DOI:
10.1039/C4CC04555F
(Communication)
Chem. Commun., 2014,
50, 9469-9472
Facile regiospecific synthesis of 2,3-disubstituted indoles from isatins†
Received
16th June 2014
, Accepted 4th July 2014
First published on 4th July 2014
Abstract
A facile regiospecific synthesis of 2,3-disubstituted indoles from isatins has been developed. Nucleophilic addition of Grignard reagents to commercially available isatins, followed by reduction with borane, afforded an array of structurally diverse 2,3-disubstituted indoles in moderate to good yields. The method described herein represents a novel and very simple approach to synthesize various 2,3-disubstituted indoles, extremely important structural motifs in the pharmaceutical industry and medicinal chemistry.
Indole represents one of the most important structural units of natural products and pharmaceutical agents,1 and continuous efforts have been devoted to the efficient synthesis of indole structures over the past century.2 Fisher indole synthesis, although discovered more than 100 years ago, still remains one of the most widely used methods.3 Many transition metal-based approaches have emerged over the past few decades, including Larock aniline–alkyne cyclizations,4 C–H activation strategies,5 and cross-dehydrogenative coupling methods,6 among others (Scheme 1). Despite the aforementioned impressive progress, straightforward and efficient methods without employing a transition metal are still highly desired. Given the importance of 2,3-disubstituted indoles in biological sciences and medicinal chemistry (Fig. 1),7 we set out to design an efficient method to access such structural motifs. The vast majority of known methods rely on the annulation of a five-membered ring to an existing benzene ring for the construction of the indole core, and synthesis of specific precursors containing the right functionalities can pose huge synthetic problems. Moreover, formation of mixed regioisomers at the 2- and 3-positions is often problematic in the above approaches. In our design, we set the following criteria for starting materials to ensure an efficient indole synthesis: (1) ready availability, (2) the existing fused ring structures, (3) latent indole precursors, and (4) easy functionalization to allow access to different 2,3-substituted indoles. Commercially available isatins seem to be excellent candidates fulfilling all the above requirements. Nucleophilic additions to the carbonyl groups at 2- and 3-positions could yield 2,3-disubstituted intermediates. Moreover, the carbonyl groups at 2- and 3-positions of isatins have different electrophilic properties, and thus careful differentiation of these groups is expected to make selective functionalizations possible, thus introducing different substituents at 2- and 3-positions. Finally, using a suitable reduction protocol,8 various 2,3-disubstituted indoles can be readily created (Scheme 2).
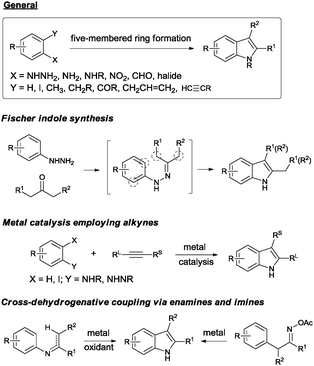 |
| Scheme 1 Common methods for indole synthesis. | |
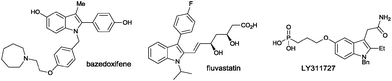 |
| Fig. 1 Selected bioactive compounds containing a 2,3-disubstituted indole moiety. | |
 |
| Scheme 2 Synthesis of 2,3-disubstituted indoles from isatins. | |
We first tested the feasibility of forming indoles from isatins, installing the same substituent at the 2- and 3-positions. The methyl group was introduced via a methyl Grignard reagent, and subsequent reduction with borane yielded the indole products (Table 1). We initially employed 5 equivalents of the Grignard reagent as well as a large excess of borane (7 eq.) to ensure high conversion of the reaction. When unprotected isatin (1a) was used, only a 3-monosubstituted indole product (2a-1′) was obtained in low yield, (entry 1). When Boc-protected isatin (3a) was used, the desired indole product was virtually not formed (entry 2). Switching the N-protection to an alkyl group proved to be effective, and the desired 2,3-disubstituted indole was obtained in high yield when N-methyl or N-benzyl isatin was used for the reaction (entries 3 and 4). However, it was observed that 3-monosubstituted indole was also formed during the reaction, which could not be easily separated from the desired disubstituted product via chromatographic separation. We reasoned that varying the molar equivalence of the Grignard reagent and borane might be the key to solving this problem. Therefore, further optimizations were carried out. When the amount of borane was reduced to 3 equivalents, the 2,3-disubstituted indole 2a was obtained as the sole product in moderate yield (entry 6). The chemical yield was easily improved to 86% with slightly increased reaction time (entries 7 and 8). Further experimentations revealed the best reaction conditions; in the presence of 4 equivalents of the Grignard reagent and 2 equivalents of borane, the desired 2,3-disubstituted indoles were formed in high yields (entry 11).9
Table 1 Investigating the synthesis of 2,3-disubstituted indoles from isatinsa
By utilizing the above optimal reaction conditions, we prepared a number of symmetric 2,3-disubstituted indoles from isatins (Scheme 3). Various alkyl and aryl substituents were easily installed at the 2- and 3-positions with employment of different Grignard reagents10 (2a–2f). Furthermore, indole core structures could also be varied if different isatins were utilized as starting materials (2g and 2h).
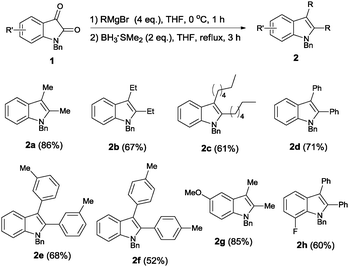 |
| Scheme 3 Substrate scope for synthesis of symmetric 2,3-disubstituted-indoles. | |
Our next goal was to regiospecifically synthesize 2,3-disubstituted indoles bearing different substituents. This is a formidable synthetic task, and previous literature efforts only met with limited success.11 We reasoned that this challenging regioselectivity issue may be easily addressed via stepwise functionalization of the indole precursor, taking advantage of the difference in electrophilicity of the 2- and 3-carbonyl groups of isatins. Treatment of N-benzyl isatins with the Grignard reagent at low temperature yielded 3-hydroxy-3-substituted oxindole substrates 3,12 which were then subjected to the second functionalization at the 2-carbonyl position. The reaction was conducted in the presence of 4 equivalents of the Grignard reagent and 2 equivalents of borane, and the results are summarized in Scheme 4. 2,3-Disubstituted indoles with different substitution patterns were easily prepared, including: 2-alkyl-3-alkyl (4a–4d), 2-aryl-3-alkyl (4e–4h), 2-alkyl-3-aryl (4i–4l), and 2-aryl-3-aryl (4m–4p) substituents. Moreover, different indole cores could be obtained if different isatins were utilized as the starting materials (4q–4t). All the products were readily prepared, within a few hours, in moderate to good chemical yields. It is noteworthy that our method allowed convenient preparation of 2,3-disubstituted indoles in a regiospecific manner, even though the substituents may have great similarity both sterically and electronically. For instance, 2-methyl-3-ethyl-indole 4a and 2-ethyl-3-methyl-indole 4b were prepared in high yields, both as a pure regioisomer, as opposed to the formation of mixed isomers reported in the literature.13 The 2,3-disubstituted indoles could also be synthesized via a one-pot protocol. Isatin 1a was treated with different Grignard reagents in a stepwise fashion, the phenyl group was first introduced into the 3-position, followed by 2-carbonyl functionalization with another Grignard reagent. Finally, the desired product was obtained upon borane reduction (eqn (1)).
| 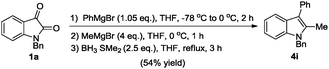 | (1) |
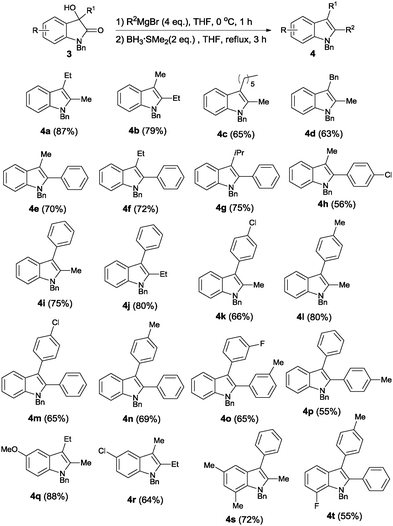 |
| Scheme 4 Regiospecific synthesis of 2,3-disubstituted-indoles bearing different substituents. | |
The synthetic value of our method was demonstrated by preparing LY311727, the first potent and selective s-PLA2 inhibitor.7b 3-Hydroxy oxindole substrate 6 was easily derived from isatin 5 (see the ESI† for details). Treatment of 6 with ethylmagnesium bromide led to the formation of indoline 7, instead of the corresponding indole intermediate. Indole 8 was obtained via a mesylation–elimination reaction.14 Removal of the silyl protection afforded the key intermediate 9, which can be converted into LY311727 using the procedures described in the literature (Scheme 5).
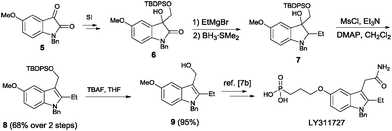 |
| Scheme 5 A formal synthesis of LY311727. | |
Unprotected indole products could be easily obtained after the N-debenzylation reaction. The benzyl group of 4o could be readily removed by treating it with potassium tert-butoxide and oxygen in DMSO/THF at room temperature, and the corresponding unprotected indole 10 was obtained in high yield (eqn (2)).15
| 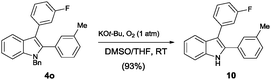 | (2) |
A plausible reaction pathway is illustrated in Scheme 6. Treatment of isatin 1a with a Grignard reagent yields intermediate A. Excess Grignard reagent leads to the formation of intermediate B. Upon the elimination of OMgBr−, iminium intermediate C is generated, which is further reduced to indoline intermediate D. The final indole product 4 is then generated upon subsequent elimination of Mg(OH)Br.
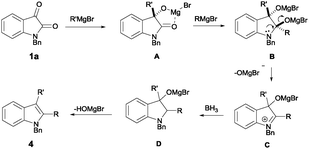 |
| Scheme 6 Proposed reaction pathway. | |
In summary, we have successfully developed a novel method for regiospecific preparation of 2,3-disubstituted indoles. Our method makes use of commercially available isatins as starting materials, and employs readily available Grignard reagents as nucleophilic partners and borane as a convenient reducing agent. Stepwise functionalization of the 2- and 3-carbonyl groups of isatins is the key to the introduction of different substituents regiospecifically at the 2- and 3-positions of the indole products. Extension of the approach described here to the synthesis of other important heterocyclic compounds is ongoing in our laboratory.
Notes and references
-
(a) M. Inman and C. J. Moody, Chem. Sci., 2013, 4, 29 RSC
;
(b) A. J. Kochanowska-Karamyan, Chem. Rev., 2010, 110, 4489 CrossRef CAS PubMed
;
(c) T. Kawasaki and K. Higuchi, Nat. Prod. Rep., 2007, 24, 843 RSC
;
(d) T. Kawasaki and K. Higuchi, Nat. Prod. Rep., 2005, 22, 761 RSC
;
(e) M. Somei and F. Yamada, Nat. Prod. Rep., 2005, 22, 73 RSC
;
(f) M. Somei and F. Yamada, Nat. Prod. Rep., 2004, 21, 278 RSC
;
(g)
R. J. Sundberg, Indoles, Academic Press, San Diego, 1996 Search PubMed
.
- For recent reviews on indole synthesis, see:
(a) M. Shiri, Chem. Rev., 2012, 112, 3508 CrossRef CAS PubMed
;
(b) S. Cacchi and G. Fabrizi, Chem. Rev., 2011, 111, PR215 CrossRef PubMed
;
(c) D. F. Taber and P. K. Tirunahari, Tetrahedron, 2011, 67, 7195 CrossRef CAS PubMed
;
(d) R. Vicente, Org. Biomol. Chem., 2011, 9, 6469 RSC
;
(e) K. Krüger, A. Tillack and M. Beller, Adv. Synth. Catal., 2008, 350, 2153 CrossRef
;
(f) G. R. Humphrey and J. T. Kuethe, Chem. Rev., 2006, 106, 2875 CrossRef CAS PubMed
;
(g) S. Cacchi and G. Fabrizi, Chem. Rev., 2005, 105, 2873 CrossRef CAS PubMed
;
(h) G. Bartoli, R. Dalpozzo and M. Mardi, Chem. Soc. Rev., 2014, 43, 4728 RSC
.
- For reviews on the Fischer indole synthesis, see:
(a) D. L. Hughes, Org. Prep. Proced. Int., 1993, 25, 607 CrossRef CAS
;
(b)
B. Robinson, The Fischer Indole Synthesis Wiley-Interscience, New York, 1982 Search PubMed
;
(c) B. Robinson, Chem. Rev., 1969, 69, 227 CrossRef CAS
;
(d) B. Robinson, Chem. Rev., 1963, 63, 373 CrossRef
. For selected recent modifications, see: ;
(e) M. Inman, A. Carbone and C. J. Moody, J. Org. Chem., 2012, 77, 1217 CrossRef CAS PubMed
;
(f) M. Inman and C. J. Moody, Chem. Commun., 2011, 47, 788 RSC
;
(g) B. A. Haag, Z.-G. Zhang, J.-S. Li and P. Knochel, Angew. Chem., Int. Ed., 2010, 49, 9513 CrossRef CAS PubMed
;
(h) K. Alex, A. Tillack, N. Schwarz and M. Beller, Angew. Chem., Int. Ed., 2008, 47, 2304 CrossRef CAS PubMed
;
(i) C. Cao, Y. Shi and A. L. Odom, Org. Lett., 2002, 4, 2853 CrossRef CAS PubMed
;
(j) S. Wagaw, B. H. Yang and S. L. Buchwald, J. Am. Chem. Soc., 1998, 120, 6621 CrossRef CAS
.
-
(a) G. Zeni and R. C. Larock, Chem. Rev., 2006, 106, 4644 CrossRef CAS PubMed
;
(b) R. C. Larock and E. K. Yum, J. Am. Chem. Soc., 1991, 113, 6689 CrossRef CAS
;
(c) R. C. Larock, E. K. Yum and M. D. Refvik, J. Org. Chem., 1998, 63, 7652 CrossRef CAS
.
- For representative examples, see:
(a) D. Zhao, Z. Shi and F. Glorius, Angew. Chem., Int. Ed., 2013, 52, 12426 CrossRef CAS PubMed
;
(b) C. Wang, H. Sun, Y. Fang and Y. Huang, Angew. Chem., Int. Ed., 2013, 52, 5795 CrossRef CAS PubMed
;
(c) B. Liu, C. Song, C. Sun, S. Zhou and J. Zhu, J. Am. Chem. Soc., 2013, 135, 16625 CrossRef CAS PubMed
;
(d) C. Wang and Y. Huang, Org. Lett., 2013, 15, 5294 CrossRef CAS PubMed
;
(e) M. P. Huestis, L. Chan, D. R. Stuart and K. Fagnou, Angew. Chem., Int. Ed., 2011, 50, 1338 CrossRef CAS PubMed
;
(f) D. R. Stuart, P. Alsabeh, M. Kuhn and K. Fagnou, J. Am. Chem. Soc., 2010, 132, 18326 CrossRef CAS PubMed
;
(g) D. R. Stuart, M. Bertrand-Laperle, K. M. N. Burgess and K. Fagnou, J. Am. Chem. Soc., 2008, 130, 16474 CrossRef CAS PubMed
. For palladium catalyzed reactions, see: ;
(h) S. P. Breazzano, Y. B. Poudel and D. L. Boger, J. Am. Chem. Soc., 2013, 135, 1600 CrossRef CAS PubMed
;
(i) Z. Shi, C. Zhang, S. Li, D. Pan, S. Ding, Y. Cui and N. Jiao, Angew. Chem., Int. Ed., 2009, 48, 4572 CrossRef CAS PubMed
.
- For a highlight, see:
(a) Z. Shi and F. Glorius, Angew. Chem., Int. Ed., 2012, 51, 9220 CrossRef CAS PubMed
. For palladium catalyzed reactions, see: ;
(b) Y. Wei, I. Deb and N. Yoshikai, J. Am. Chem. Soc., 2012, 134, 9098 CrossRef CAS PubMed
;
(c) T. Nanjo, C. Tsukano and Y. Takemoto, Org. Lett., 2012, 14, 4270 CrossRef CAS PubMed
;
(d) J. J. Neumann, S. Rakshit, T. Dröge, S. Würtz and F. Glorius, Chem. – Eur. J., 2011, 17, 7298 CrossRef CAS PubMed
;
(e) Y. Tan and J. F. Hartwig, J. Am. Chem. Soc., 2010, 132, 3676 CrossRef CAS PubMed
;
(f) S. Würtz, S. Rakshit, J. J. Neumann, T. Dröge and F. Glorius, Angew. Chem., Int. Ed., 2008, 47, 7230 CrossRef PubMed
. For other metal catalyzed reactions, see: ;
(g) A. Gogoi, S. Guin, S. K. Rout and B. K. Patel, Org. Lett., 2013, 15, 1802 CrossRef CAS PubMed
;
(h) B. Lu, Y. Luo, L. Liu, L. Ye, Y. Wang and L. Zhang, Angew. Chem., Int. Ed., 2011, 50, 8358 CrossRef CAS PubMed
;
(i) Z.-H. Guan, Z.-Y. Yan, Z.-H. Ren, X.-Y. Liu and Y.-M. Liang, Chem. Commun., 2010, 46, 2823 RSC
;
(j) R. Bernini, G. Fabrizi, A. Sferrazza and S. Cacchi, Angew. Chem., Int. Ed., 2009, 48, 8078 CrossRef CAS PubMed
;
(k) M. Shen, B. E. Leslie and T. G. Driver, Angew. Chem., Int. Ed., 2008, 47, 5056 CrossRef CAS PubMed
. For a metal-free version, see: ;
(l) W. Yu, Y. Du and K. Zhao, Org. Lett., 2009, 11, 2417 CrossRef CAS PubMed
.
-
(a) K. Alex, N. Schwarz, V. Khedkar, I. A. Sayyed, A. Tillack, D. Michalik, J. Holenz, J. L. Diaz and M. Beller, Org. Biomol. Chem., 2008, 11, 2417 Search PubMed
;
(b) J. M. Paolak, V. V. Khau, D. R. Hutchison and M. J. Martinelli, J. Org. Chem., 1996, 61, 9055 CrossRef
;
(c) A. Arcadi, S. Cacchi, V. Carnicelli and F. Marinelli, Tetrahedron, 1994, 50, 437 CrossRef CAS
. Also see ref. 1a, 2b,e.
- For reduction of oxindole to indole, see:
(a) W. Wierenga, J. Griffin and M. A. Warpehoski, Tetrahedron Lett., 1983, 24, 2437 CrossRef CAS
;
(b) S. J. Garden, R. B. da Silva and A. C. Pinto, Tetrahedron, 2002, 58, 8399 CrossRef CAS
.
- The first nucleophilic addition of a Grignard reagent to the 3-carbonyl group proceeds readily and quantitatively. The second nucleophilic addition of Grignard to the 2-carbonyl group is more difficult, due
to lower electrophilicity of the amide function. In the presence of excess borane, reduction of the amide carbonyl competes with nucleophilic addition, accounting for the formation of 3-monosubstituted indoles.
- When vinylmganesium bromide was used under the optimized reaction conditions, no desired product was observed.
- Only a handful of examples have described the synthesis of asymmetric 2,3-disubstituted indoles, see:
(a) B. Yao, Q. Wang and J. Zhu, Angew. Chem., Int. Ed., 2012, 51, 12311 CrossRef CAS PubMed
;
(b) S. Cacchi, G. Fabrizi, A. Goggiamani, A. Perboni, A. Sferrazza and P. Staqbile, Org. Lett., 2010, 12, 3279 CrossRef CAS PubMed
;
(c) B. Z. Lu, W. Zhao, H.-X. Wei, M. Dufour, V. Farina and C. H. Senanayake, Org. Lett., 2006, 8, 3271 CrossRef CAS PubMed
;
(d) H. Tokuyama, T. Yamashita, M. T. Reding, Y. Kaburagi and T. Fukuyama, J. Am. Chem. Soc., 1999, 121, 3791 CrossRef CAS
;
(e) T. Fukuyama, X. Chen and G. Peng, J. Am. Chem. Soc., 1994, 116, 3127 CrossRef CAS
;
(f) K.-Y. Ye, L.-X. Dai and S.-L. You, Chem. – Eur. J., 2014, 20, 3040 CrossRef CAS PubMed
. Also see some examples in ref. 5a,b,d,e.
- For preparation of 3-hydroxy-3-substituted oxindole from isatins, see: B. M. Trost, J. Xie and J. D. Sieber, J. Am. Chem. Soc., 2011, 133, 20611 CrossRef CAS PubMed
, and the ESI† of this article.
- A 3
:
2 mixture was obtained in Larock indole synthesis, see: R. C. Larock, E. K. Yum and M. D. Refvik, J. Org. Chem., 1998, 63, 7652 CrossRef CAS
.
- Formation of the indole core via dehydration of an indoline intermediate did not occur spontaneously under standard reaction conditions, which may be due to the formation of a stable five-membered intermediate resulting from coordination of magnesium with the two oxygen atoms at the 3-position.
- A. A. Haddach, A. Kelleman and M. V. Deaton-Rewolinski, Tetrahedron Lett., 2003, 43, 399 CrossRef
.
Footnote |
† Electronic supplementary information (ESI) available. See DOI: 10.1039/c4cc04555f |
|
This journal is © The Royal Society of Chemistry 2014 |
Click here to see how this site uses Cookies. View our privacy policy here.