DOI:
10.1039/C3FO60205B
(Paper)
Food Funct., 2014,
5, 85-91
Spontaneous supersaturation of calcium D-gluconate during isothermal dissolution of calcium L-lactate in aqueous sodium D-gluconate
Received
30th May 2013
, Accepted 29th October 2013
First published on 31st October 2013
Abstract
Continuing dissolution of solid calcium L-lactate pentahydrate in saturated aqueous solutions following addition of solid sodium D-gluconate corresponding to a gluconate/lactate ratio around three was found to result in homogeneous solutions supersaturated with calcium D-gluconate by a factor of seven, from which calcium D-gluconate monohydrate precipitated only slowly. In contrast, dissolution of calcium D-gluconate monohydrate by sodium L-lactate in aqueous solution with the reverse lactate/gluconate ratio also around three did not result in similar homogeneous solutions on the route to solid calcium L-lactate pentahydrate. This increasing supersaturation of calcium D-gluconate during dissolution of calcium L-lactate in aqueous sodium D-gluconate may enhance calcium bioavailability. The dissolution overshooting depends on competitive kinetics and is also of interest in modeling biomineralization and in designing novel food products with increased calcium bioavailability.
Introduction
Under conditions of temperature fluctuations, supersaturation is a common phenomenon in Nature and important for transport of salts of moderate aqueous solubility by endothermic dissolution.1 The degree of supersaturation traditionally defined as the ion product relative to the solubility product2 has further been found critical for structural match during biomineralization.3 Supersaturation may not only occur as a thermal effect but also as the result of changing chemical environments. Human mineral nutrition provides such important examples as salts of essential minerals present in food dissolved during digestion in the acid of the stomach, under the conditions of increasing pH in the intestines, become poorly soluble and are subjected to precipitation.4,5 The calcium content of food or total calcium intake are accordingly inappropriate parameters for the factual calcium uptake.6 The nutritive value of calcium and other essential minerals depends on the absorbability and capability to resist precipitation prior to absorption in the intestines from supersaturated solutions.7
Most calcium salts are only slightly soluble, but calcium(II) ions are made available for in vivo transport and a variety of biological functions through binding to organic molecules including specific amino acid motifs in peptides and proteins and to carbohydrate derivatives.8 Some of the carbohydrate derivatives like hydroxycarboxylates are naturally occurring fermentation products and may be important for calcium absorption in the intestines as they form supersaturated solutions from which calcium salts precipitate only slowly.9 Calcium L-lactate and calcium D-gluconate are organic salts commonly used as calcium supplements and in food and beverage fortification due to their moderate solubility and neutral taste.10 The use of salts of hydroxycarboxylates can also be of relevance in quality improvement of cheese products.11 The prevention of precipitation of calcium lactate crystals in some dairy products especially on the surface of cheeses is very important since such a process is undesirable and may lead to refusal of the final product by customers.12 Therefore, factors affecting solubility of calcium lactate have been extensively studied over a period of time.13,14 Increased solubility of calcium lactate in the presence of other ions like for instance sodium gluconate seems to be one of the solutions to prevent crystallization.11 It was proposed by the same authors that increased solubility of calcium lactate in the presence of sodium gluconate is due to formation of mixed calcium–lactate–gluconate complexes. In contrast, increased solubility for a similar system was found to be due to complex formation between each of the ligands and calcium(II) in the solutions.15 However, there is a lack of information on the solubility of calcium lactate and calcium gluconate in the presence of other potential ligands. Careful investigation of calcium salts of these sugar acids may help to develop better principles for food and beverage fortification. Special attention should be also paid to the unusual behavior of calcium salts of sugar acids like gluconic acid, which is known to remain in the supersaturated state for an unusually long time. Supersaturation of calcium lactate and calcium gluconate should accordingly be further investigated under conditions with mixtures of hydroxycarboxylates to provide a better picture of the mechanism behind the unusual supersaturation phenomenon.
While studying transformation of calcium salts in human digestion and the importance of such transformations for mineral nutrition, we have discovered a dissolution process wherein an aqueous solution becomes increasingly supersaturated up to a degree of seven. The isothermal dissolution forming a supersaturated solution is followed by a lag phase and subsequent precipitation to reach equilibrium solubility. We report herein details regarding this unusual phenomenon detected for calcium D-gluconate and include a discussion on a possible mechanism also of importance for biomineralization and for fortification of food and beverage products to increase calcium bioavailability. These results might also be of relevance in designing novel functional food products and contribute to the understanding of intestinal precipitation processes affecting calcium absorbability and consequently its bioavailability from calcium sources with different solubilities.
Experimental
Chemicals
CaCl2·2H2O, NaCl, NaOH, and P4O10 were all from Merck (Darmstadt, Germany). Calcium L-lactate pentahydrate, calcium D-gluconate monohydrate, sodium L-lactate (∼98%), D-gluconic acid sodium salt (≥99%), and ammonium purpurate 5,5-nitrilodibarbituric acid (murexide indicator) were from Sigma-Aldrich (Steinheim, Germany). Ethylenediamine-tetraacetic acid disodium salt dihydrate was from Sigma (St. Louis, MO, US). All aqueous solutions were made from water purified on a Milli-Q Plus train, Millipore Corporation (Bedford, MA, US).
Dissolution experiments
(i) CalciumL-lactate and sodiumD-gluconate. 15.00 g (0.049 mol) of calcium L-lactate pentahydrate was added to 100 ml of water and was equilibrated for two hours under constant magnetic stirring at 25.0 °C to form a saturated solution corresponding to 9.50 g (0.0307 mol) in solution and a precipitate of 5.50 g (0.018 mol). 65.0 g (0.298 mol) of solid sodium D-gluconate was now added to the saturated solution. During two hours of constant magnetic stirring at 25.0 °C, a clear solution appeared. This solution was divided into two, one was left undisturbed, while the other was continuously stirred, both thermostated at 25.0 °C. Both solutions were analyzed for total calcium content by EDTA titration and for free calcium ion concentration using a calcium ion-selective electrode after 2, 24, 48, 72, and 96 hours. The undisturbed solution was subjected to the same analysis also after 120, 144, 168, 192, and 216 hours.
(ii) CalciumD-gluconate and sodiumL-lactate. 10.87 g (0.024 mol) of calcium D-gluconate monohydrate was added to 50.0 ml of water and was equilibrated for two hours under constant magnetic stirring at 25.0 °C to form a saturated solution corresponding to 4.50 g (0.010 mol) in solution and a precipitate of 6.37 g (0.014 mol). 16.70 g (0.149 mol) of solid sodium L-lactate was now added to the saturated solution. During two hours of constant magnetic stirring at 25.0 °C some but not all solid phase dissolved. Filtered samples of the equilibrating solution were analyzed for total calcium content by EDTA titration and for free calcium ion concentration using a calcium ion-selective electrode after 2, 24, and 72 hours, while still being stirred.
Precipitation experiments
(i) A solution of calcium D-gluconate in water made approximately 25% supersaturated by heating, corresponding to 0.10 mol L−1 was observed for a 1/2 year period by frequent calcium analysis using EDTA titration.
(ii) A solution of calcium L-lactate in water made approximately 25% supersaturated by heating, corresponding to 0.37 mol L−1 was observed for 2 days by frequent calcium analysis using EDTA titration.
Precipitate collection
Precipitates were collected from the equilibrated solutions prepared as described above under Dissolution experiments after 24, 72 and 96 hours of stirring. Samples were filtered and precipitates air-dried overnight. Then 0.50 g or 0.25 g of the precipitate was dried in an oven at 105 °C until constant weight and dissolved in 50 ml or 25 ml of water, respectively. Total calcium in the precipitate was determined by EDTA titration.
Total calcium concentration
All samples were filtered (589/3, Whatman, Dassel, Germany) and titrated with EDTA (0.0500 mol L−1 standardized against 0.0200 mol L−1 of CaCl2) with murexide as indicator under alkaline conditions. 1.000 ml of calcium lactate/gluconate solution, and 500 mg or 250 mg of the precipitate were used.
Free calcium ion concentration
The calcium ion concentration [Ca2+] was measured at 25.0 °C in filtrates of solutions under equilibration with a calcium ion-selective electrode ISE25Ca and a reference REF 251 electrode (Radiometer, Copenhagen, Denmark) calibrated with standards of 0.000100, 0.00100, and 0.0100 mol L−1 Ca2+ in 1.0 mol L−1 NaCl for which a Nernst relationship has been demonstrated.7
X-ray diffraction
For precipitate identification, standards were prepared from calcium D-gluconate monohydrate and calcium L-lactate pentahydrate obtained from Sigma-Aldrich (Steinheim, Germany), which were recrystallized prior to X-ray analysis. Precipitates from dissolution experiments in the presence of sodium D-gluconate after 24 hours of stirring were collected from calcium L-lactate. X-ray diffraction (XRD) patterns were recorded using a Siemens D5000 diffractometer (Munich, Germany) applying monochromated Co-Kα (λ = 0.179021 nm) radiation and operated at 40 kV and 40 mA. Randomly oriented powder samples of calcium salt precipitates were scanned from 2° to 50° (2θ) using steps of 0.02° and a counting time of 3 s per step.
Boron analysis
The boron content was determined in the solutions from dissolution experiments after two hours of equilibration by inductively coupled plasma-optical emission spectroscopy (ICP-OES) using an ICP-OES spectrometer Optima 5300 DV (PerkinElmer, Waltham, MA, US). The samples were initially dried and homogenized followed by complete digestion in a microwave oven.
Light scattering measurement
Light scatter was measured in the solutions from dissolution experiments after two hours of equilibration using a laser light scattering system, BI-200SM (Brookhaven Instruments Corporation, Holtsville, NY, US).
Results
Calcium D-gluconate is less soluble in water than calcium L-lactate. For aqueous 1.0 mol L−1 NaCl at 25.0 °C the solubility product is 7.1 × 10−4 mol3 L−3 and for calcium L-lactate 5.8 × 10−3 mol3 L−3, when correcting for incomplete dissociation (L = lactate or gluconate):
Gluconate forms the calcium(II) complex CaL+ with an association constant K1 = 14 mol−1 L and lactate with K1 = 8 mol−1 L in aqueous 1.0 mol L−1 NaCl at 25.0 °C.15 The complex formation increases the solubility of calcium lactate in solutions of sodium gluconate and of calcium gluconate in solutions of sodium lactate, more significant for the less soluble calcium gluconate in the presence of lactate despite the weaker calcium lactate complexes formed compared to the gluconate complexes.15
The effect of complex formation could be clearly seen in the dissolution experiment, where a saturated aqueous solution of calcium lactate at 25 °C (9.5 g calcium lactate pentahydrate in 100 ml, corresponding to 0.307 mol L−1) with a solid phase of calcium lactate pentahydrate of 5.50 g was treated with 65.0 g solid sodium gluconate still at 25 °C. The solid phase disappeared and a completely transparent solution of 139 ml was formed in approximately 2 hours, see Fig. 1A–C, for which homogeneity was confirmed by light scattering measurements. This homogeneous solution with total calcium concentration analyzed to be 0.35 mol L−1 and free calcium ion concentration measured to be 0.0015 mol L−1 is supersaturated with calcium gluconate, since the ion product [Ca2+][Gl−]2 = 5.5 × 10−3 mol3 L−3 compared with the solubility product of 7.1 × 10−4 mol3 L−3 (total gluconate is 2.14 mol L−1, total lactate 0.70 mol L−1, with a [CaGl+]/[CaLact+] ratio calculated to be 1.9 from the association constants). The supersaturation is rather significant, and notably the high concentration of calcium gluconate is the result of a dissolution process by which the ion product becomes increasingly larger than the solubility product for calcium gluconate during dissolution of calcium lactate in aqueous sodium gluconate. The homogeneous solution supersaturated with calcium gluconate is clearly metastable as precipitation starts after a ten hour period when the solution is undisturbed, and faster depending on agitation or stirring, see Fig. 1D. The homogeneous solution is not supersaturated with respect to calcium lactate as [Ca2+][Lact−]2 = 4.9 × 10−4 mol3 L−3 compared with the solubility product of 5.8 × 10−3 mol3 L−3 and the precipitate slowly formed should accordingly be expected to be calcium gluconate.
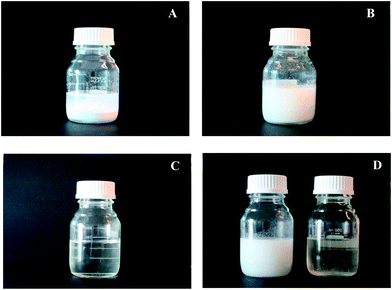 |
| Fig. 1 Spontaneous supersaturation during dissolution of calcium L-lactate pentahydrate in a saturated solution by added solid sodium D-gluconate: (A) Saturated solution of calcium L-lactate pentahydrate at 25.0 °C with excess of calcium L-lactate pentahydrate. (B) Solid excess of sodium D-gluconate added to the inhomogeneous saturated solution of calcium L-lactate pentahydrate. (C) Inhomogeneous solution of calcium L-lactate pentahydrate and added solid sodium D-gluconate after 2 hours of stirring at 25.0 °C becoming homogeneous. (D) Solution of calcium L-lactate pentahydrate and sodium D-gluconate left undisturbed at 25.0 °C for 24 hours with slow precipitation of calcium D-gluconate (to the right) and with stirring showing extensive precipitation (to the left). | |
The reverse experiment is less intriguing, since dissolution of excess calcium gluconate in a saturated solution with added solid sodium lactate does not form a homogeneous solution under conditions where the total lactate and gluconate concentrations are interchanged and are 2.14 mol L−1 and 0.70 mol L−1, respectively. In contrast, calcium gluconate does only dissolve partly with an increase in total calcium concentration due to complex formation prior to a decrease in total dissolved calcium caused by an onsetting precipitation.
The precipitation from the undisturbed supersaturated, homogeneous solution formed during dissolution of calcium lactate by gluconate was slow and could be described by a first-order reaction for disappearance of calcium in solution with a half-life of approximately 36 hours, see Fig. 2. The equilibrium concentration of total calcium was 0.084 ± 0.004 mol L−1 in the undisturbed solution and 0.070 ± 0.0010 mol L−1 in the stirred solutions. The free calcium ion concentration remained constant at 1.5 mmol L−1 during approximately the first half life of the precipitation reaction in the undisturbed solution and reached an equilibrium value of 0.35 ± 0.02 mmol L−1, which corresponds to an ion product of [Ca2+][Gl−]2 = 7.8 × 10−4 mol3 L−3, when correcting the concentration of gluconate for precipitation of calcium gluconate, a value close to the solubility product for calcium gluconate. The identity of the precipitate was further confirmed by a calcium analysis showing a calcium content of 8.5 ± 0.5% in the precipitate collected after 48 hours compared to 8.6% in calcium gluconate monohydrate and by the X-ray diffractogram of the precipitate in comparison with the diffractograms of authentic calcium lactate pentahydrate and calcium gluconate monohydrate, see Fig. 3.
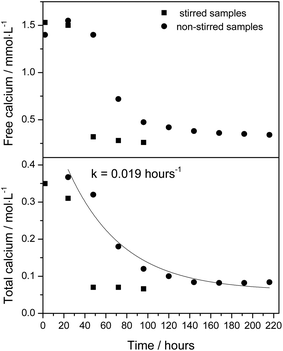 |
| Fig. 2 Lower panel: total calcium concentration in solutions of saturated calcium L-lactate with excess solid sodium D-gluconate added, spontaneously forming a homogeneous solution supersaturated with calcium D-gluconate during subsequent precipitation of calcium D-gluconate when left undisturbed (●) or with magnetic stirring (■) at 25.0 °C. Curve is an exponential fit to the concentration in the undisturbed solution. Upper panel: free calcium ion concentration (mmol L−1) measured in the same solutions. | |
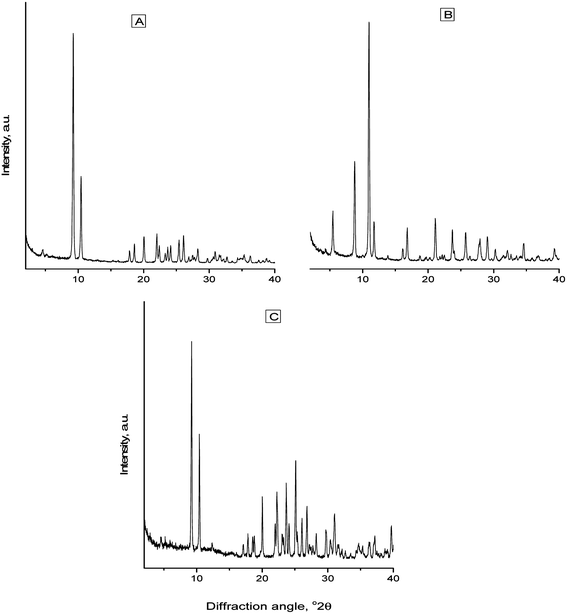 |
| Fig. 3 X-ray diffractograms of (A) calcium D-gluconate monohydrate, (B) calcium L-lactate pentahydrate, and (C) precipitate from a saturated calcium L-lactate solution with excess sodium D-gluconate added forming a homogeneous solution supersaturated with calcium D-gluconate, collected after 24 hours of equilibration. | |
The precipitation from the solution resulting from dissolution of calcium gluconate by lactate is more rapid, which is understandable since the solution is inhomogeneous throughout the dissolution of calcium gluconate. The total calcium ion concentration at equilibrium was analyzed to be 0.22 mol L−1 and the free calcium ion concentration was measured to have the value 1.0 mmol L−1, corresponding to an ion product of [Ca2+][Lact−]2 = 3.7 × 10−3 mol3 L−3 when correcting the lactate concentration for precipitation, in fair agreement with the solubility product of calcium lactate, Ksp = 5.8 × 10−3 mol3 L−3. Calcium analysis of the precipitate collected after 24 hours of precipitation gave a value of 12 ± 1% corresponding to that of calcium lactate pentahydrate (13.0% Ca).
Gluconate is known, like other vicinal dihydroxy compounds to react with boric acid and, solutions of “calcium borogluconate” have been used for injection in the calcium treatment of milk fever in cows due to the capacity of boric acid to form highly supersaturated solutions of calcium gluconate by heating.16 The solutions resulting from the two types of dissolution experiments had a boron content of 4.0 μmol L−1 and 5.5 μmol L−1, respectively, as analyzed by ICP compared with the total concentration of ∼3.0 mol L−1 of gluconate in the dissolution experiment with calcium lactate and 1.0 mol L−1 of gluconate in the dissolution experiment with calcium gluconate, respectively, excluding any interference from contamination with boron.
Discussion
Dissolution of calcium lactate in an already saturated aqueous solution of calcium lactate is, in the presence of sodium gluconate, driven by complex formation between calcium(II) and gluconate to form the CaGl+ ion. Similarly the dissolution of calcium gluconate in an already saturated solution of calcium gluconate in the presence of sodium lactate is driven by the formation of the lactate complex CaLact+. It was confirmed that the dissolution of calcium hydroxycarboxylates in the presence of sodium hydroxycarboxylates is driven by complex formation without forming mixed complexes between calcium and ligands in the solution.15 The difference between the two dissolution experiments lies in the capability of gluconate to dissolve calcium lactate resulting in calcium ion concentrations, which in the gluconate solution clearly exceed the solubility product of calcium gluconate producing homogeneous solutions highly supersaturated with calcium gluconate. In contrast, lactate will only dissolve calcium gluconate to the limit determined by the solubility product of calcium lactate not producing homogeneous solution supersaturated with calcium lactate.
Solubility equilibria like the two under consideration depend on the rate of dissolution and the rate of precipitation. For calcium lactate dissolution
| CaLact2(s) → CaLact+ + Lact− | (3) |
the primary dissolution reaction is, in the presence of gluconate enhanced by
| CaLact+ + Gl− → CaGl+ + Lact− | (4) |
for which the equilibrium constant is
K4 = 1.75. The reaction of
eqn (4) was, as a ligand exchange in a closed shell metal ion, fast under the present conditions with [Gl
−] > [Lact
−], while the precipitation
| CaGl+ + Gl− → CaGl2(s) | (5) |
was concluded to be slow considering the slow rate observed for crystallization of calcium gluconate from supersaturated solutions. Crystal growth of calcium gluconate was found to be under surface reaction control and follow a simple power law.
9
For calcium gluconate dissolution
| CaGl2(s) → CaGl+ + Gl− | (6) |
the primary dissolution reaction will in the presence of lactate to a lesser degree be followed by ligand exchange
| CaGl+ + Lact− → CaLact+ + Gl− | (7) |
since the equilibrium constant is
K7 = 0.57. Precipitation of calcium lactate
| CaLact+ + Lact− → CaLact2(s) | (8) |
is less studied but is faster than calcium gluconate.
14,17
Precipitation of calcium lactate and calcium gluconate from aqueous solutions of moderate supersaturation, formed by heating, was followed by calcium analysis, see Fig. 4. Precipitation seems to be approximately 40 times faster for calcium lactate than for calcium gluconate. An important difference between dissolution of calcium lactate in sodium gluconate and dissolution of calcium gluconate in sodium lactate is accordingly that complex binding by gluconate promotes dissolution of calcium lactate more than complex binding by lactate promotes dissolution of calcium gluconate. Precipitation of calcium gluconate will at the same time be far slower than the precipitation of calcium lactate. Combined the two effects will increase the dissolution rate of calcium lactate to form the calcium gluconate complex which will only slowly precipitate, but decrease the dissolution rate of calcium gluconate to form the calcium lactate complex which more rapidly precipitates. Calcium lactate dissolving fast in aqueous sodium gluconate will accordingly increase the calcium ion concentration, while at the same time precipitation of calcium gluconate is hampered in effect resulting in supersaturation. Calcium gluconate will dissolve more slowly in aqueous sodium lactate, and the slow dissolution will be followed by fast precipitation of calcium lactate preventing formation of a homogeneous solution.
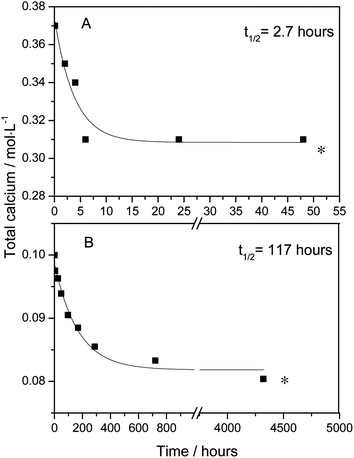 |
| Fig. 4 Precipitation at 25 °C of solid from approximately 25% supersaturated aqueous solutions of (A) calcium L-lactate and (B) calcium D-gluconate. * Indicates equilibrium solubility. Half-life for precipitate is based on an exponential fit. | |
Gluconate forms stronger complexes with calcium than lactate and is at the same time the least soluble salt. The strong complex formation makes the dissolution of calcium lactate in aqueous sodium gluconate faster than the subsequent precipitation of calcium gluconate. For calcium gluconate dissolution in aqueous sodium lactate, the weaker calcium binding hampers rate increase of dissolution allowing the subsequent precipitation fully to compensate as illustrated in Fig. 5. Other combinations of anions of biological importance may match these requirements for slow precipitation of a mineral salt of the stronger complex forming anion for increasing mineral mobility through isothermal formation of supersaturated solutions resulting from dissolution overshooting. Such phenomena may also explain the apparent paradox, that a high intake of calcium lowers the risk of kidney stone formation independent of the source of calcium.18
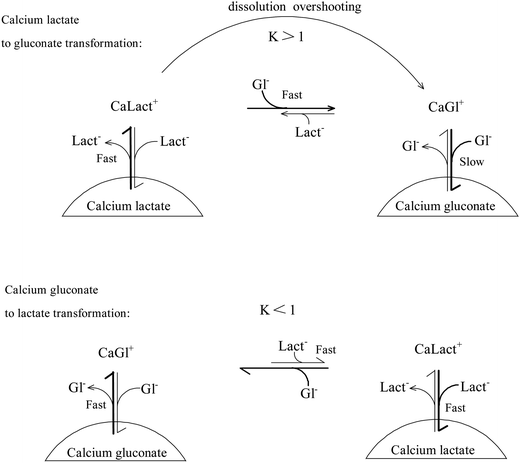 |
| Fig. 5 Isothermal calcium lactate transformation to calcium gluconate through the formation of a supersaturated calcium gluconate solution as a result of fast conversion to a calcium gluconate complex, which only slowly precipitates as calcium gluconate. For transformation of calcium gluconate to calcium lactate, the faster precipitation of calcium lactate and weaker calcium lactate complex formation prevent this dissolution overshooting. | |
Conclusion
The increasing supersaturation of calcium D-gluconate during isothermal dissolution of calcium L-lactate in aqueous sodium D-gluconate is an unprecedented example of dissolution of a salt apparently violating thermodynamics. The kinetic explanation offered for this novel observation may, however, inspire the formulation of calcium fortified foods and beverages with better calcium bioavailability. Balancing the formation of the spontaneous supersaturation, the presence of the metastable state, and precipitation are important for enhancing intestinal absorption of calcium from supplements of moderate solubility with a consequent increase of calcium bioavailability. Furthermore, biomineralization processes can benefit from this unusual phenomenon as not only spontaneous supersaturation but also the ligand exchange might be of importance.
Acknowledgements
This work was supported by the Danish Dairy Research Foundation and the Danish Council for Independent Research|Technology and Production Sciences (FTP).
References
- A. E. S. Van Driessche, L. G. Benning, J. D. Rodriguez-Blanco, M. Ossorio, P. Bots and J. M. Garcia-Ruiz, The Role and Implications of Bassanite as a Stable Precursor Phase to Gypsum Precipitation, Science, 2012, 336(6077), 69–72 CrossRef CAS PubMed.
- V. K. Lamer and R. H. Dinegar, The Limiting Degrees of Supersaturation of the Sparingly Soluble Sulfates, J. Am. Chem. Soc., 1951, 73(1), 380–385 CrossRef CAS.
- X. Y. Liu and S. W. Lim, Templating and supersaturation-driven anti-templating: Principles of biomineral architecture, J. Am. Chem. Soc., 2003, 125(4), 888–895 CrossRef CAS PubMed.
- C. Y. C. Pak and L. V. Avioli, Factors Affecting Absorbability of Calcium from Calcium Salts and Food, Calcif. Tissue Int., 1988, 43(2), 55–60 CrossRef CAS.
- R. P. Heaney, R. R. Recker and C. M. Weaver, Absorbability of Calcium Sources – the Limited Role of Solubility, Calcif. Tissue Int., 1990, 46(5), 300–304 CrossRef CAS.
- C. Hansen, E. Werner, H. J. Erbes, V. Larrat and J. P. Kaltwasser, Intestinal calcium absorption from different calcium preparations: Influence of anion and solubility, Osteoporosis Int., 1996, 6(5), 386–393 CrossRef CAS.
- M. Vavrusova, R. Raitio, V. Orlien and L. H. Skibsted, Calcium hydroxy palmitate: possible precursor phase in calcium precipitation by palmitate, Food Chem., 2013, 138, 2415–2420 CrossRef CAS PubMed.
- Y. B. Zhou, S. H. Xue and J. J. Yang, Calciomics: integrative studies of Ca2+-binding proteins and their interactomes in biological systems, Metallomics, 2013, 5(1), 29–42 RSC.
- J. Bao, K. Koumatsu, Y. Arimatsu, K. Furumoto, M. Yoshimoto, K. Fukunaga and K. Nakao, A kinetic study on crystallization of calcium gluconate in external loop airlift column and stirred tank for an immobilized glucose oxidase reaction with crystallization, Biochem. Eng. J., 2003, 15(3), 177–184 CrossRef CAS.
- K. Rafferty, G. Walters and R. P. Heaney, Calcium fortificants: Overview and strategies for improving calcium nutriture of the US population, J. Food Sci., 2007, 72(9), R152–R158 CrossRef CAS PubMed.
- C. Phadungath and L. E. Metzger, Effect of sodium gluconate on the solubility of calcium lactate, J. Dairy Sci., 2011, 94(10), 4843–4849 CrossRef CAS PubMed.
- N. Kubantseva and R. W. Hartel, Solubility of calcium lactate in aqueous solution, Food Rev. Int., 2002, 18(2–3), 135–149 CrossRef CAS PubMed.
- K. N. Pearce, L. K. Creamer and J. Gilles, Calcium lactate deposits on rindless Cheddar cheese, N. Z. J. Dairy Sci. Technol., 1973, 8, 3–7 CAS.
- N. Kubantseva, R. W. Hartel and P. A. Swearingen, Factors affecting solubility of calcium lactate in aqueous solutions, J. Dairy Sci., 2004, 87(4), 863–867 CrossRef CAS.
- M. Vavrusova, B. M. Munk and L. H. Skibsted, Aqueous solubility of calcium L-lactate, calcium D-gluconate, and calcium D-lactobionate. Importance of complex formation for solubility increase by hydroxycarboxylate mixtures, J. Agric. Food Chem., 2013, 61, 8207–8214 CrossRef CAS PubMed.
- H. T. Macpherson and J. Stewart, Investigations on the nature of calcium borogluconate, Biochem. J., 1938, 32, 76–78 CAS.
- X. Cao, H. J. Lee, H. S. Yun and Y. M. Koo, Solubilities of calcium and zinc lactate in water and water-ethanol mixture, Korean J. Chem. Eng., 2001, 18(1), 133–135 CrossRef CAS.
- E. N. Taylor and G. C. Curhan, Dietary calcium from dairy and non-dairy sources and risk of symptomatic kidney stones, J. Urol., 2013, 190, 1255–1259 CrossRef CAS PubMed.
|
This journal is © The Royal Society of Chemistry 2014 |
Click here to see how this site uses Cookies. View our privacy policy here.