, PMPhCH2), 4.29 (ddt, 1H, J = 12.8, 5.7, 1.4 Hz, OCHaH–CH
), 4.27 (dd, 1H, J = 6.4, 4.9 Hz, H-2), 4.13 (ddt, 1H, J = 12.8, 6.1, 1.4 Hz, OCHbH–CH
), 4.06 (t, 1H, J = 6.2 Hz, H-3), 4.00 (dd, 1H, J = 6.2, 3.9 Hz, H-4), 3.80 (s, 3H, OCH3), 3.66–3.60 (m, 3H, H-5, H-6a, H-6b), 2.47 (bs, 3H, OH); 13C NMR (CDCl3, 125 MHz) δ 159.4 (PMBn), 133.2 (CH
CH2); 129.61, 129.57 (PMBn); 118.6 (CH
CH2), 113.9 (PMBn), 101.2 (C-1), 82.8 (C-3), 82.7 (C-4), 77.8 (C-2), 72.1 (C-5), 71.7 (PMBn), 69.9 (OCH2–CH
), 64.2 (C-6), 55.2 (OCH3). HRMS (ESI) calcd for (M + Na) C17H24O7Na: 363.1414. Found: 363.1423.
Allyl 2,5,6-tri-O-benzyl-3-O-(4-methoxybenzyl)-α-D-galactofuranoside (13). To a solution of 12 (940 mg, 2.76 mmol) in anhyd DMF (11.8 mL), cooled to 0 °C, was added NaH (dispersion in oil 60%, 441 mg, 11.0 mmol) followed by BnBr (1.32 mL, 11.0 mmol) with stirring, under an argon atmosphere. The mixture was stirred for 1 h at 0 °C and for 1.5 h at room temperature. After cooling the mixture to 0 °C, MeOH (1.5 mL) was slowly added and the stirring continued for 10 min. The mixture was diluted with CH2Cl2 (100 mL), washed with brine (4 × 100 mL) until pH 7 and H2O (1 × 100 mL), dried (Na2SO4), filtered and concentrated. Column chromatography of the residue (hexane, then 9
:
1 hexane–EtOAc) afforded 13 (1.65 g, 97%) as a colorless syrup: Rf 0.44 (3
:
1 hexane–EtOAc); [α]D +28.5 (c 0.9, CHCl3); 1H NMR (CDCl3, 500 MHz) δ 7.40–7.21 (m, 15H, Bn), 7.15 (d, 2H, J = 8.7 Hz, PMBn), 6.81 (d, 2H, J = 8.7 Hz, PMBn), 5.86 (dddd, 1H, J = 17.1, 10.3, 6.6, 5.0 Hz, CH
CH2), 5.25 (dq, 1H, J = 17.1, 1.6 Hz, HC
CHaH), 5.16 (dq, 1H, J = 10.4, 1.4 Hz, HC
CHbH), 4.91 (d, 1H, J = 4.3 Hz, H-1), 4.72, 4.58 (2d, 2H, J = 11.7 Hz, PhCH2), 4.66, 4.57 (2d, 2H, J = 11.6 Hz, PhCH2), 4.65, 4.44 (2d, 2H, J = 11.2 Hz, PMBn), 4.49, 4.44 (2d, 2H, J = 12.1 Hz, PhCH2), 4.28 (t, 1H, J = 7.3 Hz, H-3), 4.15 (ddt, 1H, J = 13.0, 5.0, 1.5 Hz, OCHaH–CH
), 4.05 (dd, 1H, J = 4.3, 7.6 Hz, H-2), 3.94 (dd, 1H, J = 7.0, 6.2 Hz, H-4), 3.91 (ddt, 1H, J = 13.0, 6.6, 1.3 Hz, OCHbH–CH
), 3.76 (s, 3H, OCH3), 3.68 (dt, 1H, J = 6.3, 4.0 Hz, H-5), 3.61 (dd, 1H, J = 10.4, 4.0 Hz, H-6a), 3.55 (dd, 1H, J = 10.4, 6.4 Hz, H-6b); 13C NMR (CDCl3, 125 MHz) δ 159.2 (PMBn); 138.8, 138.3, 137.7 (Bn); 134.2 (CH
CH2); 130.2, 129.6 (PMBn); 128.4, 128.3, 128.2, 128.1, 127.9, 127.7, 127.49, 127.47, 127.3 (Bn); 117.5 (CH
CH2), 113.7 (PMBn), 98.6 (C-1), 84.2 (C-2), 80.4 (C-3), 80.3 (C-4), 79.5 (C-5); 73.3, 73.0, 72.4, 71.8 (PhCH2, PMPhCH2); 70.4 (C-6), 68.0 (OCH2–CH
), 55.2 (OCH3). HRMS (ESI) calcd for (M + Na) C38H42O7Na: 633.2823. Found: 633.2840.
2,5,6-Tri-O-benzyl-3-O-(4-methoxybenzyl)-D-galactofuranose (14). To a solution of 13 (165 mg, 0.27 mmol) in CH2Cl2 (2.5 mL), was added a suspension of PdCl2 (8.7 mg, 0.049 mmol) in MeOH (3.8 mL), and the mixture was stirred at room temperature covered from light exposure. After 2 h, the mixture was concentrated to dryness at room temperature to give a residue which was purified immediately after by silica gel column chromatography (12
:
1 toluene–EtOAc) to afford 14 (143 mg, 93%) as a colorless syrup; Rf 0.34 (5
:
1 toluene–EtOAc); [α]D −14.4 (c 1.4, CHCl3); 1H NMR (CDCl3, 500 MHz) δ 7.38–7.23 (m, 15H, ArH), 7.16 (d, 1.26H, J = 8.7 Hz, PMBn α anomer), 7.13 (d, 0.74H, J = 8.7 Hz, PMBn β anomer), 6.84 (d, 1.26H, J = 8.7 Hz, PMBn α anomer), 6.81 (d, 0.74H, J = 8.7 Hz, PMBn β anomer), 5.41 (bs, 0.37H, H-1β), 5.26 (dd, 0.63H, J = 4.5, 9.7 Hz, H-1α), 4.78–4.36 (m, 7H, PhCH2), 4.34 (t, 0.37H, J = 4.9 Hz, H-4β), 4.31, 4.30 (2d, 1H, J = 11.5 Hz, PhCH2), 4.09 (t, 0.63H, J = 5.0 Hz, H-3α), 4.06 (dd, 0.63H, J = 4.7, 2.4 Hz, H-4α), 4.03 (ddd, 0.37H, J = 4.9, 2.2 Hz, H-3β), 3.99 (t, 0.63H, J = 4.9 Hz, H-2α), 3.93 (dd, 0.37H, J = 2.2, 1.1 Hz, H-2β), 3.79–3.70 (m, 4H, H-5β, H-6aα, OCH3), 3.67 (dd, 0.63H, J = 10.0, 5.2 Hz, H-6bα), 3.65–3.57 (m, 1.37H, H-6aβ, H-6bβ, H-5α); 13C NMR (CDCl3, 125 MHz) δ 159.3 (PMBn); 138.5, 138.2, 138.0, 137.7, 137.5, 137.3 (Bn); 129.80, 128.79, 129.6, 129.5 (Ar); 128.5, 128.4 (×5), 128.3, 128.2, 128.1, 128.0, 127.9, 127.8 (×4), 127.7, 127.6 (×3), 127.5 (×2) (Bn); 113.8 (×2) (PMBn), 100.9 (C-1β), 96.2 (C-1α), 87.1 (C-2β), 84.6 (C-2α), 83.1 (C-4β), 81.7 (C-3β), 81.6 (C-3α), 81.5 (C-4α), 77.3 (C-5β), 77.1 (C-5α), 73.62, 73.58, 73.5, 73.3, 73.2, 71.9, 71.60, 71.59, 71.5 (PhCH2); 70.9 (C-6β), 70.6 (C-6α), 55.23, 5.21 (OCH3); HRMS (ESI) calcd for (M + Na) C35H38O7Na: 593.2510. Found: 593.2529.
2,5,6-Tri-O-benzyl-3-O-(4-methoxybenzyl)-β-D-galactofuranosyl trichloroacetimidate (6). To a solution of dry 14 (107 mg, 0.19 mmol) and Cl3CCN (93.7 μl, 0.94 mmol) in anh CH2Cl2 (5 mL), cooled to 0 °C was added DBU (11.2 μl, 0.075 mmol) with stirring in an argon atmosphere. After 25 min, TLC monitoring showed disappearance of the starting material. Toluene (0.7 mL) was added and the mixture was concentrated under reduced pressure to a final volumen of aprox. 0.7 mL. The concentrated was purified by column chromatography (6
:
1
:
0.07 hexane–EtOAc–TEA) to yield 6 (130 mg, 97%) as a colorless syrup: Rf 0.44 (3
:
1
:
0.04 hexane–EtOAc–TEA); 1H NMR (CDCl3, 200 MHz) δ 8.51 (s, 1H, NH), 7.45–7.24 (m, 15H, ArH), 7.11, 6.79 (2d, 4H, J = 8.7 Hz, PMBn), 6.37 (s, 1H, H-1), 4.73, 4.55 (2d, 2H, J = 11.5 Hz, PhCH2), 4.70, 4.53 (2d, 2H, J = 12.2 Hz, PhCH2), 4.49 (s, 2H, PhCH2), 4.38 (dd, 1H, J = 6.4, 3.8 Hz, H-4), 4.34, 4.24 (2d, 2H, J = 11.6 Hz, PhCH2), 4.20 (d, 1H, J = 2.2 Hz, H-2), 4.10 (dd, 1H, J = 6.4, 2.2 Hz, H-3), 3.87–3.64 (m, 3H, H-5, H-6a, H-6b), 3.75 (s, 3H, OCH3); 13C NMR (CDCl3, 50 MHz) δ 129.5, 128.4, 128.3, 128.1, 127.9, 127.6, 127.5, 113.7 (Ar); 104.2 (C-1), 86.8, 84.2, 82.4, 77.3; 73.4, 73.2, 72.0, 71.6 (PhCH2), 70.6 (C-6), 55.2 (OCH3).
Allyl 2,5,6-tri-O-benzyl-α-D-galactofuranoside (22). To a solution of 13 (510 mg, 0.84 mmol) in CH2Cl2 (13.5 mL) cooled to 0 °C was added TFA (1.4 mL, 1.82 mmol), and the mixture was stirred at room temperature. After 8 min, TLC showed disappearance of starting material and the solution was poured into NaHCO3 ss (150 mL). The mixture was extracted with CH2Cl2 (120 mL), and the organic phase was washed with water, dried (Na2SO4), filtered and concentrated. Purification of the residue by silica gel column chromatography (8
:
1 hexane–EtOAc) yielded 22 (413 mg, 99%) that crystallized from 4
:
1 hexane–EtOAc: mp 72–73 °C; Rf 0.38 (hexane–EtOAc 7
:
3); [α]D +51.7 (c 0.9, CHCl3); 1H NMR (CDCl3, 500 MHz) δ 7.39–7.25 (m, 15H, Bn), 5.86 (dddd, 1H, J = 17.1, 10.4, 6.3, 5.1, Hz, CH
CH2), 5.26 (dq, 1H, J = 17.1, 1.6 Hz, HC
CHaH), 5.17 (dq, 1H, J = 10.4, 1.4 Hz, HC
CHbH), 4.87 (d, 1H, J = 4.4 Hz, H-1), 4.72, 4.69 (2d, 2H, J = 11.8 Hz, PhCH2); 4.69, 4.65 (2d, 2H, J = 11.9 Hz, PhCH2), 4.54, 4.52 (2d, 2H, J = 12.0 Hz, PhCH2), 4.39 (ddd, 1H, J = 8.2, 7.5, 2.8 Hz, H-3), 4.12 (ddt, 1H, J = 13.0, 5.1, 1.5 Hz, OCHaH-CH
), 3.94–3.88 (m, 3H, H-2, OCHbH–CH
, H-4), 3.70–3.64 (m, 3H, H-5, H-6a, H-6b), 2.90 (d, 1H, J = 2.8 Hz, OH-2); 13C NMR (CDCl3, 125 MHz) δ 138.4, 137.7, 137.3 (Bn); 134.1 (CH
CH2), 128.6, 128.4, 128.3, 128.1, 128.0, 127.9, 127.8, 127.6 (Bn); 117.4 (CH
CH2), 98.8 (C-1), 83.14, 83.08 (C-2, C-4), 80.1 (C-5); 74.6 (C-3); 73.8, 73.1, 72.5 (PhCH2); 70.0 (C-6), 68.2 (OCH2–CH
); HRMS (ESI) calcd for (M + Na) C30H34O6Na: 513.2248. Found: 513.2251.
3-O-Benzoyl-2,5,6-tri-O-benzyl-β-D-galactofuranosyl trichloroacetimidate (24). The same procedure as described for 6 was followed starting from 32 (346 mg, 0.62 mmol); Cl3CCN (313 μl, 3.12 mmol) and DBU (37.0 μl, 0.25 mmol) to yield 24 (398 mg, 92%) as a colorless syrup: Rf 0.44 (3
:
1
:
0.04 hexane–EtOAc–TEA); 1H NMR (CDCl3, 200 MHz) δ 8.54 (s, 1H, NH), 8.03 (d, 2H, J = 7.0 Hz), 7.61–7.17 (m, 18H, ArH), 6.49 (s, 1H, H-1), 5.64 (d, 1H, J = 3.8 Hz, H-3), 4.86–4.65 (m, 5H, PhCH2, H-4), 4.47 (s, 2H, PhCH2), 4.28 (s, 1H, H-2), 4.02 (apparent q, 1H, J = 5.3 Hz, H-5), 3.76 (dd, 1H, J = 10.1, 5.1 Hz, H-6a), 3.69 (dd, 1H, J = 10.1, 6.0 Hz, H-6b); 13C NMR (CDCl3, 50 MHz) δ 160.6 (CONHCCl3), 138.4, 138.1, 137.3, 133.4, 129.9, 129.7, 128.5 (×2), 128.4 (×2), 128.2, 128.0, 127.9 (×2), 127.8, 127.7, 127.6, 127.5 (Ar); 104.2 (C-1), 96.2, 86.1, 85.7, 82.7, 77.2; 74.5, 73.50, 73.45, 72.1, 71.8 (PhCH2), 70.1 (C-6).
General procedures for glycosylation reactions
Method A. To a solution of dry acceptor (1 equiv.) and trichloroacetimidate donor (1.1 equiv.) in freshly anhyd CH2Cl2 (3 mL/0.070 mmol of donor) was added activated powdered 4 Å molecular sieves (50 mg mL−1) and the mixture was vigorously stirred at room temperature under argon. After 15 min, the mixture was cooled to −78 °C, TMSOTf (0.3 equiv.) was added, and the stirring continued for 2 h. The reaction was monitored by TLC, and quenched by addition of TEA (0.3 equiv). The mixture was diluted with CH2Cl2 (6 mL) and filtered by celite. The filtrate was concentrated under vacuum and the residue was purified by silica gel column chromatography as indicated in each case.
Method B. Same procedure described for Method A was followed using 0.05 equiv. of TMSOTf, and 0.05 equiv. of TEA.
Method C (inverse procedure). A suspension of dry acceptor (1 equiv.) in freshly anhyd CH2Cl2 (1.5 mL/0.07 mmol of donor) and activated powdered 4 Å molecular sieves (100 mg mL−1) was vigorously stirred for 15 min at room temperature under argon. The mixture was cooled to −78 °C and TMSOTf was added (0.05 equiv.). After 5 min, a solution of donor (1.1 equiv.) in anhyd CH2Cl2 (1.5 mL/0.07 mmol donor) was slowly added (aprox. 30–60 min). The reaction was monitored by TLC, and quenched by addition of TEA (0.05 equiv.), and treated as described in Method A.
Method D. The same inverse procedure described for Method C was followed using 0.3 equiv of TMSOTf in anhyd ethyl ether as solvent.
Allyl 2,5,6-tri-O-benzyl-β-D-galactofuranosyl-(1→2)-3,4-di-O-benzyl-α-L-rhamnopyranoside (15), 2,5,6-tri-O-benzyl-1-N-trichloroacetyl-α-D-galactofuranosylamine (16), and 2,5,6-tri-O-benzyl-β-D-galactofuranosyl-(1→3)-2,5,6-tri-O-benzyl-1-N-trichloroacetyl-α-D-galactofuranosylamine (17). Glycosylation of 6 (68.9 mg, 0.098 mmol) and 253 (31 mg, 0.081 mmol) following Method A gave a crude mixture which was purified by column chromatography (100
:
1; 60
:
1; 20
:
1 toluene–EtOAc) to give 15 (17.9 mg, 24%) as a colorless syrup; Rf 0.41 (8
:
1 toluene–EtOAc). [α]20D −29.4 (c 0.2, CHCl3); 1H NMR (CDCl3, 500 MHz) δ 7.34–7.16 (m, 25H, ArH), 5.81 (dddd, 1H, J = 17.3, 10.5, 6.1, 5.1 Hz, CH
CH2), 5.37 (d, 1H, J = 1.4 Hz, H-1′), 5.22 (dq, 1H, J = 17.3, 1.6 Hz, HC
CHaH), 5.12 (dq, 1H, J = 10.5, 1.3 Hz, HC
CHbH), 4.85, 4.60 (2d, 2H, J = 10.9 Hz, PhCH2), 4.71 (d, 1H, J = 1.7 Hz, H-1), 4.71, 4.67 (2d, 2H, J = 11.7 Hz, PhCH2), 4.69, 4.58 (2d, 2H, J = 11.8 Hz, PhCH2), 4.52, 4.46 (2d, 2H, J = 11.7 Hz, PhCH2), 4.51 (s, 2H, PhCH2), 4.13–4.07 (m, 4H, OCHaH–CH
, H-3′, H-3, H-4′), 4.04 (dd, 1H, J = 3.5, 1.4 Hz, H-2′), 3.91 (ddt, 1H, J = 13.0, 6.1, 1.3 Hz, OCHbH–CH
), 3.88 (dd, 1H, J = 3.3, 1.7 Hz, H-2), 3.75–3.69 (m, 2H, H-5′, H-5), 3.67 (dd, 1H, J = 10.6, 4.7 Hz, H-6a′), 3.65 (dd, 1H, J = 10.6, 6.2 Hz, H-6b′), 3.55 (t, 1H, J = 9.5 Hz, H-4), 2.94 (bs, 1H, OH-3′), 1.30 (d, 3H, J = 6.2 Hz, H-6); 13C NMR (CDCl3, 125 MHz) δ 138.6, 138.5, 138.4, 137.7, 137.5 (Ar); 133.9 (CH
CH2); 128.5, 128.4, 128.3 (×3), 128.0, 127.9, 127.8 (×2), 127.7 (×3), 127.6 (×3), 127.4, (Ar); 117.1 (CH
CH2), 107.8 (C-1′), 96.8 (C-1), 89.6 (C-2′), 84.6 (C-4′), 80.2 (C-4), 78.94 (C-2), 78.87 (C-3′), 77.8 (C-5′), 76.9 (C-3); 75.2, 73.6, 73.3, 73.0, 71.7 (PhCH2); 70.2 (C-6′), 68.0 (C-5), 67.8 (OCH2–CH
), 18.0 (C-6); HRMS (ESI) calcd for (M + Na) C50H56O10Na: 839.3766. Found: 839.3778.Next fraction from the column gave 17 (10.4 mg, 18%) as colorless syrup; Rf 0.30 (8
:
1 toluene–EtOAc); [α]20D −7.5 (c 0.2, CHCl3); 1H NMR (CDCl3, 500 MHz) δ 8.00 (d, 1H, J = 8.7 Hz, NH), 7.38–7.17 (m, 30H, ArH), 5.83 (dd, 1H, J = 8.7, 5.8 Hz, H-1), 4.93 (d, 1H, J = 1.4 Hz, H-1′), 4.71–4.43 (m, 12H, PhCH2), 4.33 (t, 1H, J = 5.9 Hz), 4.16 (t, 1H, J = 6.0 Hz), 4.10 (dd, 1H, J = 5.4, 1.9 Hz), 4.07 (m, 1H), 4.00 (t, 1H, J = 6.8 Hz), 3.95 (m, 1H), 3.92 (dd, 1H, J = 4.2, 1.4 Hz, H-2′), 3.71–3.58 (m, 5H), 2.72 (d, 1H, J = 3.7 Hz, OH-3′); 13C NMR (CDCl3, 125 MHz) δ 161.3 (NHCO), 138.8, 138.1, 137.31, 137.26, 129.0, 128.53, 128.46, 128.4, 128.3, 128.0, 127.9, 127.8, 127.73, 127.70, 127.6, 127.5 (Ar); 106.6 (C-1′β); 89.5, 83.8, 83.3, 82.3, 81.0, 80.7, 77.4, 76.8, 76.5; 74.5, 73.6, 73.4, 73.1, 72.9, 72.0 (PhCH2), 70.6, 70.1 (C-6, C-6′); HRMS (ESI) calcd for (M + Na) C56H58O11NaCl3N: 1048.2968. Found: 1048.2980.
Last fraction gave 16 (20.9 mg, 36%) as a colorless syrup; Rf 0.23 (8
:
1 toluene–EtOAc); [α]20D 21.4 (c 0.6, CHCl3); 1H NMR (CDCl3, 500 MHz) δ 7.84 (d, 1H, J = 8.2 Hz, NH), 7.38–7.27 (m, 15H, ArH), 5.80 (dd, 1H, J = 8.2, 5.7 Hz, H-1), 4.78–4.51 (m, 6H, PhCH2), 4.32 (t, 1H, J = 6.2 Hz), 4.11 (t, 1H, J = 6.0 Hz), 4.00 (dd, 1H, J = 6.3, 2.8 Hz), 3.79–3.72 (m, 2H), 3.65 (dd, 1H, J = 9.0, 5.0 Hz); 13C NMR (CDCl3, 125 MHz) δ 161.3 (NHCO), 138.1, 137.7, 137.33, 137.26, 129.0, 128.64, 128.58, 128.52, 128.49, 128.45, 128.42, 128.36, 128.3, 128.1, 128.01, 127.95, 127.9, 127.84, 127.81, 127.73, 127.71 (Ar); 84.1, 82.6, 80.7, 76.5, 74.9; 73.72, 73.66, 73.1 (PhCH2), 70.0 (C-6); HRMS (ESI) calcd for (M + Na) C29H30O6NaCl3N: 616.1031. Found: 616.1010.
Allyl 2,5,6-tri-O-benzyl-3-O-(4-methoxybenzyl)-α-D-galactofuranosyl-(1→2)-3,4-di-O-benzyl-α-L-rhamnopyranoside (18). Compound 18 was obtained according to general procedures (Method B) from donor 6 (46.3 mg, 0.065 mmol) and acceptor 2 (22.6 mg, 0.059 mmol). The crude was purified by column chromatography (100
:
0.75 toluene–EtOAc) to afford a first fraction of 2,5,6-tri-O-benzyl-3-O-(4-methoxybenzyl)-1-N-trichloroacetyl-α-D-galactofuranosylamine (19, 19.5 mg, 42% from 6) as a syrup; Rf 0.60 (9
:
1 toluene–EtOAc ); [α]D −5.4 (c 1.2, CHCl3); 1H NMR (CDCl3, 500 MHz) δ 8.02 (d, 1H, J = 8.8 Hz, NH), 7.38–7.22 (m, 15H, ArH), 7.14, 6.84 (2 d, 4H, J = 8.7 Hz, PMBn), 5.88 (dd, 1H, J = 8.8, 5.7 Hz, H-1); 4.70, 4.61, 4.47, 4.46, 4.41, 4.24 (6d, 6H, J = 11.4 Hz, PhCH2); 4.54, 4.50 (2d, 2H, J = 12.0 Hz, PhCH2), 4.22 (t, 1H, J = 5.6 Hz, H-2), 4.12 (t, 1H, J = 5.4 Hz, H-3), 4.09 (dd, 1H, J = 5.2, 2.1 Hz, H-4), 3.76 (s, 3H, OCH3), 3.71 (dd, 1H, J = 9.7, 6.4 Hz, H-6a), 3.65 (dd, 1H, J = 9.7, 5.4 Hz, H-6b), 3.61 (dt, 1H, J = 5.8, 2.1 Hz, H-5); 13C NMR (CDCl3, 125 MHz) δ 161.3 (NHCO), 159.4, 137.9, 137.3, 137.1, 130.0, 129.6 (×2), 129.0, 128.5, 128.4 (×3), 128.3, 128.0, 127.9, 127.7, 127.6, 113.9 (Ar); 83.9 (C-2), 82.1 (C-4), 81.4 (C-1), 81.0 (C-3), 76.7 (C-5), 73.9, 73.6, 72.9, 71.7 (PhCH2), 70.4 (C-6), 55.3 (OCH3). HRMS (ESI) calcd for (M + Na) C37H38O7NaCl3N: 736.1606. Found: 736.1614.Next fraction from the column gave syrupy 18 (22.6 mg, 41%); Rf 0.47 (9
:
1 toluene–EtOAc); [α]D +8.7 (c 1.1, CHCl3); 1H NMR (CDCl3, 500 MHz) δ 7.33–7.18 (m, 25H, Bn), 7.14, 6.76 (2d, 4H, J = 8.7 Hz, PMBn); 5.78 (dddd, 1H, J = 17.1, 10.4, 6.1, 5.1 Hz, CH
CH2), 5.29 (d, 1H, J = 4.2 Hz, H-1′), 5.19 (dq, 1H, J = 17.1, 1.6 Hz, HC
CHaH), 5.09 (dq, 1H, J = 10.4, 1.4 Hz, HC
CHbH); 4.96, 4.53 (2d, 2H, J = 11.2 Hz, PhCH2), 4.83 (d, 1H, J = 2.2 Hz, H-1); 4.71 (d, 1H, J = 11.6 Hz, PhCH2), 4.69, 4.43 (2d, 2H, J = 10.9 Hz, PhCH2), 4.65–4.60 (m, 4H, PhCH2), 4.45 (d, 1H, J = 11.2 Hz, PhCH2), 4.34, 4.31 (2d, 2H, J = 12.1 Hz, PhCH2), 4.26 (dd, 1H, J = 8.7, 3.2 Hz, H-3), 4.22 (t, 1H, J = 7.1 Hz, H-3′), 4.11–4.06 (m, 1H, OCHaH–CH
), 4.08 (dd, 1H, J = 4.2, 7.1 Hz, H-2′), 3.99 (t, 1H, J = 6.9 Hz, H-4′), 3.98 (t, 1H, J = 2.7 Hz, H-2), 3.90 (ddt, 1H, J = 13.0, 6.1, 1.3 Hz, OCHbH–CH
), 3.76 (m, 1H, H-5′), 3.71 (s, 3H, OCH3), 3.70 (m, 1H, H-5), 3.58 (t, 1H, J = 9.0 Hz, H-4), 3.46 (dd, 1H, J = 10.5, 3.9 Hz, H-6a′), 3.36 (dd, 1H, J = 10.5, 5.9 Hz, H-6b′), 1.26 (d, 3H, J = 6.3 Hz, H-6); 13C NMR (CDCl3, 125 MHz) δ 159.2 (PMBn); 139.0, 138.8, 138.4, 138.2, 138.0 (Bn); 134.0 (CH
CH2); 130.1, 129.7 (PMBn); 128.3 (×2), 128.2, 128.1, 128.0, 127.9 (×2), 127.8, 127.6 (×2), 127.5, 127.4 (×2), 127.2 (Bn); 117.1 (CH
CH2), 113.7 (PMBn), 98.2 (C-1′), 96.7 (C-1), 84.6 (C-2′), 80.9 (C-4′), 80.3 (C-3′), 80.0 (C-5′, C-4); 75.5 (C-3), 75.3 (C-2), 73.2, 73.1, 72.6, 72.0, 71.5 (PhCH2); 70.0 (C-6′), 68.0 (C-5), 67.9 (OCH2–CH
), 55.2 (OCH3), 18.0 (C-6). HRMS (ESI) calcd for (M + Na) C58H64O11Na: 959.4341. Found: 959.4346.
Method C. Compound 6 (50.7 mg, 0.071 mmol) and 2 (24.8 mg, 0.064 mmol) gave 30.5 mg of 18 (51%) and 9.1 mg of 19 (18%).
Allyl 2,5,6-tri-O-benzyl-3-O-(4-methoxybenzyl)-α-D-galactofuranosyl-(1→2)-3,4-di-O-benzoyl-α-L-rhamnopyranoside (21α) and allyl 2,5,6-tri-O-benzyl-3-O-(4-methoxybenzyl)-α-D-galactofuranosyl-(1→2)-3,4-di-O-benzoyl-α-L-rhamnopyranoside (21β). Compound 21α was obtained according to Method C from donor 6 (85.6 mg, 0.12 mmol) and allyl 3,4-di-O-benzoyl-α-L-rhamnopyranoside62 (20) (44.9 mg, 0.11 mmol). The crude was purified by column chromatography (100
:
1 toluene–EtOAc) to afford a first fraction of trichloroacetamide 19 (18 mg, 21%). Second fraction afforded 21β (13 mg, 11%) as a syrup, Rf 0.48 (9
:
1 toluene–EtOAc); [α]D +6.9 (c 0.1, CHCl3); 1H NMR (CDCl3, 500 MHz) δ 7.98, 7.89 (2d, 4H, J = 7.1 Hz, Ar), 7.50–7.18 (m, 21H, Ar), 7.11, 6.80 (2d, 4H, J = 8.6 Hz, Ar), 5.87 (dddd, 1H, J = 17.0, 10.5, 6.0, 5.2 Hz, CH
CH2), 5.65 (t, 1H, J = 9.6 Hz, H-4), 5.62 (dd, 1H, J = 10.1, 3.0 Hz, H-3), 5.29 (dq, 1H, J = 17.0, 1.6 Hz, HC
CHaH), 5.16 (dq, 1H, J = 10.5, 1.3 Hz, HC
CHbH), 5.15 (d, 1H, J = 1.4 Hz, H-1′), 4.91 (d, 1H, J = 1.7 Hz, H-1); 4.64, 4.46 (2d, 2H, J = 11.8 Hz, PhCH2), 4.50 (s, 2H, PhCH2), 4.45 (d, 1H, J = 11.8 Hz, PhCH2), 4.41 (d, 1H, J = 11.1 Hz, PhCH2), 4.37–4.34 (m, 2H, PhCH2, H-2), 4.20 (d, 1H, J = 11.1 Hz, PhCH2), 4.18 (dd, 1H, J = 4.3, 1.8 Hz, H-2′), 4.16 (dd, 1H, J = 8.5, 3.0 Hz, H-4′), 4.13 (ddt, 1H, J = 13.0, 5.2 Hz, OCHaH–CH
), 4.07 (dq, 1H, J = 8.5, 6.2, H-5), 3.99 (dd, 1H, J = 8.6, 6.0 Hz, H-3′), 3.90 (ddt, 1H, J = 13.0, 6.0 Hz, OCHbH–CH
), 3.75 (s, 3H, OCH3), 3.72 (m, 1H, H-5′), 3.66 (dd, 1H, J = 10.0, 6.4 Hz, H-6a′), 3.61 (dd, 1H, J = 10.0, 5.2 Hz, H-6b′), 1.30 (d, 3H, J = 6.2 Hz, H-6); HRMS (ESI) calcd for (M + Na) C58H60O13Na: 987.3926. Found: 987.3940.Last fraction from the column gave 21α (33 mg, 36%) as a colorless syrup; Rf 0.42 (9
:
1 toluene–EtOAc). [α]D +58.2 (c 1.1, CHCl3); 1H NMR (CDCl3, 500 MHz) δ 7.93 (m, 4H, ArH), 7.50–7.15 (m, 21H, Ar), 7.14, 6.82 (2d, 4H, J = 8.6 Hz, ArH), 5.92 (dddd, 1H, J = 17.0, 10.4, 6.2, 5.3 Hz, CH
CH2), 5.73 (dd, 1H, J = 9.8, 3.0 Hz, H-3), 5.69 (t, 1H, J = 9.5 Hz, H-4), 5.35 (dq, 1H, J = 17.0, 1.5 Hz, HC
CHaH), 5.23 (dq, 1H, J = 10.4, 1.3 Hz, HC
CHbH), 5.18 (d, 1H, J = 4.2 Hz, H-1′), 5.05 (d, 1H, J = 1.5 Hz, H-1); 4.76, 4.57 (2d, 2H, J = 11.4 Hz, PhCH2), 4.61, 4.43 (2d, 2H, J = 11.1 Hz, PhCH2), 4.47, 4.41 (2d, 2H, J = 11.8 Hz, PhCH2), 4.36–4.32 (m, 3H, PhCH2, H-2), 4.27 (t, 1H, J = 7.2 Hz, H-3′), 4.22 (dd, 1H, J = 12.9, 5.3 Hz, OCHaH–CH
), 4.09–4.05 (m, 2H, H-2′, H-5), 3.98 (dd, 1H, J = 12.9, 6.2 Hz, OCHbH–CH
), 3.93 (t, 1H, J = 7.2 Hz, H-4′), 3.77 (s, 3H, OCH3), 3.49 (ddd, 1H, J = 7.2, 5.6, 4.0 Hz, H-5′), 3.38 (dd, 1H, J = 10.5, 4.0 Hz, H-6a′), 3.34 (dd, 1H, J = 10.5, 5.6 Hz, H-6b′), 1.22 (d, 3H, J = 6.3 Hz, H-6); 13C NMR (CDCl3, 125 MHz) δ 165.9, 165.5 (CO), 159.2, 139.0, 138.5, 137.9 (Ar); 133.7 (CH
CH2); 132.00, 132.96, 129.9, 129.8, 129.74, 129.70, 129.68, 129.66, 129.6, 128.44, 128.42, 128.4, 128.3, 128.21, 128.19, 128.1, 127.9, 127.8, 127.7, 127.5, 127.3, 127.2 (Ar); 117.6 (CH
CH2), 113.7 (Ar), 98.6 (C-1′), 96.0 (C-1), 84.3 (C-2′), 81.0 (C-4′), 80.2 (C-5′, C-3′), 73.1 (C-2), 72.1 (C-4); 71.3 (C-3); 73.2, 72.8, 72.6, 72.1, 71.7 (PhCH2); 69.6 (C-6′), 68.5 (OCH2–CH
), 67.0 (C-5), 55.2 (OCH3), 17.7 (C-6); HRMS (ESI) calcd for (M + Na) C58H60O13Na: 987.3926. Found: 987.3944.
Allyl 2,3,4,6-tetra-O-benzyl-α-D-glucopyranosyl-(1→3)-2,5,6-tri-O-benzyl-α-D-galactofuranoside (23). Compound 23 was obtained according to Method D from 2,3,4,6-tetra-O-benzyl-D-glucopyranosyl trichloroacetimidate51,52 (5) (77.0 mg, 0.11 mmol) and 22 (43.0 mg, 0.087 mmol). The crude was purified by column chromatography (50
:
1 toluene–EtOAc) to afford syrupy 23 (80.5 mg, 91%); Rf 0.47 (10
:
1 toluene–EtOAc); [α]D +59.8 (c 0.8, CHCl3); 1H NMR (CDCl3, 500 MHz) δ 7.34–7.13 (m, 35H, Bn), 5.83 (dddd, 1H, J = 17.1, 10.4, 6.7, 5.1 Hz, CH
CH2), 5.23 (dd, 1H, J = 17.1, 1.6 Hz, HC
CHaH), 5.17 (d, 1H, J = 3.5 Hz, H-1′), 5.19 (ddt, 1H, J = 10.4, 1.4 Hz, HC
CHbH), 4.89, 4.75 (2d, 2H, J = 11.8 Hz, PhCH2), 4.84 (d, 1H, J = 4.5 Hz, H-1), 4.82, 4.49 (2d, 2H, J = 11.4 Hz, PhCH2), 4.74, 4.64 (2d, 2H, J = 11.8 Hz, PhCH2), 4.56, 4.34 (2d, 2H, J = 12.1 Hz, PhCH2), 4.54–4.50 (m, 5H, PhCH2, H-3), 4.48, 4.42 (d, 2H, J = 11.9 Hz, PhCH2), 4.14 (dd, 1H, J = 6.7, 5.1 Hz, H-4), 4.12 (ddt, 1H, J = 13.0, 5.1, 1.6 Hz, OCHaH–CH
), 4.08 (dd, 1H, J = 7.7, 4.4 Hz, H-2), 4.05 (dt,1H, J = 10.1, 2.7 Hz, H-5′), 3.94 (t, 1H, J = 9.3 Hz, H-3′), 3.89 (ddt, 1H, J = 13.0, 6.7, 1.2 Hz, OCHbH–CH
), 3.78 (dd, 1H, J = 10.0, 3.3 Hz, H-6a), 3.72 (m, 1H, H-5), 3.68 (t, 1H, J = 10.1 Hz, H-4′), 3.67 (dd, 1H, J = 10.0, 6.4 Hz, H-6b), 3.62 (dd, 1H, J = 10.8, 3.2 Hz, H-6a′), 3.53–3.50 (m, 2H, H-2′, H-6b′); 13C NMR (CDCl3, 125 MHz) δ 138.8, 139.6 (×2), 138.0, 137.6 (Ar); 134.1 (CH
CH2), 128.5, 128.4, 128.3 (×3), 128.0 (×2), 127.9, 127.8, 127.7 (×2), 127.6, 127.5 (×2), 127.4, 127.3 (Ar); 117.6 (CH
CH2), 98.3 (C-1), 95.8 (C-1′), 83.2 (C-2) 81.9 (C-3′), 80.7 (C-4), 79.8 (C-2′), 79.0 (C-5); 78.4 (C-3), 77.6 (C-4′); 75.6, 74.9, 73.4, 73.2, 72.9, 72.8 (×2) (PhCH2); 70.4 (C-5′), 70.1 (C-6), 68.3 (C-6′), 68.1 (OCH2–CH
); HRMS (ESI) calcd for (M + NH4) C64H72O11N: 1030.5100. Found: 1030.5098.
Allyl 3-O-benzoyl-2,5,6-tri-O-benzyl-α-D-galactofuranosyl-(1→2)-3,4-di-O-benzyl-α-L-rhamnopyranoside (36). Compound 36 was obtained according to Method C from donor 24 (398 mg, 0.57 mmol) and acceptor 2 (182 mg, 0.47 mmol). The crude was purified by column chromatography (20
:
1 hexane–EtOAc) to afford 36 (401.6 mg, 92%) as a syrup; Rf 0.56 (9
:
1 toluene–EtOAc); [α]D +19.5 (c 1.3, CHCl3); 1H NMR (CDCl3, 500 MHz) δ 7.99 (d, 2H, J = 8.5 Hz, ArH), 7.57–7.03 (m, 28H, ArH), 5.97 (t, 1H, J = 6.7 Hz, H-3′), 5.80 (dddd, 1H, J = 17.2, 10.5, 6.1, 5.1 Hz, CH
CH2), 5.30 (d, 1H, J = 4.2 Hz, H-1′), 5.20 (dq, 1H, J = 17.2, 1.6 Hz, HC
CHaH), 5.11 (dq, 1H, J = 10.5, 1.4 Hz, HC
CHbH), 5.02, 4.58 (2d, 2H, J = 10.9 Hz, PhCH2), 4.82 (d, 1H, J = 2.2 Hz, H-1), 4.75, 4.70 (2d, 2H, J = 12.1 Hz, PhCH2), 4.69, 4.54 (2d, 2H, J = 11.6 Hz, PhCH2), 4.65, 4.53 (2d, 2H, J = 12.1 Hz, PhCH2), 4.28 (dd, 1H, J = 7.0, 6.4 Hz, H-4′), 4.24 (dd, 1H, J = 8.9, 3.1 Hz, H-3), 4.18 (dd, 1H, J = 7.0, 4.2 Hz, H-2′), 4.17 (s, 2H, PhCH2), 4.11 (ddt, 1H, J = 13.0, 5.1, 1.5 Hz, OCHaH–CH
), 3.97 (t, 1H, J = 2.7 Hz, H-2), 3.92 (ddt, 1H, J = 13.0, 6.1, 1.4 Hz, OCHbH–CH
), 3.82 (dt, 1H, J = 7.6, 3.8 Hz, H-5′), 3.71 (dq, 1H, J = 9.3, 6.1 Hz, H-5), 3.63 (t, 1H, J = 9.1 Hz, H-4), 3.38 (d AB, 2H, J = 4.0 Hz, H-6a′, H-6b′), 1.30 (d, 3H, J = 6.2 Hz, H-6); 13C NMR (CDCl3, 125 MHz) δ 165.5 (CO); 138.9, 138.6, 138.3, 138.1, 137.6 (Ar); 134.0 (CH
CH2); 133.2, 129.8, 128.4, 128.3, 128.1, 128.0, 127.9, 127.8, 127.7, 127.6 (×2), 127.5, 127.2 (×2) (Ar); 117.1 (CH
CH2), 99.0 (C-1′), 97.1 (C-1), 82.3 (C-2′), 80.8 (C-4′), 80.2 (C-4), 79.7 (C-5′), 76.1 (C-3), 75.9 (C-3′), 75.2 (C-2), 74.9, 73.1, 72.9, 72.8, 72.0 (PhCH2); 68.7 (C-6′), 68.2 (C-5), 67.8 (OCH2–CH
), 17.9 (C-6); HRMS (ESI) calcd for (M + Na) C57H60O11Na: 94.34028. Found: 943.4015.3-O-Benzoyl-2,5,6-tri-O-benzyl-1-N-trichloroacetyl-α-D-galactofuranosylamine (37, 10.5 mg, 3%) was also recovered from the column as a syrup; Rf 0.64 (9
:
1 toluene–EtOAc); [α]D +19.9 (c 1.0, CHCl3); 1H NMR (CDCl3, 500 MHz) δ 8.17 (d, 1H, J = 9.1 Hz, NH), 8.01 (d, 2H, J = 8.4 Hz, ArH), 7.63–7.21 (m, 18H, ArH), 6.00 (dd, 1H, J = 9.1, 6.0 Hz, H-1), 5.68 (t, 1H, J = 4.5 Hz, H-3), 4.85 (s, 2H, PhCH2), 4.65, 4.60 (2d, 2H, J = 11.9 Hz, PhCH2), 4.55, 4.48 (2d, 2H, J = 12.0 Hz, PhCH2), 4.47 (dd, 1H, J = 6.1, 4.9 Hz, H-2), 4.26 (dd, 1H, J = 4.0, 2.6 Hz, H-4), 4.13 (dt, 1H, J = 6.3, 2.6 Hz, H-5), 3.69 (dd, 1H, J = 9.7, 6.2 Hz, H-6a), 3.66 (dd, 1H, J = 9.7, 6.4 Hz, H-6b); 13C NMR (CDCl3, 125 MHz) δ 166.3 (PhCO), 161.6 (NHCO), 137.9, 137.3, 137.0, 133.6, 129.8, 129.3, 129.2, 128.9, 128.5, 128.4 (×2), 128.3 (×2), 128.2, 128.0 (×2), 127.9, 127.8, 127.7 (×2), 127.6 (Ar); 82.8, 81.8, 81.2, 78.4, 78.0; 75.0, 73.6, 72.8 (PhCH2), 69.8 (C-6); HRMS (ESI) calcd for (M + Na) C36H34O7NaCl3N: 720.1293. Found: 720.1297.
Allyl 2,5,6-tri-O-benzyl-3-O-pentafluorobenzoyl-α-D-galactofuranosyl-(1→2)-3,4-di-O-benzyl-α-L-rhamnopyranoside (38). Compound 38 was obtained according to Method C from donor 25 (45 mg, 0.057 mmol) and acceptor 2 (18.3 mg, 0.048 mmol). The crude was purified by column chromatography (200
:
1 toluene–EtOAc) to afford 38 (36.8 mg, 75%) as a syrup; Rf 0.66 (10
:
1 toluene–EtOAc); [α]D +12.6 (c 1.0, CHCl3); 1H NMR (CDCl3, 500 MHz) δ 7.36–7.10 (m, 25H, ArH), 5.96 (t, 1H, J = 6.8 Hz, H-3′), 5.80 (dddd, 1H, J = 17.2, 10.4, 6.1, 5.2 Hz, CH
CH2), 5.30 (d, 1H, J = 4.2 Hz, H-1′), 5.20 (dq, 1H, J = 17.2, 1.6 Hz, HC
CHaH), 5.11 (dq, 1H, J = 10.4, 1.4 Hz, HC
CHbH), 4.97 (d, 1H, J = 10.9 Hz, PhCH2), 4.83 (d, 1H, J = 2.1 Hz, H-1), 4.75–4.48 (m, 7H, PhCH2), 4.29, 4.23 (2d, 2H, J = 12.2 Hz, PhCH2), 4.28 (dd, 1H, J = 7.4, 6.3 Hz, H-4′), 4.24 (dd, 1H, J = 9.0, 3.2 Hz, H-3), 4.19 (dd, 1H, J = 7.2, 4.2 Hz, H-2′), 4.10 (ddt, 1H, J = 13.0, 5.1, 1.5 Hz, OCHaH–CH
), 3.98 (t, 1H, J = 2.6 Hz, H-2), 3.92 (ddt, 1H, J = 13.0, 6.1, 1.3 Hz, OCHbH–CH
), 3.81 (dt, 1H, J = 7.4, 3.8 Hz, H-5′), 3.71 (dq, 1H, J = 9.7, 6.1 Hz, H-5), 3.62 (t, 1H, J = 9.2 Hz, H-4), 3.39 (d AB, 2H, J = 3.8 Hz, H-6a′, H-6b′), 1.31 (d, 1H, J = 6.1 Hz, H-6); 13C NMR (CDCl3, 125 MHz) δ 163.4 (CO); 138.7, 138.5, 138.2, 137.4 (Ar); 133.9 (CH
CH2), 128.3 (×2), 128.1, 128.0 (×2), 127.9, 127.8, 127.7 (×2), 127.6, 127.3, 127.2, 127.1 (Ar); 117.2 (CH
CH2), 98.5 (C-1′), 96.9 (C-1), 82.5, 80.4, 80.2, 79.7, 77.4, 75.8, 75.1, 74.9, 73.0, 72.9, 72.7, 72.3, 68.7, 68.2, 67.9; 17.9 (C-6). HRMS (ESI) calcd for (M + Na) C57H55O11F5Na: 1033.3557. Found: 1033.3544.
Allyl 2,5,6-tri-O-benzyl-3-O-(4-methoxybenzoyl)-α-D-galactofuranosyl-(1→2)-3,4-di-O-benzyl-α-L-rhamnopyranoside (39). Compound 39 was obtained according to Method C from donor 26 (71,0 mg, 0.097 mmol) and acceptor 2 (29.6 mg, 0.077 mmol). The crude was purified by column chromatography (12
:
1 hexane–EtOAc) to afford a first fraction of 2,5,6-tri-O-benzyl-3-O-(4-methoxybenzoyl)-1-N-trichloroacetyl-α-D-galactofuranosylamine (40, 20 mg, 28%) as a syrup; Rf 0.63 (8
:
1 toluene–EtOAc); [α]D +35.1 (c 0.2, CHCl3); 1H NMR (CDCl3, 500 MHz) δ 8.16 (d, 1H, J = 9.1 Hz, NH), 7.96, 6.95 (2d, 4H, J = 9.0 Hz, ArH); 7.40–7.21 (m, 15H, ArH), 5.99 (dd, 1H, J = 9.1, 6.0 Hz, H-1), 5.66 (t, 1H, J = 4.5 Hz, H-3), 4.85 (s, 2H, PhCH2), 4.65, 4.60 (2d, 2H, J = 11.9 Hz, PhCH2), 4.54, 4.48 (2d, 2H, J = 12.0 Hz, PhCH2), 4.46 (dd, 1H, J = 6.0, 4.9 Hz, H-2), 4.24 (dd, 1H, J = 4.0, 2.7 Hz, H-4), 4.14 (dt, 1H, J = 6.3, 2.7 Hz, H-5), 3.89 (s, 3H, OCH3), 3.68 (dd, 1H, J = 9.8, 6.2 Hz, H-6a), 3.66 (dd, 1H, J = 9.8, 6.4 Hz, H-6b); 13C NMR (CDCl3, 125 MHz 3) δ 166.0, 163.9, 161.6 (Ar, CO), 137.1, 131.9, 128.9, 128.5, 128.4, 128.2, 127.9, 127.8, 127.7, 127.6, 113.8 (Ar); 82.9, 81.8, 81.2, 78.1, 78.0; 75.0, 73.5, 72.7 (PhCH2), 69.9 (C-6), 55.5 (OCH3); HRMS (ESI) calcd for (M + Na) C37H36O8NaCl3N: 750.1399. Found: 750.1399.Next fraction from the column gave 39 (49.1 mg, 67%) as a colorless syrup; Rf 0.56 (8
:
1 toluene–EtOAc); [α]D +15.2 (c 1.2, CHCl3); 1H NMR (CDCl3, 500 MHz) δ 7.95, 6.89 (2d, 4H, J = 9.0 Hz, ArH), 7.34–7.06 (m, 25H, ArH), 5.94 (t, 1H, J = 6.6 Hz, H-3′), 5.80 (dddd, 1H, J = 17.2, 10.4, 6.1, 5.1 Hz, CH
CH2), 5.30 (d, 1H, J = 4.2 Hz, H-1′), 5.20 (dq, 1H, J = 17.2, 1.6 Hz, HC
CHaH), 5.10 (dq, 1H, J = 10.4, 1.4 Hz, HC
CHbH), 5.03, 4.57 (2d, 2H, J = 10.9 Hz, PhCH2), 4.81 (d, 1H, J = 2.1 Hz, H-1), 4.75, 4.71 (2d, 2H, J = 12.1 Hz, PhCH2), 4.70, 4.55 (2d, 2H, J = 11.5 Hz, PhCH2), 4.65, 4.54 (2d, 2H, J = 12.2 Hz, PhCH2), 4.27 (dd, 1H, J = 7.0, 6.4 Hz, H-4′), 4.24 (dd, 1H, J = 9.0, 3.1 Hz, H-3), 4.17 (dd, 1H, J = 6.9, 4.2 Hz, H-2′), 4.17 (s, 2H, PhCH2), 4.10 (ddt, 1H, J = 13.1, 5.1, 1.5 Hz, OCHaH–CH
), 3.97 (t, 1H, J = 2.6 Hz, H-2), 3.91 (ddt, 1H, J = 13.1, 6.1, 1.4 Hz, OCHbH–CH
), 3.87 (s, 3H, OCH3), 3.82 (dt, 1H, J = 7.0, 4.1 Hz, H-5′), 3.71 (dq, 1H, J = 9.2, 6.2 Hz, H-5), 3.63 (t, 1H, J = 9.1 Hz, H-4), 3.39 (d AB, 2H, J = 4.2 Hz, H-6a′, H-6b′), 1.30 (d, 1H, J = 6.2 Hz, H-6); 13C NMR (CDCl3, 125 MHz) δ 165.2, 163.5 (CO, Ar); 139.0, 138.6, 138.4, 138.1, 137.7 (Ar); 134.0 (CH
CH2); 131.8, 128.3 (×2), 128.1, 128.0, 127.9, 127.7 (×2), 127.6, 127.5, 127.4, 127.2, 127.1, 122.2 (Ar); 117.1 (CH
CH2), 113.6 (Ar), 99.1 (C-1′), 97.1 (C-1), 82.3 (C-2′), 80.9 (C-4′), 80.2 (C-4), 79.8 (C-5′), 76.2 (C-3), 75.6 (C-3′), 75.2 (C-2); 74.9, 73.1, 72.9, 72.8, 71.9 (PhCH2); 68.8 (C-6′), 68.2 (C-5), 67.8 (OCH2–CH
), 55.4 (OCH3), 17.9 (C-6); HRMS (ESI) calcd for (M + Na) C58H62O12Na: 973.4134. Found: 973.4160.
Allyl 2,5,6-tri-O-benzyl-3-O-triisopropylsilyl-α-D-galactofuranosyl-(1→2)-3,4-di-O-benzyl-α-L-rhamnopyranoside (41). Compound 41 was obtained according to Method C from donor 27 (49 mg, 0.065 mmol) and acceptor 2 (20.7 mg, 0.054 mmol). The crude was purified by column chromatography (160
:
1 toluene–EtOAc) to afford a first fraction of 2,5,6-tri-O-benzyl-3-O-triisopropylsilyl-α-D-galactofuranosyl-(1→1)-2,5,6-tri-O-benzyl-3-O-triisopropylsilyl-α-D-galactofuranoside (42, 10.2 mg, 16%); Rf 0.56 (20
:
1 toluene–EtOAc); Rf 0.69 (8
:
2 hexane–EtOAc); [α]20D+45.3 (c 0.2; CHCl3); 1H NMR (CDCl3, 500 MHz) δ 7.33–7.07 (m, 15H, ArH), 5.61 (d, 1H, J = 4.0 Hz, H-1), 4.70 (d, 1H, J = 11.5 Hz, PhCH2), 4.58–4.52 (m, 3H, PhCH2, H-3), 4.48, 4.45 (2d, 2H, J = 12.0 Hz, PhCH2), 3.93 (dd, 1H, J = 5.7, 4.3 Hz, H-4), 3.85 (d, 1H, J = 10.5 Hz, PhCH2), 3.80 (dt, 1H, J = 6.3, 4.4 Hz, H-5), 3.76–3.72 (m, 2H, H-2, H-6a), 3.71 (dd, 1H, J = 10.2, 6.4 Hz, H-6b), 0.97–0.89 (m, 21H, (CH3)2CH)3Si); 13C NMR (CDCl3, 125 MHz) δ 138.8, 138.32, 138.28, 128.5, 128.3, 128.2, 127.9, 127.8, 127.5, 127.4, 127.3, 127.0 (Ar); 95.8 (C-1), 84.2 (C-2), 83.6 (C-4), 77.4 (C-5), 75.0 (C-3); 73.2, 73.0, 71.9 (PhCH2), 71.4 (C-6), 18.05, 18.03 (CH3)2CH)3Si), 12.4 (CH3)2CH)3Si). HRMS (ESI) calcd for (M + Na) C72H98O11NaSi2: 1217.6540. Found: 1217.6504.Next fraction from the column gave 2,5,6-tri-O-benzyl-3-O-triisopropylsilyl-1-N-trichloroacetyl-α-D-galactofuranosylamine (43, 1.5 mg, 3%); Rf 0.63 (8
:
2 hexane–EtOAc); [α]20D +18.1 (c 0.1, CHCl3); 1H NMR (CDCl3, 500 MHz) δ 8.12 (d, 1H, J = 9.2 Hz, NH), 7.42–7.19 (m, 15H, ArH), 5.98 (dd, 1H, J = 9.2, 5.7 Hz, H-1), 4.80, 4.64 (2d, 2H, J = 11.5 Hz, PhCH2), 4.58–4.52 (m, 4H, PhCH2, H-3), 4.64 (d, 1H, J = 11.0 Hz, PhCH2), 4.15 (dd, 1H, J = 5.4, 4.7 Hz), 4.08 (t, 1H, J = 3.0 Hz), 3.81–3.74 (m, 2H), 3.70 (dd, 1H, J = 9.4, 5.1 Hz), 1.02–0.89 (m, 21H, (CH3)2CH)3Si); HRMS (ESI) calcd for (M + Na) C38H50O6SiNaCl3N: 772.2365. Found: 772.2353.
Last fraction gave 41 (30.0 mg, 57%) as a colorless syrup; Rf 0.47 (20
:
1 toluene–EtOAc); [α]D +13.5 (c 1.0, CHCl3); 1H NMR (CDCl3, 500 MHz) δ 7.35–7.17 (m, 25H, ArH), 5.78 (dddd, 1H, J = 17.2, 10.5, 6.1, 5.2 Hz, CH
CH2), 5.32 (d, 1H, J = 4.0 Hz, H-1′), 5.18 (dq, 1H, J = 17.2, 1.6 Hz, HC
CHaH), 5.08 (dq, 1H, J = 10.4, 1.4 Hz, HC
CHbH), 5.00, 4.47 (2d, 2H, J = 11.3 Hz, PhCH2), 4.80 (d, 1H, J = 2.4 Hz, H-1), 4.71 (s, 2H, PhCH2), 4.69, 4.60 (2d, 2H, J = 11.8 Hz, PhCH2), 4.67, 4.37 (2d, 2H, J = 11.2 Hz, PhCH2), 4.59 (t, 1H, J = 6.1 Hz, H-3′), 4.39 (m, 2H, PhCH2), 4.27 (dd, 1H, J = 8.6, 3.2 Hz, H-3), 4.08 (ddt, 1H, J = 13.0, 5.1, 1.5 Hz, OCHaH–CH
),3.93 (t, 1H, J = 2.7 Hz, H-2), 3.90 (t, 1H, J = 6.4 Hz, H-4′), 3.89–3.84 (m, 3H, OCHbH–CH
, H-5′, H-2′), 3.68 (dq, 1H, J = 8.8, 6.3 Hz, H-5), 3.66 (dd, 1H, J = 10.5, 4.3 Hz, H-6a′), 3.56 (dd, 1H, J = 10.5, 6.6 Hz, H-6b′), 3.55 (t, 1H, J = 8.8 Hz, H-4), 1.22 (d, 1H, J = 6.3 Hz, H-6), 0.97 (m, 21H, (CH3)2CH)3Si); 13C NMR (CDCl3, 125 MHz) δ 139.1, 139.0, 138.4, 138.3, 137.9 (Ar); 134.0 (CH
CH2); 128.3, 128.2, 128.1 (×2), 127.9, 127.8 (×2), 127.6, 127.4 (×2), 127.2, 127.1 (Ar); 117.1 (CH
CH2), 98.7 (C-1′), 96.8 (C-1), 85.0 (C-2′), 83.6 (C-4′), 79.9 (C-4), 78.9 (C-5′), 76.2 (C-3), 75.5 (C-2), 75.2 (C-3′), 74.4, 73.2, 72.7, 72.2 (PhCH2); 70.9 (C-6′), 68.1 (C-5), 67.9 (OCH2–CH
); 18.11, 18.08 (CH3)2CH)3Si); 17.9 (C-6), 12.5 (CH3)2CH)3Si); HRMS (ESI) calcd for (M + Na) C59H76O10NaSi: 995.5100. Found: 995.5130.
Allyl 2,5,6-tri-O-benzyl-α-D-galactofuranosyl-(1→2)-3,4-di-O-benzyl-α-L-rhamnopyranoside (4). To a solution of 36 (388 mg, 0.42 mmol) in CH2Cl2 (4.0 mL) cooled to 0 °C, was added 0.55 M NaOMe in methanol (1.5 mL, 0.84 mmol) and the mixture was stirred at rt. After 1.5 h, TLC examination showed disappearance of 36. The mixture was diluted with CH2Cl2 (100 mL), successively extracted with water (100 mL), 7% HCl (100 mL), water (100 mL), dried (Na2SO4), filtered and concentrated in vacuo. Purification of the residue by silica gel column chromatography (7
:
1 hexane–EtOAc) gave 4 (250 mg, 74%) as a colorless syrup. Rf 0.33 (7
:
3 hexane–EtOAc); [α]D +5.2 (c 0.8, CHCl3); 1H NMR (CDCl3, 500 MHz) δ 7.34–7.19 (m, 25H, ArH), 5.79 (dddd, 1H, J = 17.2, 10.4, 6.1, 5.1 Hz, CH
CH2), 5.22 (d, 1H, J = 4.3 Hz, H-1′), 5.19 (dq, 1H, J = 17.2, 1.6 Hz, HC
CHaH), 5.08 (dq, 1H, J = 10.4, 1.4 Hz, HC
CHbH), 4.98, 4.62 (2d, 2H, J = 11.4 Hz, PhCH2), 4.83 (d, 1H, J = 2.0 Hz, H-1), 4.74–4.68 (m, 4H, PhCH2), 4.63 (d, 1H, J = 11.9 Hz, PhCH2), 4.53 (d, 1H, J = 11.5 Hz, PhCH2), 4.31 (s, 2H, PhCH2), 4.34 (t, 1H, J = 7.8 Hz, H-3′), 4.29 (dd, 1H, J = 9.4, 3.1 Hz, H-3), 4.10 (ddt, 1H, J = 13.0, 5.1, 1.5 Hz, OCHaH–CH
), 4.01 (dd, 1H, J = 8.3, 4.3 Hz, H-2′), 3.97 (dd, 1H, J = 3.0, 2.0 Hz, H-2), 3.93 (dd, 1H, J = 8.5, 7.4 Hz, H-4′), 3.91 (ddt, 1H, J = 13.0, 6.1, 1.3 Hz, OCHbH-CH
), 3.74–3.68 (m, 2H, H-5′, H-5), 3.63 (t, 1H, J = 9.4 Hz, H-4), 3.37 (dd, 1H, J = 10.8, 5.3 Hz, H-6a′), 3.29 (dd, 1H, J = 10.8, 4.2 Hz, H-6b′), 1.31 (d, 3H, J = 6.2 Hz, H-6); 13C NMR (CDCl3, 125 MHz) δ 138.7 (×2), 138.2, 138.0, 137.0 (Ar); 133.9 (CH
CH2); 128.5, 128.3, 128.2, 128.0, 127.9 (×2), 127.8 (×2), 127.6 (×3), 127.5, 127.4, (Ar); 117.1 (CH
CH2), 97.1 (C-1′), 96.9 (C-1), 84.5 (C-4′), 83.1 (C-2′), 80.6 (C-5′), 79.9 (C-4), 74.9 (C-3′), 74.8 (C-3), 74.4 (C-2); 74.9, 73.8, 73.3, 72.7, 71.9 (PhCH2); 69.9 (C-6′), 68.2 (C-5), 67.8 (OCH2–CH
), 17.9 (C-6); HRMS (ESI) calcd for (M + Na) C50H56O10Na: 839.3766. Found: 839.3799.
Allyl 2,3,4,6-tetra-O-benzyl-α-D-glucopyranosyl-(1→3)-2,5,6-tri-O-benzyl-α-D-galactofuranosyl-(1→2)-3,4-di-O-benzyl-α-L-rhamnopyranoside (44). Compound 44 was obtained according to Method D from donor 5 (278 mg, 0.41 mmol) and acceptor 4 (278 mg, 0.41 mmol). The crude was purified by column chromatography (10
:
1 hexane–EtOAc) to afford 44 (408.1 mg, 99%), as a colorless syrup; Rf 0.51 (7
:
3 hexane–EtOAc); [α]D +36.9 (c 1.3, CHCl3); 1H NMR (CDCl3, 500 MHz) δ 7.33–7.08 (m, 45H, ArH), 5.81 (dddd, 1H, J = 17.1, 10.4, 6.1, 5.1 Hz, CH
CH2), 5.24 (d, 1H, J = 4.2 Hz, H-1′), 5.20 (dq, 1H, J = 17.1, 1.6 Hz, HC
CHaH), 5.19 (d, 1H, J = 3.5 Hz, H-1′′), 5.11 (dq, 1H, J = 10.4, 1.4 Hz, HC
CHbH), 4.94, 4.47 (2d, 2H, J = 10.9 Hz, PhCH2), 4.90, 4.76 (2d, 2H, J = 10.8 Hz, PhCH2), 4.82, 4.44 (2d, 2H, J = 11.0 Hz, PhCH2), 4.78 (d, 1H, J = 2.2 Hz, H-1), 4.66, 4.59 (2d, 2H, J = 11.0 Hz, PhCH2), 4.65 (s, 2H, PhCH2), 4.59, 4.49 (2d, 2H, J = 11.7 Hz, PhCH2), 4.52 (t, 1H, J = 6.9 Hz, H-3′), 4.45, 4.18 (2d, 2H, J = 12.1 Hz, PhCH2), 4.40, 4.39 (2d, 2H, J = 10.7 Hz, PhCH2), 4.32, 4.29 (2d, 2H, J = 12.0 Hz, PhCH2), 4.19–4.16 (m, 2H, H-3, H-4′), 4.10 (m, 3H, OCHaH–CH
, H-5′′, H-2′), 3.96 (t, 1H, J = 9.4 Hz, H-3′′), 3.95 (t, 1H, J = 2.7 Hz, H-2), 3.91 (ddt, 1H, J = 13.0, 6.1, 1.3 Hz, OCHbH–CH
), 3.81 (dt, 1H, J = 5.5, 3.3 Hz, H-5′), 3.76 (dd, 1H, J = 10.4, 3.3 Hz, H-6a′), 3.70–3.65 (m, 2H, H-4′′, H-5), 3.57 (dd, 1H, J = 10.4, 5.8 Hz, H-6b′), 3.54 (m, 2H, H-6a′′, H-4), 3.51 (dd, 1H, J = 9.7, 3.5 Hz, H-2′′), 3.36 (dd, 1H, J = 10.7, 1.8 Hz, H-6a′′), 1.26 (d, 3H, J = 6.3 Hz, H-6); 13C NMR (CDCl3, 125 MHz) δ 138.9, 138.7, 138.6, 138.5, 138.1, 138.0, 137.9, 137.8 (Ar); 133.9 (CH
CH2); 128.3 (×2), 128.2 (×5), 128.0, 127.9 (×2), 127.7 (×3), 127.6 (×2), 127.5, 127.4, 127.3 (Ar); 117.1 (CH
CH2), 98.5 (C-1′), 96.8 (C-1), 95.4 (C-1′′), 83.6 (C-2′), 81.9 (C-3′′), 81.1 (C-4′), 80.2 (C-4), 79.7 (C-2′′), 78.8 (C-5′), 77.5 (C-4′′, C-3′), 76.3 (C-3), 75.6 (C-2, PhCH2); 74.9, 73.3, 73.1, 72.8, 72.7, 72.6, 72.5 (PhCH2); 70.2 (C-5′′), 70.1 (C-6′), 68.2 (C-6′′), 68.0 (C-5), 67.8 (OCH2–CH
), 17.9 (C-6); HRMS (ESI) calcd for (M + Na) C84H90O15Na: 1361.6172. Found: 1361.6142.
2,3,4,6-Tetra-O-benzyl-α-D-glucopyranosyl-(1→3)-2,5,6-tri-O-benzyl-α-D-galactofuranosyl-(1→2)-3,4-di-O-benzyl-L-rhamnopyranoside (45). The same procedure as described for 14 was followed starting from 44 (140 mg, 0.11 mmol) and PdCl2 (6 mg, 0.034 mmol). Column chromatography (7
:
1 hexane–EtOAc) of the residue gave 45 (87.7 mg, 65%) as a colorless syrup (α
:
β 10
:
7); Rf 0.23 (7
:
3 hexane–EtOAc); [α]D +59.4 (c 0.8, CHCl3); 1H NMR (CDCl3, 500 MHz) δ 7.32–7.09 (m, 45H, ArH), 5.27 (d, 0.41H, J = 4.2 Hz, H-1′β), 5.25 (d, 0.59H, J = 4.3 Hz, H-1′α), 5.16–5.15 (m, 1.18H, H-1′′α, H-1α), 5.12 (d, 0.41H, J = 3.3 Hz, H-1′′β), 4.95–4.56 (m, 10.41H, PhCH2, H-1β), 4.54 (t, 0.41H, J = 7.4 Hz, H-3′β), 4.53 (t, 0.59H, J = 7.1 Hz, H-3′α), 4.51–4.31 (m, 8H, PhCH2), 4.22 (dd, 0.59H, J = 8.4, 3.2 Hz, H-3α), 4.20–4.13 (m, 2.41H, H-4′α, H-4′β, H-2′β, PhCH2), 4.11 (dd, 0.59H, J = 7.5, 4.2 Hz, H-2′α), 4.10–4.05 (m, 1H, H-5′′α, H-5′′β), 3.96 (t, 0.41H, J = 9.5 Hz, H-3′′β), 3.95 (t, 0.59H, J = 9.4 Hz, H-3′′α), 3.93 (t, 0.59H, J = 2.8 Hz, H-2α), 3.89–3.83 (m, 0.59H, H-5α), 3.85 (dd, 0.41H, J = 3.2, 2.1 Hz, H-2β), 3.81–3.74 (m, 2H, H-5′α, H-5′β, H-6a′α, H-6a′β), 3.69 (m, 1.41H, H-4′′α, H-4′′β, H-3β), 3.63–3.45 (m, 4H, H-4α, H-4β, H-6b′α, H-6b′β, H-6a′′α, H-6a′′β, H-2′′α, H-2′′β), 3.35 (m, 1H, H-6b′′α, H-6b′′β), 3.27 (dq, 0.41H, J = 7.3, 5.1 Hz, H-5β), 1.26 (d, 1.23H, J = 6.4 Hz, H-6β), 1.24 (d, 1.77H, J = 6.3 Hz, H-6α); 13C NMR (CDCl3, 125 MHz) δ 138.9, 138.7, 138.6, 138.4, 138.1, 138.0, 137.9, 137.8 (×2), 128.6, 128.5, 128.4, 128.3 (×2), 128.2 (×4), 128.1, 128.0, 127.9 (×2), 127.8 (×2), 127.7 (×2), 127.6 (×2), 127.5, 127.4 (×2) (Ar); 100.7 (C-1′β), 98.6 (C-1′α), 95.7 (C-1′′β), 95.5 (C-1′′α), 93.2 (C-1β), 92.5 (C-1α); 83.7, 83.4 (C-2′β, C-2′α); 81.91, 81.96 (C-3′′α, C-3′′β); 81.0, 80.8 (C-4′α, C-4′β), 80.1 (C-4α), 79.8 (C-3β); 79.8, 79.7 (C-2′′α, C-2′′β, C-4β); 78.6, 78.3 (C-5′α, C-5′β); 77.5 (C-4′′α, C-4′′β); 77.4, 76.9 (C-3′α, C-3′β), 76.4 (C-2β), 75.9 (C-2α), 75.6 (C-3α), 74.9, 74.5, 74.3, 73.5, 73.3, 73.2, 73.1, 72.9, 72.8, 72.7, 72.4 (PhCH2); 71.2 (C-5β), 70.2 (C-5′′α, C-5′′β), 70.0 (C-6′α, C-6′β), 68.5 (C-5α), 68.2 (C-6′′α, C-6′′β), 18.3 (C-6β), 18.0 (C-6α); HRMS (ESI) calcd for (M + Na) C81H86O15Na: 1321.5859. Found: 1321.5866.
α-D-Glucopyranosyl-(1→3)-α-D-galactofuranosyl-(1→2)-L-rhamnopyranoside (3). A suspension of 45 (33 mg, 0.029 mmol) dissolved in CH3OH (6 mL) and 10% Pd(C) (40 mg) was hydrogenated for 5 h at 40 psi (3 atm) and rt. The catalyst was filtered over Celite and the filtrate was concentrated at 25 °C. Purification of the residue by C-8 cartridge (H2O) gave 3 (25.9 mg, 93%) as a glassy hygroscopic solid, as 2
:
1 α/β anomeric mixture; Rf 0.44 (7
:
1
:
1 n-propanol–MeOH–H2O); [α]D +140.1 (c 1.1, H2O); 1H NMR (D2O, 500 MHz) δ 5.17 (d, 0.35H, J = 4.2 Hz, H-1′β), 5.15 (d, 0.65H, J = 4.3 Hz, H-1′α), 5.13 (d, 0.65H, J = 1.9 Hz, H-1α), 5.01 (d, 1H, J = 3.8 Hz, H-1′′), 4.86 (d, 0.35H, J = 1.0 Hz, H-1β), 4.37 (t, 1H, J = 7.4 Hz, H-3′), 4.35 (dd, 1H, J = 8.0, 4.5 Hz, H-2′), 4.14–4.11 (m, 1H, H-4′α, H-4′β), 4.07 (d, 0.35H, J = 3.1 Hz, H-2β), 4.05 (dd, 0.65H, J = 2.9, 1.9 Hz, H-2α), 3.92 (dq, 0.65H, J = 9.5, 6.2 Hz, H-5α), 3.89–3.85 (m, 2H, H-5′′, H-6a′′), 3.84 (dd, 0.65H, J = 6.4, 2.9 Hz, H-3α), 3.82–3.77 (m, 2H, H-5′, H-6b′′), 3.72 (t, 1H, J = 9.5 Hz, H-3′′), 3.69–3.59 (m, 2.35H, H-3β, H-6a′, H-6b′), 3.57 (dd, 1H, J = 9.9, 3.8 Hz, H-2′′), 3.55 (t, 0.65H, J = 9.7 Hz, H-4α), 3.50–3.42 (m, 1.65H, H-4β, H-4′′, H-5β), 1.31 (d, 1.05H, J = 5.8 Hz, H-6β), 1.30 (d, 1.95H, J = 6.3 Hz, H-6α); 13C NMR (D2O, 125 MHz) δ 100.4 (C-1′α), 100.0 (C-1′β), 99.6 (C-1′′), 94.2 (C-1α), 94.1 (C-1β); 81.5, 81.3 (C-3′α, C-3′β); 80.8, 80.7 (C-4′α, C-4′β); 80.3 (C-3β), 78.2 (C-3α); 75.54, 75.51 (C-2′α, C-2′β); 73.5 (C-3′′), 72.71, 72.68 (C-5′′β, C-5′′α), 72.4 (C-5β), 71.9 (C-2′′); 71.54, 71.52 (C-5′β, C-5′α), 71.2 (C-4α), 70.9 (C-4β); 70.1, 70.0 (C-4′′β, C-4′′α); 69.3 (C-2β), 69.0 (C-2α), 68.8 (C-5α), 63.3 (C-6′); 61.0, 60.9 (C-6′′β, C-6′′α); 17.58, 17.56 (C-6); HRMS (ESI) calcd for (M + Na) C18H32O15Na: 511.1633. Found: 511.1635.
Acknowledgements
This work was supported by grants from Agencia Nacional de Promoción Científica y Tecnológica, Universidad de Buenos Aires and CONICET. C. Gallo-Rodriguez is a research member of CONICET.
References
- Essentials of Glycobiology, ed. A. Varki, R. D. Cummings, J. D. Esko, H. H. Freeze, P. Stanley, C. R. Bertozzi, G. W. Hart and M. E. Etzler, Cold Spring Harbor Laboratory Press, New York, 2nd edn, 2009 Search PubMed.
- T. J. Boltje, T. Buskas and G.-J. Boons, Nat. Chem., 2009, 1, 611–622 CrossRef CAS PubMed.
- P. Stallforth, B. Lepenies, A. Adibekian and P. H. Seeberger, J. Med. Chem., 2009, 52, 5561–5577 CrossRef CAS PubMed.
- X. Zhu and R. R. Schmidt, Angew. Chem., Int. Ed., 2009, 48, 1900–1934 CrossRef CAS PubMed.
- L. K. Mydock and A. V. Demchenko, Org. Biomol. Chem., 2010, 8, 497–510 CAS.
- D. Crich, Acc. Chem. Res., 2010, 43, 1144–1153 CrossRef CAS PubMed.
- I. Cumpstey, Org. Biomol. Chem., 2012, 10, 2503–2508 CAS.
- D. Crich, J. Org. Chem., 2011, 76, 9193–9209 CrossRef CAS PubMed.
- A. Imamura and T. Lowary, Trends Glycosci. Glycotechnol., 2011, 23, 134–152 CrossRef CAS.
- A. Imamura and T. L. Lowary, Org. Lett., 2010, 12, 3686–3689 CrossRef CAS PubMed.
- X. Zhu, S. Kawatkar, Y. Rao and G.-J. Boons, J. Am. Chem. Soc., 2006, 128, 11948–11957 CrossRef CAS PubMed.
- D. Crich, C. M. Pedersen, A. A. Bowers and D. J. Wink, J. Org. Chem., 2007, 72, 1553–1565 CrossRef CAS PubMed.
- P.-H. Tam and T. L. Lowary, Curr. Opin. Chem. Biol., 2009, 13, 618–625 CrossRef CAS PubMed.
- A. Ishiwata and Y. Ito, J. Am. Chem. Soc., 2011, 133, 2275–2291 CrossRef CAS PubMed.
- D. Kaur, M. E. Guerin, H. Škovierová, P. J. Brennan and M. Jackson, in Adv. Appl. Microbiol., ed. I. L. Allen, S. Sima and M. G. Geoffrey, Academic Press, 2009, vol. 69, pp. 23–78 Search PubMed.
- J. C. Richards, M. B. Perry and P. J. Kniskern, Can. J. Chem., 1989, 67, 1038–1050 CrossRef CAS PubMed.
- M. Linnerborg, R. Wollin and G. Widmalm, Eur. J. Biochem., 1997, 246, 565–573 CAS.
- R. Stenutz, A. Weintraub and G. Widmalm, FEMS Microbiol. Rev., 2006, 30, 382–403 CrossRef CAS PubMed.
- A. V. Perepelov, L. Dan, L. Bin, S. y. N. Senchenkova, G. Dan, A. S. Shashkov, F. Lu, Y. A. Knirel and W. Lei, Innate Immun., 2011, 17, 164–173 CrossRef CAS PubMed.
- A. V. Perepelov, B. Liu, S. y. N. Senchenkova, A. S. Shashkov, L. Feng, Y. A. Knirel and L. Wang, Carbohydr. Res., 2011, 346, 373–376 CrossRef CAS PubMed.
- E. L. Zdorovenko, O. A. Valueva, L. D. Varbanets, V. V. Shubchinskiy, A. S. Shashkov and Y. A. Knirel, Carbohydr. Res., 2010, 345, 1812–1815 CrossRef CAS PubMed.
- O. Ahrazem, A. Prieto, G. San-Blas, J. A. Leal, J. Jimenez-Barbero and M. Bernabe, Glycobiology, 2003, 13, 743–747 CrossRef CAS PubMed.
- C. Marino, C. Gallo-Rodriguez and R. M. de Lederkremer, in Glycans: Biochemistry, Characterization and Applications, ed. H. M. Mora Montes, Nova Science, 2012, pp. 207–268 Search PubMed.
- M. R. Richards and T. L. Lowary, ChemBioChem, 2009, 10, 1920–1938 CrossRef CAS PubMed.
- P. Peltier, R. Euzen, R. Daniellou, C. Nugier-Chauvin and V. Ferrieres, Carbohydr. Res., 2008, 343, 1897–1923 CrossRef CAS PubMed.
- B. Tefsen, A. F. Ram, I. van Die and F. H. Routier, Glycobiology, 2012, 22, 456–469 CrossRef CAS PubMed.
- Y. J. Lee, B.-Y. Lee, H. B. Jeon and K. S. Kim, Org. Lett., 2006, 8, 3971–3974 CrossRef CAS PubMed.
- J. Y. Baek, Y. J. Joo and K. S. Kim, Tetrahedron Lett., 2008, 49, 4734–4737 CrossRef CAS PubMed.
- Y. Bai and T. L. Lowary, J. Org. Chem., 2006, 71, 9658–9671 CrossRef CAS PubMed.
- Y. Bai and T. L. Lowary, J. Org. Chem., 2006, 71, 9672–9680 CrossRef CAS PubMed.
- M. Gelin, V. Ferrières, M. Lefeuvre and D. Plusquellec, Eur. J. Org. Chem., 2003, 1285–1293 CrossRef CAS.
- M. J. Tilve and C. Gallo-Rodriguez, Carbohydr. Res., 2011, 346, 2838–2848 CAS.
- J.-S. Thomann, F. Monneaux, G. Creusat, M. V. Spanedda, B. Heurtault, C. Habermacher, F. Schuber, L. Bourel-Bonnet and B. Frisch, Eur. J. Med. Chem., 2012, 51, 174–183 CrossRef CAS PubMed.
- M. Gelin, V. Ferrieres and D. Plusquellec, Carbohydr. Lett., 1997, 2, 381–388 CAS.
- L. Gandolfi-Donadio, G. Gola, R. M. de Lederkremer and C. Gallo-Rodriguez, Carbohydr. Res., 2006, 341, 2487–2497 CrossRef CAS PubMed.
- S. Ghosh and A. K. Misra, Tetrahedron: Asymmetry, 2010, 21, 2755–2761 CrossRef CAS PubMed.
- G. Gola, M. J. Tilve and C. Gallo-Rodriguez, Carbohydr. Res., 2011, 346, 1495–1502 CrossRef CAS PubMed.
- K. L. O'Brien, L. J. Wolfson, J. P. Watt, E. Henkle, M. Deloria-Knoll, N. McCall, E. Lee, K. Mulholland, O. S. Levine and T. Cherian, Lancet, 2009, 374, 893–902 CrossRef.
- WHO, Pneumococcal vaccines-WHO Position Paper 2012, Weekly Epidemiological Record, 2012, vol. 87, pp. 129–144 Search PubMed.
- P. E. Bratcher, K.-H. Kim, J. H. Kang, J. Y. Hong and M. H. Nahm, Microbiology, 2010, 156, 555–560 CrossRef CAS PubMed.
- K. P. Klugman, Lancet Infect. Dis., 2001, 1, 85–91 CrossRef CAS.
- M. R. Jacobs, C. E. Good, S. Bajaksouzian and A. R. Windau, Clin. Infect. Dis., 2008, 47, 1388–1395 CrossRef PubMed.
- S. Duggan, Drugs, 2010, 70, 1973–1986 CrossRef CAS PubMed.
- B. Pichon, S. N. Ladhani, M. P. Slack, A. Segonds-Pichon, N. J. Andrews, P. A. Waight, E. Miller and R. George, J. Clin. Microbiol., 2013, 51, 820–827 CrossRef CAS PubMed.
- J. M. Skinner, L. Indrawati, J. Cannon, J. Blue, M. Winters, J. MacNair, N. Pujar, W. Manger, Y. Zhang, J. Antonello, J. Shiver, M. Caulfield and J. H. Heinrichs, Vaccine, 2011, 29, 8870–8876 CrossRef CAS PubMed.
- I. C. Skovsted, M. B. Kerrn, J. Sonne-Hansen, L. E. Sauer, A. K. Nielsen, H. B. Konradsen, B. O. Petersen, N. T. Nyberg and J. Ø. Duus, Vaccine, 2007, 25, 6490–6500 CrossRef CAS PubMed.
- L. Morelli, L. Poletti and L. Lay, Eur. J. Org. Chem., 2011, 5723–5777 CrossRef CAS.
- J. P. Kamerling, in Carbohydrate Chemistry: Volume 37, ed. A. P. Rauter and T. Lindhorst, The Royal Society of Chemistry, 2012, vol. 37, pp. 117–159 Search PubMed.
- A. M. P. van Steijn, J. P. Kamerling and J. F. G. Vliegenthart, J. Carbohydr. Chem., 1992, 11, 665–689 CrossRef CAS.
- M. L. Wolfrom, Z. Yosizawa and B. O. Juliano, J. Org. Chem., 1959, 24, 1529–1530 CrossRef CAS.
- R. R. Schmidt and J. Michel, Tetrahedron Lett., 1984, 25, 821–824 CrossRef CAS.
- R. R. Schmidt, J. Michel and M. Roos, Liebigs Ann. Chem., 1984, 1343–1357 CrossRef CAS.
- P. Westerduin, P. E. de Haan, M. J. Dees and J. H. van Boom, Carbohydr. Res., 1988, 180, 195–205 CrossRef CAS.
- G. A. Kashiwagi, V. M. Mendoza, R. M. de Lederkremer and C. Gallo-Rodriguez, Org. Biomol. Chem., 2012, 10, 6322–6332 CAS.
- L. Gandolfi-Donadio, M. Santos, R. M. de Lederkremer and C. Gallo-Rodriguez, Org. Biomol. Chem., 2011, 9, 2085–2097 CAS.
- G. Gola, P. Libenson, L. Gandolfi-Donadio and C. Gallo-Rodriguez, Arkivoc, 2005, xii, 234–242 Search PubMed.
- T. W. Greene and P. G. M. Wuts, in Protective Groups in Organic Synthesis, Wiley-Interscience, 4th edn, 2006 Search PubMed.
- S.-Y. Zhu and J.-S. Yang, Tetrahedron, 2012, 68, 3795–3802 CrossRef CAS PubMed.
- R. R. Schmidt and A. Toepfer, Tetrahedron Lett., 1991, 32, 3353–3356 CrossRef CAS.
- R. R. Schmidt and X. Zhu, in Glycoscience, ed. B. Fraser-Reid, K. Tatsuta and J. Thiem, Springer, 2008, pp. 451–453 Search PubMed.
- Y. J. Lee, K. Lee, E. H. Jung, H. B. Jeon and K. S. Kim, Org. Lett., 2005, 7, 3263–3266 CrossRef CAS PubMed.
- J. Zhang and F. Kong, Tetrahedron, 2003, 59, 1429–1441 CrossRef CAS.
- K. S. Kim and D. H. Suk, in Topics in Current Chemistry, Reactivity Tuning in Oligosaccharide Assembly, ed. B. Fraser-Reid and J. C. López, Springer, Heidelberg, 2011, vol. 301, pp.109–140 Search PubMed.
- J. Y. Baek, B.-Y. Lee, M. G. Jo and K. S. Kim, J. Am. Chem. Soc., 2009, 131, 17705–17713 CrossRef CAS PubMed.
- J. Guo and X.-S. Ye, Molecules, 2010, 15, 7235–7265 CrossRef CAS PubMed.
- H. Premathilake and A. Demchenko, in Topics in Current Chemistry, Reactivity Tuning in Oligosaccharide Assembly, ed. B. Fraser-Reid and J. C. López, Springer, Heidelberg, 2011, vol. 301, pp. 189–221 Search PubMed.
- T. H. Schmidt and R. Madsen, Eur. J. Org. Chem., 2007, 3935–3941 CrossRef CAS.
- A. V. Demchenko, E. Rousson and G.-J. Boons, Tetrahedron Lett., 1999, 40, 6523–6526 CrossRef CAS.
Footnote |
† Electronic supplementary information (ESI) available: Experimental for 25–35. 1H and 13C NMR spectra for 3, 4, 6, 8–19, 21–45; and bidimensional spectra for 3, 19, and 42. See DOI: 10.1039/c3ra45658g |
|
This journal is © The Royal Society of Chemistry 2014 |