DOI:
10.1039/C4CC05108D
(Communication)
Chem. Commun., 2014,
50, 14870-14872
The hydrogen/deuterium isotope effect of the host material on the lifetime of organic light-emitting diodes†
Received
3rd July 2014
, Accepted 15th September 2014
First published on 15th September 2014
Abstract
The hydrogen/deuterium primary kinetic isotope effect provides useful information about the degradation mechanism of OLED host materials. Thus, replacement of labile C–H bonds in the host with C–D bonds increases the device lifetime by a factor of five without loss of efficiency, and replacement with C–C bonds by a factor of 22.5.
Organic light-emitting diodes (OLEDs) being innovative devices for display and illumination,1,2 their device lifetime and hence the mechanism of device degradation remain the most pressing subjects of research.3–5 Many of the studies reported thus far have focused on the analysis of the degradation products,6 while a few have reported on the kinetics of the molecular processes of the degradation.7 We focused on the hydrogen/deuterium (H/D) primary kinetic isotope effect (KIE) as a mechanistic probe, where the rate of a chemical reaction slows down by a factor of 6.5 (a theoretical value at 298 K)8 when the cleavage of the C–H(D) bond in question is the rate determining step in the overall reaction sequence.9–14 We report here a finding that deuteration of the benzylic methyl groups in a green phosphorescent host material (i.e., CH3CZBDF vs. CD3CZBDF in Fig. 1a) increases the device lifetime by a factor of five (from 0.2 h to 1.0 h) without affecting the other properties of the device much. This rate retardation factor of five is consistent with the KIE value reported for the heterolysis of a benzylic C–H bond.15 Taking together the isotope effect and the resonance-stabilizing effect of a furan ring (Fig. 1b), we consider the degradation of the methyl group in CH3CZBDF to be involved as a critical step of the degradation process; that is, the molecule is oxidized to a radical cation (i.e., hole formation) during operation of the OLED device and suffers from the loss of either a proton or a hydrogen radical. Guided by this analysis, we replaced the heterolytically labile C–H bonds in CH3CZBDF with more stable C–CH3 bonds (t-BuCZBDF) and found the lifetime to increase by a factor of 22.5 under the same device configuration. The lifetime enhancement by a factor of five through H to D change in the OLED performance makes an interesting contrast to a reported decrease of solar cell performance by 50% where no C–H(D) cleavage is involved.16 These data indicate that isotope effects are of considerable practical and mechanistic values in organic electronic research.17,18
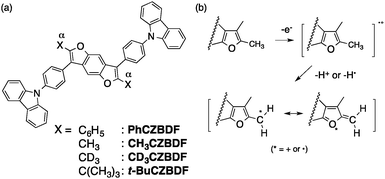 |
| Fig. 1 Host materials discussed in this study. (a) Chemical structures of CZBDF derivatives and (b) a plausible degradation path of CH3CZBDF. | |
The host material CH3CZBDF, which we focus on in this work, was designed on the basis of our previous host material PhCZBDF (Fig. 1a),19–21 whose triplet energy (T1) level of PhCZBDF, ca. 2.0 eV, was too low to be suitable as a host for green and blue phosphorescent OLEDs (PHOLEDs). We thus designed a less conjugated host CH3CZBDF in order to raise the T1 level (Fig. 1a; synthesis in ESI†), having a concern about the device durability due to the intrinsically labile benzylic methyl groups (cf.Fig. 1b). Thus, we also synthesized the deuterated (CD3CZBDF) and the tert-butyl analogues (t-BuCZBDF) for comparison.
We first found that CH3CZBDF and CD3CZBDF have essentially the same photophysical properties. This is reasonable because the C–H(D) bonds are not directly involved in the photoexcitation processes (see ESI,† Table S1). The ionization potentials (IPs) of CH3CZBDF and CD3CZBDF were the same (6.04 and 6.03 eV, respectively), as determined using photoemission yield spectroscopy (PYS).22 The IP of t-BuCZBDF was slightly lower (6.00 eV). The optical band gap (ΔEg) was the same for all compounds (3.49 eV). Using the IP and the ΔEg values, the electron affinities (EAs) were calculated to be 2.55, 2.54 and 2.51 eV for CH3CZBDF, CD3CZBDF and t-BuCZBDF, respectively, which are large enough to confine carriers within green phosphorescent Ir(ppy)323 (Fig. 2b). As determined by phosphorescence measurements at 77 K, the triplet energy levels (ET) are also high enough to suppress energy transfer from Ir(ppy)3 (ET = 2.4 eV) to the host materials: 2.64, 2.65 and 2.67 eV for CH3CZBDF, CD3CZBDF and t-BuCZBDF, respectively.
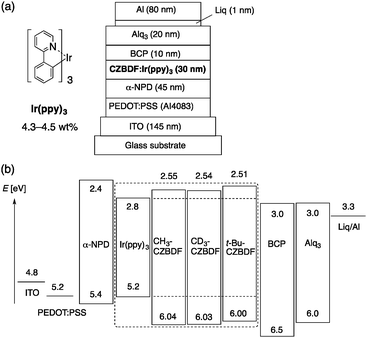 |
| Fig. 2 Materials and device structure of the CZBDF-based PHOLEDs. (a) Chemical structure of Ir(ppy)3 and device structure. (b) Energy diagram of the materials. | |
PHOLED devices were fabricated using alkyl-CZBDF as a host material in the emission layer doped with green phosphorescent Ir(ppy)3 with a standard heterojunction configuration as follows: ITO/PEDOT:PSS/α-NPD/alkyl-CZBDF:Ir(ppy)3/BCP/Alq3/Liq/Al, where PEDOT:PSS and α-NPD serve as hole injection and transport layers, BCP as a hole-blocking layer, and Alq3 and Liq as electron transport and injection layers, respectively (Fig. 2a). The PEDOT:PSS layer was spin-coated, and the other layers were deposited under vacuum. Electroluminescence from Ir(ppy)3 was observed from all devices (Fig. 3a), indicating that the carriers are appropriately confined within Ir(ppy)3.
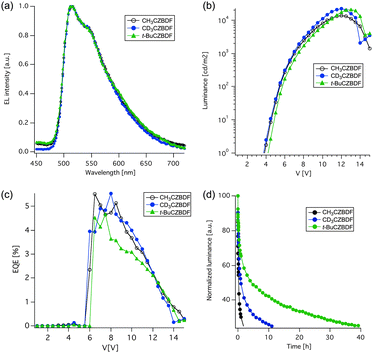 |
| Fig. 3 Device performance of the CZBDF-based PHOLEDs. (a) EL spectra. (b) L–V characteristics. (c) EQE–V characteristics. (d) Normalized L–t characteristics. | |
A comparison of the performances of the CH3CZBDF- and CD3CZBDF-based devices indicates that deuteration of the host material significantly enhanced the device stability without affecting the electroluminescence spectra (Fig. 3a) and the device efficiency (Fig. 3b and c). Thus, the driving voltage and luminance efficiency at 1000 cd m−2 (V1000 and η1000, respectively) and external quantum efficiency (EQE) barely changed (V1000 = 7.0 and 6.9 V; η1000 = 8.9 and 8.4 lm W−1; EQE = 5.5 and 5.5% for devices A and B, respectively; Table 1). The maximum luminance of the CD3CZBDF device was higher than that of the CH3CZBDF device, 22
270 and 13
800 cd m−2, respectively, because the former device degraded much less during the measurement. In consonance with this data, the half-life of the former was five times longer than the latter (1.0 h and 0.2 h, respectively, Fig. 3d and Table 1). This difference of stability is consistent with the reported KIE values for the heterolysis of a benzylic C–H bond,11,15 and hence suggests that the degradation of the α-methyl groups in CH3CZBDF (Fig. 1b) is a rate-determining step in this rapidly degrading device.
Table 1 Summary of the OLED performance dataa
Host |
V
1000 b [V] |
η
1000 c [lm W−1] |
EQEmax d [%] |
L
max e [cd A−1] |
τ
1/2 f [h] |
These data were collected at a luminance of 1000 cd m−2.
Driving voltage.
Luminance efficiency.
Maximum external quantum efficiency.
Maximum luminance.
Device half-lifetime measured at a constant current density of 12.5 mA cm−2.
|
CH3CZBDF |
7.0 |
8.9 |
5.5 |
13 800 |
0.2 |
CD3CZBDF |
6.9 |
8.4 |
5.5 |
22 270 |
1.0 |
t-BuCZBDF |
7.4 |
6.1 |
4.5 |
20 770 |
4.5 |
We next considered the possibility that the conversion of the α-C–H bonds into heterolytically more stable C–C bonds will further elongate the device lifetime (Fig. 1a). Consistent with this expectation, the t-BuCZBDF device achieved a 22.5 times longer lifetime (Fig. 3d, Table 1) and higher maximum luminance (20
770 cd m−2) than the CH3CZBDF-based device. There were slight increases in the driving voltage (7.4 V) and lower efficiencies (η1000 = 6.1 lm W−1, EQE = 4.5%), which was possibly caused by the difference of molecular packing.24 In a similar vein, a similar device using PhCZBDF (having a heterolytically stable phenyl–furyl bond) has a very long lifetime of >4000 h.19
In conclusion, we have shown that the hydrogen/deuterium exchange of a heterolytically labile C–H bond in an OLED host material increases the lifetime of the device. The deuterium atoms in CD3CZBDF did not change the device performance much except for the lifetime (and the maximum luminance which is related to the lifetime), because the photophysical and electrical processes as well as morphological changes do not include C–H(D) bond cleavage (cf. secondary kinetic isotope effects). Identification of the heterolytically labile C–H bonds in CH3CZBDF then led us to replace these bonds with C–C bonds to achieve a further increase in the lifetime. We expect that some of the data reported in the literature and patents on the favourable effects of deuterated materials may be rationalized in terms of the kinetic and equilibrium isotope effects.
We thank MEXT for financial support (KAKENHI for H.T., No. 20685005). E.N. thanks the Strategic Promotion of Innovation Research and Development from the Japan Science and Technology Agency (JST).
Notes and references
- C. W. Tang and S. A. VanSlyke, Appl. Phys. Lett., 1987, 51, 913 CrossRef CAS.
-
(a)
K. Müllen and U. Scherf, Organic Light-Emitting Devices: Synthesis, Properties and Applications, Wiley-VCH, 2006 Search PubMed;
(b)
J. Shinar, Organic Light-Emitting Devices: A Survey, Springer, 2004 Search PubMed;
(c)
Z. Li and H. Meng, Organic Light-Emitting Materials and Devices, CRC Press, 2007 Search PubMed;
(d)
Y. Sato, in Electroluminescence I, ed. G. Mueller, Academic Press, San Diego, 2000, vol. 64, pp. 209–254 Search PubMed;
(e) Y. Sato, S. Ichinosawa and H. Kanai, IEEE J. Sel. Top. Quantum Electron., 1998, 4, 40–48 CrossRef CAS.
- For reviews:
(a) H. Aziz and Z. D. Popovic, Chem. Mater., 2004, 16, 4522 CrossRef CAS;
(b) F. So and D. Kondakov, Adv. Mater., 2010, 22, 3762 CrossRef CAS PubMed.
-
(a) H. Aziz, Z. D. Popovic, N.-X. Hu, A.-M. Hor and G. Xu, Science, 1999, 283, 1900 CrossRef CAS PubMed;
(b) Y. Luo, H. Aziz, G. Xu and Z. D. Popovic, Chem. Mater., 2007, 19, 2079 CrossRef CAS.
- For a recent review: A. Chaskar, H.-F. Chen and K.-T. Wong, Adv. Mater., 2011, 23, 3876 CrossRef CAS PubMed.
-
(a) D. Y. Kondakov, W. C. Lenhart and W. F. Nichols, J. Appl. Phys., 2007, 101, 024512 CrossRef;
(b) S. Scholz, K. Walzer and K. Leo, Adv. Funct. Mater., 2008, 18, 2541 CrossRef CAS;
(c) V. Sivasubramaniam, F. Brodkorb, S. Hanning, O. Buttler, H. P. Loebl, V. van Elsbergen, H. Boerner, U. Scherf and M. Kreyenschmidt, Solid State Sci., 2009, 11, 1933 CrossRef CAS;
(d) I. R. de Moraes, S. Scholz, B. Lüssem and K. Leo, Org. Electron., 2011, 12, 341 CrossRef;
(e) I. R. de Moraes, S. Scholz, B. Lüssem and K. Leo, Org. Electron., 2012, 13, 1900 CrossRef.
-
(a) N. C. Giebink, B. W. D'Andrade, M. S. Weaver, P. B. Mackenzie, J. J. Brown, M. E. Thompson and S. R. Forrest, J. Appl. Phys., 2008, 103, 044509 CrossRef;
(b) N. C. Giebink, B. W. D'Andrade, M. S. Weaver, J. J. Brown and S. R. Forrest, J. Appl. Phys., 2009, 105, 124514 CrossRef.
- Estimated using a well-established equation for kinetic isotope effect: kH/kD = exp[hc(νH − νD)/2kT], where νH and νD stands for stretching frequencies of C–H (∼3000 cm−1) and C–D (∼2100 cm−1), respectively.
- N. Yoshikai, H. Matsuda and E. Nakamura, J. Am. Chem. Soc., 2008, 130, 15258 CrossRef CAS PubMed.
-
(a) K. B. Wiberg, Chem. Rev., 1955, 55, 713 CrossRef CAS;
(b) F. H. Webtheimer, Chem. Rev., 1955, 55, 713 CrossRef.
- For recent reviews on chemical reactions:
(a) E. M. Simmons and J. F. Hartwig, Angew. Chem., Int. Ed., 2012, 51, 3066 CrossRef CAS PubMed;
(b) T. Giagou and M. P. Meyer, Chem. – Eur. J., 2010, 16, 10616 CrossRef CAS PubMed;
(c) W. D. Jones, Acc. Chem. Res., 2003, 36, 140 CrossRef CAS PubMed;
(d) M. Gómez-Gallego and M. A. Sierra, Chem. Rev., 2011, 111, 4857 CrossRef PubMed.
- For recent reviews on energy conversion:
(a) S. Fukuzumi, Y. Yamada, T. Suenobu, K. Ohkubo and H. Kotani, Energy Environ. Sci., 2011, 4, 2754 RSC;
(b) S. Hammes-Schiffer, Acc. Chem. Res., 2009, 42, 1881 CrossRef CAS PubMed.
- For recent reviews on biological process:
(a) W. W. Cleland, Arch. Biochem. Biophys., 2005, 433, 2 CrossRef CAS PubMed;
(b) D. Antoniou, S. Caratzoulas, C. Kalyanaraman, J. S. Mincer and S. D. Schwartz, Eur. J. Biochem., 2002, 269, 3103 CrossRef CAS PubMed.
- T. D. Nguyen, G. Hukic-Markosian, F. Wang, L. Wojcik, X.-G. Li, E. Ehrenfreund and Z. V. Vardeny, Nat. Mater., 2010, 9, 345 CrossRef CAS PubMed.
-
(a) K. B. Wiberg and L. H. Slaugh, J. Am. Chem. Soc., 1958, 80, 3033 CrossRef CAS;
(b) I. Howe and F. W. McLaffert, J. Am. Chem. Soc., 1971, 93, 99 CrossRef.
- M. Shao, J. Keum, J. Chen, Y. He, W. Chen, J. F. Browning, J. Jakowski, B. G. Sumpter, I. N. Ivanov, Y.-Z. Ma, C. M. Rouleau, S. C. Smith, D. B. Geohegan, K. Hong and K. Xiao, Nat. Commun., 2014, 5, 3180 Search PubMed.
- For deuterium effect on functional materials and enhancement of OLED efficiency;
(a) C. C. Tong and K. C. Hwang, J. Phys. Chem. C, 2007, 111, 3490 CrossRef CAS;
(b) J.-L. Zuo, J.-X. Yang, F.-Z. Wang, X.-N. Dang, J.-L. Sun, D.-C. Zoub, Y.-P. Tian, N. Lin, X.-T. Tao and M.-H. Jiang, J. Photochem. Photobiol., A, 2008, 199, 322 CrossRef CAS;
(c) T. D. Nguyen, G. Hukic-Markosian, F. Wang, L. Wojcik, X.-G. Li, E. Ehrenfreund and Z. V. Vardeny, Nat. Mater., 2010, 9, 345 CrossRef CAS PubMed.
- For deuterium effect on iridium complexes:
(a) T. Abe, A. Miyazawa, H. Konno and Y. Kawanishi, Chem. Phys. Lett., 2010, 491, 199 CrossRef CAS;
(b) P. Wang, F.-F. Wang, Y. Chen, Q. Niu, L. Lu, H.-M. Wang, X.-C. Gao, B. Wei, H.-W. Wu, X. Caic and D.-C. Zou, J. Mater. Chem. C, 2013, 1, 4821 RSC.
-
(a) H. Tsuji, C. Mitsui, L. Ilies, Y. Sato and E. Nakamura, J. Am. Chem. Soc., 2007, 129, 11902 CrossRef CAS PubMed;
(b) H. Tsuji, C. Mitsui, Y. Sato and E. Nakamura, Adv. Mater., 2009, 21, 3776 CrossRef CAS;
(c) C. Mitsui, H. Tanaka, H. Tsuji and E. Nakamura, Chem. – Asian J., 2011, 6, 2296 CrossRef CAS PubMed;
(d) C. Mitsui, H. Tsuji, Y. Sato and E. Nakamura, Chem. – Asian J., 2012, 7, 1443 CrossRef CAS PubMed.
- Fused furan materials reported by ourselves:
(a) H. Tsuji, C. Mitsui, Y. Sato and E. Nakamura, Heteroat. Chem., 2011, 22, 316 CrossRef CAS;
(b) C. Mitsui, J. Soeda, K. Miwa, H. Tsuji, J. Takeya and E. Nakamura, J. Am. Chem. Soc., 2012, 134, 5448 CrossRef CAS PubMed;
(c) H. Tsuji, L. Ilies and E. Nakamura, Synlett, 2014, 25, 2099 CrossRef CAS.
- Furan-containing functional materials:
(a) R. Shukla, S. H. Wadumethrige, S. V. Lindeman and R. Rathore, Org. Lett., 2008, 10, 3587 CrossRef CAS PubMed;
(b) N. Hayashi, Y. Saito, H. Higuchi and K. Suzuki, J. Phys. Chem. A, 2009, 113, 5342 CrossRef CAS PubMed;
(c) U. Caruso, B. Panunzi, G. N. Roviello, G. Roviello, M. Tingoli and A. Tuzi, C. R. Chim., 2009, 12, 622 CrossRef CAS;
(d) H. Li, P. Jiang, C. Yi, C. Li, S.-X. Liu, S. Tan, B. Zhao, J. Braun, W. Meier, T. Wandlowski and S. Decurtins, Macromolecules, 2010, 43, 8058 CrossRef CAS;
(e) J. Santos-Pérez, C. E. Crespo-Hernández, C. Reichardt, C. R. Cabrera, I. Feliciano-Ramos, L. Arroyo-Ramírez and M. A. Meador, J. Phys. Chem. A, 2011, 115, 4157 CrossRef PubMed;
(f) J. Xiao, B. Yang, J. I. Wong, Y. Liu, F. Wei, K. J. Tan, X. Teng, Y. Wu, L. Huang, C. Kloc, F. Boey, J. Ma, H. Zhang, H. Y. Yang and Q. Zhang, Org. Lett., 2011, 13, 3004 CrossRef CAS PubMed;
(g) J. C. Bijleveld, B. P. Karsten, S. G. J. Mathijssen, M. M. Wienk, D. M. de Leeuw and R. A. J. Janssen, J. Mater. Chem., 2011, 21, 1600 RSC;
(h) C. Moussallem, F. Gohier, C. Mallet, M. Allain and P. Frère, Tetrahedron, 2012, 68, 8617 CrossRef CAS;
(i) K. Niimi, H. Mori, E. Miyazaki, I. Osaka, H. Kakizoe, K. Takimiya and C. Adachi, Chem. Commun., 2012, 48, 5892 RSC;
(j) B. M. Kobilka, A. V. Dubrovskiy, M. D. Ewan, A. L. Tomlinson, R. C. Larock, S. Chaudhary and M. Jeffries-El, Chem. Commun., 2012, 48, 8919 RSC;
(k) H. Li, P. Tang, Y. Zhao, S.-X. Liu, Y. Aeschi, L. Deng, J. Braun, B. Zhao, Y. Liu, S. Tan, W. Meier and S. Decurtins, J. Polym. Sci., Part A: Polym. Chem., 2012, 50, 2935 CrossRef CAS;
(l) C. Mitsui, T. Okamoto, H. Matsui, M. Yamagishi, T. Matsushita, J. Soeda, K. Miwa, H. Sato, A. Yamano, T. Uemura and J. Takeya, Chem. Mater., 2013, 25, 3952 CrossRef CAS;
(m) K. Nakahara, C. Mitsui, T. Okamoto, M. Yamagishi, K. Miwa, H. Sato, A. Yamano, T. Uemura and J. Takeya, Chem. Lett., 2013, 42, 654 CrossRef CAS;
(n) H. Li, J. Ding, S. Chen, C. Beyer, S.-X. Liu, H.-A. Wagenknecht, A. Hauser and S. Decurtins, Chem. – Eur. J., 2013, 19, 6459 CrossRef CAS PubMed;
(o) S. Li, J. Yuan, P. Deng, W. Ma and Q. Zhang, Sol. Energy Mater. Sol. Cells, 2013, 118, 22 CrossRef CAS;
(p) M. J. Bosiak, M. Rakowiecki, K. J. Orlowska, D. Kedziera and J. Adams, Dyes Pigm., 2013, 99, 803 CrossRef CAS;
(q) O. Gidron, A. Dadvand, E. W.-H. Sun, I. Chung, L. J. W. Shimon, M. Bendikov and D. F. Perepichka, J. Mater. Chem. C, 2013, 1, 4358 RSC;
(r) C. Mallet, Y. Didane, T. Watanabe, N. Yoshimoto, M. Allain, C. Videlot-Ackermann and P. Frère, ChemPlusChem, 2013, 78, 459 CrossRef CAS;
(s) S. Shi, X. Xie, C. Gao, K. Shi, S. Chen, G. Yu, L. Guo, X. Li and H. Wang, Macromolecules, 2014, 47, 616 CrossRef CAS;
(t) C. Moussalem, O. Segut, F. Gohier, M. Allain and P. Frére, ACS Sustainable Chem. Eng., 2014, 2, 1043 CrossRef CAS;
(u) W. Huang, B. Yang, J. Sun, B. Liu, J. Yang, Y. Zou, J. Xiong, C. Zhou and Y. Gao, Org. Electron., 2014, 15, 1050 CrossRef CAS;
(v) K. Nakahara, C. Mitsui, T. Okamoto, M. Yamagishi, H. Matsui, T. Ueno, Y. Tanaka, M. Yano, T. Matsushita, J. Soeda, Y. Hirose, H. Sato, A. Yamano and J. Takeya, Chem. Commun., 2014, 50, 5342 RSC;
(w) Z. Li, H. Li, S. Chen, T. Froehlich, C. Yi, C. Schönenberger, M. Calame, S. Decurtins, S.-X. Liu and E. Borguet, J. Am. Chem. Soc., 1014, 136, 8867 CrossRef PubMed;
(x) C. G. Péterfalvi, I. Grace, D. Z. Manrique and C. J. Lambert, J. Chem. Phys., 2014, 140, 174711 CrossRef PubMed;
(y) T. Kojima, R. Yokota, C. Kitamura, H. Kurata, M. Tanaka, H. Ikeda and T. Kawase, Chem. Lett., 2014, 43, 696 CrossRef CAS.
- M. Honda, K. Kanai, K. Komatsu, Y. Ouchi, H. Ishii and K. Seki, Mol. Cryst. Liq. Cryst., 2006, 455, 219 CrossRef CAS.
-
(a) C. Adachi, M. A. Baldo and S. R. Forrest, Appl. Phys. Lett., 2000, 77, 904 CrossRef CAS;
(b) T. Hofbeck and H. Yersin, Inorg. Chem., 2010, 49, 9290 CrossRef CAS PubMed.
- H. Tsuji, G. Cantagrel, Y. Ueda, T. Chen, L.-J. Wan and E. Nakamura, Chem. – Asian J., 2013, 8, 2377 CrossRef CAS PubMed.
Footnote |
† Electronic supplementary information (ESI) available: Synthetic details, summary of physical properties of materials and device fabrication. See DOI: 10.1039/c4cc05108d |
|
This journal is © The Royal Society of Chemistry 2014 |
Click here to see how this site uses Cookies. View our privacy policy here.