DOI:
10.1039/C3CE41885E
(Communication)
CrystEngComm, 2014,
16, 24-27
Celecoxib cocrystal polymorphs with cyclic amides: synthons of a sulfonamide drug with carboxamide coformers†
Received
18th September 2013
, Accepted 20th September 2013
First published on 23rd September 2013
Abstract
The trimorphic cocrystals of celecoxib with δ-valerolactam contain sulfonamide dimers and catemers together with carboxamide dimers, whereas the caprolactam cocrystal is sustained by a SO2N–H⋯H–NOC heterosynthon. The alternation of synthons with even–odd ring coformers offers a crystal engineering approach to sulfonamide–carboxamide cocrystals.
Celecoxib (4-[5-(4-methylphenyl)-3-(triflouromethyl)-1H-pyrazol-1-yl]benzenesulfonamide, CEL) is a non-steroidal anti-inflammatory drug (NSAID). CEL is a specific COX-2 inhibitor for pain and inflammation without inhibiting COX-1.1 A major downside to this popular NSAID is its poor aqueous solubility (9 mg L−1), which limits bioavailability (40%). Celecoxib is a Biopharmaceutical Classification System (BCS) Class II drug (low solubility and high permeability) with a dose number of 80 (i.e. a normal tablet of celecoxib will require 80 glasses of water to be fully soluble).2 Among all the coxib family of drugs,3 CEL stands out with fewer adverse side effects and lower risk of heart complications,4 because CEL is an order of magnitude less selective for COX-2 enzymes.
Several methods are reported to improve the poor aqueous solubility and bioavailability of CEL5 including solid-state forms such as polymorphs, pseudopolymorphs, amorphous dispersion, nicotinamide cocrystals, and sodium salt hydrates.6 Regarding the four polymorphs of CEL, form III (CEL-III) is the most stable and its crystal structure is reported.6e A metastable polymorph CEL-IV showed four times greater solubility and improved bioavailability compared to CEL-III. Only one cocrystal of CEL with nicotinamide (CEL–NIC) is reported,6e whose crystal structure was solved by powder XRD, but this cocrystal is very unstable and rapidly dissociates to form insoluble CEL-III within 2 min in the dissolution medium, even with surfactants added. Remenar et al.6f reported CEL–Na salt hydrate crystals of thin plate morphology after extensive studies and considerable efforts to resolve the single crystal X-ray structure. Despite its position as a top NSAID prescription drug, single crystal X-ray structures of celecoxib cocrystals and salts are scarce. We report the results of a solid form screen of celecoxib (Scheme 1) to improve its physico-chemical and pharmaco-kinetic properties. At the outset we noted that most of the cocrystals of sulfonamide drugs archived in the Cambridge Structural Database contain a SO2NH-2-aminopyridine fragment (e.g. sulfadiazine, sulfamethazine, sulfapyridine, etc.),7 and consequently the recurring synthons involve the 2-aminopyridine fragment with a COOH/CONH partner (Scheme 2), whereas the SO2N moiety is usually free (see Table S1 and Fig. S1–S3, ESI†). We recently reported sulfonamide cocrystals with pyridine-N-oxide coformers8 in heterosynthon motifs, but the latter are not pharmaceutically acceptable additives. Attempts with sulfacetamide–acetamide cocrystals gave large ring motifs,9 and so we turned to cyclic amides with the idea to establish a supramolecular synthon10 pattern for the sulfonamide group with GRAS coformers (generally regarded as safe by the US-FDA),11 using celecoxib as a case study.
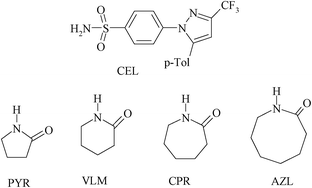 |
| Scheme 1 Chemical diagram of CEL and coformers in this study. CPR is a GRAS coformer and VLM is a pharmaceutically acceptable molecule. | |
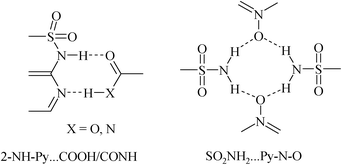 |
| Scheme 2 The dominant heterosynthon for sulfonamide drugs in the CSD (left, see Fig. S1, ESI†) does not involve the SO2NH moiety. The heterosynthon of SO2NH2 with N-oxide (right, ref. 8) is in non-GRAS cocrystals as a model study. | |
X-ray crystal structures of CEL cocrystals with 2-pyrrolidinone (PYR), δ-valerolactam (VLM), caprolactam (CPR), aza-2-cyclooctanone (AZL) are discussed (Scheme 1, Table S2 and S3, and Fig. S4, ESI†). Celecoxib afforded cocrystal polymorphs with valerolactam of N–H⋯O dimer and catemer synthons with SO2NH groups. The CEL–VLM cocrystal is a trimorphic cluster. The amide dimer R22(8) motif of valerolactam is present in all three polymorphs, the main difference being that VLM dimers are connected by sulfonamide dimers in form I, and sulfonamide catemers in form II and III (Fig. 1, 2, and Fig. S5, ESI†). Forms II and III differ by the number of symmetry-independent molecules (Z′ = 2, 1). Whereas the valerolactam cocrystal (6-member cyclic amide) contains homosynthons of SO2NH and CONH, the 7-member cyclic amide (CPR) has SO2NH⋯CONH heterosynthons of R22(8) motif (Scheme 3). PYR and CPR cocrystals are assembled via the (CEL)-sulfonamide-carboxamide-(coformer) heterosynthon with R23(8)R22(8) motif (Fig. 3 and Fig. S5, ESI†). CEL–AZL crystal packing is similar to that of CEL–VLM polymorph I. All the cocrystals were fully characterized by their signature FT-IR, PXRD, DSC, ss-NMR spectra (Table S4–S6, Fig. S6–S8, ESI†).
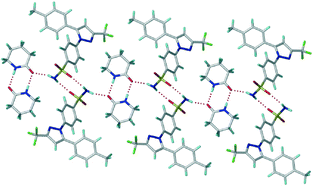 |
| Fig. 1 Sulfonamide and syn-carboxamide groups assemble via homodimer synthons in CEL–VLM-I (1 : 1). | |
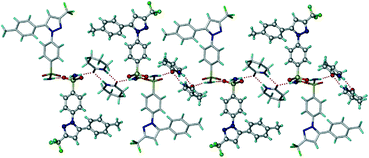 |
| Fig. 2 Symmetry-independent molecules of CEL and VLM (capped stick and ball & stick model) are connected by an N–H⋯O catemer to the carboxamide dimer in CEL–VLM-II (1 : 1). | |
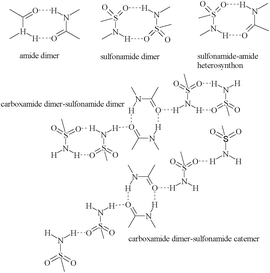 |
| Scheme 3 Schematic representation of the possible synthons present in this study between sulfonamide and syn-carboxamide functional groups. | |
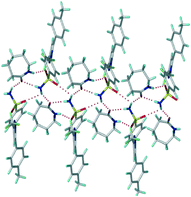 |
| Fig. 3 The structure of CEL–CPR (1 : 1) is sustained by the N–H⋯O heterosynthon R23(8)R22(8) motif. | |
A trimorphic cluster of polymorphic cocrystals12 is rare. We retrieved only 3 tetramorphic and 3 trimorphic compared to 95 dimorphic examples13 of cocrystal sets in the CSD (see summary in Table S7, ESI†). Hirshfeld surface analysis of CEL–VLM trimorphs was carried out to quantify the intermolecular interactions map. Ethenzamide–gentisic acid trimorphic cocrystals12b (EA–GA) were selected as a second API system for Hirshfeld surface analysis.14
The stability of CEL–VLM polymorphs was studied to compare with the calculated contributions of strong H bonds in Hirshfeld surfaces. The transformation of form II to form I was established by manual grinding for 20 min, after which time complete conversion was confirmed by PXRD (Fig. S7, ESI†). Furthermore, VT-PXRD of CEL–VLM-III showed that it converted to CEL–VLM-II at about 70 °C (phase transition temperature in DSC, Fig. 4 and Fig. S8†). Heating the bulk material in a temperature-controlled oven confirmed the same III to II transition at 70–80 °C. A clear shift in the amide carbonyl IR stretch was observed for trimorphic CEL–VLM cocrystals (form I, II, III: 1662.2, 1652.4, 1643.2 cm−1), indicative of differences in their hydrogen bond motifs. The DSC thermogram of CEL–VLM polymorphs indicates that form I melts at 106 °C, form II at 108 °C, and form III shows a small endotherm at 71 °C (phase transition to form II) and then melts at 110 °C. The phase transition on heating was confirmed by VT-PXRD. CEL–VLM-I and II are monotropically related, and CEL–VLM-II and III are enantiotropic. The two carbonyl peaks in the 13C ss-NMR spectrum of CEL–VLM-II at δ 173 and 175 (Fig. 5) are consistent with Z′ = 2 in the crystal structure. Hirshfeld surface analysis of the two major interactions in CEL–VLM polymorphs (O⋯H + F⋯H = 39.8%, 34.5%, 34.0% in Table 1) matches with the observed stability order of cocrystals I > II > III. In order to assess the Hirshfeld analysis method for stability assessment, another important trimorphic cocrystal system of ethenzamide–gentisic acid (EA–GA) was studied by calculating the contribution of the two major interactions in the crystal structures. The observation that form I is the most stable cocrystal, form II in between, and form III being the least stable is consistent with the Hirshfeld analysis (41.5%, 38,5%, 35.0% in Table 1; Fig. S9 and S10, ESI†). Comparing the stability of two-component systems can be more difficult than that for API polymorphs because there can be possible decomposition of one of the components or dissociation in the heating/slurry conditions. Moreover, ab initio energy computations on cocrystals are relatively less studied and more time-consuming than those for single component structures.15 In this background, Hirshfeld surface analysis of the two major hydrogen bonds provides a semi-quantitative guide to the relative energy levels of pharmaceutical cocrystals.
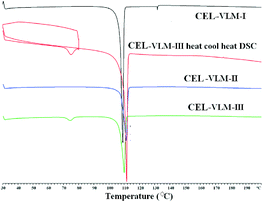 |
| Fig. 4 DSC of CEL–VLM cocrystal polymorphs. A heat–cool–heat experiment shows the transformation of form III to II at 70–80 °C (see also: VT-PXRD in Fig. S7d, ESI†), whereas form I and form II did not exhibit any phase transition on heating. | |
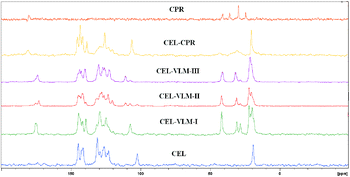 |
| Fig. 5 ss-NMR spectra of the CEL cocrystals. The two carbonyl peaks for CEL–VLM-II at δ 173 and 175 are consistent with Z′ = 2 in the crystal structure. | |
Table 1 Hirshfeld surface finger print plot percentage of intermolecular interactions in cocrystal polymorphs CEL–VLM and EA–GA. The top two major % of interactions are shown in bold for each system
Cocrystal |
C⋯H, % |
O⋯H, % |
N⋯H, % |
F⋯H, % |
Sum of top two interactions |
CEL–VLM-I |
9.9 |
19.3
|
6.8 |
20.5
|
39.8 |
CEL–VLM-II |
11.5 |
19.6
|
5.5 |
14.9
|
34.5 |
CEL–VLM-III |
13.3 |
15.5
|
6.8 |
18.5
|
34.0 |
EA–GA-I |
16.0
|
24.5
|
1.0 |
— |
41.5 |
EA–GA-II |
15.1
|
22.1
|
1.3 |
— |
38.5 |
EA–GA-III |
11.8
|
22.1
|
1.1 |
— |
35.0 |
In conclusion, single crystal X-ray structures of celecoxib cocrystals are reported for the first time with GRAS lactams and new synthons between the sulphonamide and lactam functional groups are explained as even–odd ring size changes. Hirshfeld surface analysis is shown to rank the stability of cocrystal polymorphs. The present study on sulfonamide lactams provides a starting point for synthon-based crystal engineering of sulfonamide drugs. The solubility of stable CEL–VLM-I is 2 times and that of CEL–CPR is 4 times greater than that of the reference drug. Physicochemical and stability measurements on celecoxib cocrystals are currently in progress.
Notes and references
-
(a) N. M. Davies, A. J. McLachlan, R. O. Day and K. M. Williams, Clin. Pharmacokinet., 2000, 38, 225 CrossRef CAS PubMed;
(b) X. Fan, F. Takahashi-Yanaga, S. Morimoto, D. Zhan, K. Igawa, K. Tomooka and T. Sasaguri, J. Pharmacol. Exp. Ther., 2011, 338, 2 CrossRef CAS PubMed.
-
(a)
http://166.78.14.201/tsrlinc.com/services/bcs/search.cfm
;
(b) N. Seedher and S. Bhatia, AAPS PharmSciTech, 2003, 4, E33 CrossRef CAS PubMed.
-
(a) S. S. V. Padi, N. K. Jain, S. Singh and S. K. Kulkarni, Eur. J. Pharmacol., 2004, 491, 69 CrossRef CAS PubMed;
(b) S. K. Kulakarni and N. K. Jain, Indian J. Pharmacol., 2005, 37, 86 CrossRef PubMed.
-
(a) M. Wadman, Nature, 2006, 441, 262 CrossRef CAS PubMed;
(b) M. Wadman, Nature, 2007, 448, 400 CrossRef CAS PubMed.
-
(a) S. Punitha, V. Hari and D. Karthikeyan, Int. J. Pharm. Sci., 2010, 2, 109 CAS;
(b) Y. Liu, C. Sun, Y. Hao, T. Jiang, L. Zheng and S. Wang, J. Pharm. Pharmaceut. Sci., 2010, 13, 589 CAS;
(c) G. V. M. M. Babu, V. G. Shankar, K. H. Shankar, A. Sheshasayana, N. K. Kumar and K. V. R. Murthy, Indian J. Pharmacol., 2002, 588 CAS;
(d) M. N. Reddy, T. Rehena, S. Ramakrishna, K. P. R. Chowdary and P. V. Divan, AAPS PharmSciTech, 2004, 6, 68 CrossRef PubMed;
(e) R. Swati and K. J. Sanjay, Eur. J. Pharm. Biopharm., 2004, 57, 263 CrossRef PubMed;
(f) P. Zhao, H. Jiang, T. Jiang, Z. Zhi, C. Wu, C. Sun, J. Zhang and S. Wang, Eur. J. Pharm. Sci., 2012, 45, 639 CrossRef CAS PubMed.
-
(a) G. W. Lu, M. Hawley, M. Smith, B. M. Geiger and W. Pfund, J. Pharm. Sci., 2006, 95, 305 CrossRef CAS PubMed;
(b) G. Chawla, P. Gupta, R. Thilagavathi, A. K. Chakraborti and A. K. Bansal, Eur. J. Pharm. Sci., 2003, 20, 305 CrossRef CAS;
(c)
T. Mark, European patent application, EP 2 339 328 A2 Search PubMed;
(d)
L. J. Ferro, US patent, No. US 2004/0087640 A1 Search PubMed;
(e) J. F. Remenar, M. L. Peterson, P. W. Stephens, Z. Zhang, Y. Zimenkov and M. B. Hickey, Mol. Pharmaceutics, 2007, 4, 386 CrossRef CAS PubMed;
(f) J. F. Remenar, M. D. Tawa, M. L. Peterson, O. Almarsson, M. B. Hickeya and B. M. Foxman, CrystEngComm, 2011, 13, 1081 RSC.
-
(a) E. Elacqua, D. Bucar, R. F. Henry, G. G. Z. Zhang and L. R. MacGillivray, Cryst. Growth Des., 2013, 13, 393 CrossRef CAS;
(b) S. Ghosh, P. P. Bag and C. M. Reddy, Cryst. Growth Des., 2011, 11, 3489 CrossRef CAS.
- N. R. Goud, N. J. Babu and A. Nangia, Cryst. Growth Des., 2011, 11, 1930 CAS.
- N. R. Goud and A. Nangia, CrystEngComm, 2013, 15, 7456 RSC.
-
(a) G. R. Desiraju, Angew. Chem., Int. Ed. Engl., 1995, 34, 2311 CrossRef CAS;
(b) G. R. Desiraju, Angew. Chem., Int. Ed., 2007, 46, 8342 CrossRef CAS PubMed;
(c) G. R. Desiraju, J. Am. Chem. Soc., 2013, 135, 9952 CrossRef CAS PubMed.
-
http://www.fda.gov/Food/IngredientsPackagingLabeling/ GRAS/default.htm (accessed 31 July, 2013).
-
(a) D. Braga, G. Palladino, M. Polito, K. Rubini, F. Grepioni, M. R. Chierotti and R. Gobetto, Chem.–Eur. J., 2008, 14, 10149 CrossRef CAS PubMed;
(b) S. Aitipamula, P. S. Chow and R. B. H. Tan, CrystEngComm, 2009, 11, 1823 RSC.
-
(a) A. Mukherjee and G. R. Desiraju, Chem. Commun., 2011, 47, 4090 RSC;
(b) S. Aitipamula, P. S. Chow and R. B. H. Tan, CrystEngComm, 2009, 11, 889 RSC;
(c) S. Aitipamula, P. S. Chow and R. B. H. Tan, CrystEngComm, 2010, 12, 3691 RSC.
-
(a) F. L. Hirshfeld, Theor. Chim. Acta, 1977, 44, 129 CrossRef CAS;
(b) F. L. Hirshfeld and K. Mirsky, Acta Crystallogr., 1979, 35, 366 CrossRef;
(c) K. Mirsky, Acta Chim. Hung., 1993, 130, 197 CAS;
(d) M. A. Spackman and D. Jayatilaka, CrystEngComm, 2009, 11, 19 RSC;
(e) A. Parkin, G. Barr, W. Dong, C. J. Gilmore, D. Jayatilaka, J. J. McKinnon, M. A. Spackman and C. C. Wilson, CrystEngComm, 2007, 9, 648 RSC;
(f) J. J. McKinnon, A. S. Mitchell and M. A. Spackman, Chem.–Eur. J., 1998, 4, 2136 CrossRef CAS.
-
(a) G. M. Day, T. G. Cooper, A. J. Cruz-Cabeza, K. E. Hejczyk and H. L. Ammon,
et al.
, Acta Crystallogr., 2009, 65, 107 CAS;
(b) D. A. Bardwell, C. S. Adjiman, Y. A. Arnautova, E. Bartashevich and S. X. M. Boerrigter,
et al.
, Acta Crystallogr., 2011, 67, 535 CAS;
(c) A. J. C. Cabeza, G. M. Day, W. D. S. Motherwell and W. Jones, J. Am. Chem. Soc., 2006, 128, 14466 CrossRef CAS PubMed.
Footnote |
† Electronic supplementary information (ESI) available: Experimental procedures, spectral and thermal data, Hirshfeld plots. CCDC 954140–954145. For ESI and crystallographic data in CIF or other electronic format see DOI: 10.1039/c3ce41885e |
|
This journal is © The Royal Society of Chemistry 2014 |
Click here to see how this site uses Cookies. View our privacy policy here.