DOI:
10.1039/C4QO00007B
(Research Article)
Org. Chem. Front., 2014,
1, 186-189
Copper-mediated efficient three-component synthesis of 1,2,4-triazoles from amines and nitriles†‡
Received
8th January 2014
, Accepted 15th January 2014
First published on 30th January 2014
Abstract
An efficient copper-mediated three-component reaction of one molecule of amine and two molecules of nitriles affording fully substituted 1,2,4-triazoles has been developed. A tandem double addition–oxidative cyclization mechanism has been proposed for the synthesis of 1,2,4-triazoles.
Among the various bioactive heterocycles, 1,2,4-triazoles are one of the most important scaffolds because of their wide applications in the pharmaceutical and agrochemical industries.1 A number of 1,2,4-triazole-containing compounds such as Anastrozole, Fluconazole, Letrozole, Mitratapide, Voriconazole, Maxalt, Deferasirox, Triadimefon, Triadiminol, Fuotrimazole, Bitertanol, Diclobutrazole, Diniconazole, and Tebuconazole have been successfully commercialized as medicines or pesticides (Scheme 1). All these listed compounds except Deferasirox contain the unsubstituted parent 1,2,4-triazole unit.2 Thus, efficient and new methodologies for the introduction of different substituents into the specific locations in the 1,2,4-triazoles would be of high interest for medicinal chemists. The importance of the heterocyclic moiety has prompted the development of some synthetic routes to 1,2,4-trizaole derivatives; however, there remain some limitations such as inconvenient starting materials, multistep transformation, and tedious reaction conditions in some cases which restrict their wide application.3,4 Thus, a simple, efficient, and diverse synthesis of 1,2,4-trizaole skeletons with a specific substitution pattern is still highly desirable.
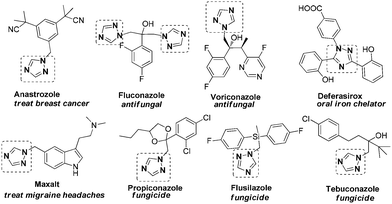 |
| Scheme 1 Some commercialized bioactive 1,2,4-triazole compounds. | |
During the study of the addition reaction of allenoates with amines in PhCN,5,6 we observed an intriguing product, which was identified as 1,2,4-triazole formed from the reaction of amine and nitrile. To the best of our knowledge, a direct synthesis of fully substituted 1,2,4-triazoles from simple amines and nitriles has been hitherto unknown. This approach offers the benefit that two C–N bonds and one N–N bond are formed in a one step fashion from simple and readily available amines and nitriles as the starting materials. Herein, we are pleased to disclose our recent work on this unprecedented copper-mediated three-component reaction.
At first, we treated BnNH2 with Cu(OAc)2 in PhCN at 100 °C, and 1,2,4-triazole 3a was formed in 38% yield (Table 1, entry 1). Further study of the effect of temperature led to the observation that the reaction at 120 °C afforded the 1,2,4-triazole 3a in 44% yield (Table 1, entry 2). Besides, the reaction could also furnish the desired product at 120 °C in a microwave reactor (Table 1, entry 3). Reducing the loading of Cu(OAc)2 led to a lower yield (Table 1, entry 4). When the reaction was conducted in DMSO with 4 equiv. of PhCN, the desired product was not observed (Table 1, entry 6). Additives such as TMEDA, ZnI2, silica gel or AgOAc did not afford better results (Table 1, entries 7–10). Thus, we defined the reaction of BnNH2 with 2 equiv. of Cu(OAc)2 in PhCN at 120 °C (oil bath) as Method A (Table 1, entry 2) and 120 °C (microwave reactor) as Method B (Table 1, entry 3).
Table 1 Optimization of reaction conditionsa
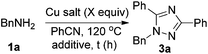
|
Entry |
Cu salts (equiv.) |
Solvent |
Additive (equiv.) |
t (h) |
Isolated yields of 3a (%) |
The reaction was conducted with 1 mmol of BnNH2 in 2 mL of PhCN in an oil bath at 120 °C, unless otherwise noted.
The reaction was conducted with 1 mmol of BnNH2 in 2 mL of PhCN in an oil bath at 100 °C.
The reaction was conducted with 1 mmol of BnNH2 in 5 mL of PhCN under microwave.
NMR yield determined by 1H NMR analysis with CH2Br2 as the internal standard.
4 equiv. of PhCN and 4 mL of DMSO were added.
100 mg of silica gel was added.
|
1b |
Cu(OAc)2 (2) |
PhCN |
— |
60 |
38 |
2 |
Cu(OAc)2 (2) |
PhCN |
— |
21 |
44 |
3c |
Cu(OAc)2 (2) |
PhCN |
— |
0.5 |
41 |
4c |
Cu(OAc)2 (1) |
PhCN |
— |
1 |
36d |
5 |
CuI (3) |
PhCN |
— |
17 |
3 |
6e |
Cu(OAc)2 (3) |
DMSO |
— |
16 |
0 |
7 |
Cu(OAc)2 (2) |
PhCN |
TMEDA (2) |
20 |
20 |
8 |
Cu(OAc)2 (2) |
PhCN |
ZnI2 (0.2) |
52 |
4 |
9 |
Cu(OAc)2 (2) |
PhCN |
Silica gelf |
47 |
37 |
10 |
Cu(OAc)2 (0.05) |
PhCN |
AgOAc (3) |
59 |
21 |
The scope of the reaction is described in Table 2. Various n-alkyl and benzyl amines may react with phenyl nitriles affording the corresponding fully substituted 1,2,4-triazoles in moderate yields (entries 1–13). It should be noted that the reaction could be complete within 21–48 h at 120 °C (oil bath) or within just 0.5 h at 120 °C in a microwave reactor. Substituted aromatic nitriles may also be applied in this reaction (entries 14–28). The desired product was not observed when alkyl nitriles (such as CH3CN) are used which indicates that aromatic nitrile is crucial for this transformation. The possible side reactions are the formation of some polymers or some copper complexes, which explains the low yields shown in Table 2.
Table 2 Substrate scope: the Cu(OAc)2-mediated cyclization reaction of amines with two molecules of aryl nitriles
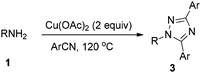
|
Entry |
R |
Ar |
Method |
T (h) |
Isolated yields of 3 (%) |
Method A: the reaction was conducted with 1.0 mmol of an amine and 2.0 mmol of Cu(OAc)2 in 2 mL of nitrile at 120 °C (oil bath); Method B: the reaction was conducted with 1.0 mmol of an amine and 2.0 mmol of Cu(OAc)2 in 5 mL of nitrile at 120 °C (MW). The reaction was conducted with 10 mmol of an amine affording a gram scale of 1,2,4-triazole. |
1 |
Bn |
Ph |
A |
21 |
44 (3a) |
2 |
Bn |
Ph |
A |
48 |
42 (3a)a |
3 |
Bn |
Ph |
B |
0.5 |
41 (3a) |
4 |
p-FBn |
Ph |
B |
0.5 |
45 (3b) |
5 |
n-Bu |
Ph |
A |
28 |
45 (3c) |
6 |
n-Bu |
Ph |
B |
0.5 |
53 (3c) |
7 |
i-Bu |
Ph |
B |
0.5 |
47 (3d) |
8 |
n-C5H11 |
Ph |
B |
0.5 |
46 (3e) |
9 |
Cy |
Ph |
B |
0.5 |
45 (3f) |
10 |
n-C6H13 |
Ph |
B |
0.5 |
48 (3g) |
11 |
n-C8H17 |
Ph |
A |
24 |
43 (3h) |
12 |
n-C8H17 |
Ph |
B |
0.5 |
50 (3h) |
13 |
n-C12H25 |
Ph |
B |
0.5 |
55 (3i) |
14 |
Bn |
m-MeC6H4 |
A |
48 |
48 (3j) |
15 |
Bn |
m-MeC6H4 |
B |
0.5 |
37 (3j) |
16 |
Bn |
p-F C6H4 |
A |
48 |
43 (3k) |
17 |
Bn |
p-F C6H4 |
B |
0.5 |
41 (3k) |
18 |
n-C8H17 |
p-F C6H4 |
A |
48 |
50 (3l) |
19 |
n-C8H17 |
p-F C6H4 |
B |
1 |
46 (3l) |
20 |
n-C8H17 |
p-MeC6H4 |
A |
48 |
54 (3m) |
21 |
n-C8H17 |
p-MeC6H4 |
B |
1 |
39 (3m) |
22 |
n-C8H17 |
o-FC6H4 |
B |
1 |
42 (3n) |
23 |
Bn |
m-MeOC6H4 |
B |
0.5 |
30 (3o) |
24 |
Bn |
p-CF3C6H4 |
B |
0.5 |
28 (3p) |
25 |
Bn |
p-ClC6H4 |
B |
0.5 |
45 (3q) |
26 |
Bn |
p-MeO2CC6H4 |
B |
0.5 |
12 (3r) |
27 |
Bn |
p-MeOC6H4 |
B |
0.5 |
13 (3s) |
28 |
Bn |
m-IC6H4 |
B |
0.5 |
20 (3t) |
The structure of the product has been further confirmed by X-ray single-crystal diffraction analysis of 3f (Fig. 1).7 In addition, the reaction could be easily conducted affording a gram scale of product 3a in a similar yield (Table 2, entry 1 vs. entry 2).
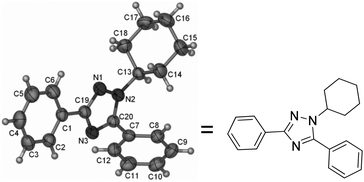 |
| Fig. 1 ORTEP presentation of 3f. | |
A possible mechanism has been proposed (Scheme 2). The direct addition of nitrile with amine mediated by Cu2+ may give imidamide intermediate Int1.8 Subsequent addition of another molecule of Cu-coordinated nitrile affords Int2. The formed Int2 would react with Cu(OAc)2 to produce metallacyclic intermediate Int3 by releasing an extra molecule of HOAc. Subsequent reductive elimination furnishes the 1,2,4-triazole product 3.
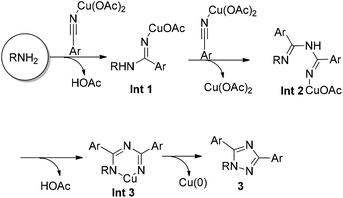 |
| Scheme 2 Possible mechanism for the synthesis of 1,2,4-triazoles. | |
In order to support the proposed mechanism, substituted benzamidine 4 was prepared according to the literature.8b Indeed, 1,2,4-triazoles 3u–3w were afforded when 4 was exposed to different nitriles following Method B (Scheme 3), which also allows access to “non-symmetrical” 1,2,4-triazoles. The formation of symmetric products 3j, 3q, and 3t indicates that the formation of a 4-type intermediate from an amine and a nitrile is reversible.
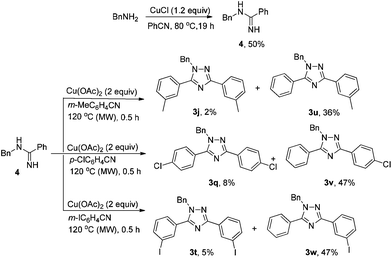 |
| Scheme 3 Synthesis of non-symmetrically substituted 1,2,4-triazoles 3u–3w. | |
The structure of these non-symmetrical products has been further confirmed by X-ray single-crystal diffraction analysis of 3w (Fig. 2).9
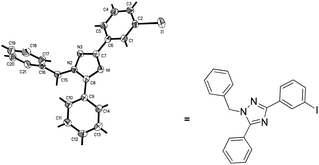 |
| Fig. 2 ORTEP presentation of 3w. | |
In conclusion, we have developed a facile reaction affording fully substituted 1,2,4-triazoles from one molecule of amine and two molecules of nitriles. A salient feature of this reaction is the utilization of easily available amines and nitriles for the synthesis of 1,2,4-triazoles, which may have a promising application in industrial synthesis for the 1,2,4-triazole drug or fungicide candidates. Considering the easy availability of starting materials and potential of the products,1 this method will be of high interest in organic chemistry and medicinal chemistry. Further studies in this area are being actively conducted in our laboratory.
Experimental
1-Benzyl-3,5-diphenyl-1H-1,2,4-triazole (3a)
Method A.
To a dried Schlenk tube were added Cu(OAc)2 (367.9 mg, 2 mmol) and BnNH2 (108.6 mg, 1 mmol)/PhCN (2 mL) sequentially at room temperature. After stirring at 120 °C (oil bath) for 21 h, the resulting mixture was cooled to room temperature, diluted with 50 mL of Et2O, filtered through a short pad of silica gel and concentrated under reduced pressure. The residue was purified by chromatography on silica gel (eluent: petroleum ether–ethyl acetate = 40/1 to 10/1) to afford 3a (139.7 mg, 44%): solid; 1H NMR (300 MHz, CDCl3) δ 8.25–8.14 (m, 2 H, ArH), 7.66–7.57 (m, 2 H, ArH), 7.52–7.16 (m, 11 H, ArH), 5.40 (s, 2 H, CH2).
Gram-scale synthesis of 3a
To a dried three-necked flask were added Cu(OAc)2 (3.6321 g, 20 mmol) and BnNH2 (1.0704 g, 10 mmol)/PhCN (20 mL) sequentially at room temperature. After stirring at 120 °C (oil bath) for 48 h, the resulting mixture was cooled to room temperature, diluted with 50 mL of Et2O, filtered through a short pad of silica gel and concentrated under reduced pressure. The residue was purified by chromatography on silica gel (eluent: petroleum ether–ethyl acetate = 30/1 to 15/1) to afford 3a (1.3062 g, 42%): solid; 1H NMR (300 MHz, CDCl3) δ 8.27–8.14 (m, 2 H, ArH), 7.64–7.52 (m, 2 H, ArH), 7.51–7.27 (m, 9 H, ArH), 7.25–7.16 (m, 2 H, ArH), 5.46 (s, 2 H, CH2).
Method B.
To a dried MW tube were added Cu(OAc)2 (363.9 mg, 2 mmol) and BnNH2 (106.8 mg, 1 mmol)/PhCN (5 mL) sequentially at room temperature. The tube was sealed and irradiated in a microwave reactor (120 °C, 30 minutes, maximum power 800 W). Then, the resulting mixture was cooled to room temperature with a stream of air, diluted with 50 mL of Et2O, filtered through a short pad of silica gel and concentrated under reduced pressure. The residue was purified by chromatography on silica gel (eluent: petroleum ether–ethyl acetate = 10/1) to afford 3a10 (127.0 mg, 41%): solid; m.p. 99–100 °C (hexane–Et2O) (lit. m.p. 98.5–99.5 °C); 1H NMR (300 MHz, CDCl3) δ 8.21 (d, J = 7.2 Hz, 2 H, ArH), 7.62–7.51 (m, 2 H, ArH), 7.48–7.13 (m, 11 H, ArH), 5.39 (s, 2 H, CH2); 13C NMR (CDCl3, 75 MHz) δ 161.4, 156.0, 135.9, 130.9, 130.0, 129.0, 128.70, 128.67, 128.6, 128.4, 127.9, 127.8, 126.6, 126.3, 52.6. IR (neat) 1519, 1497, 1476, 1463, 1443, 1406, 1353 cm−1; MS (EI) (m/z) 312 ((M + 1)+, 5.71), 311 (M+, 27.09), 91 (100).
Acknowledgements
Financial support from the National Natural Science Foundation of China (21232006) and the National Basic Research Program of China (2011CB808700) is greatly appreciated. We thank Miss Xue Can of this group for reproducing the results presented in entry 14 of Table 2.
Notes and references
- For reviews on the synthesis and reactions of 1,2,4-triazoles, see:
(a) K. T. Potts, Chem. Rev., 1961, 61, 87–127 CrossRef CAS;
(b) M. Balasubramanian, J. G. Keay and E. F. V. Scriven, Heterocycles, 1994, 37, 1951–1975 CrossRef CAS;
(c) I. A. Al-Masoudi, Y. A. Al-Souda, N. J. Al-Salihi and N. A. Al-Masoudi, Khim. Geterotsikl. Soedin., 2006, 1605–1634 Search PubMed;
(d) A. Moulin, M. Bibian, A.-L. Blayo, S. El Habnouni, J. Martinez and J.-A. Fehrentz, Chem. Rev., 2010, 110, 1809–1827 CrossRef CAS PubMed;
(e) S. C. Holm and B. F. Straub, Org. Prep. Proced. Int., 2011, 43, 319–347 CrossRef CAS.
- For the synthesis of unsubstituted parent 1,2,4-triazoles, see:
(a) C. Ainsworth and R. G. Jones, J. Am. Chem. Soc., 1955, 77, 621–624 CrossRef CAS;
(b) C. Grundmann and R. Ratz, J. Org. Chem., 1956, 21, 1037–1038 CrossRef CAS;
(c) C. Ainsworth, Org. Synth., 1973, 5, 1070 Search PubMed;
(d)
H. E. Petree, J. R. Pociask and J. T. Gupton, US4267347, 1981 Search PubMed;
(e)
K. Harald, M. Thomas, Mildenberger Hilmar and K. Helmut, US4283545 A1, 1981 Search PubMed.
- For some of the most recent reports on the synthesis of substituted 1,2,4-triazoles, see:
(a) G. M. Makara, Y. Ma and L. Margarida, Org. Lett., 2002, 4, 1751–1754 CrossRef CAS PubMed;
(b) M. J. Stocks, D. R. Cheshire and R. Reynolds, Org. Lett., 2004, 6, 2969–2971 CrossRef CAS PubMed;
(c) J. Balsells, L. DiMichele, J. Liu, M. Kubryk, K. Hansen and J. D. Armstrong, Org. Lett., 2005, 7, 1039–1042 CrossRef CAS PubMed;
(d) W. K. Su, D. W. Yang and J. J. Li, Synth. Commun., 2005, 35, 1435–1440 CrossRef CAS PubMed;
(e) K. S. Yeung, M. E. Farkas, J. F. Kadow and N. A. Meanwell, Tetrahedron Lett., 2005, 46, 3429–3432 CrossRef CAS PubMed;
(f) S. Ueda and H. Nagasawa, J. Am. Chem. Soc., 2009, 131, 15080–15081 CrossRef CAS PubMed;
(g) J. Z. Vlahakis, C. Lazar, I. E. Crandall and W. A. Szarek, Bioorg. Med. Chem., 2010, 18, 6184–6196 CrossRef CAS PubMed;
(h) G. M. Castanedo, P. S. Seng, N. Blaquiere, S. Trapp and S. T. Staben, J. Org. Chem., 2011, 76, 1177–1179 CrossRef CAS PubMed;
(i) M. M. Guru and T. Punniyamurthy, J. Org. Chem., 2012, 77, 5063–5073 CrossRef CAS PubMed.
-
(a) J. T. A. Boyle and M. F. Grundon, Chem. Commun., 1967, 1137–1138 RSC;
(b) J. T. A. Boyle, M. F. Grundon and M. D. Scott, J. Chem. Soc., Perkin Trans. 1, 1976, 207–212 RSC;
(c) L. L. Whitfield and E. P. Papadopoulos, J. Heterocycl. Chem., 1981, 18, 1197–1201 CrossRef CAS;
(d) P. H. J. Carlsen, Acta Chem. Scand., 1987, 41B, 302–303 CrossRef PubMed;
(e) P. H. J. Carlsen and O. R. Gautun, Acta Chem. Scand., 1990, 44, 485–488 CrossRef CAS PubMed;
(f) O. R. Gautun and P. H. J. Carlsen, Acta Chem. Scand., 1991, 45, 609–615 CrossRef CAS PubMed;
(g) O. R. Gautun and P. H. J. Carlsen, Acta Chem. Scand., 1992, 46, 469–473 CrossRef CAS PubMed;
(h) P. H. J. Carlsen and K. B. Jørgensen, J. Heterocycl. Chem., 1997, 34, 797–806 CrossRef CAS;
(i) K. Paulvannan, T. Chen and R. Hale, Tetrahedron, 2000, 56, 8071–8076 CrossRef CAS.
- For most recent reviews on the chemistry of allenes, see:
(a) S. Ma, Chem. Rev., 2005, 105, 2829–2871 CrossRef PubMed;
(b) S. Ma, Aldrichimica Acta, 2007, 40, 91–102 CAS;
(c) M. Brasholz, H.-U. Reissig and R. Zimmer, Acc. Chem. Res., 2008, 42, 45–56 CrossRef PubMed;
(d) S. Ma, Acc. Chem. Res., 2009, 42, 1679–1688 CrossRef CAS PubMed;
(e) B. Alcaide, P. Almendros and T. M. d. Campo, Chem.–Eur. J., 2010, 16, 5836–5842 CrossRef CAS PubMed;
(f) C. Aubert, L. Fensterbank, P. Garcia, M. Malacria and A. Simonneau, Chem. Rev., 2011, 111, 1954–1993 CrossRef CAS PubMed;
(g) F. López and J. L. Mascareñas, Chem.–Eur. J., 2011, 17, 418–428 CrossRef PubMed;
(h) S. Yu and S. Ma, Angew. Chem., Int. Ed., 2012, 51, 3074–3112 CrossRef CAS PubMed;
(i) A. Hoffmann-Röder and N. Krause, Angew. Chem., Int. Ed., 2004, 43, 1196–1216 CrossRef PubMed;
(j) S. Yu and S. Ma, Chem. Commun., 2011, 47, 5384–5418 RSC;
(k) N. Krause and C. Winter, Chem. Rev., 2011, 111, 1994–2009 CrossRef CAS PubMed;
(l) B. Alcaide, P. Almendros and C. Aragoncillo, Chem. Soc. Rev., 2010, 39, 783–816 RSC . For a monograph, see:
N. Krause and A. S. K. Hashmi, Modern Allene Chemistry, Wiley-VCH, Weinheim, 2004 Search PubMed.
- For reports on the addition reactions of allenoates with amines, see:
(a) Y. Troin, M.-E. Sinibaldi, J.-C. Gramain, M. Rubiralta and A. Diez, Tetrahedron Lett., 1991, 32, 6129–6132 CrossRef CAS;
(b) G. J. S. Doad, D. I. Okor, F. Scheinmann, P. A. Bates and M. B. Hursthouse, J. Chem. Soc., Perkin Trans. 1, 1988, 2993–3003 RSC.
- Crystal data for 3f: C20H21N3, MW = 303.40, orthorhombic; space group Pbca, final R indices [I > 2σ(I)], R1 = 0.0379, wR2 = 0.0804; R indices (all data) R1 = 0.0680, wR2 = 0.0943, a = 15.3067(9) Å, b = 12.1443(7) Å, c = 18.1692(10) Å, α = 90°, β = 90°, γ = 90°, V = 3377.5(3) A3, T = 296(2) K, Z = 8, reflections collected/unique 37
170/2975 [R(int) = 0.0709], number of observations [>2σ(I)] 2058, parameters: 208. Supplementary crystallographic data have been deposited at the Cambridge Crystallographic Data Center. CCDC 940098.
-
(a) A. Marsura, C. Luu-Duc and G. Gellon, Synthesis, 1985, 537–541 CrossRef CAS;
(b) G. Rousselet, P. Capdevielle and M. Maumy, Tetrahedron Lett., 1993, 34, 6395–6398 CrossRef CAS;
(c) N. R. Curtis, H. J. Diggle, J. J. Kulagowski, C. London, S. Grimwood, P. H. Hutson, F. Murray, P. Richards, A. Macaulay and K. A. Wafford, Bioorg. Med. Chem. Lett., 2003, 13, 693–696 CrossRef CAS.
- Crystal data for 3w: C21H16IN3, MW = 437.27, monoclinic; space group P21/c, final R indices [I > 2σ(I)], R1 = 0.0392, wR2 = 0.0975; R indices (all data) R1 = 0.0543, wR2 = 0.1069, a = 12.2051(11) Å, b = 18.0249(18) Å, c = 8.7754(8) Å, α = 90°, β = 110.387(2)°, γ = 90o, V = 1809.6(3) A3, T = 293(2) K, Z = 4, reflections collected/unique 10
832/3550 [R(int) = 0.0334], number of observations [>2σ(I)] 2687, parameters: 226. Supplementary crystallographic data have been deposited at the Cambridge Crystallographic Data Center. CCDC 978691.
- L. L. Whitfield and E. P. Papadopoulos, J. Heterocycl. Chem., 1981, 18, 1197–1201 CrossRef CAS.
Footnotes |
† Dedicated to Max Malacria on the occasion of his 65th birthday. |
‡ Electronic supplementary information (ESI) available. CCDC 940098 and 978691. For ESI and crystallographic data in CIF or other electronic format see DOI: 10.1039/c4qo00007b |
|
This journal is © the Partner Organisations 2014 |