Probing the anti-aging role of polydatin in Caenorhabditis elegans on a chip
Received
19th September 2013
, Accepted 27th October 2013
First published on 29th October 2013
Abstract
C. elegans is widely used as a model organism in the study of aging and evaluation of anti-aging drugs due to its unique characteristics. In this work, we set out to investigate polydatin, a natural resveratrol glycoside, and its role in extending lifespan, improving oxidative stress resistance, and the possible regulation mechanism involved in the Insulin/IGF-1 signaling (IIS) pathway for the first time by using a flexible microfluidic device. The effects of polydatin on the lifespan, oxidative stress resistance, mobility and the expression of aging-related proteins and genes were explored. Polydatin was found to significantly extend the mean lifespan of worms by up to 30.7% and 62.1% under normal and acute stress conditions respectively. It improved the expression of the inducible oxidative stress protein (GST-4) and corresponding stroke frequencies in the transgenic CL2166 strain. Moreover, it also increased SOD-3::GFP expression in CF1553 worms and promoted DAF-16 nucleus translocation in TJ356 worms. The longevity-extending role of polydatin is partly attributed to its anti-oxidative activity and increased oxidative stress resistance by regulating the stress-resistance related proteins SOD-3, and daf-16 expression at protein and mRNA levels involved in the IIS pathway. The established microfluidic platform is capable of flexible operation with multiple functions, which not only supports the individual worm's long-term culture with sufficient nutrient exchange, but also facilitates mobility monitoring of the worm, immobilizing and imaging in a controllable and parallel manner. These interesting findings reported here highlight the significance of the natural compound polydatin in the study of aging-related diseases, and the utility of the microfluidic platform for applications in aging studies.
Insight, innovation, integration
Polydatin, a natural resveratrol glycoside, was investigated to significantly extend the lifespan of C. elegans and increase stress resistance under different conditions on a microfluidic device. The lifespan-extension role of polydatin is mainly attributed to its anti-oxidative activity by regulating the aging-associated genes daf-16 and stress-resistance protein SOD-3 involved in insulin/IGF-1 signaling pathway. These interesting findings address the potential of polydatin to extend human average life expectancy and highlight the significance of polydatin in drug development and invention therapy in the study of aging and aging-related diseases. The functional microfluidic device provides a suitable platform for aging-related research in C. elegans by integrating micro-scale engineering technique with biomedical applications.
|
Introduction
Aging is characterized by general physiological declines over time, therefore these are of great interest in the study of aging-related diseases.1–3Caenorhabditis elegans (C. elegans) is a small free-living soil nematode widely used as a model organism in the study of aging owing to its unique features such as a fully sequenced genome, short lifespan (2–3 weeks) and lifecycle (3 days), and ease of propagation of individuals in a population.4,5 Significant progress has been made by using the model organism C. elegans to delineate the genetic and biochemical pathways involved in aging-related diseases and to identify effective strategies for therapeutic intervention in humans.6,7 Importantly, C. elegans shares many similarities with mammals and humans, including aging and functional senescence, thus facilitating the discovery of new compounds and effective evaluation of lifespan-extension drugs in pharmacology.
Until now, several chemical compounds have been found to promote lifespan-extension in C. elegans.8 Resveratrol is a naturally isolated polyphenol and is particularly found in grape skin, nuts, etc. It has been reported that polyphenol-rich resveratrol enabled the extension of lifespan by inhibiting the repressive activity of sir-2.1 genes in several model organisms, such as yeast and Drosophila, zebrafish and C. elegans.9–11 It is known to have various beneficial anti-cancer and anti-inflammatory effects.12–14 In contrast, polydatin, also know as 3,4′,5-trihydroxystibene-3-β-D-mono-D-glucoside, is the glycoside form of resveratrol, in which the glycoside group bonded in position C-3 substitutes a hydroxyl group.15 It was found that in grape juice, the average concentration of polydatin (the glycoside of resveratrol) is seven times that of resveratrol and polydatin is regarded as the most abundant form of resveratrol in nature.16,17 Several works have reported the beneficial and biological effects of polydatin in mice models, including the neuroprotective effect on ischemia/reperfusion induced cerebral injury, promotion of weight loss, and protection of the primarily cultured rat hepatocytes against CCl4-induced injury.18–22 Recent studies also verified the role of polydatin against lipid peroxidation with a slower but prolonged protective action as compared to resveratrol.16 We previously found that polydatin could extend the longevity of C. elegans significantly,23 but the mechanism underlying the anti-aging effect of polydatin is unclear.
In this work, we set out to investigate the role of polydatin in extending lifespan, improving oxidative stress resistance, and its possible regulation mechanism involved in the insulin/IGF-1 signaling (IIS) pathway by using a flexible microfluidic device. The lifespan-extending and anti-oxidative functions of polydatin in vivo were identified under normal and acute stress conditions. The anti-aging role associated with stress-resistant proteins was explored in series of transgenic worms. The regulative effects of polydatin on the mRNA expression of aging-associated genes daf-16, sod-3, daf-2, skn-1 and sir-2.1 were investigated as well. The lifespan-extension ability of polydatin is mainly attributed to its protective effects against oxidative stress, and the up-regulation of daf-16 expression involved in the IIS pathway.
Experimental
1. Worms strains and maintenance
C. elegans wild-type N2 and all transgenic strains in this study were obtained from the Caenorhabditis Genetics Center (CGC) at the University of Minnesota (St. Paul). The transgenic strain CL2166 (dvIs19) containing gst-4 reporter was used to illustrate the inducible oxidative stress in worms. The CF1553 strain (muIs84) was used to visualize SOD-3 expression by the SOD-3::GFP-linked reporter. The TJ356 strain (zIs356 IV) containing DAF-16::GFP reporter was used to indicate DAF-16 expression.
All strains were cultivated as described by Brenner.24 Briefly, worms were cultivated at 20 °C on standard nematode growth medium (NGM) agar seeded with Escherichia coli (E. coli) OP50. Prior to seeding, bacteria were incubated overnight at 37 °C and stored at 4 °C.
2. Fabrication and design of microfluidic device
The microfluidic chip was fabricated in PDMS (poly(dimethylsiloxane)), which is gas permeable, transparent, non-fluorescent and biocompatible with living organisms.25 The chip was composed of three layers: a top flow layer, PDMS membrane and bottom control layer. The schematic illustration of a single unit of the chip is shown in Fig. 1(A). The top flow layer contained 30 worm manipulation units for worm culture and imaging. The deformable PDMS membrane (40 μm thick) was assembled between the flow layer and control layer. By activating two microvalves in the control layer, the PDMS membrane deformed and worms could be individually loaded or immobilized, as shown in Fig. 1(B).
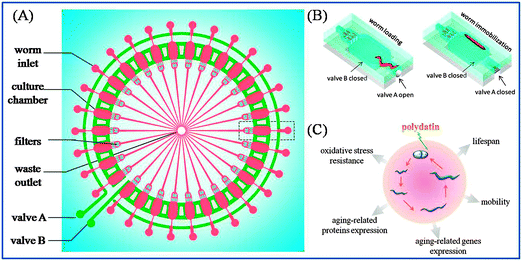 |
| Fig. 1 Schematic showing the microfluidic chip used for anti-aging drug evaluation in C. elegans. (A) The design of the chip which is composed of different functional units for worm long-term culture, mobility monitoring and imaging immobilization. (B) Magnified illustration of the microvalve operation unit for worm loading and immobilization in (A). (C) The investigated parameters of worms involved in anti-aging process influenced by polydatin. | |
3. Lifespan assays in worms
The microfluidic device was fabricated as described above and the lifespan assays were performed at 20 °C. Age-synchronous populations were prepared, as previously described.26 Briefly, collected eggs were allowed to hatch overnight at 20 °C on fresh nematode growth medium (NGM) agar plates.27 Hatched worms (L1 stage) were transferred to K medium (50 mM NaCl, 30 mM KCl, 10 mM NaOAc, pH 5.5) in microtiter plates for treatment with polydatin for 24 h. The concentration of polydatin was 0.01, 0.1 and 1 mM. The worms were then cultured at 20 °C with Escherichia coli strain OP50 as a food source, until reaching L4 stage. L4 worms were loaded into the microfluidic chip for survival monitoring and fluorescence imaging. Oxidative stress assays were performed at 20 °C by using adult worms. The worms were loaded into the chip and treated with 500 μM Cu2+ for 24 h. For all assays, the survival rate, mobility behavior and fluorescence features of worms were monitored each day.
4. Fluorescence visualization and quantification
Green fluorescence of CL2166, CF1553 and TJ356 worms was examined using an inverted fluorescent microscope (Olympus IX 71, Japan). The excitation and detection wavelengths were set at 470–495 nm and 510–550 nm, respectively. The fluorescence images were analyzed using image processing and analysis software (IMAGE-PRO, Media Cybernetics, USA).
5. Quantitative real-time PCR
L1 worms were treated with various concentrations of polydatin for 24 h. Subsequently, total RNA was extracted from the adult worms according to the Trizol treatment procedure. cDNA was produced by oligo (dT) priming. The PCR primers were as follows:
daf-16, 5′-GCGAATCGGTTCCAGCAATTCCAA-3′ and 5′-ATCCACGGACACTGTTCAACTCGT-3′; sod-3, 5′-AGCATCATGCCACCTACGTGA-3′ and 5′-CACCACCATTGAATTTCAGCG-3′; daf-2, 5′-GGCCGATGGACGTTATTTTG-3′ and 5′-TTCCACAGTGAAGAAGCCTGG-3′; skn-1, 5′-AGTGTCGGCGTTCCAGATTTC-3′ and 5′-GTCGACGAATCTTGCGAATCA-3′; act-1, 5′-CCAGGAATTGCTGATCGTATGCAGAA-3′ and 5′-TGGAGAGGGAAGCGAGGATAGA-3′.
act-1 was used as the internal control. mRNA expression was assessed by means of a real-time PCR detection system (Agilent Mx3000P) using SYBR green as the detection method. The gene expression data were analyzed using the comparative 2−ΔΔCt method, using act-1 mRNA as the normalizer.
Results
1. Extending the lifespan of C. elegans in wild-type N2 worms under normal and acute stress conditions
Initially, the influence of polydatin on the lifespan of wild-type N2 worms was evaluated. Populations of worms were treated with various concentrations of polydatin at L1 stage for 24 h. The individual worms were loaded and cultured in the microfluidic chip at L4 stage. We compared the lifespan of N2 worms after exposure to polydatin under normal condition and heavy metal stress condition. According to the design of the microfluidic chip, 30 worms could be individually treated, observed and imaged in parallel. Subsequently, the survival rates of all worms were monitored in three independent experiments every day. The experimental conditions used for the evaluation of polydatin on the lifespan of N2 worms is shown in Table 1, and the effect of polydatin on the lifespan of N2 worms under different concentrations (0, 0.01, 0.1, 1 mM) is shown in Fig. 2. In this work, the worms were maintained on the chip for up to 35 days with good viability. From Fig. 2(A), it can be seen that the mean lifespan of N2 worms was about 14 days under normal culture condition, but the mean lifespan could be extended to 16, 17 and 19 days after exposure to polydatin at various concentrations. The lifespan-extension percentages are 11.4%, 13.6% and 30.7%, respectively. As a comparison, we also investigated the lifespan-extending effect of polydatin under an acute oxidative stress condition induced by a heavy metal. Under the stress condition, the adult worms were cultured in the chip followed by exposure to 500 μM Cu2+ for up to 24 h. It was noted that, the average lifespan of worms was shortened significantly from 14 days to 8 days after treatment with heavy metal. Whereas polydatin could significantly rescue the mean lifespan under stress condition as shown in Fig. 2(B). The mean lifespan was calculated as 9, 10 and 12.5 days after polydatin treatment, which compared to untreated worms show an improvement of 17.1%, 26.6% and 62.1%, respectively. Based on the obtained results, it was observed that polydatin could extend the lifespan of C. elegans under both normal and stress conditions.
Table 1 Lifespan of N2 worms treated with polydatin under different experimental conditions
Trial |
Genotype |
Treatment (20 °C, mM) |
Total (N) |
Lifespan (days) |
P-value |
|
|
Mean |
SEMa |
Standard error of the mean.
|
Normal condition |
N2 |
Control |
30 |
14.3 |
1.09 |
0.036 |
Polydatin 0.01 |
30 |
15.9 |
1.11 |
0.0487 |
Polydatin 0.1 |
30 |
16.8 |
1.18 |
0.0157 |
Polydatin 1 |
30 |
18.6 |
2.31 |
0.0549 |
Oxidative stress condition |
N2 |
Control |
30 |
7.74 |
1.12 |
0.0474 |
Polydatin 0.01 |
30 |
9.06 |
1.17 |
0.0355 |
Polydatin 0.1 |
30 |
9.8 |
1.26 |
0.0573 |
Polydatin 1 |
30 |
12.5 |
2.62 |
0.0429 |
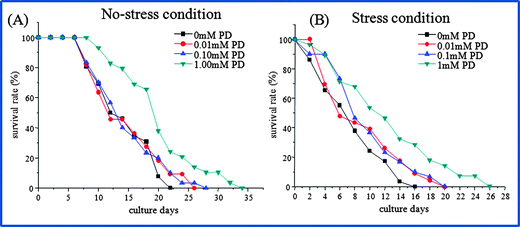 |
| Fig. 2 The effects of polydatin on the lifespan-extension of N2 worms under (A) normal culture condition without stress and (B) stress condition. The day that l4 worms were loaded into chip was defined as day 0. For the stress condition, adult worms were exposed to heavy metal at a concentration of 500 μm Cu2+ for 24 h. | |
2. Reducing expression of oxidative stress protein in transgenic CL2166 worms
Oxidative stress plays a critical role in many diseases, and is also considered to be one of the determinants related to worm's lifespan.28,29 As reported, excessive reactive oxygen species (ROS) can accelerate the aging process of organisms. To explore whether polydatin has antioxidative properties in vivo, transgenic gst-4::GFP nematodes CL2166 was employed. Prior to assay, CL2166 worms were divided into four groups for treatment with polydatin under different conditions. While weak GFP expression could be measured in gst-4::GFP worms under normal culture conditions, heavy metal Cu2+ could increase the intracellular ROS level, which in turn induced the expression of gst-4. Worms, which were exposed to a heavy metal, showed a strong GFP expression in their body.
Fig. 3 shows the fluorescence photographs and stroke frequency of individual CL2166 worms treated with polydatin under normal and stress conditions. As shown in Fig. 3(A), the inducible GFP expression in the transgenic CL2166 strain was used to represent the oxidative level produced in treated worms. Under normal culture condition, polydatin treated worms showed weaker fluorescence intensity than non-treated worms, indicating the decreased oxidative stress level in worms by polydatin. Moreover, Cu2+ treated worms exhibited higher fluorescence intensity in the body than non-treated worms, indicating the increased oxidative stress level in this condition. The expression of gst-4::GFP was reduced significantly in the body of individual worms pretreated with 1 mM polydatin. This finding implies that polydatin enhanced oxidative stress resistance of worms under heavy metal conditions. It also provides evidence that polydatin is bio-available, which has been absorbed by the worms. As previously reported, excess free radicals in the muscle could cause slow movement of the organisms.30 Therefore, in the following work, we compared the expression of oxidative stress proteins with the associated mobility behaviors in polydatin-treated worms under different conditions. The stroke frequency of the individual worms was used as an index to represent the movement activity of the worms. Fig. 3(B) illustrates the distribution of stroke frequency of 10 individual worms in different groups. The polydatin treated worms were observed to show higher stroke frequency compared with non-polydatin treated worms, and this trend was found in both normal and oxidative stress conditions, thus indicating the supporting role of polydatin to maintain higher mobility activity in worms. In particular, it implies that the oxidative stress level of the worms is closely related to their movement capabilities under the specified conditions.
 |
| Fig. 3 Expression of inducible oxidative stress protein and corresponding stroke frequencies in individual CL2166 worms exposed to polydatin under different culture conditions. (A) Fluorescent images of CL2166 worms in different conditions at day 3. (B) Quantitative results of gst-4::GFP expression in CL2166 worms during the aging process. (C) Stroke frequency of worms in each group. The L1 larvae worms were treated with 1 mm polydatin for 24 h. Fluorescence intensity and stroke frequency in each group were calculated based on three individual experiments (n = 10). Error bars indicate the SEM relative to the control: *p < 0.05, **p < 0.01. | |
3. Involvement of aging associated-proteins and genes
As above, polydatin has been demonstrated to prolong lifespan and decrease inner oxidative stress levels in worms. In the following study, we further investigated the anti-aging function of polydatin by evaluating the expression of stress-associated proteins and genes, respectively.
3.1 Forkhead transcription factor DAF-16 nuclear translocation in transgenic TJ356 worms.
The reduced insulin/IGF-1 signaling (IIS) pathway has been implicated in aging and stress resistance.6 DAF-16 forkhead transcription factor is the downstream target of the insulin/IGF-1 signaling pathway in C. elegans, which is essential for prolonging lifespan and stress resistance.31 As previously reported, the transcription factor DAF-16 is commonly expressed in the cytoplasm of worms’ body cells. Inactivation of upstream kinases in the IIS pathway results in nuclear translocation of DAF-16 from cytoplasm to nuclear. In this work, polydatin has demonstrated a positive effect in extending the lifespan and improving oxidative stress resistance in worms. In the following experiment, we further investigated whether polydatin affected DAF-16 expression in transgenic TJ356 worms. The fused GFP to daf-16 gene was used to visualize DAF-16 localization. In this experiment, DAF-16 localization was divided into three phenotypes according to the positions of worms in which green fluorescence was most apparent, named as “cytosolic”, “nuclear”, and “intermediate”, respectively. To explore the influence of polydatin on DAF-16 localization, TJ356 worms were exposed to different concentrations of polydatin (0.01, 0.1 and 1 mM). Fig. 4 shows the distribution of DAF-16 localization as the concentration of polydatin, and a dose dependent effect of the translocation ability of DAF-16 can be observed in the presence of polydatin. The treatment of C. elegans with polydatin significantly altered the subcellular distribution of DAF-16. At low concentrations of polydatin, DAF-16 translocation from cytoplasm to nucleus was observed only in a limited number of cells. A higher percentage of worms with DAF-16 translocation were observed after exposure to polydatin at a higher concentration. It is clear that polydatin can promote the translocation of DAF-16 from the cytoplasm to nucleus, which strongly indicates the active role of polydatin in regulating the DAF-16 forkhead transcription factor in the insulin/IGF-1 signaling pathway.
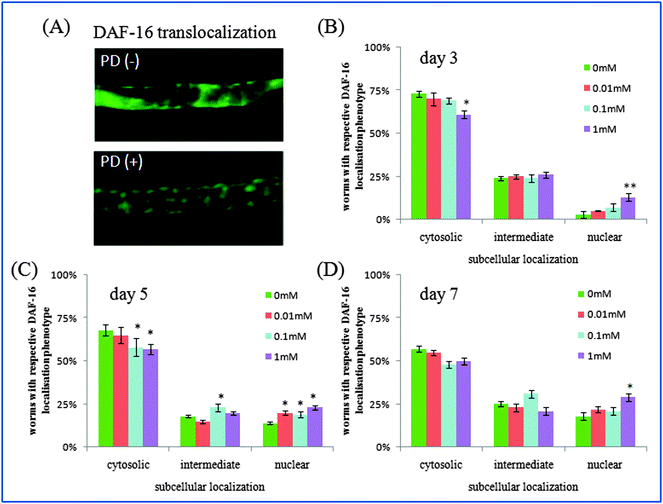 |
| Fig. 4 The effects of polydatin on the DAF-16 translocalization from cytoplasm to nucleus in TJ356 transgenic worms. (A) Image of DAF-16::GFP expression in control worms and 1 mm polydatin-treated worms. (B–D) The subcellular distributions of DAF-16 expression in worms at different time points treated with polydatin. According to DAF-16 localization, the worms were divided into three phenotypes including “cytosolic”, “intermediate” and “nuclear”. DAF-16 localization in each group was calculated based on three individual experiments (n = 10). Error bars indicate SEM relative to the control: * p < 0.05, ** p < 0.01. | |
3.2 Up-regulated SOD-3 expression in transgenic CF1553 worms.
During the insulin/IGF-1 signaling pathway, DAF-16 controls important proteins which are involved in the worms’ aging process.31 SOD-3 is one of the downstream proteins of DAF-16. It is a manganese superoxide dismutase which protects cells from oxidative stress and maintains the function to promote a normal lifespan.32 To further investigate whether SOD-3 expression is involved in the regulative role of polydatin under stress conditions, the transgenic SOD-3::GFP CF1553 worm was employed. Visualization of SOD-3::GFP expression in worms was used to examine the possible regulative effect of polydatin on the expression of the anti-oxidative enzyme SOD-3. The worms were treated with different concentrations of polydatin and the fluorescence intensities in each group of worms were calculated based on three individual experiments. As shown in Fig. 5, the worms demonstrated higher expression of SOD-3::GFP after 1 mM polydatin treatment at day 3, 5 and 7, as compared to that of the control group. This finding indicates that polydatin can significantly up-regulate the expression of oxidative stress protein SOD-3, which is probably regulated by activating ageing-related transcription factors involved in the IIS pathway.
 |
| Fig. 5 Characterization of SOD-3::GFP expression in transgenic strain CF1553 worms after exposure to polydatin at different concentrations. (A) Fluorescent image of SOD-3::GFP expression in polydatin-treated and non-treated worms. L1 larvae were exposed to 1 mm polydatin for 24 h. (B) Quantified GFP intensity in CF1553 strain in response to polydatin at different times. Fluorescence intensity in each group was calculated based on three individual experiments (n = 10). Error bars indicate the SEM relative to the control: *p < 0.05, **p < 0.01. | |
3.3 Regulation of mRNA expression in aging associated genes.
From the above experiments, it was verified that polydatin regulates protein expression of DAF-16 and SOD-3 involved in the insulin/IGF-1 signaling pathway. We further probed the regulative effect of polydatin by investigating several aging-associated genes at mRNA level. As we know, the insulin/IGF-1 pathway regulates the expression of free-radical detoxifying enzymes, which is consistent with free radical theories of aging and the involvement of mitochondria and ROS in this process.33 DAF-2 is the insulin/IGF-1 receptor that ultimately down-regulates DAF-16.31 The DAF-16 forkhead transcription factor has been shown to localize in the nucleus when the activity of the daf-2 signaling pathway is reduced. In addition, proteins SOD-3 and SKN-1 are also important regulators that can positively regulate lifespan and stress resistance in C. elegans. In the following experiment, we performed quantitative real-time PCR and investigated the expression of aging associated genes daf-2, daf-16, sod-3 and skn-1 in worms after polydatin treatment. As shown in Fig. 6, the mRNA expression of daf-2 was down-regulated and the expression of daf-16 was up-regulated significantly after polydatin treatment from 0.01 mM to 1 mM, while the expression of sod-3 and skn-1 were not affected. The mRNA expression of sir-2.1 in worms was not changed after polydatin treatment, indicating that polydatin regulated a different genetic pathway with resveratrol. The down-regulated daf-2 expression and up-regulated daf-16 expression in worms by polydatin explain the positive effect of this natural compound on stress resistance and lifespan in worms.
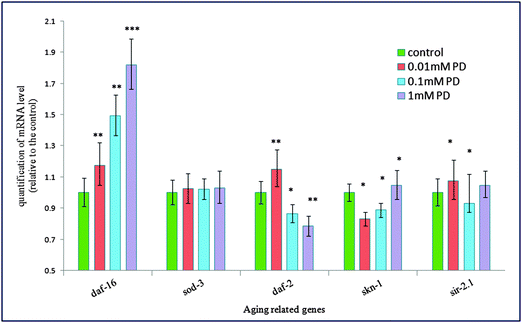 |
| Fig. 6 Effects of polydatin on the mRNA expression of aging-associated genes (daf-16, daf-2, sod-3, skn-1 and sir-2.1) in wild-type N2 worms. The worms in different groups were treated with 1 mm polydatin for 24 h. Error bars indicate the SEM relative to the control: *p < 0.05, **p < 0.01, ***p < 0.001. | |
Discussion
Despite great advances in genetics and molecular biology, the mysteries about the human lifespan are yet to be unravelled. Many theories have been proposed to explain the aging process, but none of them appears to be fully satisfactory.34 According to the ‘free radical aging theory’, which has been disputed by several authors,35 aging is the accumulation of oxidative damage to cells and tissues of organisms as a result of metabolism. Superoxide dismutase (SOD) has the capacity to decrease the concentration of superoxide anion radicals, and SOD might exert an anti-aging effect. This theory has been bolstered by experiments in which rodents fed antioxidants achieved an extended mean longevity. Antioxidants such as vitamin A, vitamin C, vitamin E and superoxide dismutase could slow the process of aging by preventing free radicals from oxidizing sensitive biological molecules or reducing the formation of free radicals. In this work, heavy metal induced acute oxidative stress resulted in a large amount of free radicals accumulating in cells. Polydatin diminished the levels of inducible oxidative protein, hence protecting the worms against oxidative stress.
In this work, polydatin was found to significantly extend the worms’ lifespan by up to 30.7% and 62.1% under normal and stress conditions, respectively. It was also observed that polydatin significantly reduced the level of GFP expression of gst-4, an inducible oxidative stress protein in transgenic worms, indicating its protective role against oxidative stress under different conditions. Moreover, the mobility ability of worms became slower as following aging process. Oxidative stress could accelerate the aging process of worms and the decline of mobility activity afterwards. Also, polydatin could save the worm's mobility under stress by slowing down the reduced stroke frequency in worms. Based on the obtained results, polydatin exhibited a significant longevity extending effect and anti-oxidative ability in worms, in which the lifespan-extension ability is closely related to its anti-oxidative activity in vivo.
The insulin/IGF-1 signaling pathway is part of an endocrine system that regulates longevity, metabolism, stress resistance and development in C. elegans.31 This pathway is homologous to the insulin/IGF-1 signaling pathway in human,36,37 in which daf-2 encodes the insulin/IGF-I receptor and decreased DAF-2 signaling extends C. elegans’ lifespan significantly. In this pathway, DAF-16 is a forkhead transcription factor, which can translocalize into the nucleus and modulates transcription when daf-2 signaling is down-regulated. In this work, polydatin significantly altered the subcellular distribution of DAF-16 from the cytoplasm to the nucleus in DAF-16::GFP transgenic TJ356 worms, and exhibited a dose dependant effect after treatment with different concentrations. Worms exposed to a higher concentration of polydatin showed more DAF-16 translocalization. These findings imply that the lifespan extending role of polydatin is partly exerted by regulating the DAF-16 forkhead transcription factor in the insulin/IGF-1 signaling pathway.
Among the multiple daf-16 transcriptional targets that mediate the diverse functions of daf-2 signaling, SOD-3 is a downstream effector of DAF-16, which can serve as stress sensitive reporter to predict longevity in C. elegans.32 In another set of experiments, polydatin exhibited a pronounced increase in the expression of SOD-3 in transgenic CF1553 worms, which also supports the previous findings that polydatin can improve the survival rate of worms under normal and oxidative stress conditions. The protective activity against oxidative stress is closely associated with the increased expression of SOD-3 by polydatin in vivo. The insulin/IGF-1 pathway also regulates the expression of free-radical detoxifying enzymes, consistent with free-radical theory of aging and the involvement of mitochondria and reactive oxygen species (ROS) in aging. These phenotypes are suppressed by daf-16 loss-of-function mutations, suggesting that daf-16 is negatively regulated by daf-2 pathway and is the major downstream mediator of genes that extend lifespan. Quantitative real-time PCR results revealed the genetic evidence for the regulation effect of the insulin/IGF-1 signaling pathway by polydatin. As shown in Fig. 7, polydatin could significantly up-regulate the expression of the aging-associated genes daf-16 and down-regulate daf-2 expression, illustrating the specific role of polydatin to extend the longevity of C. elegans and its regulative mechanism involved in the insulin/IGF-1 signaling pathway. Further work is being undertaken using RNAi technology to target daf-16 and sod-3 in this pathway to contribute to our complete understanding of polydatin in the aging process.
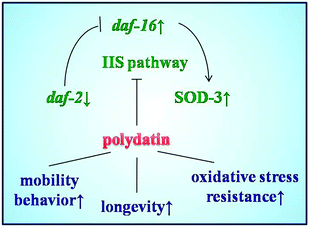 |
| Fig. 7 The proposed signaling pathway involving in the anti-aging role of polydatin in C. elegans. | |
In the work, a microscale microfluidic device was used to successfully characterize the multiple behaviors of C. elegans in response to polydatin at single animal resolution. The device is composed of a series of functional units to facilitate individual worm's loading, long-term culture, immobilization and imaging. According to the unique design of the microdevice, the worms could be maintained with sufficient nutrients exchange for up to 35 days, which is the longest worm cultivation reported on chip to date. The integrated array chambers and valves enable easy manipulation of worms in a controllable manner, and real-time tracking and imaging of worms in parallel, thus facilitating multi-parameter evaluation of worms under different conditions and anti-aging drug screening at single animal resolution. An integrated microfluidic approach was used to evaluate the anti-aging role of polydatin in individual C. elegans for the first time. The microfluidic platform used enabled the lifelong and real-time tracking of the multiple responses of individual C. elegans exposure to external stimuli in parallel, thus facilitating rapid multi-parameter analysis of worms, providing a useful tool for whole animal assay and anti-aging drug evaluation at single animal resolution.
Conclusion
This is a continuing research for the exploration of polydatin that extends lifespan in C. elegans. Polydatin, a derivative of resveratrol, was found to significantly increase stress resistance as well as extend the lifespan of worms under normal and oxidative stress conditions. The longevity extending effect of polydatin is mainly attributed to its anti-oxidative activity, which is mediated by the associated genes and proteins via up-regulating expression of daf-16 and SOD-3 in the insulin/IGF-1 signaling pathway. This also demonstrates the utility of microscale bioengineering technology for applications in the study of aging. The highly conserved functional nature of insulin/IGF-1 between worms and humans demonstrates that findings from C. elegans can contribute greatly to our understanding of aging in higher organisms. These new findings highlight the important role of polydatin in drug development and invention therapy related to aging as well as aging-related diseases. They also address the potential of polydatin to extend the human average life expectancy and the application of microscale technology for applications in aging studies.
Acknowledgements
This research was supported by the National Nature Science Foundation of China (No. 81273483, 91227123, 11161160552), the National Science & Technology Pillar Program in the Twelfth Five-year Plan Period of China (No. 2012BAK02B00, 2012BAK02B03), and the Special Fund for Agro-scientific Research in the Public Interest (No. 201303045).
References
- G. R. Boss and J. E. Seegmiller, Geriatr. Med., 1981, 135, 434–440 CAS.
- C. Huang, Proc. Natl. Acad. Sci. U. S. A., 2004, 101, 8084–8089 CrossRef CAS PubMed.
- S. Morrison and K. M. Newell, J. Aging Res., 2012, 2012, 1–14 Search PubMed.
- M. Hertweck, T. Hoppe and R. Baumeister, Exp. Gerontol., 2003, 38, 345–346 CrossRef.
- A. Olsen, Ann. N. Y. Acad. Sci., 2006, 1067, 120–128 CrossRef CAS PubMed.
- A. Antebi, PLoS Genet., 2007, 3, e129 CrossRef PubMed.
- C. D. Link, Exp. Gerontol., 2006, 41, 1007–1013 CrossRef CAS PubMed.
- J. J. Collins, K. Evason and K. Kornfeld, Exp. Gerontol., 2006, 41, 1032–1039 CrossRef CAS PubMed.
- T. M. Bass, D. Weinkove, K. Houthoofd, D. Gems and L. Partridge, Mech. Ageing Dev., 2007, 128, 546–552 CrossRef CAS PubMed.
- J. A. Baur, Mech. Ageing Dev., 2010, 131, 261–269 CrossRef CAS PubMed.
- M. Viswanathan, S. K. Kim, A. Berdichevsky and L. Guarente, Dev. Cell, 2005, 9, 605–615 CrossRef CAS PubMed.
- L. M. Hung, J. K. Chen, S. S. Huang, R. S. Lee and M. J. Su, Cardiovasc. Res., 2000, 47, 549–555 CrossRef CAS.
- P. Signorelli and R. Ghidoni, J. Nutr. Biochem., 2005, 16, 449–466 CrossRef CAS PubMed.
- S. Bereswill, M. Muñoz, A. Fischer, R. Plickert, L. M. Haag, B. Otto, A. A. Kühl, C. Loddenkemper, U. B. Göbel and M. M. Heimesaat, PLoS One, 2010, 5, e15099 CAS.
- R. H. Cichewicz and S. A. Kouzi, J. Nat. Prod., 1998, 61, 1313–1314 CrossRef CAS PubMed.
- S. Fabris, F. Momo, G. Ravagnan and R. Stevanato, Biophys. Chem., 2008, 135, 76–83 CrossRef CAS PubMed.
- A. I. R. Pérez, M. I. Gómez, R. M. Lamuela-Raventós and M. Carmen, J. Agric. Food Chem., 1999, 47, 1533–1536 CrossRef PubMed.
- Q. Miao, S. Wang, S. Miao, J. Wang, Y. Xie and Q. Yang, Phytomedicine, 2011, 19, 8–12 CrossRef CAS PubMed.
- H. Ji, X. Zhang, Y. Du, H. Liu, S. Li and L. Li, Brain Res. Bull., 2012, 87, 50–59 CrossRef CAS PubMed.
- G. Lanzilli, A. Cottarelli, G. Nicotera, S. Guida, G. Ravagnan and M. P. Fuggetta, Inflammation, 2011, 35, 240–248 CrossRef PubMed.
- S. Shu, Z. Y. Ling, Y. Mao, B. Lin, L. J. Wang, T. B. Zhang, J. Chen and T. Y. Liu, BMC Cell Biol., 2011, 12, 31 CrossRef CAS PubMed.
- Z. S. Huang, Z. W. Wang, M. P. Liu, S. Q. Zhong, Q. M. Li and X. L. Rong, World J. Gastroenterol., 1999, 5, 41–44 CAS.
- H. Wen, W. W. Shi and J. H. Qin, Biomed. Microdevices, 2012, 14, 721–728 CrossRef PubMed.
- S. Brenner, Genetics, 1974, 77, 71–94 CAS.
- J. C. McDonald, D. C. Duffy, J. R. Anderson, D. T. Chiu, H. K. Wu, O. J. A. Schueller and G. M. Whitesides, Electrophoresis, 2000, 21, 27–40 CrossRef CAS.
- S. W. Emmons, M. R. Klass and D. Hirsh, Proc. Natl. Acad. Sci. U. S. A., 1979, 76, 1333–1337 CrossRef CAS.
- J. E. Sulston and S. Brenner, Genetics, 1974, 77, 95–104 CAS.
- A. P. Wickens, Respir. Physiol., 2001, 128, 379–391 CrossRef CAS.
- R. S. Balaban, S. Nemoto and T. Finkel, Cell, 2005, 120, 483–495 CrossRef CAS PubMed.
- C. Kumsta, M. Thamsen and U. Jakob, Antioxid. Redox Signaling, 2011, 14, 1023–1037 CrossRef CAS PubMed.
- O. S. Wook, A. Mukhopadhyay, B. L. Dixit, T. Raha, M. R. Green and H. A. Tissenbaum, Nat. Genet., 2005, 38, 251–257 CrossRef PubMed.
- S. S. Lee, Science, 2003, 300, 644–647 CrossRef CAS PubMed.
- A. V. Samuelson, C. E. Carr and G. Ruvkun, Genes Dev., 2007, 21, 2976–2994 CrossRef CAS PubMed.
- M. Davidovic, G. Sevo, P. Svorcan, D. P. Milosevic, N. Despotovic and P. Erceg, Aging Dis., 2010, 1, 139–146 Search PubMed.
- S. Hekimi, J. Lapointe and Y. Wen, Trends Cell Biol., 2011, 21, 569–576 CrossRef CAS PubMed.
- M. Tatar, T. M. Yamawaki, J. R. Berman, M. Suchanek-Kavipurapu, M. McCormick, M. M. Gaglia, S. J. Lee and C. Kenyon, PLoS Biol., 2010, 8, e1000468 Search PubMed.
- W. M. Shaw, S. J. Luo, J. Landis, J. Ashraf and C. T. Murphy, Curr. Biol., 2007, 17, 1635–1645 CrossRef CAS PubMed.
|
This journal is © The Royal Society of Chemistry 2014 |
Click here to see how this site uses Cookies. View our privacy policy here.