DOI:
10.1039/C4FO00836G
(Paper)
Food Funct., 2015,
6, 264-274
Black rice (Oryza sativa L.) extracts induce osteoblast differentiation and protect against bone loss in ovariectomized rats
Received
18th September 2014
, Accepted 13th November 2014
First published on 14th November 2014
Abstract
Osteoporosis, an age associated skeletal disease, exhibits increased adipogenesis at the expense of osteogenesis from common osteoporotic bone marrow cells. In this study, black rice (Oryza sativa L.) extracts (BRE) were identified as osteogenic inducers. BRE stimulated the alkaline phosphatase (ALP) activity in both C3H10T1/2 and primary bone marrow cells. Similarly, BRE increased mRNA expression of ALP and osterix. Oral administration of BRE in OVX rats prevented decreases in bone density and strength. By contrast, BRE inhibited adipocyte differentiation of mesenchymal C3H10T1/2 cells and prevented increases in body weight and fat mass in high fat diet fed obese mice, further suggesting the dual effects of BRE on anti-adipogenesis and pro-osteogenesis. UPLC analysis identified cyanidin-3-O-glucoside and peonidin-3-O-glucoside as main anti-adipogenic effectors but not for pro-osteogenic induction. In mechanism studies, BRE selectively stimulated Wnt-driven luciferase activities. BRE treatment also induced Wnt-specific target genes such as Axin2, WISP2, and Cyclin D1. Taken together, these data suggest that BRE is a potentially useful ingredient to protect against age related osteoporosis and diet induced obesity.
Introduction
Osteoporosis, characterized by reduced bone mineral density and bone strength, is the most common skeletal disease worldwide.1,2 Because the incidence of osteoporosis progressively increases as the population ages, urgent effective strategies are required to protect against bone loss.3
Age related osteoporosis becomes more evident in elderly women due to estrogen deficiency in menopause.4 Estrogen deficiency uncouples bone resorption and formation, leading to rapid bone loss. Estrogen replacement therapy would be direct, but is questioned by the incidence of certain cancers, weight gain, and cardiovascular diseases.5 Instead, relatively enhanced actions of bone removal by osteoclastic cells compared to bone forming osteoblastic cells in osteoporotic bones provide potential targets to prevent age related bone loss. Bisphosphonate, a leading treatment for osteoporosis, targeted to bone resorption, is associated with unpleasant side effects.6 Therefore, the anabolic approaches for induction of osteoblasts and bone formation are being actively investigated as alternative strategies for preventing osteoporosis and bone fractures.
During the bone remodeling cycle, resorbed bone by osteoclasts can be replaced by osteoblasts.1,7 The dysregulation of this balance in age related osteoporosis is partly attributed to the reduced osteoblastic and enhanced osteoclastic activities in the aged bone marrow stromal cells.8 The reduced differentiation into osteoblasts is accompanied by an increased number of adipocytes from the aged bone marrow stromal cells.9 Therefore, agents that reorient the biased cell fates of the aged bone marrow cells toward the osteoblasts are believed to be promising for preventive and therapeutic strategies in osteoporosis.10
Bone marrow stromal cells can be differentiated into adipocytes or osteoblasts based on appropriate induction signals.9,11–13 Transcriptional factors that induce the adipogenic fate are PPARγ and C/EBP family members, whereas transcriptional activation of Runx2, TAZ, and osterix is osteoinductive.14 In general activated signaling cascades of TGF-β, WNT, FGF, and BMP also dictate the fate of osteoblasts.15 Identification of natural products and their derived small molecules modulating these signaling cascades is needed to induce bone formation with concomitant suppression of adipocyte generation.16–18
To identify agents that are potentially useful for bone formation, edible herbal products were investigated for their effects on anti-adipogenic and pro-osteogenic activities.18,19 In this study, black rice (Oryza sativa L.) was identified as an inducer of osteoblast differentiation and a suppressor of adipocyte differentiation. Similarly, bone protective effects in ovariectomized rats and suppressive actions on lipid accumulation in obese mice were also investigated. These studies further suggest that black rice can be useful for preventing incidences of bone fracture.
Experimental
Preparation of black rice extracts
The grains of the Korean colored rice variety, ‘Sintoheugmi’, were collected at the experimental field of the Department of Functional Crop, National Institute of Crop Science, Rural Development Administration (RDA), Miryang, Korea. The powdered black rice (Oryza sativa L.) was extracted with 20 volumes of 70% ethanol (w/v) in a shaking incubator at room temperature at 120 rpm for 24 hours. The extracts were filtered through filter paper (Whatman No. 1), concentrated and freeze-dried. The dried extracts (2.97 gram yield) were resolved in corn oil or DMSO at a concentration of 100 mg mL−1, and stored at −20 °C.
Osteoblast and adipocyte differentiation
Murine C3H10T1/2 multipotent cells and 3T3-L1 cells were purchased from the American Type Culture Collection (Manassas, VA, USA) and cultured as previously described.20 Briefly, C3H10T1/2 cells were cultured in Dulbecco's modified Eagle's medium (DMEM) (Hyclone, Logan, UT, USA) including 10% fetal bovine serum (Hyclone, Logan, UT, USA). 3T3-L1 preadipocytes were maintained in DMEM with 10% fetal calf serum (Hyclone, Logan, UT, USA). To induce osteoblast differentiation, C3H10T1/2 cells were cultured to confluence and changed to the osteogenic medium consisting of DMEM, 5% FBS, 50 μg mL−1 ascorbic acid (Sigma, St. Louis, MO, USA), and 10 mM β-glycerophosphate (Sigma). The medium was changed every 2 days in the presence of the extracts. For adipocyte differentiation, the confluent cells were induced into adipocytes using adipogenic medium containing DMEM, 10% FBS, 1 μM dexamethasone (Sigma), and 5 μg mL−1 insulin (Sigma) for 2 days. After 2 days, the adipogenic medium was changed to DMEM, FBS and insulin containing media. C3H10T1/2 cells were further supplemented with 1 μM troglitazone (Sigma) to induce adipocyte differentiation. The medium was changed every 2 days in the presence of the extracts. Primary bone marrow cells were isolated from 8 week-old Sprague–Dawley female rats (Orient. Co. Ltd, Young-In, Korea) and cultured using a procedure described previously.21
Alkaline phosphatase (ALP), Oil Red O staining, and real-time quantitative polymerase chain reaction (PCR) analysis
Osteoblasts induced for 10 days were stained with 0.2 mg mL−1 5-bromo-4-chloro-3-indolyl phosphate (BCIP, Sigma) and 0.4 mg mL−1 nitro blue tetrazolium (NBT, Sigma) as described previously.22 Adipocytes induced for 7 days were fixed and stained with 0.5% Oil Red O (Sigma). To quantify staining, dyes were resolved with isopropanol and the absorbance was measured at 520 nm. To measure expression of adipocyte and osteoblast markers, real-time PCR was performed. Total RNA was isolated from the cells using TRIzol reagent (Invitrogen, Carlsbad, CA, USA) and reverse transcribed using RTase M-MLV (2640A, Takara, Ohtsu, Japan). The cDNA was amplified in a thermal cycler (Takara, Ohtsu, Japan) using the Power SYBR Premix Ex Taq (RP041A, Takara) with gene specific primer sets. The specific oligonucleotide primers were described previously.22
Animal studies
Thirty two female Sprague–Dawley rats, aged 7 weeks, from Orient. Co. Ltd (Young-in, Korea) were individually housed at constant temperature and humidity. The rats were allowed free access to water and food (solid feed 5L79, Orient. Co. Ltd, Yong-In, Korea). After adaptation, the rats were either sham operated or ovariectomized (OVX) as previously described.21 OVX rats were further divided into three groups. The control OVX group (n = 8) was administered by oral gavage once daily the vehicle (10% corn oil and 90% water) for 8 weeks. The BRE OVX group (n = 8 per group) was administered 100 mg kg−1 BRE (OVX + 100 BRE) or 200 mg kg−1 BRE (OVX + 200 BRE) by oral gavage for 8 weeks once a day (5 times per week). The BRE was suspended in 1 ml of corn oil, sonicated for 10 minutes, and diluted in 9 ml of water. The body weight and food intake were determined twice per week. At the end of treatment, rats were anesthetized with diethyl ether followed by exsanguination. The dissected right femurs were carefully trimmed and tested for bone mineral density and strength. The femur was analyzed using dual energy X-ray absorptiometry to determine the bone mineral density (forearm: X-ray, NORLAND, Bone Densitometer, USA). Other tissues such as the liver, uterus, kidneys, and epididymal fats were removed and weighed. Serum was collected by centrifugation at 3000 rpm for 10 min.
For high fat diet induced obesity, male C57BL/6 mice (4 weeks old) were purchased from Central Lab Animal Inc. (Seoul, Korea). The mice were housed three to four per cage in a temperature-controlled room with a 12 hour light/dark cycle. After 1 week adaption, the mice were divided into four groups (n = 10 per group). Mice in one group were fed a normal diet (ND, n = 10) and the other three groups were fed a high-fat diet containing 60% fat (HFD; Research Diets Inc., New Brunswick, NJ, USA). Two groups of mice fed with HFD were either orally administered 100 mg kg−1 (HFD + 100 BRE, n = 10) or 200 mg kg−1 of BRE (HFD + 200 BRE, n = 10), and the other HFD group was orally administered water every day (5 times per week) as a control (HFD, n = 10) for 8 weeks. Body weight and food intake were determined twice per week. At the end of the experiment, the overnight fasted mice were anesthetized with CO2 gas and euthanized by cardiac puncture. The dissected tissues and organs were weighed. Epididymal fat was fixed with 4% paraformaldehyde, dehydrated, and embedded in paraffin. 4 μm thick sections were subjected to hematoxylin and eosin (H&E) staining. The adipocyte size was assessed using a light microscope with a digitalized camera (Olympus AX 70, Tokyo, Japan) and image J software (NIH). For determining the mean diameter of adipocytes in adipose tissue, 3 different fields (400×) per mouse (n = 5 per group) were measured. The sections were examined in a blinded manner. Serum triacylglycerol (TG) levels of each group (n = 10 per group) were determined using enzymatic colorimetric kits (AM 157S-K, Asan Pharm Co. Ltd, Seoul, Korea). All animal studies were carried out in accordance with the guidelines of the Animal Research Committee of Sungkyunkwan University and the Institutional Animal Care and Use Committee (IACUC) of the National Academy of Agricultural Science.
UPLC apparatus and measurements
Standard compounds, C3G and P3G, were purchased from Sigma-Aldrich. The colored rice (0.1 g) was extracted with 10 mL of 40% MeOH containing 1.0% (v/v) HCl for 12 h in a vortex mixer at room temperature to form the final extract, which was then centrifuged. The extracts used for UPLC analysis were passed through a 0.2 μm filter (Advantec MFS, Inc. CA, USA) before injection into a reverse phase Bondapak™ C18. Subsequently, 2 μL portions of these solutions were injected into the UPLC system (Waters 2695 Alliance, BEH Shield RP18 column (4 μm, 2.7 mm × 100 mm, Waters)). The mobile phase was water containing 0.1% TFA in H2O (A) and 0.1% TFA in methanol (B). The gradient conditions were as follows: 0.4 min, 15% B; 8.1 min, 20% B; 0.5 min, 30% B and then a hold for 1 min before returning to the initial conditions. The flow rate was adjusted to 0.4 mL min−1, and the detection wavelength was set at 530 nm with the temperature held constant at 30 °C.
Approximately 1 mg each of cyanidin-3-O-glucoside (C3G), petunidin-3-O-glucoside (Pt3G), and peonidin-3-O-glucoside (P3G) was accurately weighed and dissolved in 40% MeOH containing 1.0% (v/v) HCl to obtain a stock solution of 1.0 mg mL−1 concentration. Calibration curves were obtained for each standard at seven different concentrations (1, 2.5, 5, 10, 25, and 50 μg mL−1). Mean areas (n = 3) generated from the standard solutions were plotted against the concentration to establish a calibration equation.
Statistical analysis
Statistical analyses were conducted using PASW Statistics 17 (SPSS Inc., Chicago, IL, USA). Data were presented as means ± SEM. Analysis of variance followed by Student–Newman–Keuls tests was used to analyze the differences in weight, gene expression, and lipid accumulation using statistical analysis software (PASW Statistics 17, IBM Corp., Somers, NY, USA). Differences in BMD, fractures, and reporter assays were analyzed with the unpaired Student's t-test. Statistical significance was defined as P < 0.05.
Results
Black rice extract (BRE) induces osteoblast differentiation
To assess whether black rice (Oryza sativa L.) extracts (BRE, Sintoheugmi) affect cellular viability, C3H10T1/2 cells were treated with BRE. Treatment with BRE from 10 μg mL−1 to 80 μg mL−1 for 24 and 48 hours did not significantly alter cell viability (Fig. 1A and B). Therefore, the tested doses of BRE were applied to investigate its effects on osteoblast differentiation. C3H10T1/2 cells were induced into osteoblasts in the presence of BRE. BRE stimulated the alkaline phosphatase activity, a marker for osteoblast differentiation (Fig. 2A). In addition, BRE also promoted ALP activities in freshly isolated rat primary bone marrow cells (Fig. 2B). Consistently, the BRE induced expression of ALP and osterix in a dose dependent manner (Fig. 2C) further demonstrated the effects of BRE on osteoblast differentiation. These data indicate that BRE can exhibit osteoinductive effects in mesenchymal C3H10T1/2 and primary bone marrow cells.
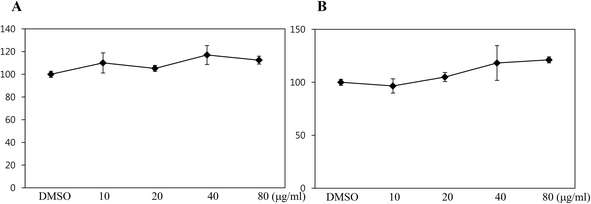 |
| Fig. 1 Cell viability of C3H10T1/2 cells with black rice extracts (BRE). (A, B) MTT assay in C3H10T1/2 cells was measured upon DMSO (control) or various concentrations of BRE treatment (10, 20, 40, and 80 μg mL−1) for 24 hours (A) and 48 hours (B). Values are expressed as the means ± SEM from three independent experiments. | |
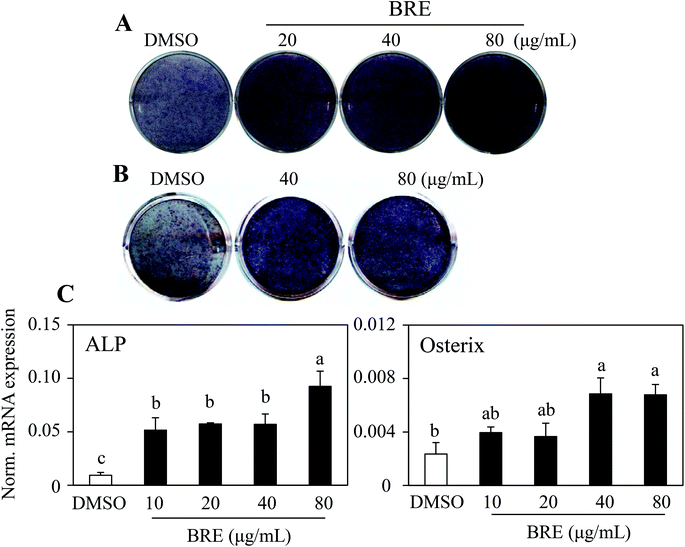 |
| Fig. 2 Black rice extracts (BRE) promote osteoblast differentiation. (A, B) BRE treatment for 10 days at the indicated doses in C3H10T1/2 cells (A) and primary bone marrow cells isolated from rat tibias (B) induced alkaline phosphatase activity. (C) BRE stimulated osteoblast marker expression in C3H10T1/2 cells. Expression of ALP (alkaline phosphatase) and osterix mRNA was measured by real time PCR. Data shown represent the means ± SEM and are representative of three independent experiments. Means with different letters at each sample are significantly different (P < 0.05) by the Student–Newman–Keuls test. | |
BRE prevents decreases in the bone mineral density and strength in OVX rats
Ovariectomized (OVX) rats have been studied to model human menopause induced bone loss.23 To test the bone protective effects of BRE in vivo, OVX rats were orally administered BRE by gavage for 8 weeks. OVX rats increased the body weight and BRE administration in OVX rats did not affect the body weight gain (Fig. 3A). Food intake was not different in OVX groups though all the OVX groups had higher feed efficiency than the sham operated group (Fig. 3B). As expected, the OVX rats presented reduced bone mineral density in femurs compared to the sham operated group. The reduced bone mineral density induced by ovariectomy was significantly protected by a daily dose of 200 mg kg−1 of BRE administration (Fig. 3C). BRE at 100 mg kg−1 did not significantly prevent the decreases in bone mineral density, but showed trends toward increases in bone density. In addition, bone strength, measured by forces to induce fractures, was also decreased by OVX and BRE treatment at both doses recovered the OVX induced weakness in bone strength (Fig. 3D). Therefore, consistent with the data in vitro, BRE administration prevented bone loss in OVX rats.
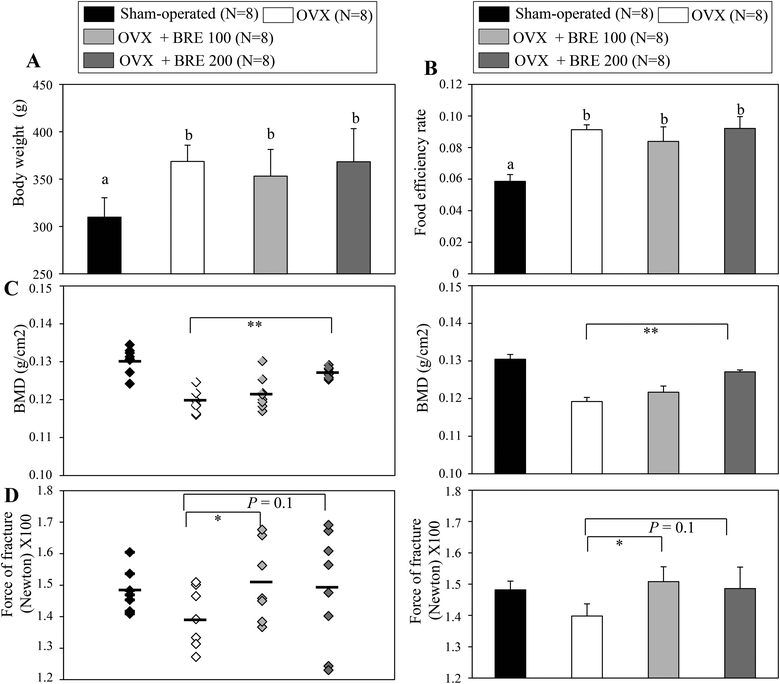 |
| Fig. 3 Black rice extracts (BRE) prevent bone loss in ovariectomized (OVX) rats. (A) Body weight and (B) food efficiency rate (body weight gain/food intake) of the sham-operated control group (N = 8), ovariectomized control group (OVX, n = 8), ovariectomized and daily dose of 100 mg kg−1 of BRE administered (OVX + 100 BRE, n = 8), and ovariectomized and daily dose of 200 mg kg−1 of BRE administered (OVX + 200 BRE, n = 8) groups. (C) Bone mineral density (BMD) of the right femur was measured using dual energy X-ray absorptiometry (DEXA). OVX rats orally administrated with BRE (OVX + BRE) prevented the loss of bone mineral density compared to the OVX rats orally administrated with the vehicle (control OVX group). Left panel, bone mineral densities of individual mice; right panel, the average of bone mineral density in each group. (D) OVX rats orally administrated with BRE (OVX + BRE) increased bone strength compared to the OVX rats orally administrated with the vehicle (control OVX group). Left panel, bone strength (fractures) of individual mice; right panel, the average of bone strength (fractures) in each group. Data are means ± SEM (n = 8). Means with different letters at each sample are significantly different (P < 0.05) by the Student–Newman–Keuls test. | |
BRE suppresses adipocyte differentiation
Recent data for age related osteoporosis showed that osteoporotic bone marrow contained fewer osteoblasts that have been replaced by adipocytes.24,25 In detailed studies, adipocytes and osteoblasts are formed from common precursor cells.26 These observations further bring the possibility of new interventions against osteoporosis by suppressing the increased adipocytes and by inducing osteoblasts from the bone marrow cells. To test this, C3H10T1/2 cells were treated with BRE and induced to differentiate into adipocytes. In contrast to the anabolic effects on osteoblasts, BRE treatment suppressed lipid accumulation as assessed by Oil Red O staining (Fig. 4A). Resveratrol (Res) at 20 μM was used as the control for anti-adipogenesis. Western blot analysis also showed reduced expression of PPARγ and its target protein, aP2 (Fig. 4B). Consistently, mRNA expression of adipocyte markers was also suppressed with BRE in a dose dependent manner (Fig. 4C). These data suggest that BRE exhibits dual effects on adipocyte and osteoblast differentiation from the common precursor cells.
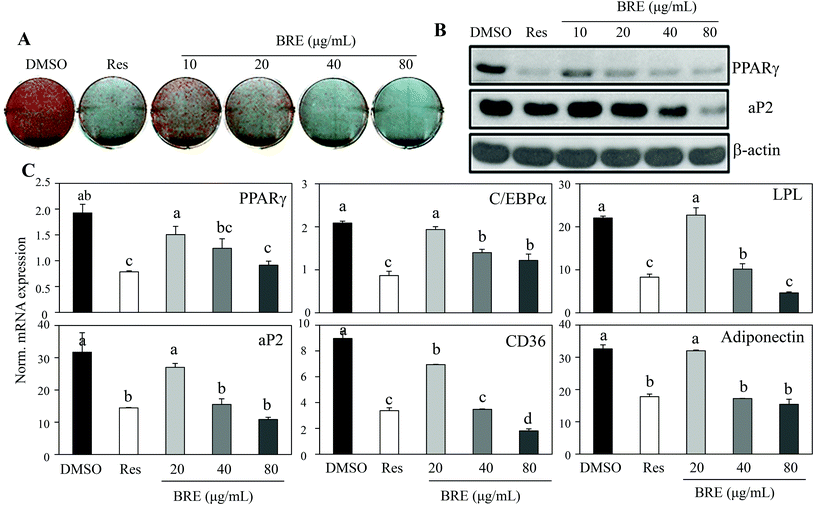 |
| Fig. 4 Black rice extracts (BRE) inhibit adipocyte differentiation of C3H10T1/2 and 3T3-L1 cells. (A) C3H10T1/2 cells were treated with BRE for 7 days during adipocyte differentiation. Oil Red O staining was performed to assess lipid accumulation. An anti-adipogenic compound, resveratrol (20 μM, Res), and DMSO were used as controls. (B) BRE inhibited PPARγ and aP2 protein expression in 3T3-L1 cells. 3T3-L1 cells were treated with the indicated concentrations of BRE and induced to differentiate for 7 days and protein expression was measured by western blotting. Actin was used as a loading control. (C) BRE suppressed the expression of adipocyte markers in C3H10T1/2 cells. The cells were treated with the indicated concentrations of BRE for 7 days during adipocyte differentiation and mRNA expression of adipogenic genes encoding PPARγ, C/EBPα, LPL, aP2, CD36, and adiponectin was measured by real-time PCR. Data shown represent the means ± SEM and are representative of three independent experiments. Means with different letters at each sample are significantly different (P < 0.05) by the Student–Newman–Keuls test. | |
BRE decreases the body weight and fat mass in HFD induced obese mice
Since BRE also inhibits adipocyte differentiation in vitro, we attempted to directly assess anti-obese effects in high fat diet induced obese mice. C57BL/6 mice were fed with a high fat diet (HFD) for 8 weeks to induce obesity and orally administered 100 mg kg−1 or 200 mg kg−1 BRE. HFD fed mice had a significant weight gain compared to the normal diet (ND) fed group. The HFD fed and BRE administered group showed dose dependently prevented weight gain compared to the HFD group (Fig. 5A and B). The decreased weight induced by HFD also paralleled the reduced weight of fat mass and the liver (Fig. 5C). Plasma triacylglycerols were also significantly decreased in the BRE administered groups compared to the HFD group (Fig. 5D). Consistently, BRE fed groups prevented enlargement of adipocytes in epididymal fats (Fig. 5E). Therefore, consistent with in vitro lipid accumulation, BRE administration also prevented obesity induced by a high fat diet further suggesting the potential roles of BRE in obesity and related diseases.
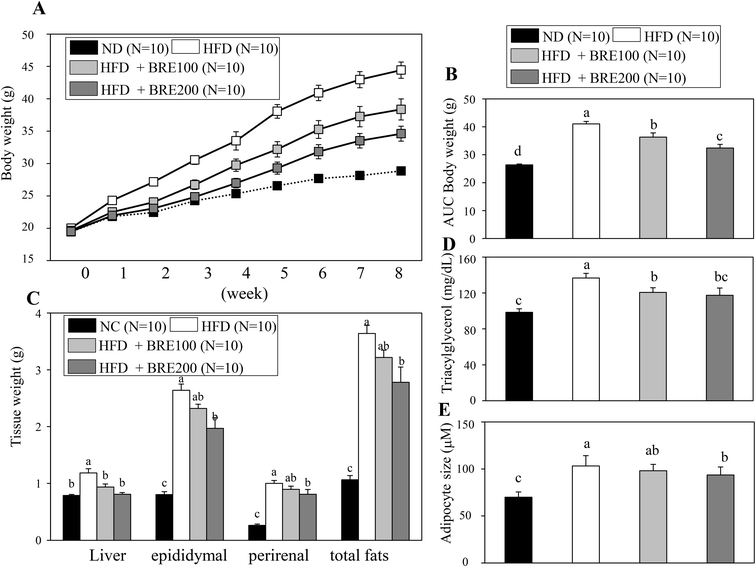 |
| Fig. 5 Black rice extracts (BRE) prevent body weight and fat mass increases in high fat diet (HFD) induced obese mice. (A) Body weights of the normal diet fed (ND, n = 10) group, high fat diet fed group orally administered with the vehicle for 8 weeks (HFD, n = 10), HFD mice orally administered with a daily dose of 100 mg kg−1 of BRE for 8 weeks (HFD + 100 BRE, n = 10), and HFD mice orally administered with a daily dose of 200 mg kg−1 of BRE for 8 weeks (HFD + 200 BRE, n = 10). (B) Significant differences in the area under the curve (AUC) of body weight changes in the HFD and HFD + BRE treated groups. (C) Liver and fat weights of ND, HFD, HFD + 100 BRE, and HFD + 200 BRE groups. Data are means ± SEM. (D) Plasma triacylglycerol levels in ND, HFD, HFD + 100, and HFD + 200 BRE groups (n = 10 per group). (E) Sections (4 μM) of epididymal fat tissues were stained with hematoxylin and eosin and quantified. Smaller adipocyte sizes in HFD + BRE groups compared to the HFD control group are shown (n = 5 per group). Data are means ± SEM. Data were analyzed by analysis of variance (ANOVA) with different letters indicating a significant difference (P < 0.05). | |
Anthocyanins in BRE are responsible for the anti-adipogenic actions
The dual actions of BRE in adipocytes and osteoblasts suggest applications to bone and fat related diseases. To further define the active components responsible for the activities of BRE, chemical constituents were determined by UPLC. BRE has been shown to suppress fatty liver through its active component anthocyanins.27,28 To test this possibility, anthocyanins were analyzed and quantified. As shown in Fig. 6A, cyanidin-3-O-glucBRE (C3G) and peonidin-3-O-glucoside (P3G) were the most abundant anthocyanins in BRE. These results indicate the possibility of anthocyanins as regulators for the adipocyte-osteoblast axis from the precursor cells. C3G and P3G were administered to C3H10T1/2 cells to test whether they can mediate the pro-osteogenic and anti-adipogenic actions of BRE. C3G effectively inhibited lipid accumulation and induction of adipocyte markers during adipocyte differentiation. Additionally, P3G also suppressed lipid accumulation and expression of PPARγ similar to the effects of BRE on adipocyte differentiation (Fig. 6B and C). C3G and P3G were also treated and induced to differentiate into osteoblasts. As expected, BRE treatments effectively induced ALP activity and expression of ALP mRNA. However, both anthocyanins failed to induce ALP activity or expression of ALP (Fig. 6D and E). Thus, high contents of both anthocyanins in BRE are, at least in part, responsible for the anti-adipogenic effects of BRE but not for the pro-osteogenic actions.
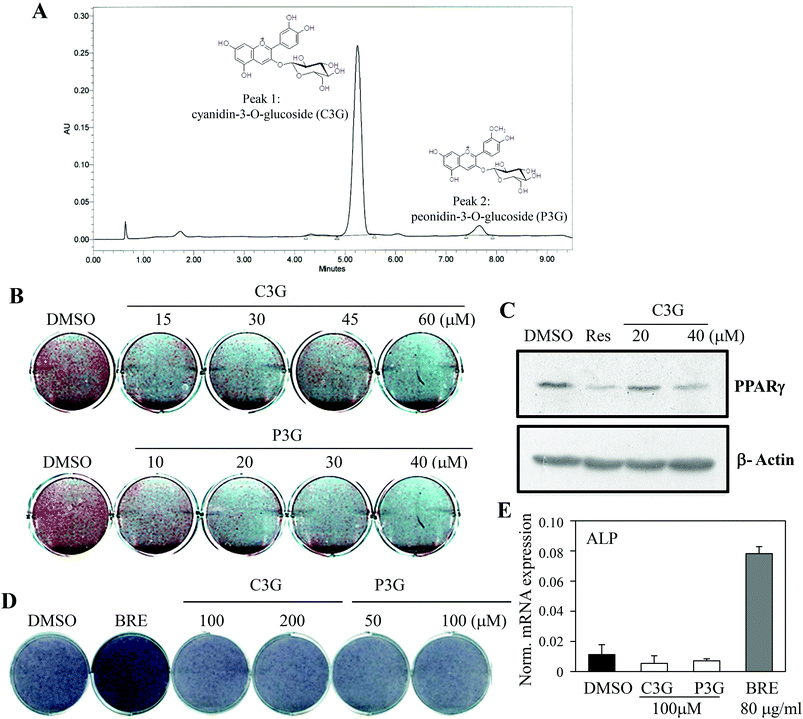 |
| Fig. 6 Cyanidin-3-O-glucoside (C3G) and peonidin-3-O-glucoside (P3G) in black rice extracts (BRE) are responsible for the inhibitory action on adipocyte differentiation. (A) Identification of cyanidin-3-O-glucoside (C3G) and peonidin-3-O-glucoside (P3G) in BRE. Liquid chromatograms of anthocyanin extracted from BRE. Peak 1, cyanidin- 3-O-glucoside; Peak 2, peonidin-3-O-glucoside (P3G). (B, C) C3G and P3G can mediate the anti-adipogenic actions of BRE. C3G (top) and P3G (bottom) were treated for 7 days at the indicated doses during adipocyte differentiation in C3H10T1/2 cells and lipid accumulation was assessed by Oil Red O staining (B) and expression of PPARγ by western blotting (C). Resveratrol (20 μM, Res) was used as a control. (D, E) C3G and P3G failed to promote osteoblast differentiation. C3G and P3G were treated for 10 days at the indicated doses in C3H10T1/2 cells followed by measurement of alkaline phosphatase activities (D). (E) C3G and P3G at 100 μM and BRE at 40 μg mL−1 were treated for 10 days in C3H10T1/2 cells and expression of ALP was measured by real time PCR. | |
BRE regulates adipocyte and osteoblast differentiation through stimulation of WNT signaling cascades
Pro-osteogenic and anti-adipogenic activities of BRE suggest that a specific signaling pathway can be regulated by BRE. Indeed, several signaling pathways were previously shown in adipocyte and osteoblast differentiation in vitro and in vivo models. Activation of Wnt, TGF-β, and BMP pathways can stimulate osteoblast differentiation whereas inhibition of Wnt, TGF-β, Hedgehog, and C/EBP can suppress adipocyte differentiation.15 To determine the molecular mechanisms of BRE, pathway selective reporter assays were employed. Signaling pathway-driven luciferase activation has been widely used as a reporter to assess the impacts of genes and bioactive compounds.29 Wnt, TGF-β, BMP, hedgehog, and C/EBP specific luciferase reporter plasmids were transiently transfected into 3T3-L1 cells and treated with BRE. The PGL3b empty vector was used as a negative control. In three repeated experiments, we consistently observed that Wnt driven luciferase (TOP flash) was selectively induced by the treatment with BRE but not by other signaling pathway-selective luciferase and control luciferase (PGL3b) activities (Fig. 7A). Consistent with the pro-osteogenic and anti-adipogenic actions of BRE, numerous reports showed that the activation of the Wnt signaling pathway drives osteoblast differentiation but prevents adipocyte differentiation.30 Therefore, our results of the effects of BRE on Wnt stimulation would be consistent with the previous observations.
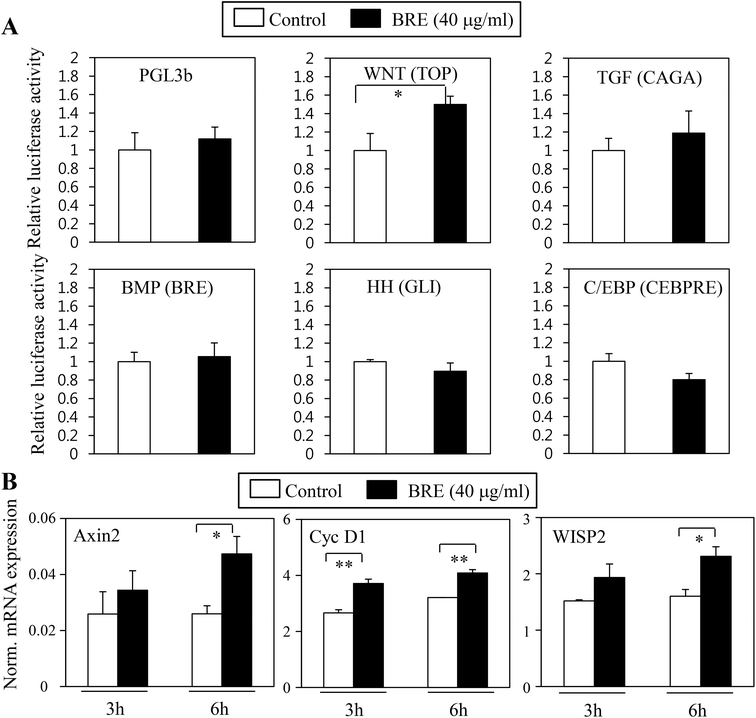 |
| Fig. 7 Black rice extracts (BRE) stimulate Wnt signaling pathways to mediate anti-adipogenic and pro-osteogenic actions. (A) BRE specifically activates Wnt signaling pathways, but not other pathways related to osteoblast or adipocyte differentiation. 3T3-L1 cells were transiently transfected with luciferase constructs of either empty vector control (PGL3b) or signaling pathway selective Wnt (TOP), TGF-β (CAGA), BMP (BRE), hedgehog (GLI), and C/EBP (CEBPRE) reporter plasmids followed by treatment with 40 μg mL−1 of BRE for 24 hours. BRE induced Wnt-specific reporter activation but not other signaling driven luciferase activities. Values are expressed as the means ± SEM from three independent experiments. Statistical significance was determined compared to a control by Student's t-test (*P < 0.05). (B) BRE treatments at 40 μg mL−1 induced expression of Wnt signaling target genes in C3H10T1/2 cells. BRE induced expression of Axin2, Cyclin D1 (CycD1), and WISP2. Gene expression was measured by real-time PCR and normalized to the expression of 36B4 cells. Values are expressed as the means ± SEM from three independent experiments. Statistical significance was determined compared to a control by Student's t-test (*P < 0.05; **P < 0.005). | |
To further show the effects of BRE on Wnt activation, BRE was applied to C3H10T1/2 cells and expression of Wnt specific target genes was determined. In line with the effects on luciferase reporter systems, BRE stimulated the expression of Axin2, Cyclin D1 (Cyc D1), and WISP2 (Fig. 7B), further suggesting that BRE can regulate adipocyte and osteoblast differentiation through stimulation of Wnt signaling cascades.
Discussion
Black rice has been consumed widely as cereal food, a food colorant, and functional food.28 Black rice has been shown to be an antioxidant, anticarcinogenic, antiatherosclerosis, and antiallergic medicinal plant.28,31–33 Recent studies have also shown that BRE prevented hepatic steatosis in the high fat diet induced fatty liver.27 However, the effects on fats and bones by BRE remain to be investigated.
Black rice (Oryza sativa L.) was shown to contain various bioactive compounds including flavones, tannins, phenolics, and γ-oryzanol.27,28,34 In particular, anthocyanins in BRE are major bioactive compounds of black rice.35 In line with this, our data show that anthocyanins are major anti-adipogenic effectors. Treatment with anthocyanins suppressed lipid accumulation and expression of adipocyte markers. Furthermore, differential contents of anthocyanins from various species of black rice (Heugjinjubyeo, Sintoheugmi, and Josaengheugchal) are well correlated with the ability to inhibit adipocyte differentiation (data not shown). However, pro-osteogenic actions of several species of BRE are not related to the contents of anthocyanins. In addition, treatment with C3G and P3G did not induce osteoblast differentiation. These data show that anthocyanins are responsible for the anti-adipogenic actions of BRE but other components contribute to the pro-osteogenic effects of BRE. Other compounds contained in BRE such as isovitexin, γ-oryzanol, and γ-aminobutyric acid also did not regulate osteoblast differentiation (data not shown). Ferulic acid and coumaric acid were previously identified as phenolic compounds in BRE21,36 and these two compounds were indeed recognized as pro-osteogenic compounds.21,37,38 Therefore, it is possible that these two compounds can mediate the pro-osteogenic effects of BRE. Further studies will be performed to identify the pro-osteogenic compounds from BRE.
Bone forming osteoblasts and fat storing adipocytes are derived from common stem cells.11 Generation of adipocytes and osteoblasts from common precursor cells is tightly regulated by various signaling cascades.9 Signaling pathways including Wnt, TGF-β, Hedgehog, BMP, PPARγ, and C/EBP cascades can dictate the adipocyte or osteoblast fates.15,30 The dual activities of BRE in pro-bone and anti-fat may affect these signaling cascades. We showed that BRE promoted Wnt-driven luciferase activities, but not other signaling selective luciferase activities. Wnt target genes were also induced by the treatment with BRE. Altogether, the stimulatory effect on Wnt signaling by black rice correlates well with the pro-osteogenic and anti-adipogenic effects of Wnt activation. Although, detailed molecular mechanisms of stimulating Wnt signaling pathways by BRE still need to be investigated, our data show that black rice promotes Wnt signaling to induce osteoblast differentiation but suppress adipocyte differentiation.
The formation of osteoblasts and adipocytes should be properly controlled in bones and fat tissues.39 Fewer bone-forming cells in the bone marrow of age related osteoporotic bones are counter balanced by enhanced adipogenic cells.40 Osteoporotic bones caused by estrogen deficiency in menopausal women also tended to increase with the amount of adipocytes with concomitant reduction of osteoblasts from bone marrow cells.24 Therefore, it is plausible that reprogramming into osteoblast cells with less adipogenic fates in osteoporotic bones can be an effective strategy to avoid osteoporosis. This notion can be supported by the recent studies on PPARγ deleted mice that these engineered mice increased bone formation and prevented osteoporosis by inducing osteoblast differentiation and inhibiting adipocyte differentiation from bone marrow cells.41 Therefore, identification of osteoinductive and anti-adipogenic bioactive ingredients can be useful to combat osteoporosis as well as obesity and its related diseases. Our data show that BRE can reprogram stem cells to differentiate into osteoblasts and not adipocytes. Future studies will be performed to further verify that BRE can be effective in preventing human obesity and osteoporosis.
Conclusion
This is the first time that the effects of black rice extracts (BRE) on osteoblast differentiation have been described. In this study, we show that BRE inhibits adipocyte differentiation but stimulates osteoblast differentiation in vitro. These dual effects of BRE are reproducible in high fat-diet fed obese mice and ovariectomized rats. These effects are probably mediated through the stimulation of Wnt signaling pathways as shown by Wnt specific luciferase activation and Wnt-selective target gene induction. Taken together, our data show that BRE can be useful in preventing diet induced obesity and hormone deficient osteoporosis.
Conflicts of interest
The authors have no conflict of interest affecting the conduct or reporting of the work submitted.
Abbreviations
BRE | Black rice (Oryza sativa L.) extracts |
OVX | Ovariectomized |
ND | Normal diet |
HFD | High fat diet |
Wnt | Wingless/int-1 |
TGF-β | Transforming growth factor- β |
BMP | Bone morphogenetic protein |
PPARγ | Peroxisome proliferator-activated receptor γ |
ALP | Alkaline phosphatase |
C3G | Cyanidin-3-O-glucoside |
P3G | Peonidin-3-O-glucoside |
Acknowledgements
This study was supported by a grant from the AGENDA program (project no. PJ008447), Rural Development Administration, Republic of Korea and also supported by the Basic Science Research Program through the National Research Foundation of Korea (NRF) funded by the Ministry of Education, Science and Technology (NRF-2013R1A1A2060447).
References
- P. Salari Sharif, M. Abdollahi and B. Larijani, Rheumatol. Int., 2010, 31, 289–300 CrossRef PubMed.
- S. C. Manolagas, Aging, 1998, 10, 182–190 CAS.
- C. J. RBREn and M. L. Bouxsein, Nat. Clin. Pract. Rheumatol., 2006, 2, 35–43 CrossRef PubMed.
- J. H. Clark, Nucl. Recept. Signal., 2006, 4, e023 Search PubMed.
- R. H. Karas, Clin. Obstet. Gynecol., 2004, 47, 489–499 CrossRef PubMed.
- J. J. Carey, Cleve. Clin. J. Med., 2005, 72, 1033–1039 CrossRef.
- B. C. Silva and J. P. Bilezikian, Annu. Rev. Med., 2010, 62, 307–322 CrossRef PubMed.
- I. R. Reid, Semin. Cell Dev. Biol., 2008, 19, 473–478 CrossRef CAS PubMed.
- M. E. Nuttall and J. M. Gimble, Curr. Opin. Pharmacol., 2004, 4, 290–294 CrossRef CAS PubMed.
- F. Veronesi, P. Torricelli, V. Borsari, M. Tschon, L. Rimondini and M. Fini, Crit. Rev. Eukaryot. Gene Expr., 2011, 21, 363–377 CrossRef CAS.
- M. Owen, J. Cell Sci., Suppl., 1988, 10, 63–76 CrossRef CAS.
- N. A. Sims and J. H. Gooi, Semin. Cell Dev. Biol., 2008, 19, 444–451 CrossRef CAS PubMed.
- P. Bianco, M. Riminucci, S. Gronthos and P. G. Robey, Stem Cells, 2001, 19, 180–192 CrossRef CAS PubMed.
- D. J. Prockop, Science, 1997, 276, 71–74 CrossRef CAS.
- K. W. Park, D. S. Halperin and P. Tontonoz, Cell Metab., 2008, 8, 454–457 CrossRef CAS PubMed.
- R. C. Muhlbauer, A. Lozano, S. Palacio, A. Reinli and R. Felix, Bone, 2003, 32, 372–380 CrossRef CAS.
- S. E. Putnam, A. M. Scutt, K. Bicknell, C. M. Priestley and E. M. Williamson, Phytother. Res., 2007, 21, 99–112 CrossRef PubMed.
- C. Y. Lai, J. Y. Yang, S. Rayalam, M. A. Della-Fera, S. Ambati, R. D. Lewis, M. W. Hamrick, D. L. Hartzell and C. A. Baile, J. Med. Food, 2011, 1352–1362 CrossRef CAS PubMed.
- J. N. Beresford, J. H. Bennett, C. Devlin, P. S. Leboy and M. E. Owen, J. Cell Sci., 1992, 102(Pt 2), 341–351 CAS.
- S. R. Jung, N. J. Song, D. K. Yang, Y. J. Cho, B. J. Kim, J. W. Hong, U. J. Yun, D. G. Jo, Y. M. Lee, S. Y. Choi and K. W. Park, Nutr. Res., 2013, 33, 162–170 CrossRef CAS PubMed.
- M. J. Kim, W. S. Jang, I. K. Lee, J. K. Kim, K. S. Seong, C. R. Seo, N. J. Song, M. H. Bang, Y. M. Lee, H. R. Kim, K. M. Park and K. W. Park, J. Med. Food, 2014, 17, 772–781 CrossRef CAS PubMed.
- K. W. Park, H. Waki, W. K. Kim, B. S. Davies, S. G. Young, F. Parhami and P. Tontonoz, Mol. Cell Biol., 2009, 29, 3905–3914 CrossRef CAS PubMed.
- D. N. Kalu, Bone Miner., 1991, 15, 175–191 CrossRef CAS.
- G. V. Halade, M. M. Rahman, P. J. Williams and G. Fernandes, J. Nutr. Biochem., 2010, 21, 1162–1169 CrossRef CAS PubMed.
- S. Botolin and L. R. McCabe, Endocrinology, 2007, 148, 198–205 CrossRef CAS PubMed.
- L. Pei and P. Tontonoz, J. Clin. Invest., 2004, 113, 805–806 CrossRef CAS.
- H. H. Jang, M. Y. Park, H. W. Kim, Y. M. Lee, K. A. Hwang, J. H. Park, D. S. Park and O. Kwon, Nutr. Metab., 2012, 9, 27 CAS.
- C. Hu, J. Zawistowski, W. Ling and D. D. Kitts, J. Agric. Food Chem., 2003, 51, 5271–5277 CrossRef CAS PubMed.
- S. J. Gould and S. Subramani, Anal. Biochem., 1988, 175, 5–13 CrossRef CAS.
- M. I. Lefterova and M. A. Lazar, Trends Endocrinol. Metab., 2009, 20, 107–114 CrossRef CAS PubMed.
- H. F. Liao, Y. Y. Chen, Y. C. Yang, C. S. Wang and Y. J. Chen, Food Chem. Toxicol., 2006, 44, 1724–1729 CrossRef CAS PubMed.
- Y. R. Lee, C. E. Kim, M. Y. Kang and S. H. Nam, Ann. Nutr. Metab., 2007, 51, 519–526 CrossRef CAS PubMed.
- R. Kumar, P. Srivastava, D. Kumari, H. Fakhr, S. Sridhara, N. Arora, S. N. Gaur and B. P. Singh, Immunobiology, 2007, 212, 141–147 CrossRef CAS PubMed.
- T. Laokuldilok, C. F. Shoemaker, S. Jongkaewwattana and V. Tulyathan, J. Agric. Food Chem., 2011, 59, 193–199 CrossRef CAS PubMed.
- G. F. Deng, X. R. Xu, Y. Zhang, D. Li, R. Y. Gan and H. B. Li, Crit. Rev. Food Sci. Nutr., 2013, 53, 296–306 CrossRef CAS PubMed.
- H. I. Jun, G. S. Song, E. I. Yang, Y. Youn and Y. S. Kim, J. Food Sci., 2012, 77, C759–C764 CrossRef CAS PubMed.
- S. Sassa, T. Kikuchi, H. Shinoda, S. Suzuki, H. Kudo and S. Sakamoto, In Vivo, 2003, 17, 277–280 CAS.
- J. Folwarczna, M. Zych, J. Burczyk, H. Trzeciak and H. I. Trzeciak, Planta Med., 2009, 75, 1567–1572 CrossRef CAS PubMed.
- B. Lecka-Czernik and L. A. Stechschulte, Arch. Biochem. Biophys., 2014, 124–129 CrossRef CAS PubMed.
- E. J. Moerman, K. Teng, D. A. Lipschitz and B. Lecka-Czernik, Aging Cell, 2004, 3, 379–389 CrossRef CAS PubMed.
- F. Wang, S. E. Mullican, J. R. DiSpirito, L. C. Peed and M. A. Lazar, Proc. Natl. Acad. Sci. U. S. A., 2013, 110, 18656–18661 CrossRef CAS PubMed.
Footnotes |
† Equal contribution. |
‡ Current address: Department of Food and Nutrition, Seoul Women's University, Seoul, 139-774, Korea. |
|
This journal is © The Royal Society of Chemistry 2015 |
Click here to see how this site uses Cookies. View our privacy policy here.