DOI:
10.1039/C3GC42189A
(Paper)
Green Chem., 2014,
16, 1462-1469
Enhanced laccase stability through mediator partitioning into hydrophobic ionic liquids
Received
23rd October 2013
, Accepted 5th December 2013
First published on 5th December 2013
Abstract
Laccase-mediator systems have numerous potential uses for green oxidations, but their practical use may be limited because the reactive, oxidised mediators deactivate the enzyme. TEMPO, 4-hydroxybenzyl alcohol, phenothiazine and 2-hydroxybiphenyl caused almost complete deactivation of laccase from Trametes versicolor within 24–140 h. By contrast, 18% activity was retained after 188 h in controls without mediator, and 15% in the presence of ABTS. A biphasic reaction system was developed to protect the laccase, by partitioning the mediator into water-immiscible ionic liquids. In the presence of [C6mim][AOT], laccase retained 54, 35, 35 and 41% activity after 188 h in the presence of 4-hydroxybenzyl alcohol, phenothiazine and 2-hydroxybiphenyl and ABTS, respectively, whilst 30% activity was retained in the presence of [N1
8
8
8][Sac] and TEMPO. The protection against deactivation by the mediators correlated strongly with the distribution coefficients of the mediators between ionic liquids and water.
Introduction
Laccases (EC 1.10.3.2) catalyse cofactor-independent, one-electron oxidation of a wide range of substrates using dioxygen as the electron acceptor. Because of this, laccases have attracted attention as versatile and practical biocatalysts for use in oxidative biotransformations, bioremediation, textile treatments, pulp bleaching, and biorefining.1–3 Their versatility is due to their ability to oxidise a range of primary substrates to produce reactive free radical intermediates, which then act as mediators to oxidise the secondary, target substrates.1,4,5 The oxidised mediator is freely diffusible, and, therefore, is able to react even with substrates that are insufficiently soluble, or too large, for direct oxidation in the laccase active site (e.g. lignin).
Unfortunately, this versatility comes at a price. Laccases are deactivated rapidly by oxidised mediators, and this can limit their practical application.6–15 Mediators are known to react with surface tryptophan residues of xylanases,16 and similar reactions may be responsible for laccase deactivation.6,14,17 Deactivation may also be associated with oxidation of glycosyl residues,15 although the extent of glycosylation does not affect the stability of different laccases.17 Laccases can also be deactivated by heat,9,11 extremes of pH,11 conventional solvents18–23 and sonication.24 The active-site copper is relatively labile, and can be removed, for example, by complexation with humic acid, although copper ions can restore activity25 and protect against deactivation.9
Laccases can be protected against mediator deactivation by adsorption onto solid substrates such as pulp.7,12 This has inspired attempts to improve laccase stability by immobilisation using cross-linked enzyme crystals,26 cross-linked enzyme aggregates (CLEAs),27 alginate-chitosan encapsulation,28 and adsorption onto various supports, either with29 or without21,30,31 additional polyelectrolyte coating. Immobilisation tends to improve thermal stability and reusability, but immobilisation in microgels makes laccase less stable than the free enzyme.32 Immobilisation also provides protection against inhibitors, solvents (except with CLEAs27), or extremes of pH. Although CLEAs provide improved oxidation of alcohols compared with free enzyme,27 stability improvements with other enzyme support systems are frequently offset by decreased catalytic activity due to mass transfer restrictions with less soluble substrates. Immobilisation can also cause problems with insoluble substrates coating the supported enzyme particles.31
As an alternative, attempts have been made to stabilise laccase using additives. Although albumens activated laccase from Polyporus pinsitus, the laccase from Myceliophthora thermophila was inhibited,33 so this is a relatively expensive and enzyme-specific approach. Addition of polyethylene glycol (PEG)34 or PEGylation23 can improve thermal stability and reduce solvent deactivation. Although activity decreases significantly after PEGylation, this is offset by the increased biocatalyst lifetime, which can reduce the enzyme loading and, hence, costs.35 Alternatively, directed evolution provides a promising approach to improve thermal stability and resistance to denaturing solvents,36,37 although it is not known if this can solve problems with mediator deactivation.
Surfactant mesophases can also be used to segregate the enzyme from destabilising agents. Thus, surfactant-laccase complexes in water in oil emulsions are active in anhydrous toluene, which would normally deactivate freeze-dried laccase.18 Similarly, productivity is improved when laccase is incorporated into micelles in a biphasic silicone oil/water system, although this did not improve stability.14 Even more promising was the observation that onion-type, multi-lamellar liposomes stimulated activity and protected against deactivation by ABTS.38 Nevertheless, these systems are relatively complex to prepare, and the presence of surfactants may complicate downstream separations.
In this paper, a new, simple solution to the problem of laccase deactivation by reactive mediators is presented. A biphasic reaction system was designed, in which the laccase is dissolved in the aqueous phase and is then contacted with a water-immiscible solvent. The system is designed so that the mediator partitions preferentially into the non-aqueous phase. This minimises contact between the enzyme and the reactive free radical, reducing the opportunity for the mediator to react with the enzyme and deactivate it. Therefore, the stability of the laccase was improved dramatically.
Success depended on being able to design enzyme-friendly, water-immiscible solvents that dissolve the mediator preferentially. We used ionic liquids, since few conventional solvents support laccase activity,18,19,21,23,39 whereas a range of ionic liquids support or even stimulate laccase activity.40–45 In particular, water-immiscible ionic liquids are frequently compatible with laccase activity.45 The massive structural diversity of ionic liquids provides the ability to fine tune their physical properties to obtain optimal dissolution of the mediators. Furthermore, ionic liquids are sufficiently stable to support chemical reactions involving radical species without oxidising the medium.46 Using this ionic liquid-water biphasic system, we showed that laccase stability is improved significantly during prolonged use.
Results and discussion
Distribution coefficients of mediators between ionic liquids and water
Water-immiscible ionic liquids were tested for their ability to partition reactive mediators away from the aqueous, enzyme-containing phase. We tested ionic liquids containing [N1
8
8
8]+ and [C6mim]+ cations and [AOT]−, [Sac]− and [NTf2]− anions (Table 2). The mediators varied from commonly used, water soluble, laccase mediators (TEMPO, ABTS) to less soluble types (phenothiazine,47,48 2-hydroxybiphenyl;49–51Table 1). 4-Hydroxybenzyl alcohol was also tested, since it is a naturally occurring laccase mediator, and may have decreased environmental impact.52,53
Table 1 Structures of mediators
Name |
Structure |
TEMPO; (2,2,6,6-tetramethyl-1-piperidinyl)oxidanyl |
|
ABTS; 2,2′-azino-bis(3-ethylbenzo-thiazoline-6-sulfonic acid) diammonium salt |
|
10H-Phenothiazine |
|
4-Hydroxybenzyl alcohol |
|
2-Hydroxy-biphenyl |
|
Table 2 Ionic liquids used in this study
Name/Abbreviation |
Structure |
Cations
|
Methyltrioctyl-ammonium/[N1 8 8 8]+ |
|
1-Methyl-3-hexyl-imidazolium/[C6mim]+ |
|
Anions
|
1,4-bis(2-ethylhexyl)sulfosuccinate (docusate)/[AOT]− |
|
Saccharinate/[Sac]− |
|
Bis{(trifluoro-methylsulfonyl}amide (bistriflamide)/[NTf2]− |
|
Oxidised mediators were prepared by laccase-catalysed oxidation for 8 h, using laccase from Trametes versicolor. The oxidised mediator solutions were equilibrated with ionic liquids for 24 h, and the partitioning of the oxidised mediators was measured between the ionic liquids and the aqueous reaction medium by spectrophotometric analysis (Fig. 1). The partitioning was striking, since accumulation of the coloured mediator products could be observed visually in the ionic liquid layers.
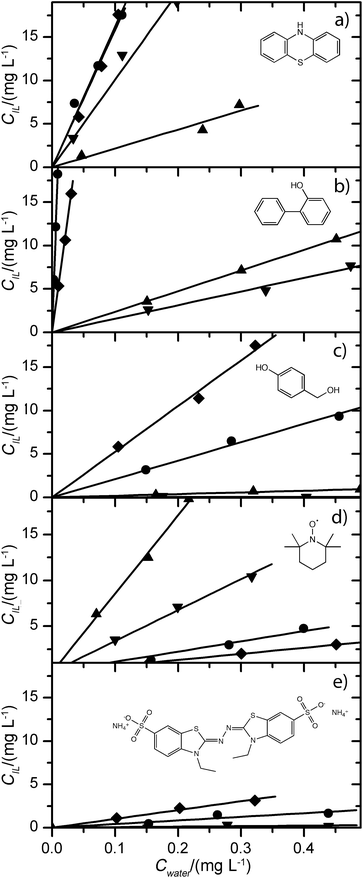 |
| Fig. 1 Mediator partitioning between laccase reaction medium and ionic liquids. The partitioning of the oxidised mediators was measured between reaction medium containing laccase, buffer and the mediators shown and the ionic liquids, [N1 8 8 8][AOT], ●; [N1 8 8 8][Sac], ▲; [N1 8 8 8][NTf2], ▼; [C6mim][AOT] ◆. The mediators were: (a) phenothiazine, (b) 2-hydroxybiphenyl (c) 4-hydroxybenzylalcohol (d) TEMPO, (e) ABTS. All measurements were made in triplicate and the mean values are shown; standard deviations were less than 10% of the mean for all data shown. | |
The isotherms followed a linear trend over the concentration range tested, and the distribution coefficients were calculated from the slope of the isotherms (Table 3). [N1
8
8
8][AOT] and [C6mim][AOT] were the best extraction solvents for all of the mediators except for TEMPO, which was extracted more efficiently by [N1
8
8
8][Sac] and [N1
8
8
8][NTf2]. The most efficient extraction was obtained using [N1
8
8
8][AOT] to extract 2-hydroxybiphenyl (DIL/W 2056), although a high DIL/W (526) was also obtained in [C6mim][AOT]. Phenothiazine was extracted efficiently by [N1
8
8
8][AOT], [C6mim][AOT], and [N1
8
8
8][NTf2] (DIL/W > 100); only [N1
8
8
8][Sac] exhibited a lower DIL/W (22). 4-Hydroxybenzyl alcohol and ABTS were extracted less efficiently into [N1
8
8
8][AOT] and [C6mim][AOT] (DIL/W 4–11); [N1
8
8
8][Sac] and [N1
8
8
8][NTf2] did not extract these mediators. As expected, there was a very poor correlation between the log(P) values of the mediators and the distribution coefficients, since the oxidised mediators present in these experiments are radical cations.4,5,54 In addition, there may be structure-specific, non-covalent interactions between the oxidized mediators and the ionic liquids, which would further complicate prediction of extraction efficiencies.
Table 3 Distribution coefficient (DIL/Wa) of mediators between ionic liquids and buffer
Ionic liquid |
ABTS |
TEMPO |
Phenothiazine |
4-HBA |
2-Hydroxybiphenyl |
D
IL/W values were calculated from the slope of the isotherms in Fig. 1 (± 95% confidence interval of the regression estimate). The octanol–water partitioning coefficients, log(P) of the mediators are also shown.
Below detection limit.
No experimental data available in the literature, estimated through Broto's fragmentation method.58
No experimental data available in the literature, estimated through Crippen's fragmentation method, as TEMPO is a radical.59
|
[N1 8 8 8][AOT] |
4.2 ± 0.6 |
11.1 ± 0.8 |
162 ± 5 |
21 ± 1 |
2056 ± 78 |
[C6mim][AOT] |
10 ± 0.4 |
6.6 ± 0.1 |
158 ± 8 |
53 ± 2 |
526 ± 6 |
[N1 8 8 8][NTf2] |
0.57 ± 0.24 |
34 ± 1 |
101 ± 6 |
0.14 ± 0.09 |
16 ± 1 |
[N1 8 8 8][Sac] |
n/ab |
86 ± 2 |
22 ± 2 |
1.91 ± 0.1 |
23 ± 1 |
log(P) |
2.840c |
2.10d |
3.910c |
0.25055 |
1.71056 |
Effect of ionic liquids on laccase activity
The effect of the ionic liquids on laccase activity was investigated by incubating the enzyme from T. versicolor in the presence of ionic liquids (but without mediators). The mixture was sampled at time intervals and the residual catechol oxidation activity was measured in a spectrophotometric assay (Fig. 2). As expected, laccase lost activity (82% decrease) over 188 h in the absence of the ionic liquids. None of the ionic liquids affected the initial activity. Although the mean activity decreased by only 65–76% in the presence of the ionic liquids, the difference from the control is only statistically significant for two data-points ([N1
8
8
8][NTf2] and [C6mim][AOT] at 188 h). Therefore, these water-immiscible ionic liquids were compatible with laccase activity, although they provided little or no protection against loss of laccase activity in the absence of mediators.
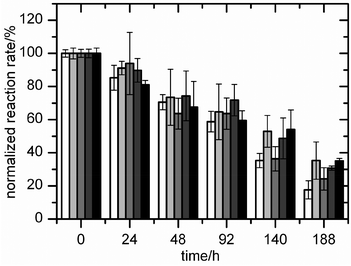 |
| Fig. 2 Effect of water-immiscible ionic liquids on aqueous laccase activity. Laccase activity was measured in the aqueous phase at intervals after incubation with no ionic liquid (white bar), and in the presence of [N1 8 8 8][AOT] ( ), [N1 8 8 8][Sac] ( ), [N1 8 8 8][NTf2] ( ), or [C6mim][AOT] (■). The mean reaction rates (measured in triplicate) are expressed as a percentage of the mean rate of triplicate control samples with no ionic liquid, taken immediately after mixing laccase with the buffer solution (time = 0, V0 = 0.11 μM s−1). Error bars represent the standard deviation. | |
Effect of mediators on laccase activity
The effect of mediators was assessed by incubating laccase in the presence and absence of mediators, sampling, and measuring the residual activity. As noted above, the enzyme lost 82% of the activity over 188 h in the absence of mediators (Fig. 3). ABTS caused a slight loss of activity (12%) immediately after addition, but the rate of activity loss was similar to the control thereafter. TEMPO, phenothiazine, and 4-hydroxybenzyl alcohol did not affect initial activity but greatly accelerated the loss of activity compared with the control and ABTS, decreasing the activity by 29, 71 and 91% after only 24 h, respectively. There was very little or no residual activity after 140 h. 2-Hydroxybiphenyl was extremely inhibitory; laccase activity had already fallen to 29% of the control activity in the sample taken at t = 0, perhaps because of the slight delay between adding the mediator, sampling the mixture, and starting the assay for residual activity. Laccase was completely inactive after incubation for only 24 h in the presence of 2-hydroxybiphenyl.
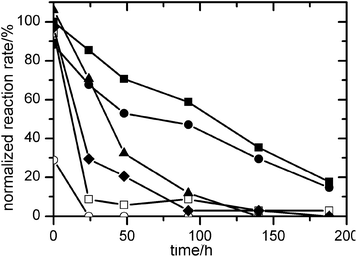 |
| Fig. 3 Effect of mediators on laccase activity. Laccase activity was measured at intervals after incubation in the presence of no mediator (■), ABTS (●), TEMPO (▲), phenothiazine (◆), 4-hydroxybenzyl alcohol (□) or 2-hydroxybiphenyl (○). Reaction rates are expressed as a percentage of the rate in the control sample taken immediately after mixing laccase with buffer solution (no mediator, time = 0, V0 = 0.112 μM s−1). All experiments were conducted in triplicate, and the standard deviation was less than 10% of the mean. | |
Using ionic liquids to protect against mediator-induced loss of laccase activity
Laccase was incubated with both the mediators and the ionic liquids, and the aqueous phase was sampled to measure the residual activity (Fig. 4). The volume ratio of ionic liquid–water was 1
:
10. The ionic liquids improved the stability of laccase dramatically in the presence of phenothiazine (Fig. 4b). Without the ionic liquids, the enzyme lost 93% activity after only 92 h, and there was no residual activity after 188 h. In the presence of [N1
8
8
8][AOT], [N1
8
8
8][NTf2], or [C6mim][AOT], the loss of activity was much slower, and 35, 31, or 35% of the initial activity was still retained after 188 h, respectively. In fact, this retention of activity was better than the control without mediators or ionic liquids (17%). All of these ionic liquids extracted phenothiazine extremely efficiently, with DIL/W values between 101 and 162. By contrast, [N1
8
8
8][Sac] provided only modest protection against deactivation (6% activity retained after 188 h), reflecting its poor extraction efficiency for phenothiazine (DIL/W = 22). Therefore, protection of the laccase was strongly correlated with the ability of the ionic liquids to extract phenothiazine (Fig. 5).
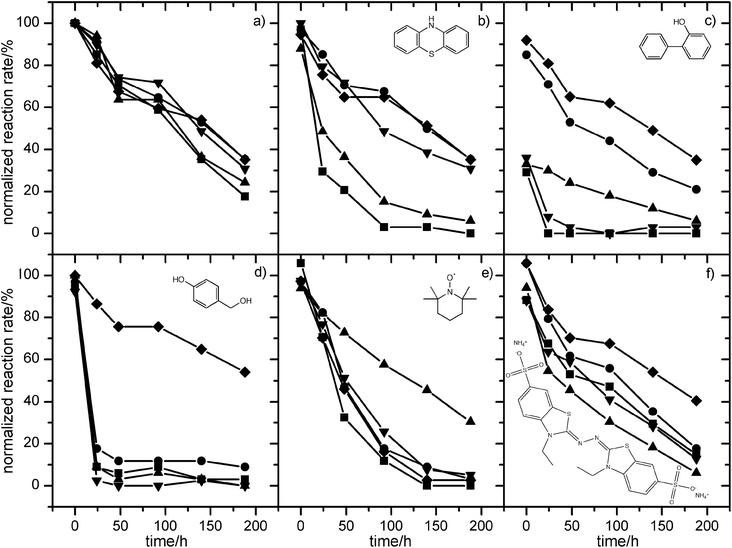 |
| Fig. 4 Relative activity of laccase in the presence of mediators and ionic liquids. Laccase activity was measured at intervals after incubation in the presence of (a) no mediator (data from Fig. 2), (b) phenothiazine, (c) 2-hydroxybiphenyl (d) 4-hydroxybenzylalcohol (e) TEMPO, (f) ABTS in biphasic systems containing [N1 8 8 8][AOT] (●), [N1 8 8 8][Sac] (▲), [N1 8 8 8][NTf2] (▼), or [C6mim][AOT] (◆) and compared with controls without ionic liquids (■). Reaction rates are expressed as a percentage of the rate in a control sample taken immediately after mixing laccase with buffer solution (no mediator and no ionic liquid, time = 0, V0 = 0.113 μM s−1). All experiments were conducted in triplicate and the standard deviation was less than 10% of the mean. | |
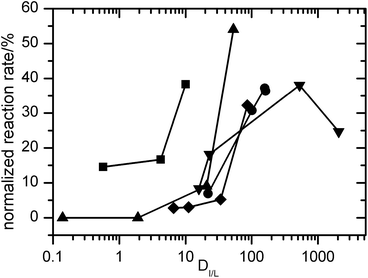 |
| Fig. 5 Effect of distribution coefficient on reaction rate. Using the data from Fig. 4 and Table 2, the residual reaction rates (after incubation for 188 h) in the presence of mediators (ABTS ■; PTZ ●; 4HBA ▲; 2HBP ▼; and TEMPO ◆) and ionic liquids were calculated as a percentage of the initial reaction rate in controls with no mediator (V0 = 0.114 μM s−1). These data were plotted against the distribution coefficient of the mediator between each ionic liquid and water. | |
Similar results were obtained with 2-hydroxybiphenyl (Fig. 4c). This mediator caused immediate loss of 71% activity in the absence of ionic liquids, and complete loss of activity after 24 h. When [N1
8
8
8][AOT] or [C6mim][AOT] were present, the immediate loss of activity was only 15 or 8%, respectively, and the enzyme still retained 21 and 35% activity after 188 h, respectively. [N1
8
8
8][Sac] provided less protection against deactivation, whilst [N1
8
8
8][NTf2] did not protect against deactivation at all. As with phenothiazine, there was an extremely strong correlation between extraction efficiency for 2-hydroxybiphenyl and the protection afforded against laccase deactivation by the ionic liquids (Fig. 5).
Although 4-hydroxybenzyl alcohol did not deactivate laccase immediately, activity fell rapidly to only 8% of the control activity after 24 h (Fig. 4d). There was little further loss of activity. [C6mim][AOT] (DIL/W = 53) provided excellent protection against this deactivation, and the enzyme still retained 54% of the activity after 188 h. By contrast, [N1
8
8
8][AOT] (DIL/W = 21) did not prevent the initial fall in activity after 24 h, although the enzyme retained 9% activity after 188 h, compared with 3% in the control. [N1
8
8
8][Sac] provided almost no protection, whilst [N1
8
8
8][NTf2] seemed to accelerate the deactivation, reflecting the inability of this ionic liquid to extract the mediator from water (Table 3). Again, the correlation between extraction efficiency and protection against mediator deactivation was very strong (Fig. 5).
When laccase was incubated with TEMPO, only [N1
8
8
8][Sac] (DIL/W = 86) provided protection against deactivation, with 30% activity remaining after 188 h, compared with complete loss of activity in the control (Fig. 4e). Other ionic liquids provided only slight protection against deactivation, perhaps reflecting their poorer affinity for TEMPO (DIL/W = 6.6–34).
When laccase was incubated with ABTS, 12% of the activity was lost immediately (Fig. 4f). This loss of activity was prevented by [N1
8
8
8][AOT] (DIL/W = 4.2) or [C6mim][AOT] (DIL/W = 10). [C6mim][AOT] also improved longer term stability, since the enzyme retained 40% activity after 188 h, compared with only 15% in the control. By contrast, [N1
8
8
8][AOT] did not improve long term stability, possibly reflecting the poorer extraction efficiency for ABTS. [N1
8
8
8][NTf2] had no effect on activity loss, as expected, since it does not extract ABTS from water (DIL/W = 0.57). [N1
8
8
8][Sac] accelerated deactivation very slightly, consistent with even worse extraction of ABTS. Thus, in all cases tested, there was a strong correlation between partitioning of the mediator into the ionic liquid phase and the ability of the ionic liquid to protect laccase from deactivation by the mediators.
Conclusions
The problem of laccase inactivation by mediators can be solved, by using a biphasic ionic liquid/water reaction system. By choosing a suitable ionic liquid, the oxidised, reactive mediator is partitioned preferentially into the ionic liquid phase, away from the enzyme in the aqueous phase. This minimises contact between the enzyme and the deactivating species, and extends the lifetime of the active laccase. This finding has important implications for implementation of laccase-catalysed oxidations, since extended enzyme lifetime has a major impact on process economics.
Experimental
Materials
Synthesis and characterisation of the ionic liquids, 1-hexyl-3-methylimidazolium 1,4-bis(2-ethylhexyl)sulfosuccinate ([C6mim][AOT]), methyl(trioctyl)ammonium bis(trifluoromethylsulfonyl)amide ([N1
8
8
8][NTf2]) and methyl(trioctyl)ammonium saccharinate ([N1
8
8
8][Sac]) was described previously.57 The same method was used to synthesize methyl(trioctyl)ammonium 1,4-bis(2-ethylhexyl)sulfosuccinate ([N1 8 8 8][AOT]) as for other [AOT]− ionic liquids.55 Laccase from Trametes versicolor, 2,2,6,6-tetramethyl-1-piperidinyloxyl (TEMPO), 10H-phenothiazine, 2,2′-azino-bis(3-ethylbenzothiazoline-6-sulfonic acid) diammonium salt (ABTS), 4-hydroxybenzyl alcohol and 2-hydroxybiphenyl, and other chemicals, were obtained from Sigma Aldrich (UK).
Measurement of distribution coefficients
Oxidised mediator solutions (0.4 mM; described in the text) in 20 mM citric acid buffer (pH 4.5) were prepared by oxidation with Trametes versicolor laccase (0.25 mg L−1) in sealed scintillation vials. The reactions were incubated for 8 h to ensure complete oxidation, which had been verified by spectrophotometric analysis of earlier time course experiments. For each combination of mediator and ionic liquid, three experimental vials containing oxidised mediator solution in buffer (0.5 mL; final concentration between 0.04 and 0.1 mM) and ionic liquid (40 μL) were allowed to equilibrate for 24 h (rotary shaker, 200 rpm, 25 °C). Control vials with the same mediator concentrations, but without ionic liquids, were incubated in the same way. The concentrations of the oxidised TEMPO, 10H-phenothiazine, ABTS, 4-hydroxybenzyl alcohol and 2-hydroxybiphenyl in the aqueous phase were measured spectrophotometrically at 228, 270, 420, 273 and 255 nm, respectively, based on their measured absorption maxima. Absorption data at the respective wavelength were correlated to previously prepared calibration curves (triplicates of 5 points equally spaced between 0.04 and 0.1 mM mediator solutions). The standards were prepared by enzymatic oxidation as described above. Incubation with the ionic liquids had no effect on the extinction coefficient of the mediator in the aqueous phase. The fraction that had partitioned into the ionic liquid phase was calculated using a mass balance based on the mediator concentration in the control vials, measured spectrophotometrically. Measurements were made at three different concentrations of each mediator in each ionic liquid, and the slope of a regression analysis of these three equilibrium points was used to determine the distribution coefficient, using eqn (1),where CIL is the calculated mediator concentration in the ionic liquid and CW is the measured mediator concentration in the aqueous phase, in mg L−1.
Measurement of laccase deactivation
Laccase deactivation in the presence and absence of mediators was measured in the presence and absence of ionic liquids. Capped glass vials (5 mL capacity) contained mixtures (1 mL) of 20 mM citric acid buffer, pH 4.5, laccase from Trametes versicolor (80 mg L−1), and mediator (5 mM, when required). The enzyme mixtures were incubated in the presence and absence of ionic liquids (100 μL) at 25 °C for 188 h (200 rpm rotary shaker). The aqueous phase was sampled for determination of residual laccase activity in quartz 96-well plates. Each well contained 20 mM citric acid buffer (100 μL, pH 4.5) and samples of the enzyme-containing aqueous phase (5 μL). Initial reaction rates were measured by adding catechol solution (5 μL, 20 mM), and measuring the formation of benzoquinone at 380 nm using a FLUOstar Optima Microplate Reader (BMG Labtech Ltd., UK). Quartz plates were used because some of the ionic liquids partially dissolve plastic plates. Rates were compared with the rate of catechol oxidation using freshly prepared aqueous enzyme solutions.
Acknowledgements
This work was supported by the UK Technology Strategy Board, and by a BBSRC Research Development Fellowship awarded to GS.
Notes and references
- R. A. Sheldon and I. Arends, Adv. Synth. Catal., 2004, 346, 1051–1071 CrossRef CAS.
- S. Riva, Trends Biotechnol., 2006, 24, 219–226 CrossRef CAS PubMed.
- A. Kunamneni, I. Ghazi, S. Camarero, A. Ballesteros, F. J. Plou and M. Alcalde, Process Biochem., 2008, 43, 169–178 CrossRef CAS PubMed.
- R. Bourbonnais, M. G. Paice, B. Freiermuth, E. Bodie and S. Borneman, Appl. Environ. Microbiol., 1997, 63, 4627–4632 CAS.
- O. V. Morozova, G. P. Shumakovich, S. V. Shleev and Y. I. Yaropolov, Appl. Biochem. Microbiol., 2007, 43, 523–535 CrossRef CAS.
- G. M. B. Soares, M. T. P. de Amorim and M. Costa-Ferreira, J. Biotechnol., 2001, 89, 123–129 CrossRef CAS.
- C. Sigoillot, S. Camarero, T. Vidal, E. Record, M. Asther, M. Perez-Boada, M. J. Martinez, J.-C. Sigoillot, M. Asther, J. F. Colom and A. T. Martinez, J. Biotechnol., 2005, 115, 333–343 CrossRef CAS PubMed.
- D. Ibarra, J. Romero, M. J. Martinez, A. T. Martinez and S. Camarero, Enzyme Microb. Technol., 2006, 39, 1319–1327 CrossRef CAS PubMed.
- L. Papinutti, P. Dimitriu and F. Forchiassin, Bioresour. Technol., 2007, 99, 419–424 CrossRef PubMed.
- R. Khlifi, S. Sayadi, L. Belbahri, S. Woodward and T. Mechichi, J. Chem. Technol. Biotechnol., 2009, 84, 1828–1833 CrossRef CAS.
- S. Kurniawati and J. A. Nicell, Biotechnol. Prog., 2009, 25, 763–773 CrossRef CAS PubMed.
- A. Fillat, J. F. Colom and T. Vidal, Bioresour. Technol., 2010, 101, 4104–4110 CrossRef CAS PubMed.
- C. Valls, J. F. Colom, C. Baffert, I. Gimbert, M. B. Roncero and J.-C. Sigoillot, Biochem. Eng. J., 2010, 49, 401–407 CrossRef CAS PubMed.
- A. Arca-Ramos, G. Eibes, M. T. Moreira, G. Feijoo and J. M. Lema, Process Biochem., 2012, 47, 1115–1121 CrossRef CAS PubMed.
- I. W. C. E. Arends, Y. Y. Li and R. A. Sheldon, Biocatal. Biotransform., 2006, 24, 443–448 CrossRef CAS.
- R. F. Bendl, J. M. Kandel, K. D. Amodeo, A. M. Ryder and E. M. Woolridge, Enzyme Microb. Technol., 2008, 43, 149–156 CrossRef CAS PubMed.
- K. Li, F. Xu and K.-E. L. Eriksson, Appl. Environ. Microbiol., 1999, 65, 2654–2660 CAS.
- S. Okazaki, M. Goto, H. Wariishi, H. Tanaka and S. Furusaki, Biotechnol. Prog., 2000, 16, 583–588 CrossRef CAS PubMed.
- J. Rodakiewicz-Nowak, S. M. Kasture, B. Dudek and J. Haber, J. Mol. Catal. B: Enzym., 2000, 11, 1–11 CrossRef CAS.
- J. Rodakiewicz-Nowak, N. Pozdnyakova and O. Turkovskaya, Biocatal. Biotransform., 2005, 23, 271–279 CrossRef CAS.
- A. I. Ruiz, A. J. Malave, C. Felby and K. Griebenow, Biotechnol. Lett., 2000, 22, 229–233 CrossRef CAS.
- F. d'Acunzo, A. M. Barreca and C. Galli, J. Mol. Catal. B: Enzym., 2004, 31, 25–30 CrossRef CAS PubMed.
- J. I. Lopez-Cruz, G. Viniegra-Gonzalez and A. Hernandez-Arana, Bioconjugate Chem., 2006, 17, 1093–1098 CrossRef CAS PubMed.
- C. Basto, C. J. Silva, G. Guebitz and A. Cavaco-Paulo, Ultrason. Sonochem., 2007, 14, 355–362 CrossRef CAS PubMed.
- Y. S. Keum and Q. X. Li, Appl. Microbiol. Biotechnol., 2004, 64, 588–592 CrossRef CAS PubMed.
- J. J. Roy and T. E. Abraham, J. Mol. Catal. B: Enzym., 2006, 38, 31–36 CrossRef CAS PubMed.
- I. Matijosyte, I. W. C. E. Arends, V. S. de and R. A. Sheldon, J. Mol. Catal. B: Enzym., 2010, 62, 142–148 CrossRef CAS PubMed.
- L. Lu, M. Zhao and Y. Wang, World J. Microbiol. Biotechnol., 2007, 23, 159–166 CrossRef CAS.
- J. F. Osma, J. L. Toca-Herrera and S. Rodriguez-Couto, Appl. Catal., A, 2010, 373, 147–153 CrossRef CAS PubMed.
- A. D'Annibale, S. R. Stazi, V. Vinciguerra and S. G. Giovannozzi, J. Biotechnol., 2000, 77, 265–273 CrossRef CAS.
- D. Areskogh and G. Henriksson, Process Biochem., 2011, 46, 1071–1075 CrossRef CAS PubMed.
- S. Schachschal, H.-J. Adler, A. Pich, S. Wetzel, A. Matura and P. K.-H. van, Colloid Polym. Sci., 2011, 289, 693–698 CAS.
- J. Kulys, K. Krikstopaitis, A. Ziemys and P. Schneider, J. Mol. Catal. B: Enzym., 2002, 18, 99–108 CrossRef CAS.
- S. C. Silverio, O. Rodriguez, A. P. M. Tavares, J. A. Teixeira and E. A. Macedo, J. Mol. Catal. B: Enzym., 2013, 87, 37–43 CrossRef CAS PubMed.
- K. Modaressi, K. E. Taylor, J. K. Bewtra and N. Biswas, Water Res., 2005, 39, 4309–4316 CrossRef CAS PubMed.
- N. J. Christensen and K. P. Kepp, J. Chem. Inf. Model., 2012, 52, 3028–3042 CrossRef CAS PubMed.
- H. Liu, L. Zhu, M. Bocola, N. Chen, A. C. Spiess and U. Schwaneberg, Green Chem., 2013, 15, 1348–1355 RSC.
- A. Prevoteau and C. Faure, Biochimie, 2012, 94, 59–65 CrossRef CAS PubMed.
- F. d'Acunzo, A. M. Barreca and C. Galli, J. Mol. Catal. B: Enzym., 2004, 31, 25–30 CrossRef CAS PubMed.
- G. Hinckley, V. Mozhaev, C. Budde and Y. L. Khmelnitsky, Biotechnol. Lett., 2002, 24, 2083–2087 CrossRef CAS.
- S. Shipovskov, H. Q. N. Gunaratne, K. R. Seddon and G. Stephens, Green Chem., 2008, 10, 806–810 CAS.
- A. P. M. Tavares, O. Rodriguez and E. A. Macedo, Biotechnol. Bioeng., 2008, 101, 201–207 CrossRef CAS PubMed.
- O. Rodriguez, R. O. Cristovao, A. P. M. Tavares and E. A. Macedo, Appl. Biochem. Biotechnol., 2011, 164, 524–533 CrossRef CAS PubMed.
- A. Dominguez, O. Rodriguez, A. P. M. Tavares, E. A. Macedo, M. A. Longo and M. A. Sanroman, Bioresour. Technol., 2011, 102, 7494–7499 CrossRef CAS PubMed.
- L. Rehmann, E. Ivanova, J. L. Ferguson, H. Q. N. Gunaratne, K. R. Seddon and G. M. Stephens, Green Chem., 2012, 14, 725–733 RSC.
- N. Jain, A. Kumar, S. Chauhan and S. M. S. Chauhan, Tetrahedron, 2005, 61, 1015–1060 CrossRef CAS PubMed.
- J. Kulys, K. Krikstopaitis and A. Ziemys, J. Biol. Inorg. Chem., 2000, 5, 333–340 CrossRef CAS.
- F. Xu, J. J. Kulys, K. Duke, K. Li, K. Krikstopaitis, H.-J. W. Deussen, E. Abbate, V. Galinyte and P. Schneider, Appl. Environ. Microbiol., 2000, 66, 2052–2056 CrossRef CAS.
- U. Jonas, E. Hammer, E. T. K. Haupt and F. Schauer, Arch. Microbiol., 2000, 174, 393–398 CrossRef CAS.
- A. Schultz, U. Jonas, E. Hammer and F. Schauer, Appl. Environ. Microbiol., 2001, 67, 4377–4381 CrossRef CAS.
- S. Camarero, D. Ibarra, M. J. Martinez and A. T. Martinez, Appl. Environ. Microbiol., 2005, 71, 1775–1784 CrossRef CAS PubMed.
- C. Johannes and A. Majcherczyk, Appl. Environ. Microbiol., 2000, 66, 524–528 CrossRef CAS.
- S.-S. Weng, K.-L. Ku and H.-T. Lai, Bioresour. Technol., 2012, 113, 259–264 CrossRef CAS PubMed.
- A. M. Barreca, B. Sjoegren, M. Fabbrini, C. Galli and P. Gentili, Biocatal. Biotransform., 2004, 22, 105–112 CrossRef CAS.
- T. Fujita, J. Iwasa and C. Hansch, J. Am. Chem. Soc., 1964, 86, 5175–5180 CrossRef CAS.
- C. Karr Jr., P. A. Estep and L. L. Hirst Jr., Anal. Chem., 1960, 32, 463–475 CrossRef.
- N. Wood, J. L. Ferguson, H. Q. N. Gunaratne, K. R. Seddon, R. Goodacre and G. M. Stephens, Green Chem., 2011, 13, 1843–1851 RSC.
- P. Broto, G. Moreau and C. Vandycke, Eur. J. Med. Chem., 1984, 19, 71–78 CAS.
- A. K. Ghose and G. M. Crippen, J. Chem. Inf. Comput. Sci., 1987, 27, 21–35 CrossRef CAS.
|
This journal is © The Royal Society of Chemistry 2014 |
Click here to see how this site uses Cookies. View our privacy policy here.