DOI:
10.1039/C4DT03074E
(Paper)
Dalton Trans., 2015,
44, 955-965
Synthesis, structure and reactivity study of magnesium amidinato complexes derived from carbodiimides and N,N′-bis(2,6-diisopropylphenyl)-1,4-diaza-butadiene ligands†
Received
6th October 2014
, Accepted 26th October 2014
First published on 4th November 2014
Abstract
We report an amidinato ligand-supported series of magnesium complexes obtained from the insertion of a magnesium–carbon bond into a carbon–nitrogen double bond of different carbodiimides and α-diimine ligands. The magnesium complexes [Mg(CH2Ph){CyN
C(CH2Ph)NCy}]2 (1), [Mg(CH2Ph){iPrN
C(CH2Ph)NiPr}]2 (2) and the homoleptic [Mg{tBuN
C(CH2Ph)NtBu}2] (3) (Cy = cyclohexyl, iPr = isopropyl, tBu = tert-butyl) were prepared by the reaction of dibenzyl magnesium [Mg(CH2Ph)2(Et2O)2] with the respective carbodiimides either in 1
:
1 or 1
:
2 molar ratio in toluene. The analogous reaction of [Mg(CH2Ph)2(Et2O)2] with the N,N′-bis(2,6-diisopropylphenyl)-1,4-diaza-1,3-butadiene (Dipp2DAD) ligand afforded the corresponding homoleptic magnesium complex [Mg{DippN
C(CH2Ph)CH2NDipp}2] (4) (Dipp = 2,6 diisopropylphenyl) in good yield. The solid-state structures of magnesium complexes 1–4 were confirmed by single-crystal X-ray diffraction analysis. It was observed that in each case, a magnesium–carbon bond was inserted into the carbon–nitrogen double bond of either carbodiimides or Dipp2DAD resulting in a monoanionic amido–imino ligand. In a further reaction between 1 and N-aryliminopyrrolyl ligand 2-(2,6-iPr2C6H3N
CH)C4H3NH (ImpDipp-H) in 1
:
2 molar ratio, a new magnesium complex [Mg(ImpDipp)2{CyN
C(CH2Ph)NHCy}] (5), with one amidinato and two aryliminopyrrolyl ligands in the coordination sphere, was obtained in good yield. In contrast, the homoleptic magnesium complex 4 reacted with one equivalent of N-aryliminopyrrolyl ligand (ImpDipp-H) to produce another mixed ligated magnesium complex [Mg{DippN
C(CH2Ph)CH2NDipp}(ImpDipp)] (6), with a benzylated DAD ligand and aryliminopyrrolyl ligands in the coordination sphere. Further reaction of complex 4 with benzyl alcohol (PhCH2OH) afforded the third mixed ligated magnesium complex [Mg{DippN
C(CH2Ph)CH2NDipp}(OCH2Ph)2] (7) in very good yield. The magnesium complexes 5–7 were characterised using standard analytical/spectroscopic techniques and their solid-state structures were established by single-crystal X-ray diffraction analysis.
Introduction
Even though organomagnesium halides of the type RMgX were discovered in 1900 by Victor Grignard, the relevance of these compounds is significant even today in organic and organometallic synthesis.1 Such ‘Grignard reagents’, which can still be accessed in high yield from the reaction between elemental magnesium and organic halides RX in the presence of ethereal solvents,2 have proved to be extremely powerful synthetic tools because of their high reactivity; they allow the nucleophilic introduction of organic groups as carbanion equivalents and for that reason, they belong to the standard repertoires of both organic and organometallic synthesis. Heteroleptic Lewis-acidic magnesium alkyl complexes have also been found to be promising catalysts for several polymerisation reactions.3–5 The importance of organomagnesium complexes led to extensive investigations of their molecular structures, both in solution and solid state, as well as their physical and chemical properties. Several review articles6–8 have described the structural principles of organomagnesium derivatives. Magnesium dialkyls are highly reactive towards diimines, comprehensively showing different reaction pathways depending on the steric bulk of the alkyl ligands and functional groups integrated into the diimine backbone. Magnesium complexes with diimine ligands such as 2,8-bis(tetramethylguanidino)naphthalene, 1,2-bis(tetra-methyl-guanidino)benzene via neutral coordination have been known.9 Bi-radical methyl bridged magnesium complexes such as [(α-diimine−˙)Mg+(μ-CH3)]2 obtained from single electron transfer (SET), with a radical anion in its coordination sphere, have also been recently reported by Bailey et al.10 In addition, more fascinating C alkylated and N alkylated magnesium complexes have also been studied using Lewis acid of p-block (AlMe3) or d-block (ZnMe2) metal alkyl compounds. In most cases, the alkyl groups migrated from the metal centre to iminic proligands to generate in situ new mono-anionic imido–amido ancillary ligands.11–14
Although some earlier reports are available,15 recently Anwander and co-workers exploited various alkyl migrations in the permethylated magnesium complex Mg(AlMe4)2 with the diimine ligand PhCH
NCH2CH2N
CHPh and dimethyl magnesium (MgMe2) with PhCH
NCH2CH2N
CHPh, yielding heteroleptic magnesium methyl complexes supported by an imino–amido ligand formed via alkyl migration.16 We also recently reported a group 4 metal–nitrogen bond inserted into a carbon–nitrogen double bond of carbodiimides and α-diimines to afford guanidinate and amido–imino ligand supported group 4 metal complexes, respectively.17 The ancillary monoanionic guanidinate ligands were formed by the migration of –NMe2 from a transition metal to the carbodiimide backbones. However, analogous reactions of carbodiimides with group 2 metal alkyls have not been reported so far. Thus, we were interested in exploring the potential of different carbodiimides as imino–amido ligands in magnesium alkyl chemistry.
In this context, the synthetic and structural details of magnesium complexes having molecular formulae [Mg(CH2Ph){CyN
C(CH2Ph)NCy}]2 (1), [Mg(CH2Ph)-{iPrN
C(CH2Ph)NiPr}]2 (2), and homoleptic [Mg{tBuN
C–(CH2Ph)NtBu}2] (3) (Cy = cyclohexyl, iPr = isopropyl, tBu = tert-butyl) and [Mg{DippN
C(CH2Ph)CH2NDipp}2] (4) are presented. We also report the preparation and molecular structures of three mixed ligand magnesium complexes, [Mg(ImpDipp)2−{CyN
C(CH2Ph)NHCy}] (5), [Mg{DippN
C–(CH2Ph)CH2NDipp}(ImpDipp)] (6) and [Mg{DippN
C(CH2Ph)–CH2NDipp}(OCH2Ph)2] (7).
Results and discussion
Magnesium amidinato complexes
The magnesium amidinato complexes of compositions [Mg(CH2Ph)–{CyN
C(CH2Ph)NCy}]2 (1) and [Mg(CH2Ph)–{iPrN
C(CH2Ph)NiPr}]2 (2) were synthesised by the reaction between magnesium dibenzyl etherate complex [Mg(CH2Ph)2(Et2O)2] and either N,N′-dicyclohexylcarbodiimide (for 1) or N,N‘-diisopropylcarbodiimide (for 2) at 60 °C (Scheme 1). Both complexes were obtained as pure forms in good yield through re-crystallisation from hot toluene. The analogous reaction of [Mg(CH2Ph)2(Et2O)2] with the more bulky N,N′-di-tert-butylcarbodiimide afforded the corresponding homoleptic magnesium complex [Mg{tBuN
C(CH2Ph)NtBu}2] (3) (Scheme 2). The new magnesium complexes 1–3 were characterised using standard analytical and spectroscopic techniques, and the solid-state structures of all three magnesium complexes were established by single-crystal X-ray diffraction analysis.
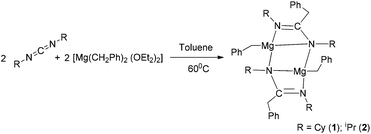 |
| Scheme 1 Synthesis of amidinato magnesium complexes 1 and 2. | |
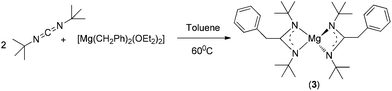 |
| Scheme 2 Synthesis of amidinato magnesium complex 3. | |
The 1H NMR spectra measured in C6D6 of complexes 1 and 2 were similar and exhibited only one set of signals in solution. Each of complexes 1 and 2 showed a sharp singlet at δ 1.72 and 1.89 ppm, respectively, for the resonance of two benzylic protons bound to a magnesium ion. These observations are in agreement with the other magnesium benzyl complexes [(PhCH2)2Mg(THF)2] (δ 1.9 ppm), [(TMEDA)Mg(CH2Ph)2] (δ 1.33 ppm), [{(THF)(CH2Ph)Mg(μ-Me)}2] (δ 1.88 ppm) and [HC{C(CH3)NDipp}2−Mg(CH2Ph)(THF)] (1.6 ppm) reported by Bailey et al.18 The singlet resonance that appeared at δ 3.70 (for complex 1) and 3.40 ppm (for complex 2) can be assigned to benzylic protons attached to the carbon atom of amidinato ligands. The multiplets for cyclohexyl moieties attached to the nitrogen atoms were in the expected region for complex 1. For complex 2, multiplets at δ 3.52–3.47 ppm can be assigned to two non-equivalent isopropyl-CH protons which are not well resolved on the NMR time scale. In addition, two doublets were observed at 1.13 ppm and 1.10 ppm for the chemically non-equivalent isopropyl methyl protons –CHMe2. In 13C{1H} NMR spectra of complexes 1 and 2, the signals at δ 173.7 and 173.5 ppm respectively indicate the presence of sp2 carbon (–N–C(CH2Ph)
N–) in the solution. In the 1H NMR spectra of homoleptic magnesium complex 3, the sharp singlet at 3.71 ppm corresponds to the resonance of four benzylic protons of –CH2Ph groups attached to the amidinato ligand backbone whereas the other sharp singlet at δ 1.01 ppm can be assigned to the 36 methyl protons CH3 present in four tert-butyl groups. We also observed a resonance signal at δ 173.5 ppm for (–N–C(CH2Ph)
N–) carbon in the 13C{1H} NMR spectra of 3 which corresponds to those for complexes 1 and 2.
1: Mg1–N1 2.1012(16), Mg1–N2 2.2054(14), Mg1–C19 2.1399(17), Mg1–N2i 2.1420(14), N1–C1 1.3125(18), N2–C1 1.3753(18), N1–C7 1.4749(17), N2–C13 1.4831(18), C1–C28 1.515(2), N1–Mg1–C19 123.96(6), N1–Mg1–N2i 107.10(5), C19–Mg1–N2i 122.68(6), N1–Mg1–N2 63.35(5), C19–Mg1–N2 126.99(6), N2i–Mg1–N2 96.77(5), C1–N1–Mg1 91.81(9), C7–N1–Mg1 143.29(10), C1–N2–C13 118.37(11), C1–N2–Mg1i 117.02(9), C13–N2–Mg1i 113.28(9), C1–N2–Mg1 85.81(9), C13–N2–Mg1 133.84(9), Mg1i–N2–Mg1 83.23(5), N1–C1–N2 114.70(12), C1–N1–C7 121.14(12).
2: Mg1–N1 2.0980(10), Mg1–N2 2.1922(10), Mg1–N2i 2.1395(11), Mg1–C15 2.1509(13), N1–C1 1.3079(13), N2–C1 1.3815(13), N2–Mg1i 2.1395(11), C1–C8 1.5201(13), N1–Mg1–N2 63.69(3), N1–Mg1–N2i 107.67(4), N1–Mg1–C15 125.41(5), N2i–Mg1–C15 121.59(4), N2i–Mg1–N2 94.09(4), C15–Mg1–N2 128.44(5), C1–N1–Mg1 93.12(6), C5–N1–Mg1 142.88(7), C1–N2–Mg1i 114.15(7), C2–N2–Mg1i 114.19(6), C1–N2–Mg1 87.14(6), Mg1i–N2–Mg1 85.91(4). N1–C1–N2 114.72(8), N1–C1–C8 123.98(9), N2–C1–C8 121.27(9).
Although there is ongoing interest in magnesium organometallics19 and particularly in the amido and amidinato-metal chemistry of magnesium,20,21 complexes 1–3 represent a series of various heteroleptic and homoleptic magnesium complexes containing amidinato ligands derived from three different carbodiimides. Therefore, their molecular structures in the solid state were determined by X-ray diffraction analysis. Complexes 1 and 2 crystallised in the monoclinic space group P21/c, having two and four molecules of 1 and 2 respectively in their unit cells. In contrast, complex 3 crystallised in the orthorhombic space group Pbca, having eight individual molecules in the unit cell. The details of their structural parameters are given in Table TS1 in the ESI.† The solid-state structures of complexes 1 and 2 are shown in Fig. 1a and 1b, respectively, and Fig. 2 represents the molecular structure of complex 3. Both the centro-symmetric complexes 1 and 2 are heteroleptic and dimeric in nature. In each case, one of the benzyl groups (CH2Ph) of [Mg(CH2Ph)2(Et2O)2] underwent nucleophilic addition at the carbodiimide sp carbon atom to generate a monoanionic amidinato ligand [RN
C(CH2Ph)NR]− [R = Cy (1) and iPr (2)] moiety. Such migration reactions are known in the literature.14 The coordination polyhedra for complexes 1 and 2 are formed by the newly formed amidinato ligand moiety through the coordination of imine and amido nitrogen atoms and the benzyl group. In the molecular structures of complexes 1 and 2 two kinds of Mg–N distances [2.101(16) and 2.205(14) Å for complex 1 and 2.098(10) and 2.192(10) Å for complex 2] were observed, indicating one short (from amido nitrogen) and one long (from imine nitrogen) attachment to the magnesium atom.20g These observations were also reflected in the C–N bond distances within the ligand moiety (C1–N1 1.313(18) and C1–N2 1.375(18) Å for complex 1 and C1–N1 1.308 (13) and N2–C1 1.382(13) Å for 2). Thus, the short–long bonds within the ligand [RN
C(CH2Ph)NR]− indicate the modified nature of the carbodiimide ligand (RN
C
NR) where identical C–N bonds were observed. The imine nitrogen atom was chelated to the second magnesium atom making the overall structure a dimer. The interactions between sp2 carbon atoms and the magnesium atom could be ignored as the bond distances (2.512 Å for complex 1 and 2.532 Å for complex 2) are larger. In each case three four-membered metallacycles N1–C1–N2–Mg1, Mg1–N2–Mg1i–N2i and N1i–C1i–N2i–Mg1i were formed. In 1, the planes containing N1, C1, N2, Mg1 and N1i, C1i, N2i, Mg1i atoms were parallel and made a dihedral angle of 71.50° (70.72° for complex 2) with the plane having Mg1, N2, Mg1i, N2i atoms. The magnesium benzylic carbon distances [2.140(17) for complex 1 and 2.151(13) Å for complex 2] were in agreement with the magnesium–carbon alkyl bonds [2.167(2) Å for [(TMEDA)Mg(CH2Ph)2]18 and analogous complexes [(2.124(3) and 2.152(6) Å] reported in the literature.22 The geometry of each magnesium ion can be best described as a distorted tetrahedral, which is quite well known in magnesium chemistry.
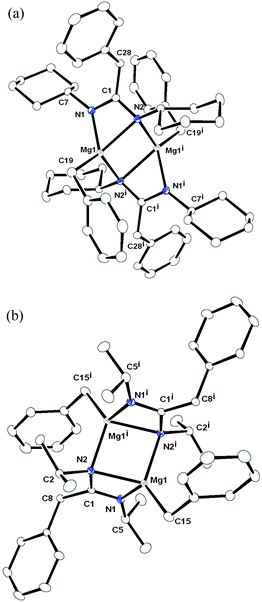 |
| Fig. 1 (a) and (b). Solid-state structures of complexes 1 (a) and 2 (b) and ellipsoids are drawn to encompass 30% probability. Hydrogen atoms are omitted for clarity. Selected bond lengths [Å] and bond angles [°]. | |
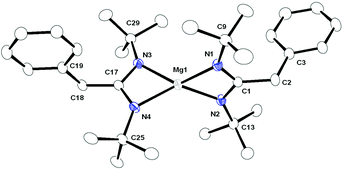 |
| Fig. 2 Solid-state structure of complex 3 and ellipsoids are drawn to encompass 30% probability. Hydrogen atoms are omitted for clarity. Selected bond lengths [Å] and bond angles [°]: Mg1–N1 2.052(4), Mg1–N2 2.044(4), Mg1–N3 2.055(4), Mg1–N4 2.044(3), N1–C1 1.347(5), N2–C1 1.349(5), N3–C17 1.334(5), N4–C17 1.345(5), C1–C2 1.523(5), C17–C18 1.519(5), N2–Mg1–N1 65.96(14), N4–Mg1–N3 65.73(14), N4–Mg1–N1 137.35(15), N4–Mg1–N2 134.18(15), N2–Mg1–N3 136.22(15), N1–Mg1–N3 131.51(15), C1–N1–Mg1 91.1(2), C1–N2–Mg1 91.4(2), C17–N3–Mg1 90.9(2), C17–N4–Mg1 91.1(2), N1–C1–N2 111.5(3), N1–C1–C2 124.1(4), N2–C1–C2 124.3(4), N3–C17–N4 112.2(3), N3–C17–C18 124.5(4), N4–C17–C18 123.1(3). | |
Complex 3 is homoleptic and monomeric in nature. The deviation from heteroleptic complexes 1 and 2 can be attributed to the higher reactivity of the corresponding heteroleptic complex [Mg(CH2Ph)–{tBuN
C(CH2Ph)NBut}], which we were unable to isolate after several attempts. As with complexes 1 and 2, both the magnesium–benzyl bonds were inserted into two carbodiimide carbon–nitrogen bonds to generate two amidinato [tBuNC(CH2Ph)NBut]− moieties, which coordinated to the magnesium atom through all the nitrogen atoms present in the ligand. Unlike in complexes 1 and 2, no sequences of short and long Mg–N distances were observed; instead all Mg–N distances [Mg1–N1 2.052(4), Mg1–N2 2.044(4), Mg1–N3 2.055(4), Mg1–N4 2.044(3)] were in the same range indicating complete delocalisation of the anionic charge over the N–C–N skeleton. This is further confirmed by the similar C–N bond distances [N1–C1 1.347(5), N2–C1 1.349(5), N3–C17 1.334(5), N4–C17 1.345(5)] observed in two [tBuNC(CH2Ph)NBut]− moieties. The Mg–C1 (2.468 Å) and Mg1–C17 (2.478 Å) distances were slightly shorter than those in complexes 1 and 2 but long enough to consider any interaction between magnesium and sp2 carbon atoms as present in the [tBuNC(CH2Ph)NBut]− ligand. Two four-membered magnesium metallacycles N1–C1–N2–Mg1 and N3–C17–N4–Mg1 were formed due to bidentate chelation of two amidinato ligands. The plane containing N1, C1, N2, Mg1 atoms was almost orthogonal (85.22°) with the plane having N3, C17, N4, Mg1 atoms. As a result, the two benzyl planes were also near to orthogonal (80.11°) orientation. The geometry around the magnesium atom can be concluded as pseudo-tetrahedral, corresponding to that observed in complexes 1 and 2.
[Mg{DippN
C(CH2Ph)CH2NDipp}2] complex
α-Diimine ligand 1,4-disubstituted diazabutadienes (DAD) have been proved to be an important class of ligands in both fundamental and applied research due to their diversity in coordination and redox properties.23 The neutral DAD molecule includes two lone pairs of electrons of nitrogen atoms and π-electrons of the multiple imine (N–C) bonds and this molecule can act both as the σ- and π-donor and coordinates to the metal atom as a neutral ligand.24 Although dianionic DAD ligands preferentially coordinate to early transition metals and alkaline earth metals in σ2- and σ2,π-coordination modes,25–27 in many cases the DAD ligands coordinate to group 3 metal atoms as a σ2-monoanion,28 and in addition, both monoanionic and dianionic coordination modes have been observed for alkaline-earth metal group 12 and group 13 metal complexes.29,30 To gain more insight into the structure–reactivity relationships of magnesium DAD complexes, we were interested in studying this chemistry further.
The homoleptic mono-benzylated magnesium complex [Mg{DippN
C(CH2Ph)–CH2NDipp}2] (4) was isolated in good yield by treating [Mg(CH2Ph)2(Et2O)2] with the neutral N,N′-bis(2,6-diisopropyl-phenyl)-1,4-diaza-1,3-butadiene (Dipp2DAD) ligand in toluene at room temperature (Scheme 3). The magnesium complex 4 is a product of the monobenzylation of one of two C
N bonds followed by intramolecular hydrogen transfer. Similar reactions have been observed in the reactions of M(CH2Ph)4 (M = Zr, Hf) with α-diimine ligands, with a 2,6-disubstituted aryl ring.14 The complex was crystallised from hot toluene as red crystals. Complex 4 was soluble in toluene and THF but insoluble in hexane and pentane. Complex 4 was characterised by 1H, 13C{1H} NMR spectroscopy and combustion analysis, and the molecular structure was established by single-crystal X-ray diffraction analysis. In the 1H NMR spectrum of complex 4 measured in C6D6, one set of signals was observed. A sharp singlet was observed at δ 4.32 ppm, which can be assigned to the methylene protons (–CH2) of the [DippN
C(CH2Ph)CH2NDipp] ligand backbone. Two distinct septet signals at δ 3.56 and 2.90 ppm and two doublet resonances having a coupling constant of 6.8 Hz each appeared at δ 1.23 and 1.13 ppm, respectively, due to the –CH hydrogen and isopropyl methyl hydrogen atoms of the ligand [DippN
C(CH2Ph)CH2NDipp]−. Additionally, resonance of benzylic protons was observed at δ 3.22 ppm, which is slightly high-field shifted to the corresponding values of complexes 1–3 (δ 3.70, 3.40 and 3.71 ppm respectively). In the 13C{1H} spectra, resonance at δ 173.5 can be assigned to the imine carbon (–N
C(CH2Ph)–) present in the [DippN
C(CH2Ph)CH2NDipp]− moiety. Other signals in 13C{1H} NMR spectra were in the expected range and in agreement with those of complexes 1–3.
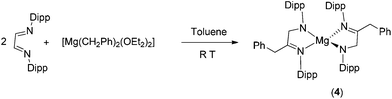 |
| Scheme 3 Synthesis of magnesium complex 4. | |
Complex 4 crystallised in the monoclinic space group C2/c, with four molecules of complex 4 and one toluene molecule as the solvent in the unit cell. The details of structural parameters are given in Table TS1 in the ESI† and the solid-state structure of complex 4 is shown in Fig. 3. Complex 4 is monomeric and the coordination polyhedron is formed by the chelation of one amido and one imine nitrogen atom from each monoanionic [DippN
C(CH2Ph)CH2NDipp]− ligand moiety formed by the monobenzylation of the DAD ligand. The geometry around the magnesium ion can be best described as a distorted tetrahedral, similar to that of complexes 1–3. Like complexes 1 and 2, two kinds of Mg–N distances 2.008(9) and 2.208(1) Å were observed in complex 4, which indicate the presence of amido (short Mg1–N1 bond) and imino (long Mg–N2 bond) nitrogen linkages to the central magnesium ion. As a consequence, C–N bond distances of C1–N1 1.442(12), C1–C2 1.492(13) and C2–N2 1.292(13) Å within the benzylated DAD moiety were observed.14 Thus, the longer C1–N1 and shorter C2–N2 bonds can be assigned to the C–N single and double bonds, respectively, whereas the C1–C2 bond is in agreement with only the C–C single bond. Two magnesium metallacycles Mg1–N1–C1–C2–N2 and Mg1i–N1i–C1i–C2i–N2i were formed and a dihedral angle of 53.61° was observed between the planes containing Mg1–N1, C1, C2, N2 and Mg1i, N1i, C1i, C2i, N2i atoms. The bite angle N1–Mg–N2 of 81.54° was significantly larger than those in complexes 1–3 (63.5° for complex 1, 63.69° for complex 2 and 65.96° for complex 3), which can be explained as the effect of a five-membered magnesocycle formation in complex 4 compared to a four-membered one in complexes 1–3.
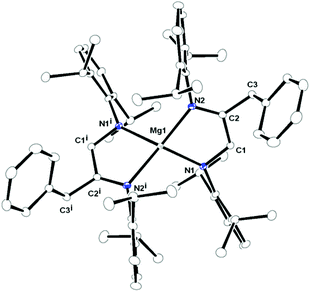 |
| Fig. 3 Solid-state structure of complex 4 and ellipsoids are drawn to encompass 30% probability. Hydrogen atoms are omitted for clarity. Selected bond lengths [Å] and bond angles [°]: Mg1–N1 2.0079(9), Mg1–N2 2.2079(10), Mg1–N1i 2.0079(9), Mg1–N2i 2.2079(10), N1–C1 1.4422(12), N2–C2 1.2915(13), C1–C2 1.4919(13), C2–C3 1.5151(14), N1–Mg1–N2 81.54(3), N1–Mg1–N1i 153.44(6), N1–Mg1–N2i 107.39(3), N1i–Mg1–N2i 81.54(3), N1i–Mg1–N2 107.39(3), N2i–Mg1–N2 141.43(5), C22–N1–Mg1 139.13(6), C1–N1–Mg1 112.82(6), C2–N2–Mg1 110.08(6), C10–N2–Mg1 133.34(6), C22–N1–C1 107.73(7), N2–C2–C1 119.23(9), C2–N2–C10 116.50(8), N2– C2–C3 125.66(9). | |
Reaction with N-aryliminopyrrole
When the toluene solution of complex 1 was treated with the N-aryl iminopyrrole ligand (ImpDipp-H) in 1
:
2 molar ratio at 60 °C, a penta-coordinated magnesium complex 5, with benzylated carbodiimide and two iminopyrrolyl ligands was isolated in good yield (Scheme 4). In the 1H NMR spectra of complex 5, the broad resonance signal at δ 4.06 ppm can be assigned to the amine nitrogen, which is formed after the protonation of the benzylated N,N dicyclocarbodiimide moiety. A singlet peak at δ 3.48 ppm indicates the resonance of two benzylic protons of the [CyN
C(CH2Ph)NHCy] moiety, which is slightly high-field shifted from the corresponding value in complex 1. The resonances of the two imine (DippN
CH–) protons from two iminopyrrolyl ligands appeared as two singlets at δ 7.91 and 7.87 ppm. The resonance of the cyclohexyl protons was in the expected range and similar to those in complex 1. A septet signal centred at δ 3.34 ppm and one doublet at 1.18 ppm, having a coupling constant of 6.4 Hz, are due to the resonance of four –CH protons and 24 methyl protons, respectively, of the diisopropylphenyl (Dipp) groups present in the iminopyrrolyl ligands. In the 13C{1H} NMR spectra, signals at 173.5 and 143.4 ppm can be assigned to the carbon atoms present in the –N
C–N and ImpN
C groups, respectively. It was noted that the amido nitrogen and the magnesium-bound benzyl group in complex 1 undergo protonations in the presence of two equivalents of iminopyrrole ligands to afford complex 5 due to a higher stabilisation of the aromatic pyrrolyl moieties. As a result, Mg–N(amido) and Mg–C bonds in 1 cleaved to form two new amido and two imino Mg–N covalent bonds to stabilise complex 5.
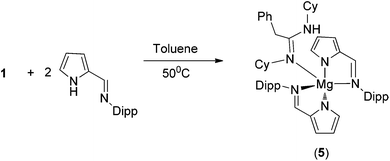 |
| Scheme 4 Synthesis of magnesium complex 5. | |
X-ray quality crystals were obtained from hot toluene and the solid-state structure of complex 5 was established by single-crystal X-ray diffraction analysis. Heteroleptic magnesium complex 5 crystallised in the triclinic space group P
, with two molecules in the unit cell. The details of structural parameters are given in Table TS1 in the ESI† and the solid-state structure of complex 5 is shown in Fig. 4. The metal centre of the magnesium complex 5 was coordinated to two chelating iminopyrrolyl ligands and one imine nitrogen atom from the benzylated carbodiimide ligand [CyN
C(CH2Ph)NHCy], adopting a five-coordinated distorted square bipyramidal geometry. Four nitrogen atoms N1, N2, N3 and N4, of two iminopyrrolyl ligands constructed the basal plane of the square pyramid and the imine nitrogen N5 from the [CyN
C(CH2Ph)NHCy] moiety was located at the axial position. The magnesium atom was located 0.70 Å above the basal plane containing the N1, N2, N3 and N4 atoms. The two chelating iminopyrrolyl moieties of 5 were not coplanar and had a dihedral angle of 47.60° due to the more bulky aromatic substituent 2,6-diisopropylphenyl group on the imine nitrogen atom. The bond distances of Mg−Npyr [Mg1−N2 2.157(4) Å; Mg1−N4, 2.166(3) Å] were shorter than those of Mg−Nimine (Mg1−N1, 2.196(3) Å; Mg1−N3, 2.174(4) Å). The Mg1–N5 distance of 2.215(4) Å also fell in the Mg–Nimine range. Thus, two six-membered magnesium metallacycles Mg1–N1–C42–C41–N4 and Mg1–N3–C25–C24 were formed due to the ligation of two iminopyrrolyl ligands to the magnesium atom. Similar coordination spheres in magnesium chemistry have been reported by us31 and others.6–10,21,22
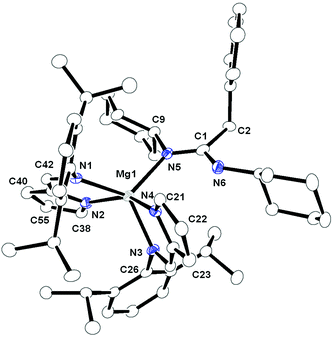 |
| Fig. 4 Solid-state structure of complex 5 and ellipsoids are drawn to encompass 30% probability. Hydrogen atoms are omitted for clarity. Selected bond lengths [Å] and bond angles [°]: Mg1–N1 2.196(3), Mg1–N2 2.157(4), Mg1–N3 2.174(4), Mg1–N4 2.166(3), Mg1–N5 2.215(4), N5–C1 1.311(5), N5–C9 1.492(5), N6–C1 1.350(5), N2–Mg1–N1 77.45(13), N3–Mg1–N4 77.64(14), N2–Mg1–N4 151.34(14), N2–Mg1–N3 92.70(14), N4–Mg1–N1 89.56(13), N3–Mg1–N1 133.21(14), N2–Mg1–N5 106.96(14), N4–Mg1–N5 101.67(13), N3–Mg1–N5 106.56(14), N1–Mg1–N5 120.13(14), C42–N1–C43 120.0(3), C42–N1–Mg1 111.9(3), C25–N3–Mg1 112.6(3), C26–N3–Mg1 128.8(3), C21–N4–Mg1 143.4(3) C24–N4–Mg1 111.6(3), C1–N5–Mg1 125.9(3), C9–N5–Mg1 117.6(2), C1–N5–C9 115.8(3). | |
A tetra-coordinated magnesium complex 6, with mixed iminopyrrolyl and benzylated DAD ligands in the magnesium coordination sphere, was isolated in good yield (Scheme 5) from an analogous reaction between complex 4 and [ImpDippH] in 1
:
1 molar ratio at room temperature. In the 1H proton NMR spectra of complex 6, the resonance of the one imine (DippN
CH–) proton from the iminopyrrolyl ligand appeared at δ 7.55 ppm, which is slightly high-field shifted from the corresponding value (7.87 ppm) in complex 5. The singlet resonance at δ 4.36 ppm can be assigned to benzylic protons of the benzylated DAD ligand and this value is in the same range (3.32 ppm) as that of the corresponding value in complex 4. Two septets at δ 2.82 and 2.73 ppm were obtained for chemically non-equivalent four isopropyl –CH groups present in the benzylated DAD ligand. One additional septet at δ 3.10 ppm was observed and can be assigned to two isopropyl –CH groups which can undergo free rotation around the C–N bond present in the iminopyrrolyl ligand. Three doublets at δ 1.18, 1.08 and 0.89 ppm with coupling constants of 6.4, 6.0 and 6.6 Hz, respectively, were also observed for methyl protons of 2,6-diisopropylphenyl groups present in iminopyrrolyl and benzylated DAD ligands. The resonances in the 13C{1H} NMR spectra were in the expected regions.
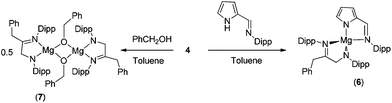 |
| Scheme 5 Syntheses of magnesium complexes 6 and 7. | |
Complex 6 crystallised in the monoclinic space group P21/c, with four molecules in the unit cell. The details of structural parameters are given in Table TS1 in the ESI† and the solid-state structure of complex 6 is shown in Fig. 5. The central metal magnesium atom adopted a distorted tetrahedral geometry due to the coordination of one bidentate iminopyrrolyl ligand and one bidentate benzylated DAD ligand. Both ligands bonded to the magnesium atom via the amido nitrogen and imine nitrogen atoms. Thus, the coordination mode of σ2 was observed for both ligands. The Mg–Npyr bond distance of 2.036(3) Å was in the range of Mg–NDAD amido distance 1.974(3) Å but slightly shorter than the Mg–Npyr distance [2.157(4) Å] observed in complex 5. The Mg–Nimine distances [Mg1–N2 2.150(3) Å and Mg1–N3 2.136(3) Å] were slightly shorter than the corresponding distances obtained in complex 5. These slight differences in bonding can be attributed to more tightly bound tetrahedral magnesium compared to the loosely bound square pyramidal magnesium with the respective ligands. The plane containing the magnesium five-membered metallacycle Mg1–N1–C4–C5–N2 was coplanar, with a pyrrole ring, but made a dihedral angle of 50.50° with the plane containing a second five-membered magnesium metallacycle Mg1–N3–C18–C19–N4. The C–N bond distances of N4–C19 1.437(5), C19–C18 1.482(5) and C18–N3 1.290(4) Å within the benzylated DAD moiety were similar to those present in complex 4 (1.442(12), 1.492(13) and 1.292(13) respectively).
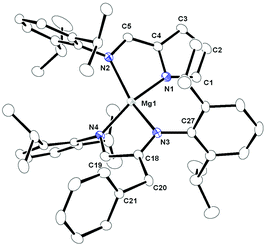 |
| Fig. 5 Solid-state structure of complex 6 and ellipsoids are drawn to encompass 30% probability. Hydrogen atoms are omitted for clarity. Selected bond lengths [Å] and bond angles [°]: Mg1–N1 2.036(3), Mg1–N2 2.150(3), Mg1–N3 2.136(3), Mg1–N4 1.974(3), N3–C18 1.290(4), N3–C27 1.447(5), N4–C19 1.437(5), C18–C19 1.482(5), C18–C20 1.519(5), N1–Mg1–N2 82.32(13), N1–Mg1–N3 100.19(13), N4–Mg1–N1 58.94(15), N4–Mg1–N3 81.63(12), N4–Mg1–N2 110.06(13), N3–Mg1–N2 139.50(13), C1–N1–Mg1 144.3(3), C4–N1–Mg1 110.1(2), C5–N2–Mg1 108.0(3), C18–N3–Mg1 110.6(2), C19–N4–Mg1 111.5(2), C27–N3–Mg1 127.4(2), C39–N4–Mg1 132.9(2), C18–N3–C27 122.0(3). | |
The substitution of one benzylated DAD ligand in the magnesium atom by one pyrrolyl ligand can be explained as a result of higher stability of the anionic pyrrolyl ligand over the anionic benzylated DAD ligand. The solid-state structure is in accordance with the 1H and 13C{1H} NMR spectra, as already discussed.
Reaction with benzyl alcohol
We observed that the Mg–Namido bonds in complex 4 were unstable towards acidic ligands such as N-aryl iminopyrrole, resulting in the mixed ligand magnesium complex 6. To explore further the reactivity of the homoleptic complex 4, we treated it with benzyl alcohol in 1
:
1 molar ratio in toluene at ambient temperature to obtain another mixed ligand magnesium complex 7 in good yield (Scheme 4). Complex 7 shows one set of signals in the 1H NMR spectra measured in C6D6. A singlet resonance at δ 4.42 ppm was observed for two methylene protons (–CH2) of the benzylated DAD ligand. Two other singlet resonances at δ 3.99 and 3.38 ppm can be assigned to two protons each of –OCH2Ph and –CH2Ph groups, respectively. Two septet signals at δ 2.92 and 2.74 ppm were observed due to resonances of protons present in chemically non-equivalent –CH groups of the benzylated DAD ligand. In addition, two doublets with coupling constants of 6.6 and 6.4 Hz at 1.15 and 1.00 ppm were obtained due to the resonance of the methyl protons present in the ligand. The 13C{1H} NMR spectra were also in the expected range and very similar to that of complex 4. The solid-state structure of complex 7 was established by single-crystal X-ray diffraction analysis (Fig. 6).
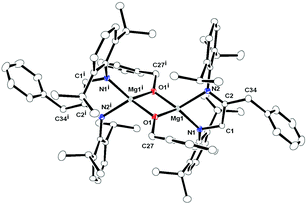 |
| Fig. 6 Solid-state structure of complex 7 and ellipsoids are drawn to encompass 30% probability. Hydrogen atoms are omitted for clarity. Selected bond lengths [Å] and bond angles [°]: Mg1–O1 1.9560(16), Mg1–O1i 1.9602(17), O1–Mg1i 1.9602(17), Mg1–N1 1.9788(19), Mg1–N2 2.1452(19), N1–C1 1.438(3), N2–C2 1.291(3), O1–C27 1.413(3), C1–C2 1.506(3), C(2)–C(34) 1.513(3), O(1)–C(27 1.413(3), O1–Mg1–O1i 84.53(7), O1–Mg1–N1 124.62(8), O1–Mg1–N1i 133.37(8), O1–Mg1–N2 117.76(7), O1i–Mg1–N2 117.70(7), N1–Mg1–N2 82.73(7), C1–N1–Mg1 111.17(13), C2–N2–Mg1 109.45(14), C27–O1–Mg1 134.31(14), C27–O1–Mg1i 126.09(13), Mg1–O1–Mg1i 95.47(7), N1–C1–C2 114.70(17), N2–C2–C1 118.47(18), N2–C2–C34 123.29(19), C1–C2–C34 118.23(18). | |
X-ray quality crystal of complex 7 was obtained from hot toluene. Complex 7 crystallised in the triclinic space group P
, with one molecule of complex 7 and one toluene as a solvent in the unit cell. The details of its structural parameters are given in Table TS1 in the ESI† and the solid-state structure of complex 7 is shown in Fig. 6. The molecular structure revealed that the centro-symmetric magnesium complex 7 is dimeric in nature, through μ bridging of two benzyloxy(–OCH2Ph) groups towards two magnesium ions. The coordination polyhedron in complex 7 was formed by the chelation of one amido and one imine nitrogen atoms from the monoanionic [DippN
C(CH2Ph)–CH2NDipp]− ligand moiety and two bridging benzyloxy groups to adopt each magnesium geometry as a distorted tetrahedral. To the best of our knowledge, complex 7 is the first example of a magnesium complex having a [DippN
C(CH2Ph)CH2NDipp] ligand as well as a benzyloxy ligand. Two kinds of Mg–N distances [Mg1–N1 1.9788(19) Å and Mg–N2 2.1452(19) Å] revealed that each magnesium atom is asymmetrically attached to one amido nitrogen (N1 and N1i) and one imine nitrogen (N2 and N2i) of the benzylated DAD ligand. The Mg–O distances [1.9560(16) and 1.9602(17) Å] were in the range of the reported Mg–O distances in the literature [(1.896(4) Å].22a Thus, two magnesium metallacycles Mg1–N1–C1–C2–N2 and Mg1i–N1i–C1i–C2i–N2i along with a diamond core Mg1–O1–Mg1i–O1i were observed in complex 7 due to ligation of two different ligand systems. Both metallacycles were not coplanar and magnesium ions lay 0.443 Å above the mean plane containing N1, C1, C2 and N2 atoms, and 0.443 Å down the plane containing N1i–C1i–C2i–N2i atoms. The aromatic rings in benzyloxy groups were trans to each other to maintain the symmetry of the molecule.
Experimental
General consideration
All manipulations of air-sensitive materials were performed with the rigorous exclusion of oxygen and moisture in flame-dried Schlenk-type glassware either on a dual manifold Schlenk line, interfaced to a high vacuum (10−4 torr) line, or in an argon-filled M. Braun glove box. Toluene was distilled under nitrogen from LiAlH4 and stored in the glove box. 1H NMR (400 MHz) and 13C{1H} NMR spectra were recorded on a Bruker Avance III-400 spectrometer. Bruker Alpha FT-IR was used for FT-IR measurements. Elemental analyses were performed on a Bruker Euro EA at the Indian Institute of Technology Hyderabad (IITH). Carbodiimides (RN
C
NR; R = Cy, iPr, tBu) were purchased from Sigma Aldrich and used as received. [Mg(CH2Ph)2(Et2O)2],18 Dipp2DAD32 and [ImpDipp-H]33 were prepared according to a procedure described in the literature. The NMR solvent C6D6 was purchased from Sigma Aldrich and dried under Na/K alloy prior to use.
Preparation of [Mg(CH2Ph){CyN
C(CH2Ph)NCy}]2 (1).
In a 50 mL dry Schlenk flask N,N′-dicyclohexylcarbodiimide (DCC) (104 mg, 0.5 mmol) and [Mg(CH2Ph)2(Et2O)2] (178 mg, 0.5 mmol) were mixed together in 1
:
1 ratio in 10 mL of toluene at ambient temperature and stirred for 12 hours at 60 °C. The resulting clear solution was evaporated to dryness in vacuo and 2 mL of fresh toluene was introduced into the flask. The complex was recrystallised from hot toluene as colourless crystals. Yield 176 mg (85%). 1H NMR (400 MHz, C6D6): δ = 7.28 (d, 4H, J = 7.5 Hz, ArH), 7.15 (t, 4H, ArH), 6.99 (t, 2H, ArH), 3.70 (s, 2H, Ph–CH2), 3.21–3.14 (m, 2H, CH), 1.72 (s, 2H, CH2Ph), 1.70–1.67 (m, 8H, Cy–H) 1.43–1.23 (m, 12H, Cy–H) ppm; 13C{1H} NMR (100 MHz, C6D6): δ = 173.7 (N
C–N), 137.7 (ArC), 128.8 (ArC), 126.4 (ArC), 67.1 (Cy–C–N
C), 53.3 (Cy–C–N–C), 37.5 (Ph–CH2), 26.4 (Cy–CH2), 25.9 (Cy–CH2), 25.3 (Cy–CH2) ppm. (C54H72Mg2N4) (825.79) Calc. C 78.54, H 8.79, N 6.78; found C 78.14 H 8.45, N 6.66.
Preparation of [Mg(CH2Ph){iPrN
C(CH2Ph)NiPr}]2 (2).
In a 50 mL dry Schlenk flask N,N′-diisopropylcarbodiimide (64 mg, 0.5 mmol) and [Mg(CH2Ph)2(Et2O)2] (178 mg, 0.5 mmol) were mixed together in 1
:
1 ratio in 10 mL of toluene at ambient temperature and the reaction mixture was stirred for 12 hours at 60 °C. The resulting clear solution was evaporated to dryness in vacuo and 2 mL of fresh toluene was introduced into the flask. The complex was recrystallised from hot toluene as colourless crystals. Yield 135 mg (80%). 1H NMR (400 MHz, C6D6): δ = 7.32 (d, 4H, J = 7.5 Hz, ArH), 7.22 (t, 4H, ArH), 7.08 (t, 2H, ArH), 3.71 (s, 2H, Ph–CH2), 3.52–3.47 (m, 2H, CH), 3.40 (s, 2H, CH2), 1.89 (s, 2H, CH2Ph), 1.13 (d, 6H, J = 1.8 Hz, CH3) 1.10 (m, 6H, J = 1.8 Hz, CH3) ppm; 13C{1H} NMR (100 MHz, C6D6): δ = 173.5 (N
C–N), 137.4 (ArC), 128.3 (ArC), 126.4 (ArC), 67.1 (iPr–C–N
C), 45.7 (iPr–C–N–C), 31.2 (Ph–CH2), 26.9 (iPr-C), 25.5 (CH3) ppm. (C42H56Mg2N4) (665.53) Calc. C 75.80, H 8.48, N 8.42; found C 75.36 H 8.13, N 8.22.
Preparation of [Mg{tBuN
C(CH2Ph)NtBu}2] (3).
In a 50 mL dry Schlenk flask N,N′-di-tert-butylcarbodiimide (154 mg, 1 mmol) and [Mg(CH2Ph)2(Et2O)2] (178 mg, 0.5 mmol) were mixed together in 2
:
1 ratio in 10 mL of toluene at ambient temperature and stirred for 12 hours at 60 °C. The resulting clear solution was evaporated in vacuo to dryness and 2 mL of toluene was introduced into the flask. The title complex was recrystallised from hot toluene as colourless crystals. Yield 210 mg (81%). 1H NMR (400 MHz, C6D6): δ = 7.30 (d, 4H, J = 7.4 Hz, ArH), 7.19 (t, 4H, ArH), 7.06 (t, 2H, ArH), 3.71 (s, 4H, Ph–CH2), 1.01 (s, 36H, CH3) ppm; 13C{1H} NMR (100 MHz, C6D6): δ = 173.5 (N
C–N), 137.4 (ArC), 128.3 (ArC), 126.4 (ArC), 62.3 (CMe3), 29.8 (CMe3) ppm. (C32H50MgN4) (515.07) Calc. C 74.62, H 9.78, N 10.88; found C 74.39 H 9.53, N 10.41.
Preparation of [Mg{DippN
C(CH2Ph)CH2NDipp}2] (4).
In a 50 mL dry Schlenk flask N,N′-bis(2,6-diisopropylphenyl)-1,4-diaza-butadiene(DAD) (376 mg, 1 mmol) and {Mg(CH2Ph)2(Et2O)2} (178 mg, 0.5 mmol) were mixed together in 2
:
1 ratio in 10 mL of toluene at ambient temperature and stirred for 12 hours at room temperature. The resulting red solution was evaporated in vacuo to dryness and 2 mL of toluene was introduced into the flask. Complex 4 was recrystallised from hot toluene as red crystals. Yield 340 mg (77%). 1H NMR (400 MHz, C6D6): δ = 7.19–7.09 (m, 4H, ArH), 7.03–6.99 (m, 12H, ArH), 6.94–6.89 (m, 6H, ArH), 4.32 (s, 4H, N–CH2), 3.56 (sept, 4H, CH), 3.22 (s, 4H, Ph–CH2), 2.90 (sept, 4H, CH), 1.23 (d, 24H, J = 6.8 Hz, CH3) 1.13 (d, 24H, J = 6.8 Hz, CH3) ppm; 13C{1H} NMR (100 MHz, C6D6): δ = 173.5 (ArC), 137.4 (ArC), 128.3 (ArC), 126.4 (ArC), 67.1, 45.7, 31.2, 26.9 (CHMe2), 25.5 ppm (CHMe2). (C73H94MgN4) (1051.86) Calc. C 83.36, H 9.01, N 5.33; found C 82.92 H 8.77, N 4.88.
Preparation of [Mg(ImpDipp)2{CyN
C(CH2Ph)NHCy}] (5).
In a 50 mL dry Schlenk flask complex 1 (206 mg, 0.5 mmol) and N-aryl iminopyrrole [ImpDippH] (248 mg, 1 mmol) were mixed together in 1
:
2 ratio in 10 mL of toluene at ambient temperature and stirred for 12 hours at 60 °C. The resulting clear solution was evaporated to reduce the whole volume to 2 mL. The complex was recrystallised from hot toluene as colourless crystals. Yield 305 (74%).1H NMR (400 MHz, C6D6): δ = 7.91 and 7.87 (s, 2H, N
CH), 7.28–7.15 (m, 11H, ArH), 7.07 (d, 2H, J = 6.6, Py–H), 6.73 (m, 2H, Py–H), 6.15 (d, 2H, J = 6.6, Py–H), 4.06 (br, 1H, NH), 3.82–3.77 (m, 1H, Cy–CH), 3.48 (s, 2H, CH2) 3.34 (sept, 4H, CH), 3.04–2.98 (m, 1H, Cy–CH), 1.58–1.45 (m, 12H, Cy–CH2) 1.18 (d, 24H, J = 6.4 Hz, CH3), 0.80–0.75 (m, 8H, Cy–CH2) ppm; 13C {1H} NMR (100 MHz, C6D6): δ = 173.5 (N
C–N), 143.4 (ImpN
C), 140.7 (Ar–C), 138.9 (Ar–C), 137.9 (Ar–C), 137.3 (Ar–C), 136.8 (Ar–C), 129.1 (Ar–C), 128.8 (Ar–C), 125.5 (Py–C), 125.4 (Py–C), 59.1 (Cy–C–N), 52.3 (Cy–C–N), 32.1 (Ph–CH2), 29.2 (CH), 28.9 (CH), 28.5 (CH2), 26.4 (CH2), 25.4 (CH2), 23.6 (CH2), 22.1 (CH3), 21.3 (CH3) ppm. (C54H70MgN6) (827.48) Calc. C 78.38, H 8.53, N 10.16; found C 77.87 H 8.08, N 9.73.
Preparation of [Mg{DippN
C(CH2Ph)CH2NDipp}(ImpDipp)] (6).
In a 25 mL dry Schlenk flask magnesium complex 4 (240 mg, 0.25 mmol) and N-aryl iminopyrrole [ImpDippH] (63 mg, 0.25 mmol) were mixed together in 1
:
1 ratio in 10 mL of toluene at ambient temperature and stirred for 12 hours at room temperature. The resulting red solution was evaporated to dryness in vacuo and 2 mL of toluene was introduced into the flask. Complex 6 was recrystallised from hot toluene as yellow crystals. Yield 160 mg (78%). 1H NMR (400 MHz, C6D6): δ = 7.55 (s, 1H, N
CH), 7.08 (d, 6H, J = 6.4 Hz, ArH), 6.99–6.92 (m, 10H, Py–H and Ar–H), 6.78 (t, H, Py–H), 6.47 (d, 1H, J = 6.6, Py–H), 4.49 (s, 2H, N–CH2), 4.36 (s, 2H, Ph–CH2), 3.10 (sept, 2H, CH), 2.82 (sept, 2H, CH), 2.73 (sept, 2H, CH), 1.18 (d, 12H, J = 6.4 Hz, CH3), 1.08 (d, 12H, J = 6.0 Hz, CH3), 0.89 (d, 12H, J = 6.6 Hz, CH3) ppm; 13C{1H} NMR (100 MHz, C6D6): δ = 163.8 (N
C–CH2Ph), 153.6 (ImpN
C), 148.6 (ImpN
C), 138.0 (Ar–C), 136.8 (Ar–C), 135.0 (Ar–C), 129.5 (Ar–C), 128.8 (Ar–C), 127.3 (Ar–C), 126.8 (Ar–C), 125.9 (Ar–C), 124.6 (Py–C), 124.3 (Py–C), 123.7 (Py–C), 123.4 (Py–C), 64.9 (N–CH2), 40.7 (Ph–CH2), 29.1 (CH), 28.9 (CH), 25.3 (CH3), 25.0 (CH3), 24.9 (CH3), 24.6 (CH3) ppm. (C50H64MgN4) (745.36) Calc. C 80.57, H 8.65, N 7.52; found C 80.02 H 8.21, N 7.13.
Preparation of [Mg{DippN
C(CH2Ph)CH2NDipp}(OCH2Ph)]2 (7).
In a 25 mL dry Schlenk flask magnesium complex 4 (240 mg, 0.25 mmol) and benzyl alcohol (0.025 mL, 0.25 mmol) were mixed together in 1
:
1 ratio in 10 mL of toluene at ambient temperature and stirred for 12 hours at room temperature. The resulting red solution was evaporated to dryness and 2 mL of toluene was introduced into the flask. Complex 7 was recrystallised from hot toluene as yellow crystals. Yield 280 mg (74%). 1H NMR (400 MHz, C6D6): 7.17–6.98 (m, 16H, Ar–H), 4.42 (s, 2H, N–CH2), 3.99 (s, 2H, O–CH2), 3.38 (s, 2H, PhCH2), 2.92 (sept, 2H, CH), 2.74 (sept, 2H, CH), 1.15 (d, 12H, J = 6.6 Hz, CH3), 1.00 (d, 12H, J = 6.4 Hz, CH3) ppm; 13C[1H] NMR (100 MHz, C6D6): δ 172.5 (ArC), 137.4 (ArC), 128.3 (ArC), 126.4 (ArC), 64.7 (O–CH2), 45.7, 31.2, 26.9 (CHMe2), 25.5 ppm (CHMe2) ppm; (C94H116Mg2N4O2) (1382.5) Calc. C 81.66, H 8.46, N 4.05; found C 81.11 H 8.21, N 3.79.
X-Ray crystallographic studies of complexes 1–7
Single crystals of complexes 1–7 were grown from hot toluene under inert atmosphere. For complexes 1–7, a crystal of a suitable dimension was mounted on a CryoLoop (Hampton Research Corp.) with a layer of light mineral oil and placed in a nitrogen stream at 120(2) K. All measurements were made on a Rigaku RAXIS RAPID imaging plate area detector with graphite monochromated Mo-Kα (0.71075 Å) radiation. Crystal data and structure refinement parameters are summarised in Table TS1 in the ESI.† The structures were solved by direct methods (SIR92)34 and refined on F2 by full-matrix least-squares methods, using SHELXL-97.35 Non-hydrogen atoms were anisotropically refined. H atoms were included in the refinement at calculated positions riding on their carrier atoms. No restraint was made for any of the complexes. The function minimised was [∑w(Fo2 − Fc2)2] (w = 1/[σ2(Fo2) + (aP)2 + bP]), where P = (Max(Fo2,0) + 2Fc2)/3 with σ2(Fo2) from counting statistics. The function R1 and wR2 were (∑||Fo| − |Fc||)/∑|Fo| and [∑w(Fo2 − Fc2)2/∑(wFo4)]1/2, respectively. ORTEP-3 program was used to draw the molecular structure. Crystallographic data (excluding structure factors) for the structures reported in this paper have been deposited at the Cambridge Crystallographic Data Centre as supplementary publication no. CCDC 1019669–1019675.
Conclusion
We have demonstrated the synthetic and structural details of a novel series of magnesium amidinato complexes where the amidinato moieties were obtained from the insertion of a magnesium–carbon bond into the carbon–nitrogen double bond of three different carbodiimides and 1,4-diaza-1,3-butadiene. In each case the proligand either carbodiimide or DAD was converted to a mono-anionic amidinato ligand, which coordinated to the magnesium ion through amido and imino nitrogen atoms to act as a bidentate ligand. In a further reaction with N-aryl iminopyrrole, the magnesium amidinato complexes 1 and 4 afforded another class of magnesium complexes, with newly generated amidinato and pyrrolyl ligands in the metal coordination spheres. Complex 4 also readily reacted with benzyl alcohol in a controlled manner, resulting in a hybrid magnesium complex with benzyloxy(–OCH2Ph) along with a benzylated DAD ligand in the coordination sphere.
Acknowledgements
This work was supported by the Council of Scientific and Industrial Research (CSIR) scheme no. 01(2530)/11/EMRI) and start-up grant from IIT Hyderabad. S.A. and A.H. thank CSIR, India and J.B. thanks the University Grant Commission (UGC), India for their PhD fellowships. We sincerely thank Prof. Kazushi Mashima and Dr Hayato Tsurugi, Osaka University for their generous support.
Notes and references
-
(a) V. C. R. Grignard, Acad. Sci., 1900, 130, 1322–1324 CAS;
(b) V. C. R. Grignard, Ann. Chim., 1901, 24, 433–490 CAS;
(c) D. Seyferth, Organometallics, 2009, 28, 1598–1605 CrossRef CAS.
-
(a) F. J. Bickelhaupt, Organomet. Chem., 1994, 475, 1–14 CrossRef CAS;
(b) M. Westerhausen, Angew. Chem., Int. Ed., 2001, 40, 2975–2977 CrossRef CAS;
(c) M. Westerhausen, M. Gärtner, R. Fischer, J. Langer, L. Yu and M. Reiher, Chem. – Eur. J., 2007, 13, 6292–6306 CrossRef CAS PubMed.
- Y. Sarazin, M. Schormann and M. Bochmann, Organometallics, 2004, 23, 3296–3302 CrossRef CAS.
- L. J. Sanchez-Barba, A. Garces, M. Fajardo, C. Alonso-Moreno, J. Fernandez-Baeza, A. Otero, A. Antinolo, J. Tejeda, A. Lara-Sanchez and M. L_opez-Solera, Organometallics, 2007, 26, 6403–6411 CrossRef CAS.
- Z.-X. Wang and C.-Y. Qi, Organometallics, 2007, 26, 2243–2251 CrossRef CAS.
- C. E. Holloway and M. Melnik, J. Organomet. Chem., 1994, 465, 1–63 CrossRef CAS.
- P. R. Markies, O. S. Akkerman, F. Bickelhaupt, W. J. J. Smeets and A. J. Spek, Adv. Organomet. Chem., 1991, 32, 147–226 CrossRef CAS.
-
F. Bickelhaupt, in Grignard Reagents: New Developments, ed. H. G. Richey, Wiley, Chichester, UK, 2000, ch. 9, pp. 299–328 Search PubMed.
- M. Reinmuth, U. Wild, D. Rudolf, E. Kaifer, M. Enders, H. Wadepohl and H.-J. Himmel, Eur. J. Inorg. Chem., 2009, 4795–4808 CrossRef CAS.
-
(a) P. J. Bailey, C. M. Dick, S. Fabre, S. Parsons and L. J. Yellowlees, Dalton Trans., 2006, 1602–1610 RSC;
(b) P. J. Bailey, C. M. Dick, S. Fabre, S. Parsons and L. J. Yellowlees, Chem. Commun., 2005, 4563–4565 RSC.
- For AlMe3-promoted alkyl migrations, see, for example:
(a) R. Lechler, H.-D. Hausen and J. Weidlein, J. Organomet. Chem., 1989, 359, 1–12 CrossRef CAS;
(b) V. C. Gibson, C. Redshaw, A. J. P. White and D. J. Williams, J. Organomet. Chem., 1998, 550, 453–456 CrossRef CAS;
(c) B. Walfort, A. P. Leedham, C. A. Russell and D. Stalke, Inorg. Chem., 2001, 40, 5668–5674 CrossRef CAS PubMed;
(d) S. B. Cortright, J. N. Coalter-III, M. Pink and J. N. Johnston, Organometallics, 2004, 23, 5885–5888 CrossRef CAS;
(e) T. Chivers, M. C. Copsey and M. Parvez, Chem. Commun., 2004, 2818–2819 RSC;
(f) Q. Knijnenburg, J. M. M. Smits and P. H. M. Budzelaar, Organometallics, 2006, 25, 1036–1046 CrossRef CAS;
(g) V. C. Gibson, C. Redshaw, G. A. Solan, A. J. P. White and D. J. Williams, Organometallics, 2007, 26, 5119–5123 CrossRef CAS;
(h) J. A. Olson, R. Boyd, J. W. Quail and S. R. Foley, Organometallics, 2008, 27, 5333–5338 CrossRef CAS.
- For ZnMe2-promoted alkyl migrations, see, for example:
(a) U. Braun, B. B€ock, H. N€oth, I. Schwab, M. Schwartz, S. Weber and U. Wietelmann, Eur. J. Inorg. Chem., 2004, 3612–3628 CAS;
(b) I. J. Blackmore, V. C. Gibson, P. B. Hitchcock, C. W. Rees, D. J. Williams and A. J. P. White, J. Am. Chem. Soc., 2005, 127, 6012–6020 CrossRef CAS PubMed;
(c) I. L. Fedushkin, A. N. Tishkina, G. K. Fukin, M. Hummert and H. Schumann, Eur. J. Inorg. Chem., 2008, 483–489 CrossRef CAS.
- For group 3-promoted alkyl migrations, see, for example:
(a) M. Zimmermann, K. W. T€ornroos and R. Anwander, Angew. Chem., Int. Ed., 2007, 46, 3126–3130 CrossRef CAS PubMed;
(b) H. Kaneko, H. Tsurugi, T. K. Panda and K. Mashima, Organometallics, 2010, 29, 3463–3466 CrossRef CAS.
- For group 4 metal-promoted diimine alkylation, see:
(a) K. Mashima, R. Ohnishi, T. Yamagata and H. Tsurugi, Chem. Lett., 2007, 36, 1420–1421 CrossRef CAS;
(b)
R. E. Murray, U.S. Patent6,096,676, Union Carbide Corp., 2000 Search PubMed;
(c) P. De Waele, B. A. Jazdzewski, J. Klosin, R. E. Murray, C. N. Theriault, P. C. Vosejpka and J. L. Petersen, Organometallics, 2007, 26, 3896–3899 CrossRef CAS;
(d) H. Tsurugi, R. Ohnishi, H. Kaneko, T. K. Panda and K. Mashima, Organometallics, 2009, 28, 680–687 CrossRef CAS;
(e) H. Tsurugi, K. Yamamoto and K. Mashima, J. Am. Chem. Soc., 2011, 133, 732–735 CrossRef CAS PubMed.
-
(a) J. L. Atwood and G. D. Stucky, J. Am. Chem. Soc., 1969, 91, 2538–2543 CrossRef CASK. Liebigs, Ann. Chem., 1957, 605, 93–97 Search PubMed;
(b) O. Michel, C. Meermann, K. W. Törnroos and R. Anwander, Organometallics, 2009, 28, 4783–4790 CrossRef CAS.
- O. Michel, K. Yamamoto, H. Tsurugi, C.-M. Mössmer, K. W. Törnroos, K. Mashima and R. Anwander, Organometallics, 2011, 30, 3818–3825 CrossRef CAS.
- T. K. Panda, H. Tsurugi, K. Pal, H. Kaneko and K. Mashima, Organometallics, 2010, 29, 34–37 CrossRef CAS.
- P. J. Bailey, R. A. Coxall, C. M. Dick, S. Fabre, L. C. Henderson, C. Herber, S. T. Liddle, D. L. Gonzalez, A. Parkin and S. Parsons, Chem. – Eur. J., 2003, 9, 4820–4828 CrossRef CAS PubMed.
-
(a) R. J. Wehmschulte and P. P. Power, Organometallics, 1995, 14, 3264–3267 CrossRef CAS;
(b) S. S. Al-Juaid, C. Eaborn, P. B. Hitchcock, C. A. McGeary and J. D. Smith, Chem. Commun., 1989, 273–274 RSC;
(c) P. B. Hitchcock, J. A. K. Howard, M. F. Lappert, W. P. Leung and S. A. Mason, Chem. Commun., 1990, 847–849 RSC;
(d) K. B. Starowieyski, J. Lewinski, R. Wozniak, J. Lipkowski and A. Chrost, Organometallics, 2003, 22, 2458–2463 CrossRef CAS.
-
(a) M-Dar Li, C-Cheng Chang, Y. Wang and G.-H. Lee, Organometallics, 1996, 15, 2571–2574 CrossRef CAS;
(b) A. Xia, M. J. Heeg and C. H. Winter, Organometallics, 2002, 21, 4718–4725 CrossRef CAS;
(c) H. Hao, S. Bhandari, Y. Ding, H. W. Roesky, J. Magull, H. G. Schmidt, M. Noltemeyer and C. Cui, Eur. J. Inorg. Chem., 2002, 1060–1065 CrossRef CAS;
(d) Y. Matsuo, H. Tsurugi, T. Yamagata and K. Mashima, Bull. Chem. Soc. Jpn., 2003, 76, 1965–1968 CrossRef CAS;
(e) A. Xia, H. M. El-Kaderi, M. J. Heeg and C. H. Winter, J. Organomet. Chem., 2003, 682, 224–232 CrossRef CAS;
(f) T. K. Panda, K. Yamamoto, K. Yamamoto, H. Kaneko, Y. Yang, H. Tsurugi and K. Mashima, Organometallics, 2012, 31, 2268–2274 CrossRef CAS;
(g) A. J. Blake, W. Lewis and D. L. Kays, Dalton Trans., 2014, 43, 4838–4846 RSC;
(h) M. Westerhausen and H.-D. Hausen, Z. Anorg. Allg. Chem., 1992, 615, 27–34 CrossRef CAS.
-
(a) R. T. Boeré, M. L. Colew and P. C. Junk, New J. Chem., 2005, 29, 128–134 RSC;
(b) G. J. Moxey, F. Ortu, L. G. Sidley, H. N. Strandberg, T. Chivers, M. C. Copsey, C. Fedorchuk, M. Parvez and M. Stubbs, Organometallics, 2005, 24, 1919–1928 CrossRef;
(c) M. Lin, W. Liu, Z. Chen, L. Yang, H. Pei, J. Wu, X. Wan, T. Lei and Y. Li, RSC Adv., 2012, 2, 3451–3457 RSC;
(d) B. Liu, T. Roisnel, J.-F. Carpentier and Y. Sarazin, Angew. Chem., Int. Ed., 2012, 51, 4943–4946 CrossRef CAS PubMed;
(e) P. L. Arnold, I. S. Edworthy, C. D. Carmichael, A. J. Blake and C. Wilson, Dalton Trans., 2008, 3739–3748 RSC;
(f) B. M. Day, N. E. Mansfield, M. P. Coles and P. B. Hitchcock, Chem. Commun., 2011, 47, 4995–4997 RSC;
(g) L.-F. Hsueh, N.-T. Chuang, C.-Y. Lee, A. Datta, J.-H. Huang and T.-Y. Lee, Eur. J. Inorg. Chem., 2011, 5530–5537 CrossRef CAS;
(h) J.-C. Buffet, J. P. Davin, T. P. Spaniol and J. Okuda, New J. Chem., 2011, 35, 2253–2257 RSC;
(i) M. H. Chisholm, J. C. Gallucci and K. Phomphrai, Inorg. Chem., 2005, 44, 8004–8010 CrossRef CAS PubMed;
(j) L. Wang and H. Ma, Macromolecules, 2010, 43, 6535–6537 CrossRef CAS;
(k) X. Xu, Y. Chen, G. Zou, Z. Ma and G. Li, J. Organomet. Chem., 2010, 695, 1155–1162 CrossRef CAS PubMed.
-
(a) X. Zhang, T. J. Emge and K. C. Hultzsch, Angew. Chem., Int. Ed., 2012, 51, 394–398 CrossRef CAS.
-
(a) J. Scholz, M. Dlikan, D. Ströhl, A. Dietrich, H. Schumann and K.-H. Thiele, Chem. Ber., 1990, 123, 2279–2285 CrossRef CAS;
(b) J. Scholz, A. Dietrich, H. Schumann and K.-H. Thiele, Chem. Ber., 1991, 124, 1035–1039 CrossRef CAS;
(c) J. Scholz, B. Richter, R. Goddard and C. Krüger, Chem. Ber., 1993, 126, 57–61 CrossRef CAS;
(d) B. Richter, J. Scholz, B. Neumüller, R. Weimann and H. Schumann, Z. Anorg. Allg. Chem., 1995, 621, 365–372 CrossRef CAS;
(e) H. Görls, B. Neumüller, A. Scholz and J. Scholz, Angew. Chem., Int. Ed. Engl., 1995, 34, 673–676 CrossRef.
-
(a) K. Vrieze, J. Organomet. Chem., 1986, 300, 307–326 CrossRef CAS;
(b) H. Bock and H. tom Dieck, Chem. Ber., 1967, 100, 228–246 CrossRef CAS.
-
(a) I. L. Fedushkin, N. M. Khvoinova, A. A. Skatova and G. K. Fukin, Angew. Chem., Int. Ed., 2003, 42, 5223–5226 CrossRef CAS PubMed;
(b) I. L. Fedushkin, A. A. Skatova, V. K. Cherkasov, V. A. Chudakova, S. Dechert, M. Hummert and H. Schumann, Chem. – Eur. J., 2003, 9, 5778–5783 CrossRef CAS PubMed;
(c) I. L. Fedushkin, A. A. Skatova, M. Hummert and H. Schumann, Eur. J. Inorg. Chem., 2005, 1601–1608 CrossRef CAS;
(d) I. L. Fedushkin, A. A. Skatova, G. K. Fukin, M. Hummert and H. Schumann, Eur. J. Inorg. Chem., 2005, 2332–2338 CrossRef CAS;
(e) I. L. Fedushkin, A. N. Lukoyanov, M. Hummert and H. Schumann, Z. Anorg. Allg. Chem., 2008, 634, 357–361 CrossRef CAS;
(f) Y. Liu, P. Yang, J. Yu, X.-J. Yang, J. D. Zhang, Z. Chen, H. F. Schaefer and B. Wu, Organometallics, 2008, 27, 5830–5835 CrossRef CAS;
(g) S. Anga, K. Naktode, H. Adimulam and T. K. Panda, Dalton Trans., 2014, 43, 14876–14888 RSC.
-
(a) A. Galindo, A. Ienco and C. Mealli, New J. Chem., 2000, 24, 73–75 RSC;
(b) P. J. Daff, M. Etienne, B. Donnadieu, S. Z. Knottenbelt and J. E. McGrady, J. Am. Chem. Soc., 2002, 124, 3818–3819 CrossRef CAS PubMed;
(c) M. Ghosh, S. Sproules, T. Weyhermüller and K. Wieghardt, Inorg. Chem., 2008, 47, 59635970 Search PubMed;
(d) M. Ghosh, T. Weyhermüller and K. Wieghardt, Dalton Trans., 2008, 5149–5151 RSC.
-
(a) K. Mashima, Y. Matsuo and K. Tani, Chem. Lett., 1997, 767–768 CrossRef CAS;
(b) K. Mashima, Y. Matsuo and K. Tani, Organometallics, 1999, 18, 1471–1481 CrossRef CAS;
(c) Y. Matsuo, K. Mashima and K. Tani, Angew. Chem., Int. Ed., 2001, 40, 960–962 CrossRef CAS;
(d) T. K. Panda, H. Kaneko, O. Michel, H. Tsurugi, K. Pal, K. W. Törnroos, R. Anwander and K. Mashima, Organometallics, 2012, 31, 3178–3184 CrossRef CAS;
(e) T. K. Panda, H. Kaneko, K. Pal, H. Tsurugi and K. Mashima, Organometallics, 2010, 29, 2610–2615 CrossRef CAS;
(f) S. Anga, R. K. Kottalanka, T. Pal and T. K. Panda, J. Mol. Struct., 2013, 1040, 129–138 CrossRef CAS PubMed.
-
(a) A. A. Trifonov, E. N. Kirillov, S. Dechert, H. Schumann and M. N. Bochkarev, Eur. J. Inorg. Chem., 2001, 2509–2514 CrossRef CAS;
(b) A. A. Trifonov, Y. A. Kurskii, M. N. Bochkarev, S. Muehle, S. Dechert and H. Schumann, Russ. Chem. Bull., 2003, 52, 601–606 CrossRef CAS;
(c) A. A. Trifonov, E. A. Fedorova, V. N. Ikorskii, S. Dechert, H. Schumann and M. N. Bochkarev, Eur. J. Inorg. Chem., 2005, 2812–2818 CrossRef CAS;
(d) P. Cui, Y. Chen, G. Wang, G. Li and W. Xia, Organometallics, 2008, 27, 4013–4016 CrossRef CAS. For lanthanide complexes having μ-dianionic DAD ligands:
(e) A. A. Trifonov, L. N. Zakharov, M. N. Bochkarev and Y. T. Struchkov, Russ. Chem. Bull., 1994, 43, 148–151 Search PubMed;
(f) J. Scholz, H. Görls, H. Schumann and R. Weimann, Organometallics, 2001, 20, 4394–4402 CrossRef CAS.
-
(a) F. S. Mair, R. Manning, R. G. Pritchard and J. E. Warren, Chem. Commun., 2001, 1136–1137 RSC;
(b) R. J. Baker, R. D. Farley, C. Jones, M. Kloth and D. M. Murphy, J. Chem. Soc., Dalton Trans., 2002, 3844–3850 RSC;
(c) R. J. Baker, C. Jones and D. M. Murphy, Chem. Commun., 2005, 1339–1341 RSC;
(d) X.-J. Yang, J. Yu, Y. Liu, Y. Xie, H. F. Schaefer, Y. Liang and B. Wu, Chem. Commun., 2007, 2363–2365 RSC;
(e) C. Jones, A. Stasch and W. D. Woodul, Chem. Commun., 2009, 113–115 RSC.
- Acenaphthene-based DAD complexes:
(a) I. L. Fedushkin, A. A. Skatova, V. A. Chudakova, N. M. Khvoinova, A. Y. Baurin, S. Dechert, M. Hummert and H. Schumann, Organometallics, 2004, 23, 3714–3718 CrossRef CAS;
(b) I. L. Feduchkin, N. M. Khvoinova, A. Y. Baurin, G. K. Fukin, V. K. Cherkasov and M. P. Bubnov, Inorg. Chem., 2004, 43, 7807–7815 CrossRef PubMed;
(c) I. L. Fedushkin, V. A. Chudakova, A. A. Skatova, N. M. Khvoinova, Y. A. Kurskii, T. A. Glukhova, G. K. Fukin, S. Dechert, M. Hummert and H. Schumann, Z. Anorg. Allg. Chem., 2004, 630, 501–507 CrossRef CAS;
(d) I. L. Fedushkin, V.A. Chudakova, A. A. Skatova and G. K. Fukin, Heteroat. Chem., 2005, 16, 663–669 CrossRef CAS;
(e) H. Schumann, M. Hummert, A. N. Lukoyanov and I. L. Fedushkin, Organometallics, 2005, 24, 3891–3896 CrossRef CAS;
(f) A. N. Lukoyanov, I. L. Feduchkin, H. Schumann and M. Hummert, Z. Anorg. Allg. Chem., 2006, 632, 1471–1476 CrossRef CAS;
(g) I. L. Fedushkin, V. M. Makarov, E. C. E. Rosenthal and G. K. Fukin, Eur. J. Inorg. Chem., 2006, 827–832 CrossRef CAS;
(h) I. L. Fedushkin, M. Hummert and H. Schumann, Eur. J. Inorg. Chem., 2006, 3266–3273 CrossRef CAS;
(i) H. Schumann, M. Hummert, A. N. Lukoyanov and I. L. Fedushkin, Chem. – Eur. J., 2007, 13, 4216–4222 CrossRef CAS PubMed;
(j) I. L. Feduchkin, A. A. Skatova, O. V. Eremenko, M. Hummert and H. Schumann, Z. Anorg. Allg. Chem., 2007, 633, 1739–1742 CrossRef;
(k) I. L. Fedushkin, A. N. Tishkina, G. K. Fukin, M. Hummert and H. Schumann, Eur. J. Inorg. Chem., 2008, 483–489 CrossRef CAS;
(l) I. L. Feduchkin, A. G. Morozov, M. Hummert and H. Schumann, Eur. J. Inorg. Chem., 2008, 1584–1588 CrossRef;
(m) I. L. Fedushkin, A. S. Nikipelov, A. A. Skatova, O. V. Maslova, A. N. Lukoyanov, G. K. Fukin and A. V. Cherkasov, Eur. J. Inorg. Chem., 2009, 3742–3749 CrossRef CAS;
(n) I. L. Fedushkin, A. G. Morozov, V. A. Chudakova, G. K. Fukin and V. K. Cherkasov, Eur. J. Inorg. Chem., 2009, 4995–5003 CrossRef CAS.
-
(a) R. K. Kottalanka, A. Harinath, J. Bhattacharjee, H. V. Babu and T. K. Panda, Dalton Trans., 2014, 43, 8757–8766 RSC;
(b) R. K. Kottalanka, K. Naktode, S. Anga, H. P. Nayek and T. K. Panda, Dalton Trans., 2013, 42, 4947–4956 RSC.
-
(a) J. M. Kliegman and R. K. Barnes, Tetrahedron, 1970, 26, 2555–2560 CrossRef CAS;
(b) J. M. Kliegman and R. K. Barnes, J. Org. Chem., 1970, 35, 3140–3143 CrossRef CAS;
(c) H. A. Zhong, J. A. Labinger and J. E. Bercaw, J. Am. Chem. Soc., 2002, 124, 137801399 Search PubMed.
- Y. Matsuo, K. Mashima and K. Tani, Chem. Lett., 2000, 29, 1114–1115 CrossRef.
- A. Altomare, M. C. Burla, G. Camalli, G. Cascarano, C. Giacovazzo, A. Gualiardi and G. Polidori, J. Appl. Crystallogr., 1994, 27, 435 Search PubMed.
- G. M. Sheldrick, Acta Crystallogr., Sect. A: Fundam. Crystallogr., 2008, 64, 112–122 CrossRef CAS PubMed.
Footnote |
† Electronic supplementary information (ESI) available. CCDC 1019669–1019675. For ESI and crystallographic data in CIF or other electronic format see DOI: 10.1039/c4dt03074e |
|
This journal is © The Royal Society of Chemistry 2015 |
Click here to see how this site uses Cookies. View our privacy policy here.