DOI:
10.1039/C3FO60400D
(Paper)
Food Funct., 2014,
5, 158-166
Astaxanthin alleviates brain aging in rats by attenuating oxidative stress and increasing BDNF levels
Received
9th September 2013
, Accepted 9th November 2013
First published on 11th November 2013
Abstract
Astaxanthin (AST) is a carotenoid pigment which possesses potent antioxidative, anti-inflammatory, and neuroprotective properties. The aim of this study was to investigate whether administration of AST had protective effects on D-galactose-induced brain aging in rats, and further examined its protective mechanisms. The results showed that AST treatment significantly restored the activities of glutathione peroxidase (GSH-PX) and superoxide dismutase (SOD), and increased glutathione (GSH) contents and total antioxidant capacity (T-AOC), but decreased malondialdehyde (MDA), protein carbonylation and 8-hydroxy-2- deoxyguanosine (8-OHdG) levels in the brains of aging rats. Furthermore, AST increased the ratio of Bcl-2/Bax, but decreased the expression of Cyclooxygenase-2 (COX-2) in the brains of aging rats. Additionally, AST ameliorated histopathological changes in the hippocampus and restored brain derived neurotrophic factor (BDNF) levels in both the brains and hippocampus of aging rats. These results suggested that AST could alleviate brain aging, which may be due to attenuating oxidative stress, ameliorating hippocampus damage, and upregulating BDNF expression.
1. Introduction
The aging process in humans is a complex and natural process that brings about many physiological changes and functional impairments. Brain aging is an important aspect of the aging process. Considerable experimental evidence suggests that brain aging has been implicated in the development of neurodegenerative diseases, such as Alzheimer's disease (AD) and Parkinson's disease (PD).1–4 Thus, it is extremely important to search for materials which can prevent or retard brain aging and to explore the preventive mechanisms.
The mechanisms underlying the process of aging are not completely understood so far, and it is safe to say that they are multifactorial and complex. One widely acknowledged theory in relation to the mechanisms of aging is referred to as the oxidative stress theory.2,5,6 The theory holds that the progressive declines in physiological function are due to the accumulation of oxidative damage induced by excessive reactive oxygen species (ROS) and free radicals. In addition, recent studies reveal that chronic inflammation is a major risk factor resulting in aging and age-related diseases.7 The pro-inflammatory mediators are continuously upregulated during the aging process, which can generate a great deal of reactive species and ultimately cause aging and age-related diseases.
On the other hand, recent accumulating evidence indicates that dysregulation of the apoptotic process may be involved in some aging processes and may contribute to age-associated changes such as the progressive decline of physiologic function and significant increases in the incidence of degenerative diseases.8 The loss of neurons mediated by apoptosis is closely associated with functional impairments such as dementia and motor neuron disability in neurodegenerative diseases.2
Brain derived neurotrophic factor (BDNF), a small dimeric protein, is one of the neurotrophic factors. BDNF plays a key role not only in regulating neuronal development, maintenance and survival, but also in regulating the cognition, formation and storage of memories. Amounts of BDNF and/or its receptors are reduced in hippocampus of AD and normal aging animals, which may contribute to synaptic and cellular loss and memory deficits.9–12
Considerable research suggests that dietary supplementation with natural antioxidants, such as polyphenols, carotenoids, vitamin C, and vitamin E, exerts potent effects on relieving brain aging and age-related diseases.13–16
Astaxanthin (AST), a red-orange carotenoid pigment, naturally exists in many aquatic animals, such as shrimp, crab and salmon. It has been reported that AST has potent antioxidative, anti-inflammatory, and neuroprotective effects.17–20 Our previous study has shown that AST can inhibit ROS-mediated cellular toxicity in dopaminergic SH-SY5Y cells.21 However, it is unclear whether the administration of AST could inhibit brain aging in vivo. In the present study, we investigated whether supplementation of AST had protective effects on D-galactose (D-G)-induced brain aging in rats, and further examined the mechanisms of its action.
2. Material and methods
2.1. Reagents
Astaxanthin emulsifier (0.5%, w/v) was purchased from Hubei Astaxanthin Biotechnology Incorporated (Jingzhou, China), which was produced by mixing the astaxanthin (3S,3′S-astaxanthin) extracted from Haematococcus pluvialis powder with starch ester. D-Galactose, 2,4-dinitrophenylhydrazine (DNPH) and anti-DNP (2,4-dinitrophenyl) polyclonal antibody were purchased from Sigma-Aldrich (St. Louis, MO, USA). The malonaldehyde (MDA), superoxide dismutase (SOD), glutathione peroxidase (GSH-PX), glutathione (GSH), and total antioxidant capacity (T-AOC) assay kits were purchased from Nanjing Jiancheng Bioengineering Institute (Nanjing, China). The 8-hydroxy-2-deoxyguanosine (8-OHdG) ELISA kit was purchased from Tsz scientific llc (Boston, USA). Goat anti-COX-2 (sc-1747), rabbit anti-BDNF (sc-546), rabbit anti-Bcl-2 (sc-492), rabbit anti-Bax (sc-493), mouse anti-α-Tubulin (sc-5286), goat-anti-rabbit IgG-HRP (sc-2004), and goat-anti-mouse IgG-HRP (sc-2005) antibodies were purchased from Santa Cruz Biotechnology (Santa Cruz, CA, USA). 3,3′-Diaminobenzidine (DAB) and rabbit anti-BDNF (BA0565) antibody were purchased from Wuhan Boster Bio-engineering Limited Compony (Wuhan, China). SP-9001 Histostain™-Plus Kits were obtained from Beijing Zhongshan Golden Bridge Biotechnology Corporation (Beijing, China). Bicinchoninic acid (BCA) protein assay kit and enhanced chemiluminescence (ECL) kit were from Thermo Fisher Scientific Inc (Rockford, IL, USA). All solvents and chemicals used in the study were of analytical grade.
2.2. Animals and administration
A total of 40 male Sprague-Dawley rats (SD), weighing 200 ± 20 g, were obtained from the Laboratorial Animal Center of Xi'an Jiaotong University (Xi'an, Shaanxi, China). The rats were kept on a 12-hour light–dark cycle at a constant temperature (21 ± 1 °C) with food and water ad libitum. The animal experiment was carried out in accordance with the National Institutes of Health Guide for the Care and Use of Laboratory Animals (NIH Publications no. 80-23) revised 1996 and approved by the Northwest A&F University Animal Care and Use Committee (Yangling, Shaanxi, China). Every effort was made to minimize the number of animals used and their suffering. After one week of adaptation, the rats were randomly divided into four groups, each consisting of 10 rats. The four groups were designed as follows:
Group 1 (Con group): saline (intraperitoneally injected, i.p.) + emulsifier without AST (orally administered, p.o.);
Group 2 (D-G group): D-G (i.p.) + emulsifier without AST (p.o.);
Group 3 (D-G + AST group): D-G (i.p.) + AST emulsifier (p.o.);
Group 4 (AST group) : saline (i.p.) + AST emulsifier (p.o.).
Rats in group 2 and group 3 were intraperitoneally injected (i.p.) with D-G (in physiological saline, 150 mg mL−1) at a volume of 2 mL kg−1 body weight to arrive at a dose of 300 mg kg−1 body weight once daily, while rats in group 1 and group 4 were treated with the same volume of physiological saline (2 mL kg−1 body weight) per day. After injection, rats in group 3 and group 4 were treated with AST emulsifier by intragastric administration at the dose of 0.02% of daily diet three times weekly,22 and rats in group 1 and group 2 were orally administrated with emulsifier without AST at the same volume. In the present study, the daily feed intake of a rat was 30 g. The study was carried out for a period of 8 weeks.
2.3. Preparation for brain samples
The rats were sacrificed after being anaesthetised with 10% chloralic hydras (in physiological saline, 4 mL kg−1 body weight). In each group, five rats were used for the biochemical analysis including the levels of GSH, MDA, T-AOC and 8-OHdG, and activities of SOD and GSH-PX in brain homogenate, and another five rats were used for histopathological examination and immunohistochemistry tests in the hippocampus, and for testing the expression of some proteins in the brains by the Western blot method.
The brains of the rats were carefully and quickly removed and put into cold physiological saline. The hippocampus were immediately separated from the cerebrums on a cold plate and stored in 4% phosphate-buffered paraformaldehyde (in 0.1 M phosphate buffer, pH 7.4). The rest of the brain was stored at −80 °C for subsequent experiments.
2.4. Biochemical analysis
The brains of the rats were homogenized in ice-cold physiological saline to form brain homogenate (1 g tissue in 9 mL physiological saline). The homogenate was then centrifuged at 4000g for 10 min and the supernatant was used for the subsequent tests. The concentration of protein was measured by using a commercially available BCA protein assay kit and using bovine serum albumin (BSA) as a standard. The levels of GSH, MDA and T-AOC, and the activities of SOD and GSH-PX in the brain homogenate were assayed using kits. 8-OHdG levels were measured by using an 8-OHdG ELISA kit.
2.5. Protein carbonylation assay
The levels of protein carbonyl groups were determined using an anti-DNP specific antibody.23 In brief, the samples were separated by sodium dodecyl sulfate polyacrylamide gels electrophoresis (SDS-PAGE) and transferred to polyvinylidene fluoride (PVDF) membranes, then derivated with 10 mM DNPH (in 2 M HCl) for 15 min. The membranes were washed three times in 2 M HCl and five times in 100% methanol (5 min each time), then blocked with 5% non-fat milk and incubated in a 1
:
2500 dilution of rabbit anti-DNP polyclonal antibody at 4 °C overnight, followed by incubating in a 1
:
2000 dilution of goat anti-rabbit IgG-HRP antibody at room temperature for 2.5 h. Blots were developed by an ECL kit and visualized on a ChemiDoc XRS gel documentation system (Bio-Rad, Hercules, CA, USA).
2.6. Western blot analysis
The brain samples were homogenized with ice-cold lysis buffer (1 g tissue in 9 mL lysis buffer) and kept at 4 °C for 0.5 h, then centrifuged at 12
000g for 15 min at 4 °C and the supernatant was used for Western blot tests. After measuring the protein concentration of the brain homogenate using a BCA protein assay kit, protein samples were mixed with loading buffer and heated at 100 °C for 5 min. Then the samples (total protein content was 40 μg) were separated by SDS-PAGE and transferred onto PVDF membranes. Then the membranes were blocked with 5% skim milk dissolved in TBST (20 mM Tris, 166 mM NaCl, and 0.05% Tween 20, pH 7.5) for 2 h. After that, the membranes were washed with TBST and incubated with the primary antibodies in an appropriate dilution (anti-COX-2, 1
:
1200; anti-Bcl-2, 1
:
500; anti-Bax, 1
:
500; anti-BDNF, 1
:
300; anti-α-Tubulin, 1
:
1000) at 4 °C overnight. After washing with TBST, the membranes were further incubated with horseradish peroxidase-conjugated secondary antibodies diluted in TBST for 2 h at room temperature. Target proteins in the blots were detected by using an ECL kit, and visualized with the ChemiDoc XRS gel documentation system. The equivalent loading of samples was confirmed using the anti-α-Tubulin antibody. The optical density scores of the bands were calculated to analyze the relative contents of COX-2, Bcl-2, Bax, and BDNF after normalization with α-Tubulin values in the same lane.
2.7. Histopathological examination
The hippocampus fixed in 4% paraformaldehyde were dehydrated with graded ethanol, vitrificated with xylene, embedded in paraffin, sectioned, dewaxed, rehydrated, stained with haematoxylin and eosin and examined under a Motic BA400 microscope (Motic Co., Ltd., Causeway Bay, HongKong, China).
2.8. Immunohistochemistry
Immunohistochemistry was performed as previously described.24 The hippocampus sections were dewaxed prior to analysis. Using the microwave antigen retrieval method (in Tris–EDTA antigen retrieval fluid, pH 9.0) to expose the antigenic sites, the sections were then rinsed in phosphate buffered saline (PBS, 0.01 M, pH 7.4) three times (5 min each). The sections were subsequently incubated in 3% H2O2 for 15 min at room temperature to eliminate endogenous peroxidase, then rinsed in PBS three times (5 min each). After blocking in normal goat serum for 2 h at room temperature, the sections were incubated with the anti-BDNF antibody at 4 °C overnight. The sections were washed in PBS three times (5 min each) and incubated with the biotinylated secondary antibody for 30 min at 37 °C. After washing them in PBS three times (5 min each), the sections were incubated with a horseradish peroxidase-conjugated streptavidin complex for 30 min at 37 °C. Then the sections were rinsed in PBS three times (5 min each), and developed with a chromogenic substrate DAB, then fully washed with tap water. Finally, the staining was examined under an Eclipse 80i microscope (Nikon, Japan).
2.9. Statistical analyses
All values for each group were given as mean ± standard deviation (S.D.). The data were analysed using the one-way analysis of variance (ANOVA) followed by Tukey's multiple-range test of the SPSS 16.0 system. Any p-values less than 0.05 were considered to be statistically significant.
3. Results
3.1. Effects of AST on MDA levels in the brains of D-G-treated rats
As shown in Fig. 1A, compared with the control group, D-G treatment caused an obvious increase in MDA levels in the brains (p < 0.05), however, the elevation of MDA levels in D-G treated rats was significantly attenuated by administration of AST (p < 0.05).
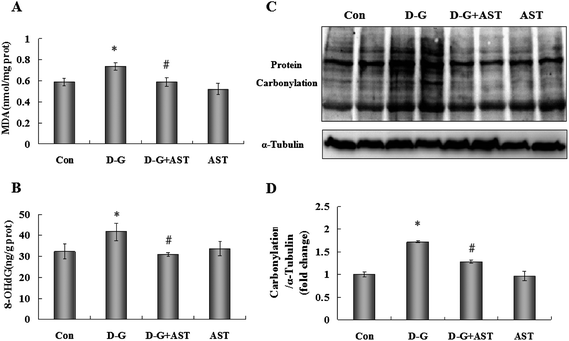 |
| Fig. 1 Effects of astaxanthin on oxidative damage in the brains of SD rats. (A) The MDA levels in the brains. (B) The 8-OHdG levels in the brains. (C) The protein carbonylation levels in the brains. (D) Quantification of protein carbonylation by densitometric analysis. The four groups were the control group (Con); D-galactose alone group (D-G); astaxanthin alone group (AST); and D-galactose plus astaxanthin group (D-G + AST). Bars represent mean ± S.D. (n = 5). *p < 0.05 vs. Con group, #p < 0.05 vs. D-G group; one-way ANOVA. | |
3.2. Effects of AST on 8-OHdG levels in the brains of D-G-treated rats
As shown in Fig. 1B, the contents of 8-OHdG in the brains of D-G treated rats were markedly increased (p < 0.05). In contrast, AST administration suppressed the increase in the 8-OHdG levels compared to the D-G group (p < 0.05).
3.3. Effects of AST on protein carbonylation levels in the brains of D-G-treated rats
As shown in Fig. 1C and D, D-G treatment caused an obvious increase in protein carbonylation levels in the brains (p < 0.05), while AST treatment suppressed the increase in protein carbonylation contents compared to the D-G group (p < 0.05).
3.4. Effects of AST on the antioxidant activities in the brains of D-G-treated rats
In order to evaluate the effects of AST administration on the antioxidant capacity in the brains of aging rats, we measured the GSH-PX and SOD activities, GSH and T-AOC levels in the brains. As shown in Fig. 2, the D-G treated rats showed a significant decrease in the activities of GSH-PX and SOD, and in the levels of GSH and T-AOC in the brains compared with the control group (p < 0.05), while AST treatment alleviated the reduction of antioxidant capacity in the brains of the D-G-treated rats (p < 0.05).
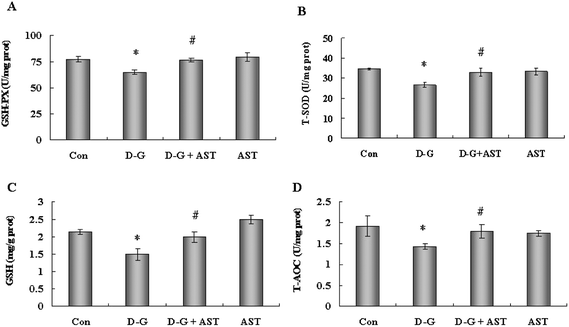 |
| Fig. 2 Effects of astaxanthin on the antioxidant capacity in the brains of SD rats. (A) The GSH-PX activity in the brains. (B) The SOD activity in the brains. (C) The GSH contents in the brains. (D) The T-AOC levels in the brains. Bars represent mean ± S.D. (n = 5). *p < 0.05 vs. Con group, #p < 0.05 vs. D-G group; one-way ANOVA. | |
3.5. Effects of AST on Bcl-2 and Bax expression in the brains of D-G-treated rats
The expression of the anti-apoptotic protein Bcl-2 and the pro-apoptotic protein Bax were measured by the Western blot method. As shown in Fig. 3A, the expression of Bcl-2 was decreased, but the expression of Bax was increased in the brains of the D-G group rats, as compared to control group. However, AST treatment upregulated the expression of Bcl-2 and reduced the expression of Bax in the brains of aging rats, as compared to the D-G group. The ratio of Bcl-2 to Bax has been reported to be correlated with apoptosis in cells. As shown in Fig. 3B, the ratio of Bcl-2/Bax in the D-G group was approximately reduced by 60% compared with the control group (p < 0.05), while AST administration approximately increased by 1-fold the ratio of Bcl-2/Bax compared with the D-G group (p < 0.05).
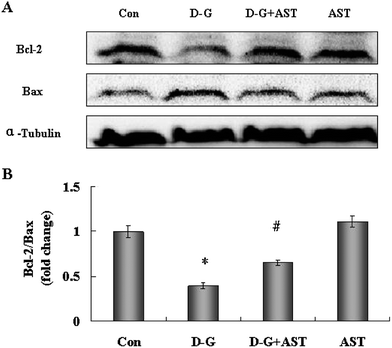 |
| Fig. 3 Influence of astaxanthin treatment on Bcl-2 and Bax expression in the brains of SD rats. (A) The expression of Bcl-2 and Bax in the brains of SD rats. (B) Quantification the ratio of Bcl-2/Bax by densitometric analysis. Bars represent mean ± S.D. (n = 5). *p < 0.05 vs. Con group, #p < 0.05 vs. D-G group, one-way ANOVA. | |
3.6. Effects of AST on COX-2 expression in the brains of D-G-treated rats
As shown in Fig. 4, the expression of COX-2 in the brains of the D-G group were higher than those in the control group (p < 0.05), while AST administration significantly decreased the expression of COX-2 in the brains of aging rats (p < 0.05).
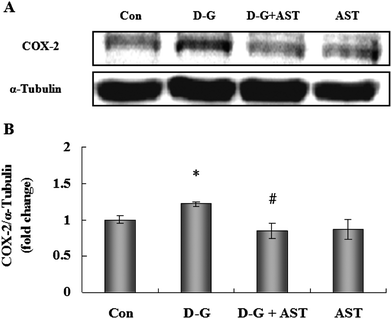 |
| Fig. 4 Influence of astaxanthin treatment on COX-2 expression in the brains of SD rats. (A) The expression of COX-2 in the brains of SD rats. (B) Quantification of COX-2 expression by densitometric analysis. Bars represent mean ± S.D. (n = 5). *p < 0.05 vs. Con group, #p < 0.05 vs. D-G group, one-way ANOVA. | |
3.7. Effects of AST on histopathological changes in the hippocampus
Fig. 5 is the representative photomicrographs of hematoxylin and eosin staining in the hippocampus of the rats. In the hippocampal cornu ammonis region 1 (CA1), cornu ammonis region 3 (CA3) and dentate gyrus region (DG) of the control group, neurons were normal, with well preserved cytoplasm, prominent nucleus and nucleolus (Fig. 5A, D and G). However, neuronal degeneration and nucleus shrinkage were easily observed as the arrow pointed in the hippocampal CA1, CA3 and DG regions of the D-G group (Fig. 5B, E and H). With long-term administration of AST, the neuronal injuries were markedly prevented (Fig. 5C, F and I).
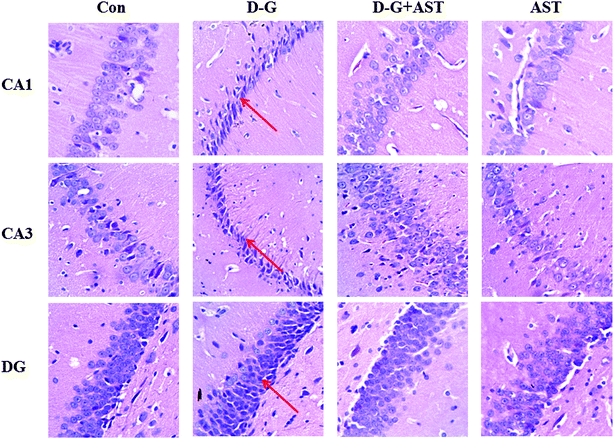 |
| Fig. 5 Effects of astaxanthin on histopathological changes in the hippocampus of SD rats revealed by hematoxylin and eosin (HE) staining (10 × 40). Upper: Histopathological changes in the hippocampal CA1 region. Middle: Histopathological changes in the hippocampal CA3 region. Lower: Histopathological changes in the hippocampal DG region. Neuronal degeneration and nucleus shrinkage were easily observed as the arrow pointed. CA1, cornu ammonis region 1; CA3, cornu ammonis region 3; DG, dentate gyrus. | |
3.8. Effects of AST on the expression of BDNF in the brains and hippocampus
As shown in Fig. 6A, the expression of BDNF in the brains of the D-G group were significantly lower than those in the control group (p < 0.05), whereas AST treatment restored BDNF expression in the brains of aging rats compared with the D-G group (p < 0.05). The expression of BDNF in the hippocampus was measured by an immunohistochemistry method. The changes of BDNF expression are obvious in the hippocampus and the results are shown in Fig. 6C. In the control group, a large amount of claybank dyes were dispersed in the hippocampus, indicating that BDNF expressed in high levels in the hippocampus of the control group. However, positive staining with the claybank dyes in the hippocampus of the D-G group was obviously decreased compared to the control group, which suggested that the expression of BDNF was decreased in the hippocampus of aging rats. In contrast, the positive staining with the claybank dyes observed in the hippocampus was obviously more than those observed in the D-G group and approached the levels of the control group, suggesting AST treatment restored the expression of BDNF in the hippocampus of aging rats.
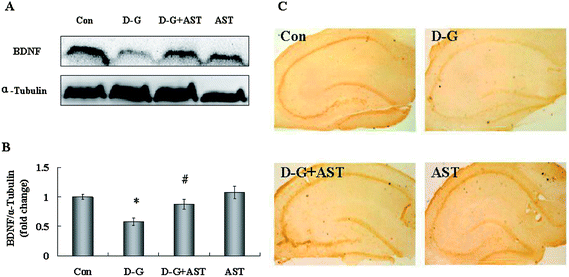 |
| Fig. 6 Influence of astaxanthin treatment on BDNF expression in the brains of SD rats. (A) The expression of BDNF in the brains. (B) Quantification of BDNF expression by densitometric analysis. (C) Influences of astaxanthin treatment on BDNF expression in the hippocampus of SD rats revealed by immunohistochemistry staining (10 × 4). Bars represent mean ± S.D. (n = 5). *p < 0.05 vs. Con group, #p < 0.05 vs. D-G group, one-way ANOVA. | |
4. Discussion
Aging is an inevitable process characterized by progressive impairment in physiological function. The brain is the most susceptible organ to aging and the aged brain may provide a sensitive environment for the development of neurodegenerative diseases such as Parkinson's disease and Alzheimer's disease. So it is extremely important to explore methods to retard or reverse brain aging.
AST is a red-orange carotenoid pigment and has exhibited potent antioxidative, anti-inflammatory, and neuroprotective effects. In the present study, we investigated whether supplementation with AST would alleviate brain aging in D-galactose-lesioned rats and its protective mechanisms.
D-G-lesioned rats are widely used as aging models for aging studies, as they can mimic many characters of the natural aging process, such as cognitive deficits and neurologic impairments.25–27
Accumulating evidence indicates that oxidative stress plays an important role in brain aging. It is well known that the brain is the most susceptible organ to oxidative damage.5 Thus, improving the antioxidant capacity of brain may be an important avenue for alleviating brain aging.
Oxidative stress always results in the oxidative damage of biomacromolecules, such as the lipids of cellular membranes, proteins, and DNA. MDA increase is an important indicator of lipid peroxidation, which is a well-known paradigm of the oxidative damage of membranes under conditions of oxidative stress.15 Protein carbonylation has been demonstrated to be a reliable marker of oxidative stress.28 Carbonylation causes major changes in protein structure and function, which results in the loss of cell viability. In addition, carbonylation brings about the formation of protease-resistant protein aggregates, which can mediate cell death.29 In nuclear and mitochondrial DNA, 8-OHdG is one of the predominant forms of free radical-induced oxidative lesions, and has therefore been widely used as a biomarker for oxidative stress.30 In the present study, D-galactose treatment caused notable oxidative damage including the increase in MDA, protein carbonylation and 8-OHdG levels in the brains of rats, which was similar to the previous reports.31–33 However, AST administration significantly decreased the levels of MDA, protein carbonylation and 8-OHdG in the brains of D-G induced aging rats, suggesting that AST can alleviate oxidative stress by reducing the oxidative damage in the brains of aging rats induced by D-G.
There are some antioxidants in vivo, which safely interact with free radicals and terminate the chain reactions before vital molecules are damaged. Antioxidants could be enzymatic or non-enzymatic, the former include GSH-PX and SOD, and the latter include GSH. The action of SOD is to protect tissues against harmful effects of superoxide free radicals. GSH-PX could specifically catalyse GSH to reduce hydroperoxide (H2O2) to H2O. Concordant with previous observations,34–37 D-G exposure reduced the GSH-PX and SOD activities, GSH contents and T-AOC levels in the brains. However, AST treatment restored the GSH-PX and SOD activities, GSH contents and T-AOC levels in the brains of aging rats induced by D-G, suggesting that AST can alleviate oxidative stress by enhancing the antioxidant capacity in the brains of aging rats induced by D-G.
Apoptosis has been implicated in various pathological conditions resulting in aging and is commonly used as an index of brain injury.38 Mitochondria play a pivotal role in apoptosis in both the intrinsic and the extrinsic pathways.39 The anti-apoptotic protein Bcl-2 and pro-apoptotic protein Bax, which reside in the upstream of mitochondria, have an important influence on apoptosis by regulating the release of several pro-apoptotic proteins into the cytosol, such as cytochrome c. Bcl-2 appears to inhibit the mitochondria depolarization and ROS production, while Bax induces mitochondria depolarization and ROS production.40 Therefore, the ratio of Bcl-2/Bax is a crucial factor in determining the progress of cell apoptosis.41 In the present study, the ratio of Bcl-2/Bax was decreased in the brains of aging rats induced by D-G, which is in agreement with previous reports.34,42 However, supplementation with AST restored the ratio of Bcl-2/Bax in brains as compared with the D-G group. The results suggested that AST may enhance the anti-apoptosis capacity via increasing the Bcl-2/Bax ratio in the brain of aging rats induced by D-G.
In addition, recent research strongly proves that the molecular inflammatory process plays a momentous role in the aging process and age-related diseases.7 Cyclooxygenase-2 (COX-2), the inducible isoform of cyclooxygenase, is primarily responsible for prostanoid production, which has potently pro-inflammatory and potentially pathogenic actions. In this study, in agreement with previous reports,7,43 the expression of COX-2 in the brains of aging rats was increased as compared with the control group, which was reversed by AST treatment. The results indicated that long-term supplementation with AST can relieve inflammation reaction, at least in part, by decreasing the expression of the pro-inflammatory mediator COX-2 in the brains of aging rats.
It is well known that the hippocampus plays an important role in the learning and memory process. The age-associated decline in memory is usually attributed to biochemical and structural modifications of the hippocampus, which is related to oxidative damage.42,44 Reports have demonstrated that D-galactose could induce neurodegeneration in the hippocampus.26,34 In this study, significant neurodegeneration in the hippocampus of D-G treated rats was observed. However, AST administration significantly ameliorated the histopathological changes in the hippocampus as compared to the D-G group. Thus, the results suggested that AST treatment could protect the hippocampus from neurodegeneration in aging rats induced by D-G.
BDNF is the most abundant neurotrophic factor in the brain, and mainly exists in the hippocampus and frontal cortex.45 BDNF has a vital effect on the genesis, survival, growth and synaptic plasticity of neurons in mammalian brains, and it can improve learning and memory capacity.46 In the present study, in agreement with previous reports,10,16 the expression of BDNF was decreased in both the brain and hippocampus of aging rats induced by D-G. However, AST supplementation enhanced the levels of BDNF in both the brain and hippocampus. The mechanism by which AST upregulates the expression of BDNF in the brains of aging rats deserves further investigation.
It has been reported that AST improved the reaction time, attention, and working memory of old men.47 Additionally, Zhang et al. reported that AST significantly improved the memory performance of mice in the Morris water maze.48 These studies suggest that AST can improve the learning and memory capacity in vivo. However, the mechanism by which astaxanthin could enhance cognitive functions is not clear. Based on previous research, we investigated the mechanism of in vivo astaxanthin improved learning and memory capacity. The results showed that astaxanthin significantly ameliorated the histopathological changes in the hippocampus and enhanced the expression of BDNF both in the brain and hippocampus of aging rats, which were beneficial to enhance cognitive functions. Therefore, our study provided a theoretical basis for the effect of astaxanthin to enhance cognitive functions.
In conclusion, our study demonstrated that AST significantly alleviated brain aging in D-G-induced rats. The protective effects may be mediated, at least partly, through reducing the oxidative damage, enhancing the capacity of the antioxidant system, restoring the ratio of Bcl-2/Bax, inhibiting the expression of pro-inflammatory factor COX-2, upregulating BDNF levels and ameliorating the hippocampal neurodegeneration. These results suggested that AST had a potential to be used as a novel nutrient for preventing brain aging and age-related neurodegenerative diseases.
Conflict of interest
The authors declare that there are no conflicts of interest.
Abbreviations
AST | Astaxanthin; |
D-G |
D-Galactose |
SD | Sprague-Dawley rats |
ROS | Reactive oxygen species |
GSH-PX | Glutathione peroxidase |
SOD | Superoxide dismutase |
GSH | Glutathione |
T-AOC | Total antioxidant capacity |
MDA | Malonaldehyde |
8-OHdG | 8-Hydroxy-2-deoxyguanosine |
COX-2 | Cyclooxygenase-2 |
BDNF | Brain derived neurotrophic factor |
CA1 | Cornu ammonis region 1 |
CA3 | Cornu ammonis region 3 |
DG | Dentate gyrus |
Acknowledgements
This work was supported by the Doctoral Program Foundation of Institutions of Higher Education of China (no.20090204120027).
References
- J. N. Keller, Age-related neuropathology, cognitive decline, and Alzheimer's disease, Ageing Res. Rev., 2006, 5, 1–13 CrossRef CAS PubMed.
- M. T. Lin and M. F. Beal, Mitochondrial dysfunction and oxidative stress in neurodegenerative diseases, Nature, 2006, 443, 787–795 CrossRef CAS PubMed.
- S. Middei, S. Daniele, A. Caprioli, O. Ghirardi and M. Ammassari-Teule, Progressive cognitive decline in a transgenic mouse model of Alzheimer's disease overexpressing mutant hAPPswe, Genes Brain Behav., 2006, 5, 249–256 CrossRef CAS PubMed.
- W. O. Opii, G. Joshi, E. Head, N. W. Milgram, B. A. Muggenburg, J. B. Klein, W. M. Pierce, C. W. Cotman and D. A. Butterfield, Proteomic identification of brain proteins in the canine model of human aging following a long-term treatment with antioxidants and a program of behavioral enrichment: relevance to Alzheimer's disease, Neurobiol. Aging, 2008, 29, 51–70 CrossRef CAS PubMed.
- R. J. Reiter, Oxidative processes and antioxidative defense mechanisms in the aging brain, FASEB J., 1995, 9, 526–533 CAS.
- M. E. Harper, L. Bevilacqua, K. Hagopian, R. Weindruch and J. J. Ramsey, Ageing, oxidative stress, and mitochondrial uncoupling, Acta Physiol. Scand., 2004, 182, 321–331 CrossRef CAS PubMed.
- H. Y. Chung, B. Sung, K. J. Jung, Y. Zou and B. P. Yu, The molecular inflammatory process in aging, Antioxid. Redox Signaling, 2006, 8, 572–581 CrossRef CAS PubMed.
- Y. Higami and I. Shimokawa, Apoptosis in the aging process, Cell Tissue Res., 2000, 301, 125–132 CrossRef CAS.
- C. Hock, K. Heese, C. Hulette, C. Rosenberg and U. Otten, Region-specific neurotrophin imbalances in Alzheimer disease: decreased levels of brain-derived neurotrophic factor and increased levels of nerve growth factor in hippocampus and cortical areas, Arch. Neurol., 2000, 57, 846–851 CrossRef CAS.
- L. Tapia-Arancibia, E. Aliaga, M. Silhol and S. Arancibia, New insights into brain BDNF function in normal aging and Alzheimer disease, Brain Res. Rev., 2008, 59, 201–220 CrossRef CAS PubMed.
- A. H. Nagahara, D. A. Merrill, G. Coppola, S. Tsukada, B. E. Schroeder, G. M. Shaked, L. Wang, A. Blesch, A. Kim, J. M. Conner, E. Rockenstein, M. V. Chao, E. H. Koo, D. Geschwind, E. Masliah, A. A. Chiba and M. H. Tuszynski, Neuroprotective effects of brain-derived neurotrophic factor in rodent and primate models of Alzheimer's disease, Nat. Med., 2009, 15, 331–337 CrossRef CAS PubMed.
- S. Peng, D. J. Garzon, M. Marchese, W. Klein, S. D. Ginsberg, B. M. Francis, H. T. Mount, E. J. Mufson, A. Salehi and M. Fahnestock, Decreased brain-derived neurotrophic factor depends on amyloid aggregation state in transgenic mouse models of Alzheimer's disease, J. Neurosci., 2009, 29, 9321–9329 CrossRef CAS PubMed.
- J. A. Joseph, B. Shukitt-Hale and G. Casadesus, Reversing the deleterious effects of aging on neuronal communication and behavior: beneficial properties of fruit polyphenolic compounds, Am. J. Clin. Nutr., 2005, 81, 313S–316S CAS.
- M. E. Obrenovich, N. G. Nair, A. Beyaz, G. Aliev and V. P. Reddy, The role of polyphenolic antioxidants in health, disease, and aging, Rejuvenation Res., 2010, 13, 631–643 CrossRef CAS PubMed.
- S. Z. Zhong, Q. H. Ge, R. Qu, Q. Li and S. P. Ma, Paeonol attenuates neurotoxicity and ameliorates cognitive impairment induced by D-galactose in ICR mice, J. Neurol. Sci., 2009, 277, 58–64 CrossRef CAS PubMed.
- F. Li, Q. H. Gong, Q. Wu, Y. F. Lu and J. S. Shi, Icariin isolated from Epimedium brevicornum Maxim attenuates learning and memory deficits induced by D-galactose in rats, Pharmacol. Biochem. Behav., 2010, 96, 301–305 CrossRef CAS PubMed.
- G. Hussein, H. Goto, S. Oda, U. Sankawa, K. Matsumoto and H. Watanabe, Antihypertensive potential and mechanism of action of astaxanthin: III. Antioxidant and histopathological effects in spontaneously hypertensive rats, Biol. Pharm. Bull., 2006, 29, 684–688 CAS.
- Y. Ikeda, S. Tsuji, A. Satoh, M. Ishikura, T. Shirasawa and T. Shimizu, Protective effects of astaxanthin on 6-hydroxydopamine-induced apoptosis in human neuroblastoma SH-SY5Y cells, J. Neurochem., 2008, 107, 1730–1740 CrossRef CAS PubMed.
- H. Shen, C. C. Kuo, J. Chou, A. Delvolve, S. N. Jackson, J. Post, A. S. Woods, B. J. Hoffer, Y. Wang and B. K. Harvey, Astaxanthin reduces ischemic brain injury in adult rats, FASEB J., 2009, 23, 1958–1968 CrossRef CAS PubMed.
- C. H. Chang, C. Y. Chen, J. Y. Chiou, R. Y. Peng and C. H. Peng, Astaxanthine secured apoptotic death of PC12 cells induced by beta-amyloid peptide 25–35: its molecular action targets, J. Med. Food, 2010, 13, 548–556 CrossRef CAS PubMed.
- X. Liu, T. Shibata, S. Hisaka and T. Osawa, Astaxanthin inhibits reactive oxygen species-mediated cellular toxicity in dopaminergic SH-SY5Y cells via mitochondria-targeted protective mechanism, Brain Res., 2009, 1254, 18–27 CrossRef CAS PubMed.
- W. Aoi, Y. Naito, K. Sakuma, M. Kuchide, H. Tokuda, T. Maoka, S. Toyokuni, S. Oka, M. Yasuhara and T. Yoshikawa, Astaxanthin limits exercise-induced skeletal and cardiac muscle damage in mice, Antioxid. Redox Signaling, 2003, 5, 139–144 CrossRef CAS PubMed.
- C. E. Robinson, A. Keshavarzian, D. S. Pasco, T. O. Frommel, D. H. Winship and E. W. Holmes, Determination of protein carbonyl groups by immunoblotting, Anal. Biochem., 1999, 266, 48–57 CrossRef CAS PubMed.
- D. Y. Yoo, W. Kim, C. H. Lee, B. N. Shin, S. M. Nam, J. H. Choi, M. H. Won, Y. S. Yoon and I. K. Hwang, Melatonin improves D-galactose-induced aging effects on behavior, neurogenesis, and lipid peroxidation in the mouse dentate gyrus via increasing pCREB expression, J. Pineal Res., 2012, 52, 21–28 CrossRef CAS PubMed.
- Q. Zhang, X. Li, X. Cui and P. Zuo,
D-Galactose injured neurogenesis in the hippocampus of adult mice, Neurol. Res., 2005, 27, 552–556 CrossRef CAS PubMed.
- X. Cui, P. Zuo, Q. Zhang, X. Li, Y. Hu, J. Long, L. Packer and J. Liu, Chronic systemic D-galactose exposure induces memory loss, neurodegeneration, and oxidative damage in mice: protective effects of R-alpha-lipoic acid, J. Neurosci. Res., 2006, 84, 647–654 CrossRef CAS PubMed.
- J. Lu, Y. L. Zheng, D. M. Wu, L. Luo, D. X. Sun and Q. Shan, Ursolic acid ameliorates cognition deficits and attenuates oxidative damage in the brain of senescent mice induced by D-galactose, Biochem. Pharmacol., 2007, 74, 1078–1090 CrossRef CAS PubMed.
- J. Greilberger, C. Koidl, M. Greilberger, M. Lamprecht, K. Schroecksnadel, F. Leblhuber, D. Fuchs and K. Oettl, Malondialdehyde, carbonyl proteins and albumin-disulphide as useful oxidative markers in mild cognitive impairment and Alzheimer's disease, Free Radical Res., 2008, 42, 633–638 CrossRef CAS PubMed.
- A. Dasgupta, J. Zheng, N. I. Perrone-Bizzozero and O. A. Bizzozero, Increased carbonylation, protein aggregation and apoptosis in the spinal cord of mice with experimental autoimmune encephalomyelitis, ASN Neuro, 2013, 5, e00111 CrossRef PubMed.
- A. Valavanidis, T. Vlachogianni and C. Fiotakis, 8-Hydroxy-2′-deoxyguanosine (8-OHdG): a critical biomarker of oxidative stress and carcinogenesis, J. Environ. Sci. Health, Part C: Environ. Carcinog. Ecotoxicol. Rev., 2009, 27, 120–139 CrossRef CAS PubMed.
- S. Aydin, K. Yanar, P. Atukeren, E. Dalo, M. E. Sitar, E. Uslu, N. Caf and U. Cakatay, Comparison of oxidative stress biomarkers in renal tissues of D-galactose induced, naturally aged and young rats, Biogerontology, 2012, 13, 251–260 CrossRef CAS PubMed.
- H. M. Hsieh, W. M. Wu and M. L. Hu, Soy isoflavones attenuate oxidative stress and improve parameters related to aging and Alzheimer's disease in C57BL/6J mice treated with D-galactose, Food Chem. Toxicol., 2009, 47, 625–632 CrossRef CAS PubMed.
- A. Kumar, A. Prakash and S. Dogra, Naringin alleviates cognitive impairment, mitochondrial dysfunction and oxidative stress induced by D-galactose in mice, Food Chem. Toxicol., 2010, 48, 626–632 CrossRef CAS PubMed.
- D. Zhang, G. Liu, J. Shi and J. Zhang,
Coeloglossum viride var. bracteatum extract attenuates D-galactose and NaNO2 induced memory impairment in mice, J. Ethnopharmacol., 2006, 104, 250–256 CrossRef PubMed.
- W. J. Li, S. P. Nie, X. P. Peng, X. Z. Liu, C. Li, Y. Chen, J. E. Li, W. R. Song and M. Y. Xie, Ganoderma atrum polysaccharide improves age-related oxidative stress and immune impairment in mice, J. Agric. Food Chem., 2012, 60, 1413–1418 CrossRef CAS PubMed.
- S. J. Tsai, C. P. Chiu, H. T. Yang and M. C. Yin, s-Allyl cysteine, s-ethyl cysteine, and s-propyl cysteine alleviate beta-amyloid, glycative, and oxidative injury in brain of mice treated by D-galactose, J. Agric. Food Chem., 2011, 59, 6319–6326 CrossRef CAS PubMed.
- L. Wu, Z. Huang, P. Qin, Y. Yao, X. Meng, J. Zou, K. Zhu and G. Ren, Chemical characterization of a procyanidin-rich extract from sorghum bran and its effect on oxidative stress and tumor inhibition in vivo, J. Agric. Food Chem., 2011, 59, 8609–8615 CrossRef CAS PubMed.
- C. Zhang and N. G. Bazan, Lipid-mediated cell signaling protects against injury and neurodegeneration, J. Nutr., 2010, 140, 858–863 CrossRef CAS PubMed.
- J. Sastre, F. V. Pallardo and J. Vina, Mitochondrial oxidative stress plays a key role in aging and apoptosis, IUBMB Life, 2000, 49, 427–435 CrossRef CAS PubMed.
- J. C. Martinou and R. J. Youle, Mitochondria in apoptosis: Bcl-2 family members and mitochondrial dynamics, Dev. Cell, 2011, 21, 92–101 CrossRef CAS PubMed.
- R. Raghupathi, Cell death mechanisms following traumatic brain injury, Brain Pathol., 2004, 14, 215–222 CrossRef.
- Z. Mao, Y. L. Zheng, Y. Q. Zhang, B. P. Han, X. W. Zhu, Q. Chang and X. B. Hu, The anti-apoptosis effects of daidzein in the brain of D-galactose treated mice, Molecules, 2007, 12, 1455–1470 CrossRef CAS PubMed.
- H. J. Kim, K. J. Jung, B. P. Yu, C. G. Cho, J. S. Choi and H. Y. Chung, Modulation of redox-sensitive transcription factors by calorie restriction during aging, Mech. Ageing Dev., 2002, 123, 1589–1595 CrossRef CAS.
- M. Gallagher and M. M. Nicolle, Animal models of normal aging: relationship between cognitive decline and markers in hippocampal circuitry, Behav. Brain Res., 1993, 57, 155–162 CrossRef CAS.
- J. M. Conner, J. C. Lauterborn, Q. Yan, C. M. Gall and S. Varon, Distribution of brain-derived neurotrophic factor (BDNF) protein and mRNA in the normal adult rat CNS: evidence for anterograde axonal transport, J. Neurosci., 1997, 17, 2295–2313 CAS.
- T. Numakawa, S. Suzuki, E. Kumamaru, N. Adachi, M. Richards and H. Kunugi, BDNF function and intracellular signaling in neurons, Histol. Histopathol., 2010, 25, 237–258 CAS.
- A. Satoh, S. Tsuji and Y. Okada, Preliminary clinical evaluation of toxicity and efficacy of a new astaxanthin-rich Haematococcus pluvialis extract, J. Clin. Biochem. Nutr., 2009, 44, 280–284 CrossRef CAS PubMed.
- X. Zhang, L. Pan, X. Wei, H. Gao and J. Liu, Impact of astaxanthin-enriched algal powder of Haematococcus pluvialis on memory improvement in BALB/c mice, Environ. Geochem. Health, 2007, 29, 483–489 CrossRef CAS PubMed.
|
This journal is © The Royal Society of Chemistry 2014 |
Click here to see how this site uses Cookies. View our privacy policy here.