DOI:
10.1039/C4QO00159A
(Research Article)
Org. Chem. Front., 2014,
1, 940-946
Titanium-mediated cross-coupling reactions of 1,3-butadiynes with α-iminonitriles to 3-aminopyrroles: observation of an imino aza-Nazarov cyclization†
Received
28th May 2014
, Accepted 15th July 2014
First published on 16th July 2014
Abstract
Ti(OiPr)4/2 nBuLi-mediated highly efficient cross-coupling reactions of 1,3-butadiynes with α-iminonitriles are described. The method provides convenient access to functionalized 3-aminopyrroles with a wide diversity of substituents in a highly regioselective manner. When optically pure α-iminonitrile is employed in this reaction, a chiral pyrrole derivative is obtained without loss of enantiopurity. Mechanistic study indicates that the 3-aminopyrroles are formed upon treatment of the crude hydrolysis products with silica gel or Lewis acid. A novel imino aza-Nazarov cyclization reaction is proposed to account for the formation of the pyrrole products. Employing the titanium-monoyne complex in this reaction resulted in the homo-coupling of α-iminonitriles, leading to the formation of 1,2-diimines.
3-Aminopyrroles are found in a variety of biologically active substances or natural products,1 and are also useful building blocks in synthetic chemistry.2 For example, as shown in Fig. 1, natural products such as distamycin and netropsin with 3-aminopyrrole skeletons are well-known DNA-minor groove binding agents with a strong preference for adenine/thymine-rich sequences.3 The 3-aminopyrrole alkaloid solsodomine A, isolated from fresh berries of Solanum sodomaeum L., shows activity against Mycobacterium intracellulare.4 3-Aminopyrrole-2-carboxylates, such as “a”, were identified as common pharmacophores which exhibit potent anticonvulsant activity by blocking sodium channels in a frequency-dependent manner.5 Although a large number of pyrrole syntheses have been reported in recent years, efficient synthetic routes to 3-aminopyrroles are quite limited.6–9 General synthetic methods include: functional group transformation of pyrrole derivatives such as 3-nitropyrrole,6a–d 3-arylazopyrroles,6e pyrrol-3-carboxylic acids,6fetc.,7 and intramolecular cyclization of enaminonitrile derivatives8 such as Thorpe–Ziegler type cyclization,8e–jetc.9 However, these methods usually are restricted to special substituted substrates. Thus, the development of new methodologies using readily available starting materials is highly desired. On the other hand, titanium-mediated cross-coupling reactions have been proved to be highly efficient for the construction of carbon–carbon or carbon–heteroatom bonds, and the thus generated titanacycles are important intermediates for further selective transformations.10 During our continued studies on the chemistry of titanium-butadiyne complexes,11 we have developed the coupling reactions of titanium-butadiyne complexes with aldehydes or ketones, leading to the selective synthesis of stereodefined trans-enynols11a or trisubstituted [3]cumulenols.11b Inspired by these results, we were next interested in the potential coupling reactions of titanium-butadiyne complexes with functionalized nitriles. In this paper, we report titanium-mediated cross-coupling reactions of 1,3-butadiynes with α-iminonitriles, which provide a convenient one-pot synthesis of functionalized 3-aminopyrroles upon treatment of the crude hydrolysis products with silica gel or Lewis acid (Scheme 1). Interestingly, 3-aminopyrroles were formed, likely via a rare imino aza-Nazarov cyclization of the diimines produced by hydrolysis of the in situ generated azatitanacycles.
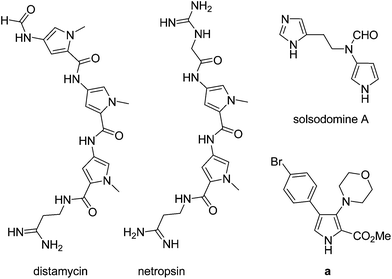 |
| Fig. 1 Representative examples of bioactive 3-aminopyrrole derivatives. | |
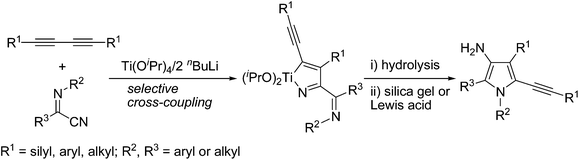 |
| Scheme 1 | |
The reactivity of nitriles with group 4 metal complexes has been intensively studied during the last few decades, in which C
N insertion into a metal–carbon bond12 or a heterobimetallic complex,13 and formation of metal–keteniminate species14 have been observed. However, so far only one report, by Sato et al., related to titanium-mediated cross-coupling reactions of nitriles (restrict to α-heterofunctionalized nitriles) with alkynes has been precedented.15 They demonstrated that azatitanacyclopentadienes formed by cross-coupling reactions of alkynes with α-methoxyacetonitriles could react with aldehydes, sulfonylacetylenes or second α-methoxyacetonitrile to afford furans, pyridines and pyrroles, respectively.15 The α-iminonitriles (imidoyl cyanides) are important synthetic precursors for the synthesis of α-keto acids, diimines, amides, amidines, etc.,16 and also serve as effective components in various cycloaddition reactions.17 Due to the enhanced reactivities of C
N and C
N bonds, both of these two unsaturated moieties in α-iminonitriles might couple with alkynes in the presence of low-valent titanium species. We were therefore quite interested in exploring new reaction patterns using these substrates. α-Iminonitriles can be readily prepared by the following two methods (Scheme 2): IBX/TBAB-mediated oxidative Strecker reaction (method a)18 and the reactions of imidoyl chlorides with CuCN (method b). Initially, we investigated the titanium-mediated coupling reactions of 1,4-bis(tert-butyldimethylsilyl)buta-1,3-diyne 1a with (Z)-N-(4-methoxyphenyl)benzimidoyl cyanide 3a. The results are shown in Table 1. Based on our previous studies,11 titanium-butadiyne complex 2a (also referred to as alkynyltitanacyclopropene) was prepared in situ by the reaction of diyne 1a with 1.3 equiv. of the Ti(OiPr)4/2 nBuLi reagent in THF. Addition of 1.1 equiv. of 3a to the mixture containing 2a at −78 °C and stirring for 2 h resulted in the formation of 5-alkynyl-3-aminopyrrole 4a in 47% yield after quenching the mixture with 3 M HCl followed by silica gel purification. To our delight, the yield of 4a could be improved to 72% by quenching the reaction mixture with saturated aqueous NaHCO3 solution. It was noted that in this case, in addition to 4a, one more byproduct could be detected in the crude reaction mixture. However, after the crude material was dissolved in CH2Cl219 followed by addition of silica gel and evaporation of the solvent, TLC analysis showed that only 4a remained. The results indicated that the observed byproduct converted to 4a on the silica gel. This conclusion was also confirmed by control experiments (vide infra). With the optimized reaction conditions in hand, the substrate scope of this reaction was examined (Table 1). We first investigated the effect of the substituents on α-iminonitriles. When N-phenyl- or N-(p-bromophenyl)-substituted iminonitriles were employed, the corresponding 3-aminopyrroles 4b and 4c were obtained in 88% and 85% yields, respectively. The results indicated that the presence of electron-neutral or withdrawing N-aryl group on iminonitrile afforded higher product yields. N-Alkyl-substituted iminonitriles such as N-(R)-(1-phenylethyl) or N-cyclohexyl-substituted ones were also examined. Although the substrates with iminonitriles could occur at −78 °C, the desired products were not observed after silica gel purification. The results suggested that the silica gel was not effective in promoting the transformations to pyrroles in these cases. To our delight, further optimizations indicated that when the crude products obtained by quenching with saturated aqueous NaHCO3 solution followed by normal work-up were stirred at 50 °C in THF for 1 h in the presence of 4.0 equiv. of BF3·Et2O, the desired products 4d and 4e could be obtained in 73% and 75% yields, respectively. It was noted that the use of enantiomerically pure N-(R)-(1-phenylethyl)-substituted iminonitrile gave 4d without racemization, as determined by chiral-column HPLC analysis of 4d and (±)-4d. The iminonitriles bearing p-Cl, p-CF3, 3,4,5-(MeO)3 and 1-naphthyl substituents on the C-aryl rings (R3 group) reacted smoothly with 1a to afford 4e–4h in 72–84% yields. C-Heteroaryl-substituted iminonitriles such as (5-methyl-2-furanyl) or (2-thienyl)-substituted ones were tolerated well during the reaction to afford 4i and 4j in 73% and 79% yields, respectively. The reaction was also compatible with C-alkyl substituted iminonitrile, furnishing 4k in 55% yield. Next, we made an effort to employ aryl- or alkyl-substituted 1,3-butadiynes as the diyne substrates. As shown in Table 1, when phenyl or p-tolyl-substituted butadiynes were used as the coupling components, the corresponding products 4l–4n could be formed in 42–48% yields by treatment of the crude products with BF3·Et2O in CH2Cl2 at room temperature. 4l–4n could also be formed without the need to use Lewis acid. The use of alkyl-substituted butadiynes such as Hex–C
C–C
C–Hex and tBuC
C–C
CtBu were also suited for this reaction, furnishing the pyrrole products 4o and 4p in 39% and 55% yields, respectively. One of the reasons for the lower yields of 4l–4p derived from aryl- or alkyl-substituted butadiynes might be due to the lower yields of the first step for the formation of the corresponding titanium-butadiyne complexes.11a The structure and the regioselectivity of 3-aminopyrroles 4 were unambiguously confirmed by X-ray crystallographic analyses of the product 4a (Fig. 2) and the N-tosylated derivatives of 4l and 4p.20
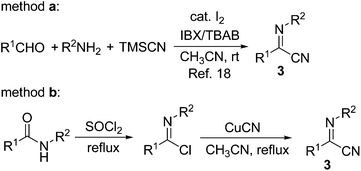 |
| Scheme 2 | |
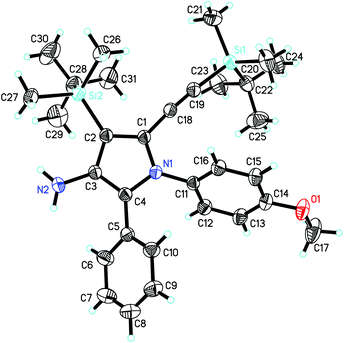 |
| Fig. 2 X-ray crystal structure of 3-aminopyrrole 4a. | |
Table 1 Synthesis of 3-aminopyrroles from 1,3-butadiynes and α-iminonitrilesa
Isolated yields.
The crude products were treated with BF3·Et2O.
−78 °C to 0 °C, 3–5 h.
|
|
To understand the reaction mechanism, we tried to isolate the possible intermediate before treating the crude products with silica gel. A diimine product 5, derived from the coupling reaction of titanium-butadiyne complex 2a with N-(4-bromophenyl)-3,4,5-trimethoxybenzimidoyl cyanide 3g, was found to be stable upon isolation through neutral Al2O3. The structure of 5 was also confirmed by X-ray crystallographic analysis.205 converted to 3-aminopyrrole 4g in high yield of 85% upon loading on silica gel followed by column chromatography. The results indicated that 3-aminopyrrole 4 was formed during the silica gel purification process (Scheme 3).
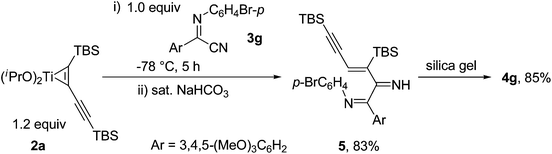 |
| Scheme 3 | |
Based on the above results, we propose the following reaction mechanism for this novel transformation using butadiyne as the substrate (Scheme 4): First, the addition of the C
N moiety of iminonitrile to the less hindered propargyl titanium moiety in complex 2via SE2′-type21,22 reaction gives an azatitanacyclocumulene 7, which might isomerize to azatitanacyclopentadiene 8. 8 might also be formed by direct insertion of a CN bond into the Ti–C(sp2) bond close to the R1 group of 2. However, the attack of the nitrile from this side may encounter large steric hindrance. Hydrolysis of 8 affords the diimine product 5. Further transformation of 5 to 3-aminopyrroles 4 might proceed via an interesting imino aza-Nazarov cyclization promoted by silica gel due to its weak Lewis acidity.23 As we know, imino24 and aza-Nazarov25 cyclizations are variations of the classical Nazarov reaction involving 4π electrocyclizations. Until now, the reports for imino-type Nazarov cyclizations are quite limited.24 Calculations by Smith et al. indicated that the 3-imino-type Nazarov reaction is energetically disfavored due to the stabilization of 3-aminopentadienyl cation over the cyclic allylic cation by conjugation with the lone pair electrons on the nitrogen atom (Scheme 5).26 To the best of our knowledge, the imino aza-Nazarov cyclization has only been reported as a postulated reaction step in a diamine monotriflate-catalyzed asymmetric cyclization of ketoazirines.27 In our case, the allylic cation 11 could be more favored due to the stronger stabilization by an adjacent nitrogen atom on the NR2 group.
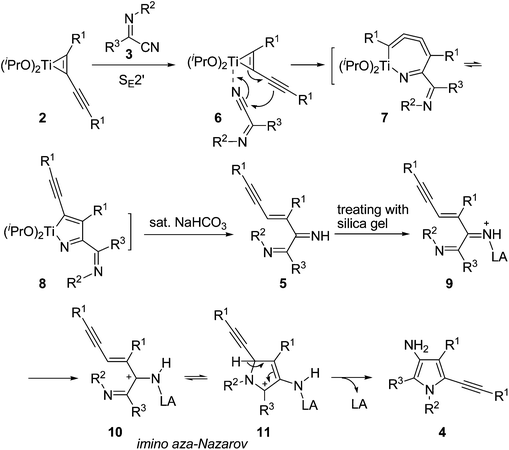 |
| Scheme 4 | |
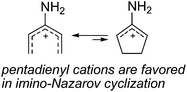 |
| Scheme 5 | |
We also investigated the reactions of titanium-monoyne complexes with α-iminonitriles (Scheme 6). Interestingly, the expected 3-aminopyrroles were not obtained, instead, the diimine 15 was obtained in 59% yield, along with 74% of diphenylacetylene, after quenching the reaction mixture with water. In addition, one more byproduct was also observed, the structure of which was not defined yet. The reaction might proceed by first ligand exchange to give a titanium-imine complex 13, which undergoes homo-coupling with another α-iminonitrile to give 14 by insertion of the C
N moiety to azatitanacycle 13. This is followed by elimination of CN groups to deliver the desired product 15. Diimines are valuable ligands in transition-metal chemistry,28 for example, the diimine-Ni complex shows high catalytic activity in olefin polymerization.28b,c Our reaction provides a simple and efficient method for the synthesis of diimines.
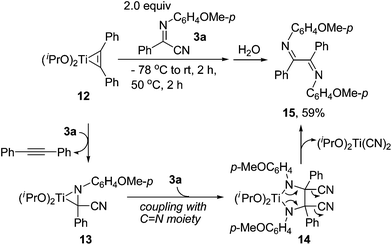 |
| Scheme 6 | |
In summary, we have developed a titanium-mediated regioselective cross-coupling reaction of 1,3-butadiynes with α-iminonitriles. Functionalized 3-aminopyrroles were efficiently constructed upon treatment of the crude hydrolysis products with silica gel or Lewis acid. The reaction likely proceeds via a novel imino aza-Nazarov type cyclization of the diimines produced by hydrolysis of the in situ generated azatitanacycles. Employing titanium-monoyne complex in this reaction resulted in homo-coupling of α-iminonitriles, leading to the formation of 1,2-diimines. Further studies to expand the reaction scope are in progress.
Acknowledgements
We thank the National Natural Science Foundation of China (grant no. 21125210, 21121062), Chinese Academy of Science and the Major State Basic Research Development Program (grant no. 2011CB808700) for financial support.
Notes and references
- For selected recent reviews and papers, see:
(a) T. Bando and H. Sugiyama, Acc. Chem. Res., 2006, 39, 935 CrossRef CAS PubMed;
(b) P. G. Baraldi, D. Preti, F. Fruttarolo, M. A. Tabrizi and R. Romagnoli, Bioorg. Med. Chem., 2007, 15, 17 CrossRef CAS PubMed;
(c) P. G. Baraldi, A. N. Zaid, D. Preti, F. Fruttarolo, M. A. Tabrizi, A. Iaconinoto, M. G. Pavani, M. D. Carrion, C. L. Cara and R. Romagnoli, ARKIVOC, 2006, vii, 20 Search PubMed;
(d) K. Wakabayashi, H. Miyachi, Y. Hashimoto and A. Tanatani, Bioorg. Med. Chem., 2005, 13, 2837 CrossRef CAS PubMed;
(e) M. T. Cocco, C. Congiu and V. Onnis, Bioorg. Med. Chem., 2003, 11, 495 CrossRef CAS PubMed.
- For selected papers, see:
(a) D. A. Chenoweth, D. A. Harki and P. B. Dervan, J. Am. Chem. Soc., 2009, 131, 7175 CrossRef CAS PubMed;
(b) G. Fridkin and W. D. Lubell, Org. Lett., 2008, 10, 849 CrossRef CAS PubMed;
(c) H. Bekolo and G. Kirsch, Can. J. Chem., 2007, 85, 1 CrossRef CAS;
(d) C. Rochais, V. Lisowski, P. Dallemagne and S. Rault, Bioorg. Med. Chem., 2006, 14, 8162 CrossRef CAS PubMed;
(e) E. Patanè, V. Pittalà, F. Guerrera, L. Salerno, G. Romeo, M. A. Siracusa, F. Russo, F. Manetti, M. Botta, I. Mereghetti, A. Cagnotto and T. Mennini, J. Med. Chem., 2005, 48, 2420 CrossRef PubMed;
(f) B. K. S. Yeung and D. L. Boger, J. Org. Chem., 2003, 68, 5249 CrossRef CAS PubMed.
- C. Bailly and J. B. Chaires, Bioconjugate Chem., 1998, 9, 513 CrossRef CAS PubMed.
- K. A. El-Sayed, M. T. Hamann, H. A. A. El-Rahman and A. M. Zaghloul, J. Nat. Prod., 1998, 61, 848 CrossRef CAS PubMed.
- K. Unverferth, J. Engel, N. Höfgen, A. Rostock, R. Günther, H. J. Lankau, M. Menzer, A. Rolfs, J. Liebscher, B. Müller and H. J. Hofmann, J. Med. Chem., 1998, 41, 63 CrossRef CAS PubMed.
-
(a) M. Valík, J. Malina, L. Palivec, J. Foltýnová, M. Tkadlecová, M. Urbanová, V. Brabec and V. Král, Tetrahedron, 2006, 62, 8591 CrossRef;
(b) M. D. Rosa and D. Arnold, J. Org. Chem., 2013, 78, 1107 CrossRef PubMed;
(c) L. Fu and G. W. Gribble, Synthesis, 2008, 788 CAS;
(d) L. Fu and G. W. Gribble, Tetrahedron Lett., 2008, 49, 3545 CrossRef CAS;
(e) G. Dattolo, G. Cirrincione, A. M. Almerico, G. Presti and E. Aiello, Heterocycles, 1984, 22, 2269 CrossRef CAS;
(f) F. Fabis, P. Dallemagne, S. Rault and M. Robba, Org. Prep. Proced. Int., 1995, 27, 236 CrossRef CAS.
-
(a) Y. Brouillette, F. J. R. Rombouts and W. D. Lubell, J. Comb. Chem., 2006, 8, 117 CrossRef CAS PubMed;
(b) F.-A. Marcotte and W. D. Lubell, Org. Lett., 2002, 4, 2601 CrossRef CAS PubMed;
(c) M. Nagarajan and H. Shechter, J. Org. Chem., 1984, 49, 62 CrossRef CAS.
-
(a) T. Murata and K. Ukawa, Chem. Pharm. Bull., 1974, 22, 240 CrossRef CAS;
(b) T. Murata, T. Sugawara and K. Ukawa, Chem. Pharm. Bull., 1978, 26, 3080 CrossRef CAS;
(c) E. Bandini, G. Martelli, G. Spunta, A. Bongini, M. Panunzio and G. Piersanti, Tetrahedron: Asymmetry, 1999, 10, 1445 CrossRef CAS;
(d) T. Sasada, T. Sawada, R. Ikeda, N. Sakai and T. Konakahara, Eur. J. Org. Chem., 2010, 4237 CrossRef CAS;
(e) A. J. Elliott, P. E. Morris, S. L. Petty and C. H. Williams, J. Org. Chem., 1997, 62, 8071 CrossRef CAS PubMed;
(f) S. A. Ryndina, A. V. Kadushkin, N. P. Solov'eva and V. G. Granik, Chem. Heterocycl. Compd., 2000, 36, 1409 CrossRef CAS;
(g) N. Chen, Y. Lu, K. Gadamasetti, C. R. Hurt, M. H. Norman and C. Fotsch, J. Org. Chem., 2000, 65, 2603 CrossRef CAS PubMed;
(h) C. Rochais, V. Lisowski, P. Dallemagne and S. Rault, Tetrahedron, 2004, 60, 2267 CrossRef CAS;
(i) A. M. Salaheldin, A. M. F. Oliveira-Campos and L. M. Rodrigues, ARKIVOC, 2008, xiv, 180 Search PubMed;
(j) R. N. Solomyannyi, S. R. Slivchuk, A. N. Vasilenko, E. B. Rusanov and V. S. Brovarets, Russ. J. Gen. Chem., 2012, 82, 317 CrossRef CAS.
-
(a) E. Barnea, S. Majumder, R. J. Staples and A. L. Odom, Organometallics, 2009, 28, 3876 CrossRef CAS;
(b) Y. Cheng, Z.-M. Kang, Y.-G. Ma, J.-H. Peng and M.-F. Liu, Tetrahedron, 2008, 64, 7362 CrossRef CAS;
(c) A. Rolfs, P. G. Jones and J. Liebscher, J. Chem. Soc., Perkin Trans. 1, 1996, 2339 RSC;
(d) O. A. Attanasi, G. Favi, P. Filippone, A. Golobiobič, B. Stanovnik and J. Svete, J. Org. Chem., 2005, 70, 4307 CrossRef CAS PubMed.
- For reviews, see:
(a)
Titanium and Zirconium in Organic Synthesis, ed. I. Marek, Wiley-VCH, Weinheim, 2002 Search PubMed;
(b) A. Wolan and Y. Six, Tetrahedron, 2010, 66, 3097 CrossRef CAS. For recent papers, see:
(c) S. Majumder and A. L. Odom, Tetrahedron, 2010, 66, 3152 CrossRef CAS;
(d) Z. Song, Y. Hsieh, K.-I. Kanno, K. Nakajima and T. Takahashi, Organometallics, 2011, 30, 844 CrossRef CAS;
(e) S. N. Greszler, H. A. Reichard and G. C. Micalizio, J. Am. Chem. Soc., 2012, 134, 2766 CrossRef CAS PubMed;
(f) G. Fan and Y. Liu, Tetrahedron Lett., 2012, 53, 5084 CrossRef CAS;
(g) K. Mao, G. Fan, Y. Liu, S. Li, X. You and D. Liu, Beilstein J. Org. Chem., 2013, 9, 621 CrossRef CAS PubMed;
(h) H. Chen, G. Fan, S. Li, K. Mao and Y. Liu, Tetrahedron Lett., 2014, 55, 1593 CrossRef CAS;
(i) Q. Hu, D. Li, H. Zhang and Z. Xi, Tetrahedron Lett., 2007, 48, 6167 CrossRef CAS;
(j) J. Zhao, S. Zhang, W.-X. Zhang and Z. Xi, Organometallics, 2014, 33, 8 CrossRef CAS.
-
(a) J. Chen and Y. Liu, Tetrahedron Lett., 2008, 49, 6655 CrossRef CAS;
(b) J. Chen and Y. Liu, Organometallics, 2010, 29, 505 CrossRef CAS.
-
(a) S. L. Buchwald, B. T. Watson, R. T. Lum and W. A. Nugent, J. Am. Chem. Soc., 1987, 109, 7137 CrossRef;
(b) T. Takahashi, C. Xi, Z. Xi, M. Kageyama, R. Fischer, K. Nakajima and E. Negishi, J. Org. Chem., 1998, 63, 6802 CrossRef CAS PubMed;
(c) S. Zhou, D. Liu and Y. Liu, Organometallics, 2004, 23, 5900 CrossRef CAS;
(d) X. Sun, C. Wang, Z. Li, S. Zhang and Z. Xi, J. Am. Chem. Soc., 2004, 126, 7172 CrossRef CAS PubMed;
(e) W. Zhang, S. Zhang, X. Sun, M. Nishiura, Z. Hou and Z. Xi, Angew. Chem., Int. Ed., 2009, 48, 7227 CrossRef CAS PubMed;
(f) S. Zhang, W. Zhang and Z. Xi, Chem. – Eur. J., 2010, 16, 8419 CrossRef CAS PubMed;
(g) S. Zhang, W. Zhang, J. Zhao and Z. Xi, J. Am. Chem. Soc., 2010, 132, 14042 CrossRef CAS PubMed;
(h) S. Zhang, W. Zhang, J. Zhao and Z. Xi, Chem. – Eur. J., 2011, 17, 2442 CrossRef CAS PubMed.
- J. R. Fulton, T. A. Hanna and R. G. Bergman, Organometallics, 2000, 19, 602 CrossRef CAS.
- J. Zhao, S. Zhang, W. Zhang and Z. Xi, Organometallics, 2011, 30, 3464 CrossRef CAS.
- D. Suzuki, Y. Nobe, Y. Watai, R. Tanaka, Y. Takayama, F. Sato and H. Urabe, J. Am. Chem. Soc., 2005, 127, 7474 CrossRef CAS PubMed.
-
(a) J. G. Smith and D. C. Irwin, Synthesis, 1978, 894 CrossRef CAS;
(b) E. R. Alonso, K. A. Tehrani, M. Boelens, A. V. Tkachev, Z. Szakonyi, F. Fülöp and N. D. Kimpe, Org. Prep. Proced. Int., 2003, 35, 215 CrossRef;
(c) B. S. Jursic, F. Douelle, K. Bowdy and E. D. Stevens, Tetrahedron Lett., 2002, 43, 5361 CrossRef CAS;
(d) R. Surmont, B. D. Corte and N. D. Kimpe, Tetrahedron Lett., 2009, 50, 3877 CrossRef CAS;
(e) A. M. Dias, A. S. Vila-Chã, A. L. Costa, D. P. Cunha, N. Senhorães and M. F. Proença, Synlett, 2011, 2675 CrossRef CAS;
(f) A. Roychowdhury, V. V. Kumar and A. P. Bhaduri, Synth. Commun., 2006, 36, 715 CrossRef CAS.
-
(a) N. J. Sisti, I. A. Motorina, M. T. H. Dau, C. Riche, F. W. Fowler and D. S. Grierson, J. Org. Chem., 1996, 61, 3715 CrossRef CAS PubMed;
(b) N. J. Sisti, E. Zeller, D. S. Grierson and F. W. Fowler, J. Org. Chem., 1997, 62, 2093 CrossRef CAS PubMed;
(c) D. T. Amos, A. R. Rensio and R. L. Danheiser, J. Am. Chem. Soc., 2003, 125, 4970 CrossRef CAS PubMed;
(d) K. M. Maloney and R. L. Danheiser, Org. Lett., 2005, 7, 3115 CrossRef CAS PubMed;
(e) P. Fontaine, G. Masson and J. Zhu, Org. Lett., 2009, 11, 1555 CrossRef CAS PubMed.
- For the synthesis of α-iminonitriles, see: P. Fontaine, A. Chiaroni, G. Masson and J. Zhu, Org. Lett., 2008, 10, 1509 CrossRef CAS PubMed . Note: when we synthesized 3b according to the above reference, a mixture of two products were obtained in the ratio of 1.1
:
1, which could be separated by preparative HPLC. Analysis of these products by NMR indicated that they are the desired 3b and the brominated α-iminonitrile 3c, but not the Z/E-isomers of 3b as reported. 3b was then synthesized by method b, and characterized by X-ray crystal analysis.
.
- The use of CH2Cl2 as the solvent enables an efficient transformation to pyrrole on silica gel.
- The X-ray crystal structures of compounds 3b, 4a, N-tosylated derivatives of 4l and 4p, 5 and 15 are shown in ESI.† CCDC 988139 (3b), CCDC 988138 (4a), CCDC 988137 (N-tosylated derivative of 4l), CCDC 988141 (N-tosylated derivative of 4p), CCDC 988140 (5) and CCDC 988142 (15) contain the supplementary crystallographic data for this paper.
- For SE2′-type reactions of the titanium-enyne complex, see:
(a) T. Hamada, R. Mizojiri, H. Urabe and F. Sato, J. Am. Chem. Soc., 2000, 122, 7138 CrossRef CAS. For SE2′-type reactions of the titanium-butadiyne complex, see:
(b) C. Delas, H. Urabe and F. Sato, Chem. Commun., 2002, 272 RSC.
- For SE2′-type reactions of the α-alkynylzirconacycles, see:
(a) X. Fu, J. Chen, G. Li and Y. Liu, Angew. Chem., Int. Ed., 2009, 48, 5500 CrossRef CAS PubMed;
(b) X. Fu, S. Yu, G. Fan, Y. Liu and Y. Li, Organometallics, 2012, 31, 531 CrossRef CAS (a calculation which supports SE2′-type reaction pathway is included in this paper);
(c) S. Yu, X. You and Y. Liu, Chem. – Eur. J., 2012, 18, 13936 CrossRef CAS PubMed;
(d) X. You, S. Yu and Y. Liu, Organometallics, 2013, 32, 5273 CrossRef CAS.
- For silica gel promoted transformations, see:
(a) L. Shao, Y. Li and M. Shi, Chem. – Eur. J., 2007, 13, 862 CrossRef CAS PubMed;
(b) S. Eşsiz, M. E. Şengül, E. Şahin and A. Daştan, Turk. J. Chem., 2011, 35, 587 Search PubMed;
(c) Ref. 10g.
- For selected papers for imino-Nazarov reaction, see:
(a) M. A. Tius, C. C. Chu and R. Nieves-Colberg, Tetrahedron Lett., 2001, 42, 2419 CrossRef CAS;
(b) S. Suárez-Pantiga, E. Rubio, C. Alvarez-Rúa and J. M. González, Org. Lett., 2009, 11, 13 CrossRef PubMed;
(c) W. F. Bow, A. K. Basak, A. Jolit, D. A. Vicic and M. A. Tius, Org. Lett., 2010, 12, 440 CrossRef CAS PubMed;
(d) Z. Ma, S. He, W. Song and R. P. Hsung, Org. Lett., 2012, 14, 5736 CrossRef CAS PubMed;
(e) S. A. Bonderoff, T. N. Grant, F. G. West and M. Tremblay, Org. Lett., 2013, 15, 2888 CrossRef CAS PubMed.
- For selected papers for aza-Nazarov reaction, see:
(a) K. Matoba, T. Terada, M. Sugiura and T. Yamazaki, Heterocycles, 1987, 26, 55 CrossRef CAS;
(b) J. Dieker, R. Fröhlich and E.-U. Würthwein, Eur. J. Org. Chem., 2006, 5339 CrossRef CAS;
(c) D. A. Klumpp, Y. Zhang, M. J. O'Connor, P. M. Esteves and L. S. Almeida, Org. Lett., 2007, 9, 3085 CrossRef CAS PubMed;
(d) N. Ghavtadze, R. Fröhlich and E.-U. Würthwein, Eur. J. Org. Chem., 2008, 3656 CrossRef CAS;
(e) K. K. S. Sai, M. J. O'Connor and D. A. Klumpp, Tetrahedron Lett., 2011, 52, 2195 CrossRef CAS PubMed;
(f) R. Narayan, C.-G. Daniliuc and E.-U. Würthwein, Eur. J. Org. Chem., 2012, 6021 CrossRef CAS.
- D. A. Smith and C. W. Ulmer II, J. Org. Chem., 1997, 62, 5110 CrossRef CAS.
- N. Shimada, B. O. Ashburn, A. K. Basak, W. F. Bow, D. A. Vicic and M. A. Tius, Chem. Commun., 2010, 46, 3774 RSC.
- For reviews, see:
(a) S. D. Ittel, L. K. Johnson and M. Brookhart, Chem. Rev., 2000, 100, 1169 CrossRef CAS PubMed;
(b) A. A. Trifonov, Eur. J. Inorg. Chem., 2007, 3151 CrossRef CAS;
(c) J. Yuan, F. Wang, W. Xu, T. Mei, J. Li, B. Yuan, F. Song and Z. Jia, Organometallics, 2013, 32, 3960 CrossRef CAS.
Footnote |
† Electronic supplementary information (ESI) available: Experimental details, spectroscopic characterization of all new compounds, X-ray crystallography of compounds 3b, 4a, N-tosylated derivatives of 4l, 4p, 5, 15 and CIF files giving crystal data are given in the supporting information file. CCDC 988137–988142. For ESI and crystallographic data in CIF or other electronic format see DOI: 10.1039/c4qo00159a |
|
This journal is © the Partner Organisations 2014 |