DOI:
10.1039/C4QO00097H
(Research Article)
Org. Chem. Front., 2014,
1, 546-550
Palladium-catalyzed direct C–H allylation of arenes without directing groups†
Received
29th March 2014
, Accepted 27th April 2014
First published on 28th April 2014
Abstract
A palladium-catalyzed direct C–H allylation of simple arenes without directing groups was developed for allyl arene synthesis. The use of bidentate monoanionic nitrogen ligands enabled the progress of initial C–H activation of arenes, as well as the subsequent insertion and β-OAc elimination.
Allylic substitution is a process that has been widely studied over the past few decades.1 Several nucleophiles have been employed with good regioselectivity and stereoselectivity. Aryl metal reagents are mainly used in relation to aryl nucleophiles (Scheme 1a).2 From the viewpoint of economical processes, using accessible prefunctionalized materials is attractive. Reported examples mostly relied on the highly active electron-rich arenes3 or arene-bearing directing groups (DGs)4 (Scheme 1b and 1c) that facilitate C–H functionalization5 at the ortho position. By contrast, electron-neutral or electron-deficient arenes without DGs have not been analyzed because of difficulty in cleaving inert C–H bonds, except polyfluoroarenes and heteroarenes, which both bear highly acidic C–H bonds.6 We reported a palladium-catalyzed C–H allylation of simple arenes that efficiently yielded a series of allyl arenes (Scheme 1d).
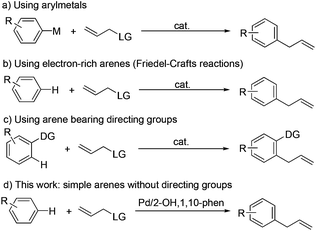 |
| Scheme 1 Synthetic routes for allylarenes. | |
We previously described a palladium/2-OH-1,10-phenanthroline(phen)-catalyzed Fujiwara–Moritani reaction7 of simple arenes,8 in which bidentate monoanionic nitrogen ligands accelerated the C–H activation of various arenes smoothly.9,10 We speculated that the generated aryl–palladium intermediate could react with allylic acetate through insertion and subsequent β-OAc/β-H competing elimination procedures for allyl arene synthesis.11 Bidentate characteristics of the employed ligand also inhibit the β-H elimination pathway because these characteristics reduce the available number of free coordinate sites on the palladium center, which is a prerequisite for the progress of β-H elimination.12
This study began with the reaction of allylic acetate 2 with benzene in the presence of nitrogen ligands and various additives. Screening additives indicated that copper acetate and silver salts yielded the desired coupling products (Table 1, entries 1–3). Optimal amounts of silver carbonate were found, and adding 4 Å molecular sieves was beneficial to the product yield (Table 1, entries 4 and 6). The use of cesium carbonate or cesium acetate did not form the product (Table 1, entries 12 and 13). A series of ligands was tested to examine the effects on this reaction. Mono-hydroxy-bipyridine 5 also exhibited a certain activity (Table 1, entry 15). By contrast, 2,9-OH-1,10-phen, 2-methoxy-1,10-phen, N-acetyl-8-amino-quinoline, and 1,10-phen showed no activities (Table 1, entries 16–19). Monopyridine ligands (e.g., ethyl nicotinoate13a and ortho-disubstituted pyridine13b developed by Yu et al.) were also examined, which exhibited relatively low product yields (Table 1, entries 20 and 21). Protected amino acids, which had important functions in promoting Pd-catalyzed C–H activation reactions,9 also showed no activity (Table 1, entry 22).
Table 1 Palladium-catalyzed reactions of benzene with allylic acetatesa
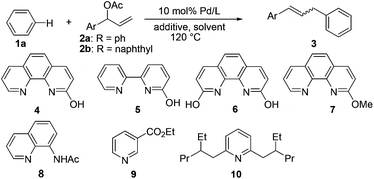
|
Entry |
Substrate |
Ligand |
Additive (equiv.) |
Yieldb |
Ratio of E/Z isomersc |
All the reactions were conducted with 0.20 mmol of allylic acetates in 1.0 mL of arenes unless specifically noted.
Yields were determined by 1H NMR analysis of the crude product using CH2Br2 as the internal standard.
The E/Z ratio was determined by 1H NMR analysis of the crude product.
Isolated yield.
|
1 |
2a
|
4
|
Cu(OAc)2 (1.0) |
62% |
1.3/1 |
2 |
2a
|
4
|
AgOAc (0.5) |
21% |
1.3/1 |
3 |
2a
|
4
|
Ag2CO3 (0.5) |
40% |
1.3/1 |
4 |
2a
|
4
|
Ag2CO3 (0.5)/4 Å MS |
82%d |
1.3/1 |
5 |
2b
|
4
|
Ag2CO3 (0.5) |
55 |
1.4/1 |
6 |
2b
|
4
|
Ag2CO3 (0.5)/4 Å MS |
61 |
1.4/1 |
7 |
2b
|
4
|
Cu(OAc)2 (1.0) |
12 |
1.6/1 |
8 |
2b
|
4
|
Ag2O (0.5) |
50 |
1.4/1 |
9 |
2b
|
4
|
AgBF4 (0.5) |
0 |
— |
10 |
2b
|
4
|
Ag2SO4 (0.5) |
0 |
— |
11 |
2b
|
4
|
Ag3PO4 (0.5) |
0 |
— |
12 |
2b
|
4
|
Cs2CO3 (0.5) |
0 |
— |
13 |
2b
|
4
|
CsOAc (0.5) |
0 |
— |
14 |
2a
|
No ligand |
Ag2CO3 (0.5)/4 Å MS |
4 |
— |
15 |
2a
|
5
|
Ag2CO3 (0.5)/4 Å MS |
68 |
1.6/1 |
16 |
2a
|
6
|
Ag2CO3 (0.5)/4 Å MS |
0 |
— |
17 |
2a
|
7
|
Ag2CO3 (0.5)/4 Å MS |
0 |
— |
18 |
2a
|
8
|
Ag2CO3 (0.5)/4 Å MS |
0 |
— |
19 |
2a
|
1,10-Phen |
Ag2CO3 (0.5)/4 Å MS |
0 |
— |
20 |
2a
|
9
|
Ag2CO3 (0.5)/4 Å MS |
26% |
E only |
21 |
2a
|
10
|
Ag2CO3 (0.5)/4 Å MS |
12% |
E only |
22 |
2a
|
Boc-L-valine |
Ag2CO3 (0.5)/4 Å MS |
3 |
— |
The scope of allylic acetates was subsequently examined with the optimal conditions obtained. The electron-rich or electron-deficient substituent groups at ortho, meta, or para positions were found to be suitable electrophiles and yielded corresponding products with moderate to good yields (Table 2). The alkyl-substituted groups were also examined, and the product was generated with the formation of a double addition product (Table 2, entry 10: 3ka′, 15% yield). Bis-substituted allylic acetates were tested under the same conditions to obtain substituted products with 65% and 70% yields (Table 2, entries 11 and 12: 3la and 3ma). Table 3 summarizes the results of substituted arenes with allylic acetates. 1,4-Difluorobenzene and 1,4-dichlorobenzene are suitable aryl donors with yields of 70% and 78%, respectively. The allyl moiety was selectively installed at the less hindered position for 4-chloro-trifluorobenzene. Tetrafluorobenzene was also examined to obtain a 65% product yield, which was not described in the reported catalytic system6 because of the higher pKa of the C–H bond compared with that of pentafluorobenzene. Electron-rich arenes were also investigated; 1,3-dimethoxybenzene was functionalized only at the meta-position, which suggested that ligand 4 had a key function in determining regioselectivity.14 Naphthalene also exhibited relatively good regioselecitivty15 because of the steric effects.
|  | (1) |
|  | (2) |
|  | (3) |
|  | (4) |
Table 2 Palladium-catalyzed allylation of benzene with allylic acetatesa
Table 3 Palladium-catalyzed allylation of arenes with allylic acetatesa
To further understand the reaction, we conducted a reaction of deuterated benzene with 2a under identical conditions and obtained KH/KD = 2.9, indicating that C–H bond cleavage was involved in the rate-limiting step (Scheme 2).16 The use of homoallylic alcohol acetate yielded a functionalized allylic acetate as the β-H elimination product (eqn (1)). The use of cinnamic acetate as the substrate did not yield the allylation product (eqn (2)), which ruled out the involvement of a π-allylic palladium intermediate. The use of phenylboronic acid as a nucleophile instead of benzene also generated a mixture of E/Z products (eqn (3)). The enantio-enriched allylic acetate (R)-2l (78% ee) reacted with benzene, (S)-trans-alkene was isolated in 72% ee, and (R)-cis-alkene was obtained in 65% ee (eqn (4)).17 On the basis of the above results, a possible stereochemical pathway was proposed for the current reaction in Scheme 3. The C–H cleavage of benzene enabled by palladium ligated with ligand 4 generated a palladium–aryl intermediate. A facial selective insertion of alkene into the aryl–palladium bond produced two alkyl palladium intermediates. Finally, cis-β-OAc elimination yielded the (E) and (Z)-products.
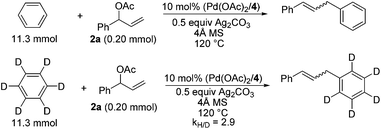 |
| Scheme 2 Experiments with isotopically labelled compounds. | |
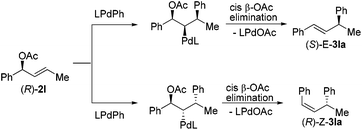 |
| Scheme 3 Proposed stereochemical pathway. | |
In summary, we have reported a palladium-catalyzed C–H allylation of simple arenes without directing groups with allylic acetates, which yielded the corresponding allyl arenes with moderate to good yields. The bidentate monoanionic ligand framework has a significant effect on the success of C–H activation and subsequent β-OAc elimination.
We thank the National Basic Research Program of China (973 Program; 2010CB833300), the NSFC (20902099 and 21172238), and SIOC for funding this work. We thank Prof. Shi-Kai Tian for kindly donating an authentic sample of (S)-(E)-3la.
Notes and references
- For selected reviews on allylic substitution reactions, see:
(a) J. Tsuji, Acc. Chem. Res., 1969, 2, 144 CrossRef CAS;
(b) B. M. Trost, Tetrahedron, 1977, 33, 2615 CrossRef CAS;
(c) B. M. Trost and D. L. van Vranken, Chem. Rev., 1996, 96, 395 CrossRef CAS PubMed;
(d) B. M. Trost, Acc. Chem. Res., 1996, 29, 355 CrossRef CAS;
(e) G. Helmchen and A. Pfaltz, Acc. Chem. Res., 2000, 33, 336 CrossRef CAS PubMed;
(f) T. Hayashi, Acc. Chem. Res., 2000, 33, 354 CrossRef CAS PubMed;
(g) B. M. Trost and M. L. Crawley, Chem. Rev., 2003, 103, 2921 CrossRef CAS PubMed;
(h) L.-X. Dai, T. Tu, S.-L. You, W.-P. Deng and X.-L. Hou, Acc. Chem. Res., 2003, 36, 659 CrossRef CAS PubMed;
(i) H. Yorimitsu and K. Oshima, Angew. Chem., Int. Ed., 2005, 44, 4435 CrossRef CAS PubMed;
(j) B. M. Trost, M. R. Machacek and A. Aponick, Acc. Chem. Res., 2006, 39, 747 CrossRef CAS PubMed;
(k) G. Helmchen, A. Dahnz, P. Dübon, M. Schelwies and R. Weihofen, Chem. Commun., 2007, 675 RSC;
(l) Z. Lu and S. Ma, Angew. Chem., Int. Ed., 2008, 47, 258 CrossRef CAS PubMed;
(m) A. Alexakis, J. E. Bäckvall, N. Krause, O. Pàmies and M. Diéguez, Chem. Rev., 2008, 108, 2796 CrossRef CAS PubMed;
(n) S. R. Harutyunyan, T. den Hartog, K. Geurts, A. J. Minnaard and B. L. Feringa, Chem. Rev., 2008, 108, 2824 CrossRef CAS PubMed;
(o) B. M. Trost, T. Zhang and J. D. Sieber, Chem. Sci., 2010, 1, 427 RSC;
(p) F. C. Pigge, Synthesis, 2010, 1745 CrossRef CAS PubMed.
- For selected examples of using aryl metal reagents in allylic substitution reactions, see:
(a) T. Hayashi, M. Konishi, K. Yokota and M. Kumada, J. Chem. Soc., Chem. Commun., 1981, 313 RSC;
(b) T. Hayashi, M. Konishi and M. Kumada, J. Chem. Soc., Chem. Commun., 1984, 107 RSC;
(c) N. Miyaura, K. Yamada, H. Suginome and A. Suzuki, J. Am. Chem. Soc., 1985, 107, 972 CrossRef CAS;
(d) Y. Uozumi, H. Danjo and T. Hayashi, J. Org. Chem., 1999, 64, 3384 CrossRef CAS;
(e) H. Ohmiya, Y. Makida, T. Tanaka and M. Sawamura, J. Am. Chem. Soc., 2008, 130, 17276 CrossRef CAS PubMed;
(f) H. Ohmiya, Y. Makida, D. Li, M. Tanabe and M. Sawamura, J. Am. Chem. Soc., 2010, 132, 879 CrossRef CAS PubMed;
(g) D. Li, T. Tanaka, H. Ohmiya and M. Sawamura, Org. Lett., 2010, 12, 2438 CrossRef PubMed;
(h) D. Li, T. Tanaka, H. Ohmiya and M. Sawamura, Org. Lett., 2010, 12, 3344 CrossRef CAS PubMed;
(i) Y. Makida, H. Ohmiya and M. Sawamura, Chem. – Asian J., 2011, 6, 410 CrossRef CAS PubMed;
(j) S. M. Sarkar, Y. Uozumi and Y. M. A. Yamada, Angew. Chem., Int. Ed., 2011, 50, 9437 CrossRef CAS PubMed;
(k) M.-B. Li, Y. Wang and S.-K. Tian, Angew. Chem., Int. Ed., 2012, 51, 2968 CrossRef CAS PubMed;
(l) C. Li, J. Xing, J. Zhao, P. Huynh, W. Zhang, P. Jiang and Y. J. Zhang, Org. Lett., 2012, 14, 390 CrossRef CAS PubMed.
- For examples of using electron-rich arenes in allylic substitution reactions, see:
(a) M. Bandini, E. Emer, S. Tommasi and A. Umani-Ronchi, Eur. J. Org. Chem., 2006, 3527 CrossRef CAS;
(b) T. B. Poulsen and K. A. Jorgensen, Chem. Rev., 2008, 108, 2903 CrossRef CAS PubMed;
(c) M. Bandini and A. Eichholzer, Angew. Chem., Int. Ed., 2009, 48, 9608 CrossRef CAS PubMed;
(d) C.-X. Zhuo, W. Zhang and S.-L. You, Angew. Chem., Int. Ed., 2012, 51, 12662 CrossRef PubMed.
-
(a) H. Wang, N. Schröder and F. Glorius, Angew. Chem., Int. Ed., 2013, 52, 5386 CrossRef CAS PubMed;
(b) S. Asako, L. Ilies and E. Nakamura, J. Am. Chem. Soc., 2013, 135, 17755 CrossRef CAS PubMed;
(c) C. Feng, D. Feng and T.-P. Loh, Org. Lett., 2013, 15, 3670 CrossRef CAS PubMed.
- For selected reviews on C–H bond functionalization, see:
(a) F. Kakiuchi and S. Murai, Acc. Chem. Res., 2002, 35, 826 CrossRef CAS PubMed;
(b) F. Kakiuchi and N. Chatani, Adv. Synth. Catal., 2003, 345, 1077 CrossRef CAS;
(c) B. Li, S. Yang and Z. Shi, Synlett, 2008, 949 CAS;
(d) X. Chen, K. M. Engle, D.-H. Wang and J.-Q. Yu, Angew. Chem., Int. Ed., 2009, 48, 5094 CrossRef CAS PubMed;
(e) R. Giri, B.-F. Shi, K. M. Engle, N. Maugel and J.-Q. Yu, Chem. Soc. Rev., 2009, 38, 3242 RSC;
(f) L. Ackermann, R. Vicente and A. Kapdi, Angew. Chem., Int. Ed., 2009, 48, 9792 CrossRef CAS PubMed;
(g) L.-M. Xu, B.-J. Li, Z. Yang and Z.-J. Shi, Chem. Soc. Rev., 2010, 39, 712 RSC;
(h) M. Albrecht, Chem. Rev., 2010, 110, 576 CrossRef CAS PubMed;
(i) D. A. Colby, R. G. Bergman and J. A. Ellman, Chem. Rev., 2010, 110, 624 CrossRef CAS PubMed;
(j) T. W. Lyons and M. S. Sanford, Chem. Rev., 2010, 110, 1147 CrossRef CAS PubMed;
(k) L. Ackermann, Chem. Rev., 2011, 111, 1315 CrossRef CAS PubMed;
(l) N. Kuhl, M. N. Hopkinson, J. Wencel-Delord and F. Glorius, Angew. Chem., Int. Ed., 2012, 51, 10236 CrossRef CAS PubMed;
(m) G. Song, F. Wang and X. Li, Chem. Soc. Rev., 2012, 41, 3651 RSC;
(n) J. Wencel-Delord and F. Glorius, Nat. Chem., 2013, 5, 369 CrossRef CAS PubMed;
(o) N. Yoshikai and Y. Wei, Asian J. Org. Chem., 2013, 2, 466 CrossRef CAS;
(p) R. Ferraccioli, Synthesis, 2013, 581 CrossRef CAS PubMed.
-
(a) T. Yao, K. Hirano, T. Satoh and M. Miura, Angew. Chem., Int. Ed., 2011, 50, 2990 CrossRef CAS PubMed;
(b) S. Fan, F. Chen and X. Zhang, Angew. Chem., Int. Ed., 2011, 50, 5918 CrossRef CAS PubMed;
(c) Y. Makida, H. Ohmiya and M. Sawamura, Angew. Chem., Int. Ed., 2012, 51, 4122 CrossRef CAS PubMed.
- For leading examples of Fujiwara–Moritani reactions, see:
(a) I. Moritani and Y. Fujiwara, Tetrahedron Lett., 1967, 8, 1119 CrossRef;
(b) Y. Fujiwara, I. Moritani, S. Danno, R. Asano and S. Teranishi, J. Am. Chem. Soc., 1969, 91, 7166 CrossRef CAS;
(c) C. Jia, W. Lu, T. Kitamura and Y. Fujiwara, Org. Lett., 1999, 1, 2097 CrossRef CAS;
(d) C. Jia, D. Piao, J. Oyamada, W. Lu, T. Kitamura and Y. Fujiwara, Science, 2000, 287, 1992 CrossRef CAS;
(e) C. Jia, T. Kitamura and Y. Fujiwara, Acc. Chem. Res., 2001, 34, 633 CrossRef CAS PubMed.
- C.-H. Ying, S.-B. Yan and W.-L. Duan, Org. Lett., 2014, 16, 500 CrossRef CAS PubMed.
- For selected examples of amino acid-promoted Pd-catalyzed C–H activation reactions, see:
(a) K. M. Engle and J.-Q. Yu, J. Org. Chem., 2013, 78, 8927 CrossRef CAS PubMed;
(b) D.-H. Wang, K. M. Engle, B.-F. Shi and J.-Q. Yu, Science, 2010, 327, 315 CrossRef CAS PubMed;
(c) K. M. Engle, D.-H. Wang and J.-Q. Yu, J. Am. Chem. Soc., 2010, 132, 14137 CrossRef CAS PubMed;
(d) K. M. Engle, P. S. Thuy-Boun, M. Dang and J.-Q. Yu, J. Am. Chem. Soc., 2011, 133, 18183 CrossRef CAS PubMed;
(e) P. Novák, A. Correa, J. Gallardo-Donaire and R. Martin, Angew. Chem., Int. Ed., 2011, 50, 12236 CrossRef PubMed;
(f) W. Masayuki, K. S. L. Chan, X.-G. Zhang, J. He and M. Miura, J. Am. Chem. Soc., 2012, 134, 18570 CrossRef PubMed;
(g) X.-F. Cheng, Y. Li, Y.-M. Su, F. Yin, J.-Y. Wang, J. Sheng, H. U. Vora, X.-S. Wang and J.-Q. Yu, J. Am. Chem. Soc., 2013, 135, 1236 CrossRef CAS PubMed.
- For sulfoxide and nitrogen ligand-promoted Pd-catalyzed C–H activation reactions, see:
(a) M. S. Chen and M. C. White, J. Am. Chem. Soc., 2004, 126, 1346 CrossRef CAS PubMed;
(b) M. S. Chen, N. Prabagaran, N. A. Labenz and M. C. White, J. Am. Chem. Soc., 2005, 127, 6970 CrossRef CAS PubMed;
(c) S. A. Reed, A. R. Mazzotti and M. C. White, J. Am. Chem. Soc., 2009, 131, 11701 CrossRef CAS PubMed;
(d) G. T. Rice and M. C. White, J. Am. Chem. Soc., 2009, 131, 11707 CrossRef CAS PubMed;
(e) Y. Izawa and S. S. Stahl, Adv. Synth. Catal., 2010, 352, 3223 CrossRef CAS PubMed;
(f) A. N. Campbell, P. B. White, I. A. Guzei and S. S. Stahl, J. Am. Chem. Soc., 2010, 132, 15116 CrossRef CAS PubMed;
(g) A. N. Campbell, E. B. Meyer and S. S. Stahl, Chem. Commun., 2011, 47, 10257 RSC.
- For reviews on Pd-catalyzed β-elimination reactions, see:
(a) D. Pan and J. Ning, Synthesis, 2010, 1577 CAS;
(b) J. Le Bras and J. Muzart, Tetrahedron, 2012, 68, 10065 CrossRef CAS PubMed.
- For examples of Pd-catalyzed C–H alkenylation of arenes with allylic acetates via the β-H elimination pathway, see:
(a) D. Pan, M. Yu, W. Chen and J. Ning, Chem. – Asian J., 2010, 5, 1090 CrossRef CAS PubMed;
(b) Z. Li, Y. Zhang and Z.-Q. Liu, Org. Lett., 2012, 14, 74 CrossRef CAS PubMed.
-
(a) E. M. Ferreira and B. M. Stoltz, J. Am. Chem. Soc., 2003, 125, 9578 CrossRef CAS PubMed;
(b) Y.-H. Zhang, B.-F. Shi and J.-Q. Yu, J. Am. Chem. Soc., 2009, 131, 5072 CrossRef CAS PubMed.
-
ortho- and para-Selectivities were observed for ligand free Pd-catalyzed C–H activation of electron-rich arenes, see:
(a) T. Yokota, M. Tani, S. Sakaguchi and Y. Ishii, J. Am. Chem. Soc., 2003, 125, 1476 CrossRef CAS PubMed;
(b) M. Dams, D. E. De Vos, S. Celen and P. A. Jacobs, Angew. Chem., Int. Ed., 2003, 42, 3512 CrossRef CAS PubMed.
- For examples of Pd/Pt-catalyzed C–H arylation of naphthalene with good selectivity, see:
(a) A. J. Hickman and M. S. Sanford, ACS Catal., 2011, 1, 170 CrossRef CAS;
(b) A. N. Wagner, A. J. Hickman and M. S. Sanford, J. Am. Chem. Soc., 2013, 135, 15710 CrossRef CAS PubMed.
- For an essay on the interpretation of deuterium kinetic isotope effects in C–H bond functionalization, see: E. M. Simmons and J. F. Hartwig, Angew. Chem., Int. Ed., 2012, 51, 3066 CrossRef CAS PubMed.
- Sawamura and coworkers reported that Pd/1,10-phen-catalyzed stereospecific allyl–aryl coupling between acyclic allylic acetates and arylboronic acid with excellent chirality transfer, see ref. 2e and 2f for details.
Footnote |
† Electronic supplementary information (ESI) available: Detailed experimental procedures and analytical data for all new compounds. See DOI: 10.1039/c4qo00097h |
|
This journal is © the Partner Organisations 2014 |
Click here to see how this site uses Cookies. View our privacy policy here.