Effect of cerium oxide nanoparticles on asparagus lettuce cultured in an agar medium†
Received
15th February 2014
, Accepted 11th June 2014
First published on 12th June 2014
Abstract
The increasing chances of manufactured nanoparticles (NPs) being released into the environment highlight the importance of understanding their interactions with plants, which are the basis of ecosystems. In this study, the phytotoxicity of CeO2 NPs on asparagus lettuce was assessed. Lettuce seeds were treated with CeO2 NPs in a plant agar medium at a wide range of concentrations (0–2000 mg L−1) for 5 days. At high concentrations (≥500 mg L−1), CeO2 NPs altered the activity of superoxide dismutase (SOD), induced lipid peroxidation and cell membrane damage, and inhibited the root growth. The results of X-ray absorption near edge fine structure (XANES) indicate that part of the CeO2 NPs were transformed from Ce(IV) to Ce(III) in the roots. The released Ce3+ may account for the phytotoxicity of CeO2 NPs.
Nano impact
Cerium oxide nanoparticles are a widely used nanomaterial with many important applications, and their interactions with ecosystems are inevitable. We previously found CeO2 NPs inhibited root elongation of Lactuca plants in aqueous suspensions. This study for the first time evaluates the phytotoxicity of CeO2 NPs in a plant agar medium, which provides a soil-like environment while avoiding the uncertainties intrinsic to natural ecosystems. Compared to deionized water, the bioavailability of CeO2 NPs in the agar medium was reduced, but the sensitivity of asparagus lettuce to CeO2 NPs was increased. This study implies the phytotoxicity of CeO2 NPs was affected by the exposure medium employed.
|
Introduction
Engineered nanoparticles (NPs) are widely used in cosmetics, electronics, optical devices, medicine, chemical catalysis, etc. The unique properties of these materials might present adverse effects to both organisms and the environment. Plants as the basic components of the ecosystem are easily exposed to NPs in atmospheric, terrestrial and aquatic environments. Moreover, NPs that enter into plants may be transferred to herbivorous consumers and further cause potential effects to human beings.1 Accordingly, the interactions between NPs and plants are of particular concern.2
Cerium dioxide nanoparticles (CeO2 NPs), as one of the most important manufactured nanoparticles, have extensive commercial applications.3,4 Previous studies have demonstrated that CeO2 NPs could be taken up by plant roots and transported to the aerial parts including the edible tissues.5,6 The interactions between CeO2 NPs and plants are complex. Enhancing, inhibitive and no effects of NPs on plant growth at different developmental stages have been documented. Ma et al. found that CeO2 NPs inhibited root elongation of lettuce, but showed no toxicity to rape, radish, wheat, cabbage, tomato and cucumber.7 The results of Wang et al. demonstrated that low concentrations of CeO2 NPs (≤10 mg L−1) enhanced plant growth and fruit yield of tomato plants.8 However, a further study indicated that second generation tomato seedlings grown from seeds collected from treated parent plants with CeO2 NPs accumulated much smaller biomass and were somewhat weaker than seedlings grown from seeds from untreated parent plants.9 López-Moreno and colleagues found that CeO2 NPs reduced corn, tomato and cucumber germination.5 The root growth was promoted by CeO2 NPs in cucumber and corn but reduced in alfalfa and tomato. These authors later reported that CeO2 NPs could compromise the quality of rice, diminish plant growth and yield of soybean and shut down nitrogen fixation.10 CeO2 NPs are generally recognized as stable in biological or environmental systems and remain unaltered after uptake by plant roots.5,6 However, we recently proved that CeO2 NPs can be transformed to CePO4 and cerium carboxylates in hydroponic plants with the assistance of biogenic reducing substances and organic acids.11 The high sensitivity of Lactuca plants to the released Ce3+ results in the species-specific toxicity of CeO2 NPs mentioned above.7,12 Biotransformation of CeO2 NPs to Ce(III) species in soil cultured plants was also reported recently.13
The above mentioned studies on the phytotoxicity of CeO2 NPs were carried out under either hydroponic or soil culture conditions. Due to their small size and surface properties, CeO2 NPs tend to aggregate and precipitate in aquatic solutions. It is almost impossible to maintain a constant exposure concentration of CeO2 NPs in nutrient solutions. Soil is a complex system of minerals, organic material, water, gasses, and living organisms. All these components can interact with NPs. It is difficult to carry out mechanism studies on interactions between plants and NPs in real soil environments. Recently, plant agar media have been introduced in phytotoxicity studies of NPs to optimize dispersion and prevent aggregation.14 Moreover, the viscous soft gels resemble soil more than H2O does, and thus allow the interactions between plants and NPs to be studied in a soil-like environment while avoiding the uncertainties intrinsic to natural ecosystems, which can affect both the rhizosphere and the properties of the NPs.2
In the present study, asparagus lettuce was used to assess the phytotoxicity of CeO2 NPs. Representative parameters such as root/shoot lengths, antioxidant enzyme activities, hydrogen peroxide content, lipid peroxidation and ion leakage were investigated to understand the plant's defence and response to abiotic stress caused by CeO2 NPs. To explore the proximate mechanisms of the observed toxicity, contents and chemical species of Ce in asparagus lettuce were analyzed and the effect of Ce3+ on root elongation of asparagus lettuce was assessed.
Experimental methods
Synthesis and characterization of CeO2 NPs
CeO2 NPs used in this study were synthesized by a precipitation method.6 Particle size and morphology were determined using a transmission electron microscope (TEM, JEM 200CX, Japan). X-ray diffraction (XRD, X'pert PRO MPD, Holland) was used to determine their crystal forms. A CeO2 NP suspension (100 mg L−1) in deionized water was prepared for measurement of hydrodynamic sizes and zeta potential (Nicomp 380 ZLS Zeta potential/Particle system, Santa Barbara, CA, USA). Chemical purity of the particles was analyzed using an inductively coupled plasma-mass spectrometer (ICP-MS, Thermo X7, USA).
Plant culture and nanoparticle application
Seeds of asparagus lettuce (Lactuca sativa Linn. var. angustata Irish ex Bremer) were purchased from the Chinese Academy of Agricultural Sciences. The plant assays were conducted in an agar medium. Each test unit contained 30 mL of 0.5% agar along with a specific concentration of NPs. CeO2 NP stock solutions in deionized water were ultraviolet sterilized and ultrasonically dispersed for 15 min before use. Autoclaved agar medium (120 °C for 2 h) was thoroughly mixed with or without the NPs to obtain a series of CeO2 suspensions (0, 2, 20, 200, 500, 1000, 2000 mg L−1). After an ultrasonic treatment at 60 °C for 15 min, the CeO2 NP suspensions were poured into 90 mm × 18 mm Petri dishes and quick-frozen in a −20 °C freezer to avoid aggregation and precipitation. To test the homogeneity of the distribution of CeO2 NPs, 10 agar samples were taken from 5 different points of the agar medium containing 200 mg L−1 CeO2 in a Petri dish (5 from upper parts and 5 from lower parts, as shown in Fig. S1†). Contents of Ce in the samples were analyzed by ICP-MS.
Uniform asparagus lettuce seeds were selected and sterilized by 10% NaClO solution for 5 min and washed 3 times with deionized water. After being soaked in water for two hours, fourteen seeds were inserted onto the surface of the agar medium. Four replicates were set for each treatment. All the Petri dishes were covered and sealed with parafilm, and placed in the dark at 20 °C in a climate incubator. After 5 days of treatment, the germination was halted. Germination rates were calculated. Root and shoot lengths were measured with a meter ruler.
Ce content determination
Shoots and roots were separated from the thoroughly washed seedlings and lyophilized to constant weights in a freeze dryer. The dried tissues were digested on a heating plate using a mixture of concentrated plasma-pure HNO3 and H2O2 (v/v: 4
:
1). Ce contents were analyzed by ICP-MS. A standard reference material (bush branches and leaves, GBW07602) was also digested to examine the recovery. Indium (20 ng mL−1) was used as an internal standard to compensate for matrix suppression and signal drifting. Analytical runs concluded the obtained residual solution, spike recovery samples, and calibration verification samples. Recovery from GBW07602 was 99% and spike recovery averaged 102%. Relative standard deviation was 2.5%. Detection limit was 0.01 ng ml−1.
Stress response of asparagus lettuce to CeO2 NPs
After 5 days of treatment, the seedlings were thoroughly washed with flowing tap water then deionized water to remove the agar medium. The roots were excised and homogenized with PBS (50 mM, pH 7.8) in an ice bath. Then the extracts were centrifuged for 10 min at 4 °C and 10
000 × g. The supernatants were preserved for SOD, POD activities and MDA contents analyses, using the assay kits purchased from Nanjing Jiancheng Bioengineering Institute (Nanjing, China).
H2O2 contents in the roots were examined by the method previously described by Begum and Ikhtiari.15 Fresh roots from the control and CeO2 treated plants were excised and immersed in 0.1 mg L−1 diaminobenzidine (DAB, pH 3.2) for 35 min in the dark at room temperature. Then the root segments were washed thoroughly followed by grinding in a mixture of 2 M KOH and DMSO at a ratio of 1
:
1.167 (v/v). Subsequently, the extracts were centrifuged at 10
000 × g for 5 min and the absorbance of the supernatants at 700 nm was measured on a Multimode Microplate Reader (SpectraMax M2, USA).
Ion leakage from roots was measured by a conductivity method based on the procedure of Lutts et al. with slight modifications.16 The conductivity of deionized water (Cw) was first measured. The 5 day-old root samples were immersed in test tubes containing 2 mL of deionized water after three washes with deionized water to remove external contamination. Test tubes were covered and incubated on a shaker (100 rpm) at room temperature (25 °C) for 1 h. The electrolyte conductivity of the solution (C0) was measured. Samples were then boiled at 100 °C for 15 min, and the conductivity of the solution (Ct) was measured after cooling. Electrolyte leakage (EL) was defined as: EL (%) = (Ct − Cw)/(C0 − Cw) × 100%.
Microscopy observations
After 5 days of treatment, fresh roots from the control and the sample treated with 2000 mg L−1 were observed using a light microscope (LM) to examine the morphology and cell division state of the root tips. Samples for the LM were prepared following standard procedures.17
Speciation analysis by X-ray absorption near edge fine structure spectroscopy (XANES)
Seedlings treated with 2000 mg L−1 CeO2 NPs were thoroughly washed. Roots were separated, lyophilized, ground into powder and pressed into slices (10 mm × 2 mm) for the XANES analysis. CeL3-edge (5723 eV) spectra were collected in the fluorescence mode. Measurements were performed at the beamline 1W1B at Beijing Synchrotron Radiation Facility. The ring storage energy of the synchrotron radiation accelerator in the data acquisition was 2.5 GeV with current intensity of 50 mA. CePO4, Ce(CH3COO)3, Ce2(C2O4)3 and CeO2 NPs were used as standard compounds and analyzed in transmission mode. Normalization and linear combination fitting (LCF) of the XANES spectra was accomplished by the Athena software.
Impact of Ce3+ ions on root growth
A series of Ce(NO3)3 solutions (0.005, 0.01, 0.05, 0.1, 0.5, 1 and 5 mg L−1) in 0.5% agar culture media were prepared to test the sensitivity of asparagus lettuce to Ce3+ ions. After 5 days of treatment, root lengths were measured by a meter rule.
Statistical analysis
The data processing was performed on Statistical Packages for the Social Sciences (SPSS) 17.0. One-Way ANOVA followed by Tukey's HSD or Bonferroni test to examine the statistical differences. All errors are expressed as standard deviations (SD). P < 0.05 was considered to be a significant difference.
Results
Physicochemical characteristics of CeO2 NPs
The TEM image shows that the NPs present as a truncated octahedral shape with an average size of 7.1 ± 0.4 nm (Fig. 1). Hydrodynamic size was 99.3 ± 2.0 nm and the NPs were well dispersed with a positive zeta-potential (47.4 mV). Hydrodynamic size reflects the interaction between initial particles and the solvent. There is a hydration layer capping on the particle surface. Herein, CeO2 NPs were aggregated in water and capped by a hydration layer. Therefore, the hydrodynamic size is larger than the TEM size. The XRD spectrum of the ceria NPs exhibits the cubic fluorite structure. Chemical purity of the NPs was 99.98%. The distribution of CeO2 NPs in the agar medium was homogeneous (ESI,† Fig. S2).
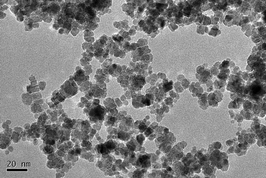 |
| Fig. 1 TEM image of CeO2 NPs. | |
Ce contents
As shown in Fig. 2, Ce contents in the roots were much higher than that in the shoots and the contents increased with the increasing of the exposure concentrations.
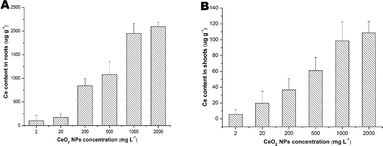 |
| Fig. 2 Ce contents in the roots (A) and shoots (B) of asparagus lettuce treated with CeO2 NPs for 5 days. The means are averaged from 4 replicates of asparagus lettuce. The values are given as mean ± SD (standard deviation). Significant differences versus control are marked with * (p < 0.05). | |
Growth analysis
CeO2 NPs had no significant effects on seed germination (ESI,† Fig. S3) and shoot growth (Fig. 3B) of asparagus lettuce at all exposure concentrations. But CeO2 NPs inhibited the root elongation at concentrations higher than 500 mg L−1 (Fig. 3A).
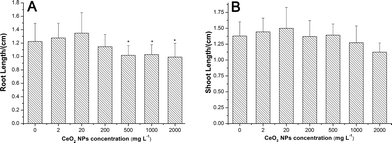 |
| Fig. 3 Root lengths (A) and shoot lengths (B) of asparagus lettuce cultured in agar amended with different concentrations of CeO2 NPs for 5 days. The means are averaged from 4 replicates with 14 seeds of asparagus lettuce. The values are given as mean ± SD (standard deviation). Significant differences versus control are marked with * (p < 0.05). | |
Stress responses of asparagus lettuce to CeO2 NPs
As shown in Fig. 4, there was no significant change of H2O2 accumulation at all exposure concentrations.
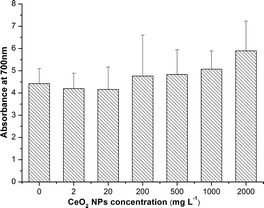 |
| Fig. 4 H2O2 content in roots of asparagus lettuce treated with CeO2 NPs for 5 days. The means are averaged from 4 replicates with 14 seeds of asparagus lettuce. The values are given as mean ± SD (standard deviation). | |
SOD activities were significantly down-regulated at high concentrations (500, 1000 and 2000 mg L−1) compared to the control (Fig. 5A). However, POD activities were only slightly enhanced at all exposure concentrations except 2000 mg L−1 (Fig. 5B). Significant increases of MDA levels (Fig. 5C) and leakage of electrolytes (Fig. 5D) were observed at high concentrations (500, 1000, 2000 mg L−1) of CeO2 NPs treatment, which indicate the membrane damage of the root cells.
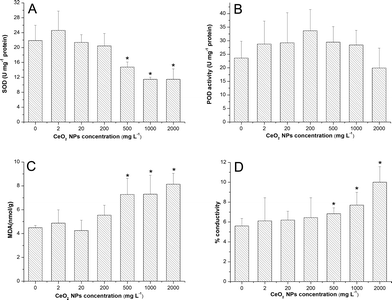 |
| Fig. 5 SOD (A), POD (B) activities, MDA contents (C) and ion leakage (D) in roots of asparagus lettuce treated with CeO2 NPs for 5 days. The means are averaged from 4 replicates of asparagus lettuce. The values are given as mean ± SD (standard deviation). Significant differences versus control are marked with * (p < 0.05). | |
Morphological change of root structure
At 500 mg L−1 and higher concentrations, the roots of asparagus lettuce were swollen and twist, along with brownish lesions. As can be seen from the LM photograph of the root tips, the region close to the end of the root swelled (Fig. 6). Meanwhile, the cell population in the meristem zone of the treatment group was far less than control.
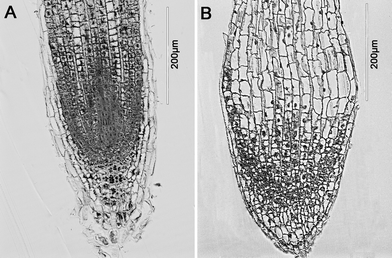 |
| Fig. 6 Light microscopic images of roots of asparagus lettuce after 5 days exposure. (A) control, (B) exposure with 2000 mg L−1 CeO2 NPs. | |
Chemical species of Ce in the roots
Normalized Ce LIII XANES spectra of model compounds and roots of asparagus lettuce are shown in Fig. 7. Peak A in the spectra represents the characteristic peak of Ce(II), which comes from CePO4, Ce(CH3COO)3 and Ce2(C2O4)3 here. Double peaks B and C indicate the characteristic peak of Ce(IV), which come from CeO2 in this study.18 Compared with spectra of the standard references, the spectra from roots of treated asparagus lettuce obviously showed the mixed features of peaks A, B and C. These indicate that Ce in roots presents a mixed oxidation state of Ce(IV) and Ce(III). To obtain the quantitative information of Ce species in the roots, LCF was performed on the normalized spectra of samples using CeO2, CePO4, Ce(CH3COO)3 and Ce2(C2O4)3 as the standard compounds. The fitted lines and fitting parameters indicate that the results are satisfying and convincing (ESI,† Fig. S4). In the root samples, the Ce species presented as 78.3% CeO2 and 21.7% Ce carboxylates.
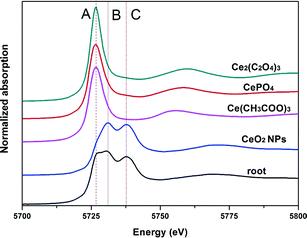 |
| Fig. 7 XANES Ce LIII-edge spectra (5723 eV) of standard references and roots of asparagus lettuce treated with 2000 mg L−1 CeO2 NPs for 5 days. Vertical dashed line and dotted lines mark the features of Ce(III) and Ce(IV) compounds, respectively. | |
Effects of Ce3+ on root elongation
As shown in Fig. 8, root elongation of lettuce was inhibited by Ce3+ ions at all exposure concentrations even as low as 0.005 mg L−1.
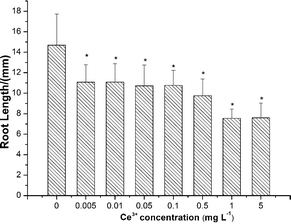 |
| Fig. 8 Root lengths of asparagus lettuce treated with Ce3+ for 5 days. The means are averaged from 4 replicates of asparagus lettuce. The values are given as mean ± SD (standard deviation). Significant differences versus control are marked with * (p < 0.05). | |
Discussion
Phytotoxicity of NPs is influenced by various factors such as physicochemical properties of NPs, plant growth stage and properties of culture media. It has been demonstrated that exposure medium has an important effect on phytotoxicity of NPs.19 Agar is a commonly used culture medium for plants. It is semisolid and more close to a realistic environment for plant growth. Agar media can avoid aggregation of NPs and distribute NPs evenly in test units.20 But the soft gels restrict NP transport within the polysaccharide structure, and thus hinder NP bioavailability.2 In the agar medium, Ce contents in both the roots and shoots of asparagus lettuce treated with CeO2 NPs for 5 days were much lower than those cultured in CeO2 NP aqueous suspensions. For example, at the same exposure concentration of 2000 mg L−1, Ce contents in the roots and shoots of asparagus lettuce treated with CeO2 NPs in the agar medium were 2090 μg g−1 and 110 μg g−1. While in the aqueous suspension, Ce contents were 36
220 μg g−1 and 1210 μg g−1, respectively.12 This was further confirmed by the TEM images of the root sections (ESI,† Fig. S5). In deionized water, a large quantity of CeO2 NPs distributed on the root epidermis. However, in agar medium, no CeO2 NPs can be found.
Although the bioavailability of CeO2 NPs was limited, it seems that asparagus lettuce was more sensitive to the toxic effects of CeO2 NPs in the agar medium than in distilled water (ESI,† Fig. S6). It is generally accepted that the toxicity of NPs may be caused by excess production of reactive oxygen species (ROS).21 ROS are normal products of plant cell functions, including free radicals such as superoxide anion (O2˙−), hydroxyl radical (˙OH), as well as hydrogen peroxide (H2O2), and so forth, and a delicate balance between their generation and removal must be strictly controlled to prevent oxidative stress.22 Excessive production of ROS may cause lipid peroxidation, further promoting oxidative stress.23 Malondialdehyde (MDA), which forms during peroxidation of unsaturated fatty acids and is indicative of lipid peroxide, was determined as a function of NP treatments. In the present study, with the increases in CeO2 NP concentrations, SOD activities exhibited a decreasing tendency. Meanwhile, the significant increases of MDA and ionic conductance levels at high concentrations suggest that the production of excess ROS had not been cleared timely. Membrane systems of the root cells were damaged, and ultimately the permeability of the cell membrane was enhanced. Further, the changed osmotic pressure induced cell water loss and plasmolysis. The reduced root elongation and increased root diameters at high concentrations of CeO2 NPs probably resulted from retarded cell division and increased cell width, along with more cortical cell columns in the case of CeO2 NPs. These symptoms were similar to that of cucumber plants’ exposure to bulk Yb2O3 (ref. 24) and the thick root syndrome in cucumber induced by ethylene.25
Biotransformation determines the ultimate fate and toxicity of manufactured nanoparticles in living organisms. The release of heavy metal ions may play a key role in phytotoxicity of metal-based NPs.2 Plants can produce reducing substances such as phenols and sugars which will bring about redox reaction with the aid of biogenic organic acids.11,24 We previously demonstrated that Ce3+ released from CeO2 NPs could induce species-specific toxicity to Lactuca plants.12 In aqueous suspensions, about 6% of CeO2 was reduced to cerium(III) carboxylates in lettuce roots after 5 days treatment. Now in this study, at the same exposure concentration (2000 mg L−1), we found more than 20% of total Ce in the roots was transformed to Ce(III). Moreover, asparagus lettuce cultured in the agar medium showed a higher sensitivity to Ce3+ than those cultured in deionized water.12 It is reasonable to postulate that the phytotoxicity of CeO2 NPs observed in the present study was also attributed to the released Ce3+.
As a conclusion, we evaluated the phytotoxicity of CeO2 NPs in a plant agar medium, which provides a soil-like environment while avoiding the uncertainties intrinsic to natural ecosystems for the first time. CeO2 NPs showed a higher toxicity to asparagus lettuce in the agar medium than in aqueous suspensions. The phytotoxicity of CeO2 NPs was probably attributed to the released Ce3+ in the roots.
Acknowledgements
This work was financially supported by the Ministry of Science and Technology of China (grant no. 2011CB933400, 2013CB932703), Ministry of Environmental Protection of China (grant no. 201209012), and National Natural Science Foundation of China (grant no. 11275215, 11275218 and 11375009).
Notes and references
- J. D. Judy, J. M. Unrine and P. M. Bertsch, Evidence for biomagnification of gold nanoparticles within a terrestrial food chain, Environ. Sci. Technol., 2011, 45(2), 776–781 CrossRef CAS PubMed.
- P. Miralles, T. L. Church and A. T. Harris, Toxicity, Uptake, and Translocation of Engineered Nanomaterials in Vascular plants, Environ. Sci. Technol., 2012, 46(17), 9224–9239 CrossRef CAS PubMed.
- X. He, H. F. Zhang, Y. H. Ma, W. Bai, Z. Y. Zhang, K. Lu, Y. Y. Ding, Y. L. Zhao and Z. F. Chai, Lung deposition and extrapulmonary translocation of nano-ceria after intratracheal instillation, Nanotechnology, 2010, 21(28), 285103 CrossRef PubMed.
- M. T. Tseng, X. Lu, X. Duan, S. S. Hardas, R. Sultana, P. Wu, J. M. Unrine, U. Graham, D. A. Butterfield, E. A. Grulke and R. A. Yokel, Alteration of hepatic structure and oxidative stress induced by intravenous nanoceria, Toxicol. Appl. Pharmacol., 2012, 260(2), 173–182 CrossRef CAS PubMed.
- M. L. López-Moreno, G. de la Rosa, J. A. Hernández-Viezcas, J. R. Peralta-Videa and J. L. Gardea-Torresdey, X-ray absorption spectroscopy (XAS) corroboration of the uptake and storage of CeO2 nanoparticles and assessment of their differential toxicity in four edible plant species, J. Agric. Food Chem., 2010, 58(6), 3689–3693 CrossRef PubMed.
- Z. Y. Zhang, X. He, H. F. Zhang, Y. H. Ma, P. Zhang, Y. Y. Ding and Y. L. Zhao, Uptake and distribution of ceria nanoparticles in cucumber plants, Metallomics, 2011, 3(8), 816–822 RSC.
- Y. H. Ma, L. L. Kuang, X. He, W. Bai, Y. Y. Ding, Z. Y. Zhang, Y. L. Zhao and Z. F. Chai, Effects of rare earth oxide nanoparticles on root elongation of plants, Chemosphere, 2010, 78(3), 273–279 CrossRef CAS PubMed.
- Q. Wang, X. M. Ma, W. Zhang, H. C. Pei and Y. S. Chen, The impact of cerium oxide nanoparticles on tomato (Solanum lycopersicum L.) and its implications for food safety, Metallomics, 2012, 4(10), 1105–1112 RSC.
- Q. Wang, S. D. Ebbs, Y. S. Chen and X. M. Ma, Trans-generational impact of cerium oxide nanoparticles on tomato plants, Metallomics, 2013, 5(6), 753–759 RSC.
- C. M. Rico, M. I. Morales, A. C. Barrios, R. McCreary, J. Hong, W. Y. Lee, J. Nunez, J. R. Peralta-Videa and J. L. Gardea-Torresdey, Effect of cerium oxide nanoparticles on the quality of rice (Oryza sativa L.) grains, J. Agric. Food Chem., 2013, 61(47), 11278–11285 CrossRef CAS PubMed.
- P. Zhang, Y. H. Ma, Z. Y. Zhang, X. He, J. Zhang, Z. Guo, R. Z. Tai, Y. L. Zhao and Z. F. Chai, Biotransformation of Ceria Nanoparticles in Cucumber Plants, ACS Nano, 2012, 6(11), 9943–9950 CrossRef CAS PubMed.
- P. Zhang, Y. Ma, Z. Zhang, X. He, Y. Li, J. Zhang, L. Zheng and Y. Zhao, Species-specific toxicity of ceria nanoparticles to Lactuca plants, Nanotoxicology DOI:10.3109/17435390.2013.855829.
- J. A. Hernandez-Viezcas, H. Castillo-Michel, J. C. Andrews, M. Cotte, C. Rico, J. R. Peralta-Videa, Y. Ge, J. H. Priester, P. A. Holden and J. L. Gardea-Torresdey, In situ synchrotron X-ray fluorescence mapping and speciation of CeO(2) and ZnO nanoparticles in soil cultivated soybean (Glycine max), ACS Nano, 2013, 7(2), 1415–1423 CrossRef CAS PubMed.
- C. W. Lee, S. Mahendra, K. Zodrow, D. Li, Y. C. Tsai, J. Braam and P. J. Alvarez, Developmental phytotoxicity of metal oxide nanoparticles to Arabidopsis thaliana, Environ. Toxicol. Chem., 2010, 29(3), 669–675 CrossRef CAS PubMed.
- P. Begum, R. Ikhtiari and B. Fugetsu, Graphene phytotoxicity in the seedling stage of cabbage, tomato, red spinach, and lettuce, Carbon, 2011, 49(12), 3907–3919 CrossRef CAS PubMed.
- S. Lutts, J. Kinet and J. Bouharmont, NaCl-induced senescence in leaves of rice (Oryza sativa L.) cultivars differing in salinity resistance, Ann. Bot., 1996, 78(3), 389–398 CrossRef CAS.
-
J. J. Bozzola and L. D. Russell, Electron microscopy: principles and techniques for biologists, Jones & Bartlett Learning, 1999 Search PubMed.
- A. Bianconi, A. Marcelli, H. Dexpert, R. Karnatak, A. Kotani, T. Jo and J. Petiau, Specific intermediate-valence state of insulating 4f compounds detected by L3 x-ray absorption, Phys. Rev. B: Condens. Matter Mater. Phys., 1987, 35(2), 806 CrossRef CAS.
- W. M. Lee, J. I. Kwak and Y. J. An, Effect of silver nanoparticles in crop plants Phaseolus radiatus and Sorghum bicolor: media effect on phytotoxicity, Chemosphere, 2012, 86(5), 491–499 CrossRef CAS PubMed.
- W. M. Lee, Y. J. An, H. Yoon and H. S. Kweon, Toxicity and bioavailability of copper nanoparticles to the terrestrial plants mung bean (Phaseolus radiatus) and wheat (Triticum aestivum): Plant agar test for water-insoluble nanoparticles, Environ. Toxicol. Chem., 2008, 27(9), 1915–1921 CrossRef CAS.
- S. Sharifi, S. Behzadi, S. Laurent, M. L. Forrest, P. Stroeve and M. Mahmoudi, Toxicity of nanomaterials, Chem. Soc. Rev., 2012, 41(6), 2323–2343 RSC.
- O. Blokhina and K. V. Fagerstedt, Reactive oxygen species and nitric oxide in plant mitochondria: origin and redundant regulatory systems, Physiol. Plant., 2010, 138(4), 447–462 CrossRef CAS PubMed.
- P. Sharma, A. B. Jha, R. S. Dubey and M. Pessarakli, Reactive oxygen species, oxidative damage, and antioxidative defense mechanism in plants under stressful conditions, J. Bot., 2012, 2012, 217037 Search PubMed.
- P. Zhang, Y. H. Ma, Z. Y. Zhang, X. He, Z. Guo, R. Z. Tai, Y. Y. Ding, Y. L. Zhao and Z. F. Chai, Comparative toxicity of nanoparticulate/bulk Yb2O3 and YbCl3 to cucumber (Cucumis sativus), Environ. Sci. Technol., 2012, 46(3), 1834–1841 CrossRef CAS PubMed.
- R. Pierik, W. Verkerke and E. J. Visser, Thick root syndrome in cucumber (Cucumis sativus L.): a description of the phenomenon and an investigation of the role of ethylene, Ann. Bot., 1999, 84(6), 755–762 CrossRef CAS.
Footnotes |
† Electronic supplementary information (ESI) available. See DOI: 10.1039/c4en00025k |
‡ These authors contributed equally. |
|
This journal is © The Royal Society of Chemistry 2014 |