DOI:
10.1039/C4QO00084F
(Research Article)
Org. Chem. Front., 2014,
1, 674-677
Asymmetric synthesis of (S)-tylophorine and (S)-cryptopleurine via one-pot Curtius rearrangement and Friedel–Crafts reaction tandem sequence†
Received
21st March 2014
, Accepted 20th May 2014
First published on 21st May 2014
Abstract
A general and practical enantioselective synthetic approach to both the phenanthroindolizidine and phenanthroquinolizidine alkaloids (S)-tylophorine and (S)-cryptopleurine was developed, which features a stereoselective alkylation and a one-pot Curtius reaction rearrangement/intramolecular cyclization cascade sequence.
Phenanthroindolizidine and phenanthroquinolizidine alkaloids are a group of pentacyclic natural products (Fig. 1), which are mainly isolated from Tylophora indica, Pergularia, and Cynanchum species.1 They exhibit a variety of biological activities, including antitumor, antiamoebic, and antifungal activities,2,3 among which their antitumor activity has been well studied. Because of their remarkable bioactivities coupled with their low natural abundance and interesting structures, numerous synthetic strategies have been developed.1,4,5
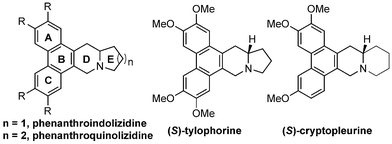 |
| Fig. 1 Representatives of phenanthroindo/quinolizidine alkaloids. | |
Although great synthetic efforts have been expended on the total synthesis of these alkaloids, highly efficient and practical strategies are still desirable. We have a great interest in cascade and rearrangement reactions, which can usually be used to construct the skeletons of organic molecules in an amazing way with high efficiency. Recently, we developed a novel strategy to prepare phenanthroindolizidine alkaloid (S)-tylophorine from azidoaldehyde 1, featuring a one-pot intramolecular Schmidt/Bischler–Napieralski/imine-reduction cascade sequence, in which three new chemical bonds and two rings are formed in good yield (Scheme 1).6 The key step of the cascade sequence was the initial transformation from azidoaldehyde 1 to six-membered ring intermediate azidohydrin 2, which was followed by alkyl migration to furnish formamide 3 (Scheme 1). However, when the strategy was applied to the synthesis of phenanthroquinolizidine alkaloid 5, it was found that the initial Schmidt rearrangement step did not occur under similar reaction conditions. Attempting to execute this transformation, various Lewis acids and Brønsted acids were screened, none of which proved to be effective for this rearrangement, giving no reaction or very complex products. The main reason for the failure of the transformation is believed to be that formation of a seven-membered ring is unfavorable due to the relatively large energy of the transition state. Therefore, to explore a general and expedient synthetic strategy for the rapid preparation of both types of alkaloids, an alternative design was taken into consideration (Scheme 2).
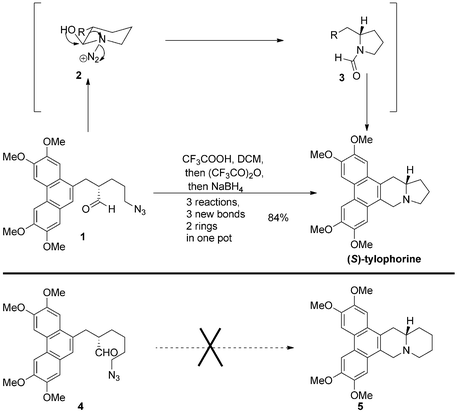 |
| Scheme 1 Intramolecular Schmidt/Bischler–Napieralski/imine-reduction cascade sequence of aldehyde. | |
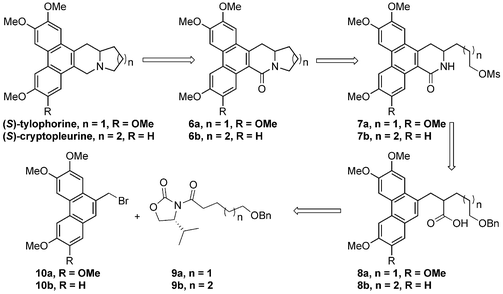 |
| Scheme 2 Retrosynthetic analysis of phenanthroindo/quinolizidine alkaloids. | |
Retrosynthetically, (S)-tylophorine was used as an example to showcase our synthetic strategy. The target molecule could be accessible via reduction from lactam 6a, which could be easily derived from lactam 7avia an intramolecular nucleophilic substitution. We planned to construct the D ring of compound 7a through a Curtius rearrangement/intramolecular cyclization sequence from acid 8a, which could be prepared from oxazolidinone 9a and phenanthryl bromide 10a using Evans’ stereoselective alkylation protocol.
Based on the design above, the synthesis of (S)-tylophorine started with Evans’ stereoselective alkylation from the readily available phenanthryl bromide 10a7 and oxazolidinone 9a8 under standard conditions,9,10 giving compound 11a as a single diastereoisomer after chromatographic purification (Scheme 3). After hydrolysis, the precursor of the desired Curtius rearrangement acid 8a was obtained. Since the discovery of the Curtius rearrangement 100 years ago, this classic reaction has been investigated extensively and applied frequently in the total synthesis of natural products.11 The precursor of the Curtius rearrangement is an acyl azide, which can usually produce the important synthetic intermediate isocyanate under thermal conditions. The process can also be induced by photolysis, but this pathway always gives rise to several side-products in addition to the desired isocyanate.12 Common nucleophiles added to the isocyanate include water, alcohols, and amines, resulting in the corresponding amines, carbamates, and ureas. However, internal electron-rich arenes as nucleophiles have rarely been reported, which is probably due to their relatively poor nucleophilicity, and usually harsh conditions are needed.4,13 Unexpectedly, when the acid 8a was treated with diphenylphosphoryl azide (DPPA) and triethylamine in toluene at 0 °C, isocyanate 12a was formed, a crude sample of which was verified by 1H NMR and HRMS after flash chromatography. The formation of an isocyanate under such mild reaction conditions is really uncommon, and the detailed reasons are unclear at present, which deserves further investigation. After extensive screening of Lewis acids and Brønsted acids, BF3·Et2O was found to be the optimal catalyst for this transformation, giving lactam 13a in 55% yield over 2 steps. Pentacyclic lactam 6a, obtained from compound 13a through sequential hydrogenation, methanesulfonation, and intramolecular nucleophilic substitution, was reduced by lithium aluminium hydride, giving (S)-tylophorine in good yield and 94% ee. (S)-Cryptopleurine could be also prepared in comparable yield in 98% ee, using similar procedures from phenanthryl bromide 10b and oxazolinone 9b. Melting points, NMR spectra, and optical rotations were in accordance with the literature (for details see Experimental section†).
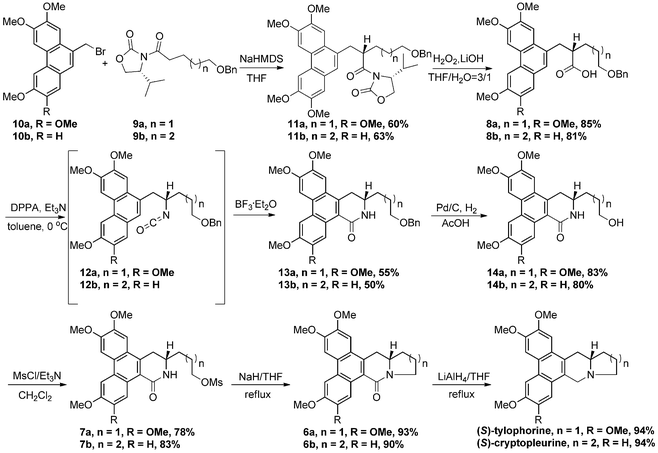 |
| Scheme 3 Synthesis of (S)-tylophorine and (S)-cryptopleurine. | |
Conclusions
In summary, we have developed an efficient and practical enantioselective strategy for the synthesis of (S)-tylophorine and (S)-cryptopleurine. Advantages of this new approach include: (1) a stereoselective alkylation to introduce the stereogenic center, which also enables the synthesis of the antipode via converting the Evans auxiliary, and (2) an unprecedented one-pot Curtius rearrangement/intramolecular cyclization cascade sequence to construct the D ring.
Acknowledgements
This work was supported by the National Key Project for Basic Research (2010CB126106), the National Natural Science Foundation of China (21132003, 21121002, 21372131), and the Specialized Research Fund for the Doctoral Program of Higher Education (20130031110017).
Notes and references
-
(a) A. N. Ratnagiriswaran and K. Venkatachalam, Indian J. Med. Res., 1935, 22, 433 CAS;
(b) N. B. Mulchandani and S. R. Venkatachalam, Phytochemistry, 1976, 15, 1561 CrossRef CAS.
-
(a) D. Stærk, A. K. Lykkeberg, J. B. Christensen, A. Budnik, F. Abe and J. W. Jaroszewski, J. Nat. Prod., 2002, 65, 1299 CrossRef PubMed;
(b) Y. Luo, Y. Liu, D. Luo, X. Gao, B. Li and G. Zhang, Planta Med., 2003, 69, 842 CrossRef CAS PubMed;
(c) M. G. Banwell, A. Bezos, C. Burns, I. Kruszeknicki, C. R. Parish, S. Su and M. O. Sydnes, Bioorg. Med. Chem. Lett., 2006, 16, 181 CrossRef CAS PubMed;
(d) Y. Fu, S. K. Lee, H. Y. Min, T. Lee, J. Lee, M. Cheng and S. Kim, Bioorg. Med. Chem. Lett., 2007, 17, 97 CrossRef CAS PubMed;
(e) C. W. Yang, T. H. Chuang, P. L. Wu, W. H. Huang and S. J. Lee, Biochem. Biophys. Res. Commun., 2007, 354, 942 CrossRef CAS PubMed;
(f) W. Gao, S. Busson, S. P. Grill, E. A. Gullen, Y. C. Hu, X. Huang, S. Zhong, C. Kaczmarek, J. Gutierrez, S. Francis, D. C. Baker, S. Yu and Y. C. Cheng, Bioorg. Med. Chem. Lett., 2007, 17, 4338 CrossRef CAS PubMed;
(g) X. Yang, Q. Shi, K. F. Bastow and K. H. Lee, Org. Lett., 2010, 12, 1416 CrossRef CAS PubMed.
- Reviews:
(a) Z. Li, Z. Jin and R. Huang, Synthesis, 2001, 2365 CrossRef CAS PubMed;
(b) E. Gellert, J. Nat. Prod., 1982, 45, 50 CrossRef CAS;
(c) S. R. Chemler, Curr. Bioact. Compd., 2009, 5, 2 CrossRef CAS PubMed;
(d) I. R. C. Bick and W. Sinchai, Alkaloids, 1981, 19, 193 CAS;
(e) T. R. Govindachari and N. Viswanathan, Heterocycles, 1978, 11, 587 CrossRef CAS.
- T. H. Chuang, S. J. Lee and C. W. Yang, Org. Biomol. Chem., 2006, 4, 860 CAS.
- For the most recent selected examples, see:
(a) X. Yang, Q. Shi, K. F. Bastow and K. H. Lee, Org. Lett., 2010, 12, 1416 CrossRef CAS PubMed;
(b) A. Stoye and T. Opatz, Org. Lett., 2010, 12, 2140 CrossRef CAS PubMed;
(c) M. Cui, H. Song, A. Feng, Z. Wang and Q. Wang, J. Org. Chem., 2010, 75, 7018 CrossRef CAS PubMed;
(d) G. I. Georg and M. J. Niphakis, J. Org. Chem., 2010, 75, 6019 CrossRef PubMed;
(e) J. P. Wolfe and D. N. Mai, J. Am. Chem. Soc., 2010, 132, 12157 CrossRef PubMed;
(f) G. I. Georg and M. J. Niphakis, Org. Lett., 2011, 13, 196 CrossRef PubMed;
(g) S. F. Hsu, C. W. Ko and Y. T. Wu, Adv. Synth. Catal., 2011, 353, 1756 CrossRef CAS;
(h) B. Su, M. Deng and Q. Wang, Eur. J. Org. Chem., 2013, 1979 CrossRef CAS;
(i) J. C. Orejarena Pacheco, G. Lahm and T. Opatz, J. Org. Chem., 2013, 78, 4985 CrossRef CAS PubMed;
(j) G. Han, Y. Liu and Q. Wang, Org. Lett., 2013, 15, 5334 CrossRef CAS PubMed.
- B. Su, F. Z. Chen and Q. M. Wang, J. Org. Chem., 2013, 78, 2775 CrossRef CAS PubMed.
- K. L. Wang, B. Su, Z. W. Wang, M. Wu, L. Zheng, Y. N. Hu, Z. J. Fan, N. Mi and Q. M. Wang, J. Agric. Food Chem., 2010, 58, 2703 CrossRef CAS PubMed.
-
(a) J. Weinstock, J. Org. Chem., 1961, 26, 3511 CrossRef CAS;
(b) T. Shioiri, K. Ninomiya and S. Yamada, J. Am. Chem. Soc., 1972, 94, 6203 CrossRef CAS;
(c) G. K. Prakash, P. S. Lyer, M. Arvanaghi and G. Olah, J. Org. Chem., 1983, 48, 3358 CrossRef CAS.
- V. Guerlavais, P. J. Carroll and M. M. Joullie, Tetrahedron: Asymmetry, 2002, 13, 675 CrossRef CAS.
-
(a) D. A. Evans, M. D. Ennis and D. J. Mathre, J. Am. Chem. Soc., 1982, 104, 1737 CrossRef CAS;
(b) D. A. Evans, S. L. Bender and J. Morris, J. Am. Chem. Soc., 1988, 110, 2506 CrossRef CAS;
(c) R. M. Jason and B. Christian, Chem. Commun., 2003, 1738 Search PubMed;
(d) R. M. Jason, A. H. Michael and B. Christian, Org. Biomol. Chem., 2004, 2, 1484 RSC;
(e) F. Hettche, P. Reiβ and R. W. Hoffmann, Chem. – Eur. J., 2002, 8, 4946 CrossRef CAS.
-
(a) M. Carda, F. Gonzalez, R. Sanchez and J. A. Marco, Tetrahedron: Asymmetry, 2002, 13, 1005 CrossRef CAS;
(b) D. L. Boger, K. C. Cassidy and S. Nakahara, J. Am. Chem. Soc., 1993, 115, 10733 CrossRef CAS;
(c) S. Nagumo, A. Nishida, C. Yamazaki, A. Matoba, K. Murashige and N. Kawahara, Tetrahedron, 2002, 58, 4917 CrossRef CAS.
- E. Eibler and J. Sauer, Tetrahedron Lett., 1974, 2569 CrossRef CAS.
-
(a) D. Dou, P. Viwanathan and C. G. William, J. Comb. Chem., 2010, 12, 836 CrossRef CAS PubMed;
(b) S. Hanessian, E. Demontand and W. A. L. V. Otterlo, Tetrahedron Lett., 2000, 41, 4999 CrossRef CAS;
(c) K. E. Judd, M. F. Mahon and L. Caggiano, Synthesis, 2009, 2809 CAS.
Footnote |
† Electronic supplementary information (ESI) available. See DOI: 10.1039/c4qo00084f |
|
This journal is © the Partner Organisations 2014 |
Click here to see how this site uses Cookies. View our privacy policy here.