DOI:
10.1039/C2TX20050C
(Paper)
Toxicol. Res., 2013,
2, 115-122
Toxicological and pharmacological analysis of selenohomolanthionine in mice†
Received 16th July 2012, Accepted 23rd November 2012
First published on 27th November 2012
Abstract
The toxic and therapeutic effects of selenohomolanthionine (4,4′-selenobis[2-aminobutanoic acid], SeHLan), a newly identified selenoamino acid found in selenized Japanese pungent radish, were compared with those of selenomethionine (SeMet) and selenite in mice. Each Se compound was injected intravenously at the dose of 0.1, 1.0, and 10 mg Se kg−1 body weight. SeHLan and selenite were equally distributed to the liver and the kidneys, whereas SeMet was more preferably distributed to the liver than to the kidneys. Although a part of SeHLan was assimilated and transformed into the urinary Se metabolite, Se-methylseleno-N-acetyl-galactosamine (MeSeGalNAc), the major part of the urinary Se species was intact SeHLan. The results suggest that the metabolic capacity of SeHLan to MeSeGalNAc reached a plateau even at the lowest dose. SeMet at the highest dose significantly increased ALT and amylase activities in mice. On the other hand, treatment with SeHLan at the dose of 10 mg Se kg−1 body weight significantly increased BUN and creatinine levels in mouse sera, that is, nephrotoxicity was noted. SeHLan more effectively improved the survival rate reduced by LPS treatment than selenite and SeMet at the non-toxic dose of Se. The results indicate that SeHLan may be safely and effectively used to treat sepsis in place of selenite that is currently used in the clinical setting.
Introduction
Selenium (Se) is an essential micronutrient in humans and animals, and is required to promote the activities of some selenoenzymes, such as glutathione peroxidases, iodothyronine 5′-deiodinase, and thioredoxin reductase.1 These selenoenzymes contain Se as selenocysteine (SeCys) in their active centers. Animals can assimilate both inorganic and organic Se species in meat, fish, and vegetables, transform them for use in the synthesis of selenoproteins, and excrete them into urine as a urinary Se metabolite, i.e., the selenosugar 1β-methylseleno-N-acetyl-D-galactosamine (MeSeGalNAc).2,3 Se is not essential for plants and no selenoproteins have been identified so far in them. However, some plants are known to be Se accumulators, metabolizing inorganic Se, such as selenite and selenate, to form organic Se compounds.4 Alliaceae plants belonging to Family Liliaceae, such as garlic, onion, wild leek, and shallot, and Brassicaceae plants, such as Indian mustard and radish, are well-known Se accumulators.5,6 In those plants, specific Se-containing amino acids, such as Se-methylselenocysteine (MeSeCys) and γ-glutamyl-Se-methylselenocysteine (GluMeSeCys), are biosynthesized.7,8 As selenoamino acids are less toxic than inorganic Se, they are considered to be more appropriate for use as a nutritional supplement than the inorganic forms.9 Selenomethionine (SeMet) is another chemical form in biota. Many derivatives of SeMet were found in Se-enriched yeast.10,11 However, it was pointed out in a clinical trial that SeMet supplementation at the dose of 200 μg day−1 for several years increased the risk of type 2 diabetes mellitus.12 The novel selenoamino acid, selenohomolanthionine (4,4′-selenobis[2-aminobutanoic acid] or Se-(3-amino-3-carboxypropyl)-homocysteine, SeHLan) (Fig. 1), was identified in selenized Japanese pungent radish.13 SeHLan shows less toxicity to cultured human cells than SeMet.13 It has been reported that the tissue distribution of SeHLan differs from that of SeMet at trace (25 μg Se kg−1 body weight) and large (1.0 mg Se kg−1 body weight) doses in rats.14,15 SeMet was preferably distributed to the liver and the pancreas, whereas SeHLan was preferably distributed to the kidneys. Thus, SeHLan is expected to take the place of SeMet as a nutritional supplement because it can avoid pancreatic damage.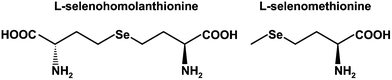 |
| Fig. 1 Structures of selenohomolanthionine and selenomethionine. | |
Sodium selenite is used for the amelioration of sepsis and septic stress.16,17 Although sodium selenite is more toxic than organic Se species, its antiseptic effect is promising when used at a bolus dose.18,19 In the clinical protocol, sodium selenite is intravenously administered to patients at doses of 4000 μg on the first day and 1000 μg day−1 on the subsequent 9 days. The dose is extremely high compared to the recommended daily allowance of Se (30–50 μg day−1). Thus, a safer and more effective Se compound than selenite is desired for clinical use. As mentioned above, SeHLan is a less toxic and nutritionally effective chemical form of Se. However, at present, there are limited toxicological and pharmacological data for SeHLan when it is used at a large dose to treat septic stress and sepsis.
In this study, we evaluated the toxic and therapeutic effects of SeHLan in mice at three doses and compared them with those of known Se species, such as selenite and SeMet. The therapeutic effect of Se compounds was determined from the amelioration of septic stress in mice administered lipopolysaccharide (LPS). The metabolism of SeHLan at the three doses was also evaluated by speciation of urinary Se with HPLC hyphenated with an inductively coupled plasma mass spectrometer (ICP-MS).
Materials and methods
Chemicals
Sodium selenite, powdered elemental Se, nitric acid, ammonium acetate, N,N-dimethylformamide dehydrate (DMF), sodium tetrahydroborate, hydrochloric acid, and Wakogel® 100C18 were purchased from Wako Pure Chemical Industries, Ltd (Osaka, Japan). L-Selenomethionine (SeMet) and (S)-(+)-2-amino-4-bromobutyric acid hydrobromide were purchased from Tokyo Chemical Industry (Tokyo, Japan). Amberlite® 120-R and lipopolysaccharide (LPS, from E. coli 0111:B4) were purchased from Sigma-Aldrich (St. Louis, MO, USA). All reagents were of the highest or analytical grade. Deionized water (18.3 MΩ cm) was used throughout.Synthesis of selenohomolanthionine
SeHLan was synthesized in our laboratory by the method previously reported.13 Briefly, elemental Se (powder, 80 mg) was suspended in 2 mL of DMF under nitrogen atmosphere. Then, sodium tetrahydroborate (600 mg) in DMF (12 mL) was added and the mixture was stirred at room temperature for 60 min. (S)-(+)-2-Amino-4-bromobutyric acid hydrobromide (574 mg) dissolved in DMF (2 mL) was gradually added and the reaction mixture was left to stand at room temperature for 48 h. The reaction was stopped by the addition of 1 mol L−1 HCl and the reaction mixture was evaporated in vacuo. The residue was dissolved in deionized water and purified on a cation-exchange column (Amberlite® 120-R) and an ODS column (Wakogel® 100C18) to afford SeHLan. Obtained SeHLan was further purified by HPLC (Shodex Asahipak GS-520P; 20.0 mm i.d. × 500 mm; Showa Denko, Tokyo). Chemical purity was confirmed by HPLC-ICP-MS as described below.Animal experiments
All animal experiments were carried out according to the “Principles of Laboratory Animal Care” (NIH version, revised 1996) and the Guidelines of the Animal Investigation Committee, Graduate School of Pharmaceutical Sciences, Chiba University, Japan.Four-week-old male ICR mice were purchased from Japan SLC, Inc. (Shizuoka, Japan). All mice were acclimated for one week at 22 ± 2 °C with a 12/12 h light/dark cycle and fed a standard diet (MF; Oriental Yeast, Tokyo) and tap water ad libitum.
For the evaluation of the metabolism and the toxic effects of Se compounds, four or five mice were housed in a metabolic cage to collect urine samples. A whole Se amount in the mixed urine of 4 or 5 mice was determined, and the amount was divided by the number of mice in the cage to obtain the amount of excreted Se per mouse. Each group of mice received an intravenous injection of SeHLan, SeMet or sodium selenite dissolved in saline at doses of 0.1, 1.0, and 10 mg Se kg−1 body weight. Selenite was not given at the dose of 10 mg Se kg−1 body weight because it was beyond the lethal dose of sodium selenite. Blood was collected under light ether anesthesia 24 h after the injection, and clotted blood was centrifuged at 1600g for 10 min to obtain serum. Then, the liver and the kidneys were excised. Tissues and sera were preserved at −30 °C prior to use. Four mice receiving saline served as control.
For the evaluation of the therapeutic effect of Se compounds, forty male ICR mice were divided into four groups of ten mice each. All mice were intraperitoneally injected with LPS at the dose of 15 mg kg−1 body weight, and three groups of mice were intravenously injected with SeHLan, SeMet or selenite at the dose of 1.0 mg Se kg−1 body weight, respectively. The number of surviving mice was counted every 6 h over a 72 h period.
Determination of Se concentration in samples
The organ and urine samples were wet-ashed with concentrated nitric acid (HNO3) and 30% H2O2, and then the ashed samples were diluted with deionized water. Se concentration in the samples was determined by ICP-MS at m/z 82. To validate the Se determination, the Se concentration in a standard reference material (dogfish muscle, DORM2, National Research Council, Ottawa, Canada) was determined.HPLC-ICP-MS analysis
The HPLC system consisted of an on-line degasser (DG660B-2, GL Science Inc., Tokyo), an HPLC pump (PU713, GL Science Inc., Tokyo), a six-port injector (model 7125, Rheodyne, CA) with 20 μL and 200 μL sample loops, and a column. A multi-mode gel filtration column, Shodex Asahipak GS-320HQ (7.5 mm i.d. × 300 mm, with a guard column, 7.5 mm i.d. × 75 mm, Showa Denko), was injected with a 20 μL aliquot of urine sample and then eluted with 50 mmol L−1 ammonium acetate, pH 6.5, at the flow rate of 0.6 mL min−1. The eluate was introduced directly into the Babington nebulizer of the ICP-MS, and Se distribution was monitored at m/z 82.Measurement of serum biochemical parameters
Measurement of serum biochemical parameters, i.e., alanine aminotransferase (ALT), blood urea nitrogen (BUN), creatinine, albumin, and amylase, was performed using a Hitachi7170 model auto-analyzer (Hitachi High-Technologies Corporation, Tokyo). Kits used for ALT, BUN, creatinine, albumin, and amylase measurements were L-type Wako GPT-J2, L-type Wako UN, L-type Wako CRE-M, albumin II-HA rest Wako, and L-type Wako amylase, respectively.Statistics
The results are presented as means ± standard deviations (S.D.) of four or five samples. All statistical analyses were performed with JMP7.0 (SAS, USA). Differences from the control group were tested using the Dunnett method. A p value <0.05 was considered to be statistically significant. Survival curves were analyzed using the Kaplan–Meier test.Results and discussion
Se determination in organ and urine samples after injection of Se compounds
Se concentration in the livers of control mice was 0.74 ± 0.11 μg g−1 liver (Fig. 2a). There were no significant differences in the Se concentration in the livers of mice treated with the three Se compounds at the dose of 0.1 mg Se kg−1 body weight, suggesting that all the Se compounds at that dose are within the metabolic capacity of the livers. The Se concentrations in the livers of SeHLan- or selenite-injected mice at the dose of 1.0 mg Se kg−1 body weight tended to be higher than that of control. On the other hand, the Se concentration in the livers of SeMet-injected mice at the dose of 1.0 mg Se kg−1 body weight was significantly higher than that of control. Selenite at the dose of 10 mg Se kg−1 body weight was lethal to mice, as indicated by the intravenous LD50 of 6.7 mg kg−1 body weight in mice. Thus, the group was omitted from the experimental protocol. Se concentration in the livers of SeHLan-injected mice was significantly increased, and that of SeMet-injected mice was significantly higher than that of SeHLan-injected mice at the dose of 10 mg Se kg−1 body weight. These results suggest that SeMet is more efficiently accumulated in the liver than SeHLan despite their having similar structures.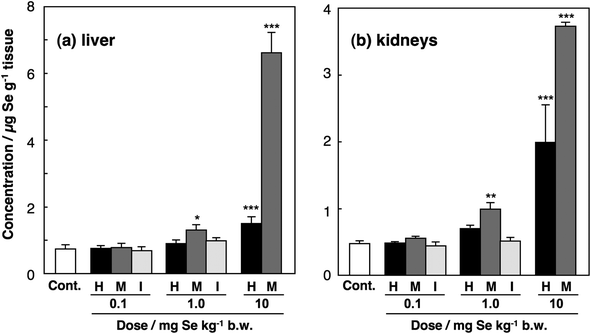 |
| Fig. 2 Se concentrations in the organs of mice injected with Se compounds. Male ICR mice were intravenously injected with SeHLan (H), SeMet (M) or selenite (I) at the doses of 0.1, 1.0, and 10 mg Se kg−1 body weight. Selenite was not administered at the dose of 10 mg Se kg−1 body weight because that dose was lethal. The livers and kidneys were excised 24 h after the injection. Se concentrations in the livers (a) and kidneys (b) were determined by ICP-MS. Columns and bars represent means ± standard deviation for 4 animals. Significant levels between control group and treated groups are indicated by *, **, and *** at p < 0.05, p < 0.01, and p < 0.001, respectively. | |
In the kidneys, changes in the Se concentration showed the same tendency after the injection of the three Se compounds at the doses of 0.1 and 1.0 mg Se kg−1 body weight (Fig. 2b). At the dose of 10 mg Se kg−1 body weight, the Se concentrations in the kidneys of SeHLan- and SeMet-injected rats were 1.99 ± 0.56 and 3.73 ± 0.06 μg g−1 kidney, respectively, whereas the Se concentrations in the livers of SeHLan- and SeMet-injected rats were 1.50 ± 0.19 and 6.62 ± 0.59 μg g−1 liver, respectively (Fig. 2a). This indicates that excess SeHLan that is beyond the metabolic capacity is more preferably accumulated in the kidneys than in the liver, whereas excess SeMet is more preferably accumulated in the liver than in the kidneys. It is well known that SeMet is incorporated into proteins in general in place of methionine (Met) because all organisms cannot completely distinguish SeMet from Met in the process of protein translation.9,20 As a result, SeMet is particularly accumulated in the liver and the pancreas, both of which have higher protein synthesis activity than the other organs. Indeed, we reported that SeMet was accumulated in the pancreas of rats.14,15 On the other hand, SeHLan may be strictly discriminated from proteinaceous amino acids, such as Met and cysteine (Cys), and thus excess SeHLan seems to be excreted into urine through the kidneys. Hence, the severe Se accumulation in the kidneys was induced by the administration of SeHLan at the highest dose.
Se speciation in urine
It is well known that Se is excreted mainly into urine as selenosugar (1β-methylseleno-N-acetyl-D-galactosamine, MeSeGalNAc) within the adequate level of Se ingested.2,21 In control mice, MeSeGalNAc was detected as the major urinary Se metabolite at the retention time of 20.6 min on the elution profile of Se (Fig. 3a). In addition to MeSeGalNAc, the intact form of SeHLan was also detected at the retention time of 17.9 min in the urine of mice treated with SeHLan at the dose of 0.1 mg Se kg−1 body weight (Fig. 3b). The amount of MeSeGalNAc at that dose was increased by approximately 3 times compared to that of control, as shown by the peak height in the elution profile. However, the major part of urinary Se species was SeHLan. This suggested that part of the ingested SeHLan was assimilated, metabolized, and excreted into urine although most of the ingested SeHLan was excreted into urine without being metabolized. The peaks of TMSe and an unknown Se metabolite appeared at the retention times of 22.1 and 16.0, respectively, in mice that received SeHLan at the dose of 1.0 mg Se kg−1 body weight (Fig. 3c and c′). TMSe is known as a urinary metabolite when the amount of Se ingested exceeds the nutritional level in animals.22 The chemical structure of Se whose peak appeared at the retention time of 16.0 min has not been assigned so far. It is reported that dimethylselenide (DMSe) and dimethyldiselenide (DMDSe) are also excreted into urine despite the fact that these Se metabolites are volatile species.23,24 However, our chromatographic conditions do not allow the elution of volatile Se species from the column. Hence, these unknown peaks may correspond to complexes between volatile Se species and a biomolecule, or the oxidized forms of volatile Se compounds, such as dimethylselenide oxide or methylseleninic acid. It should be noted that the amount of MeSeGalNAc was still increased at this dose. Other Se metabolites were detected in the urine of mice treated at the dose of 10 mg Se kg−1 body weight (Fig. 3d). The Se peak detected at the retention time of 11.0 min corresponded to the void volume of the column, suggesting that the Se peak consisted of Se metabolite(s) and a macro biomolecule, such as a urinary protein whose molecular mass was beyond the exclusion size of the column (>40 kDa). Se peaks appearing at the retention times of 23.5 and 26.5 min seemed to be novel urinary Se metabolites, although they have not been identified yet. The elucidation of their structures is expected to facilitate our understanding of the metabolic pathway of Se, in particular, selenoamino acids. It has been pointed out that HPLC-ICP-MS has several limitations when used for the identification of metal/metalloid-containing unknown species.25 Contrary to HPLC-ICP-MS, an organic tandem mass spectrometer, such as ESI-MS-MS and APCI-MS-MS, coupled with HPLC can detect novel Se-containing metabolites in living organisms.3,26 However, ICP-MS has higher sensitivity and robustness to matrices than the organic tandem mass spectrometer. As rodent urine contains large amounts of matrices, such as salts, hydrophilic metabolites, and proteins, preconcentration and partial purification are needed to detect Se metabolites by ESI-MS-MS in urine. We intend to identify these urinary Se metabolites in a future study. As shown in Fig. 3, the amount of MeSeGalNAc was not increased with the increase in dose from 1.0 to 10 mg Se kg−1 body weight. On the other hand, the amount of TMSe was slightly increased with the dose. Together, the results suggested that the metabolic capacity of SeHLan to MeSeGalNAc reached a plateau at the dose of 1.0 mg Se kg−1 body weight.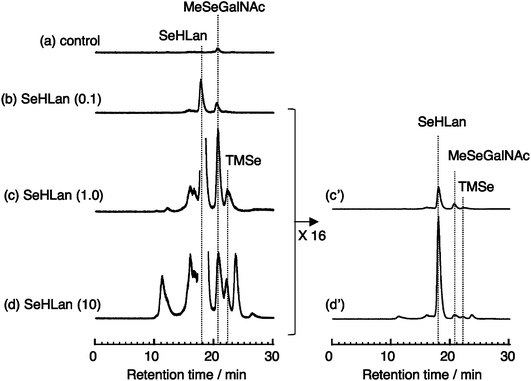 |
| Fig. 3 Elution profiles of Se in 24 h urine samples from mice injected with SeHLan at the doses of 0.1, 1.0, and 10 mg Se kg−1 body weight. Urine was collected 24 h after injection, combined in each group of 4 mice, and then subjected to HPLC-ICP-MS analysis on a multi-mode gel filtration column (Shodex Asahipak GS-320HQ) eluted with 50 mmol L−1 ammonium acetate. Se in the eluate was monitored at m/z 82. Numbers in parenthesis show the dose in mg Se kg−1 body weight. | |
The amount of Se in the 24 h urine sample proportionally increased with the dose (Table 1). As mentioned above, the major chemical form of urinary Se was intact SeHLan; thus, the proportional increase in urinary Se may reflect the amount of SeHLan in the urine sample.
Table 1 Amount of Se excreted into urine of mice injected with SeHLan at the doses of 0.1, 1.0, and 10 mg Se kg−1 body weight. Numbers in the parentheses are the number of mouse in the metabolic cage
Dose (mg Se kg−1 body weight) | Amount excreted (μg Se day−1 per mouse) |
---|
Control | 0.12 (5) |
0.1 | 0.98 (4) |
1.0 | 8.01 (4) |
10 | 99.6 (4) |
Comparison of toxic effects among Se compounds
No significant changes in serum biochemical parameters were observed at low and intermediate doses except the serum amylase activity of mice treated with SeMet at the dose of 0.1 mg Se kg−1 body weight (Fig. 4). ALT and amylase activities were significantly increased in mice treated with SeMet at the highest dose (Fig. 4a and d). Albumin level was decreased in the sera of mice treated with SeMet at the dose (data not shown). SeMet was more preferably accumulated in the liver than other Se species, and it was reported that SeMet was also accumulated in the pancreas.27 Indeed, [75Se]-labeled SeMet has been used as a contrast agent for pancreatic autoradiography.28,29 Hence, the accumulated SeMet damaged the liver and the pancreas, resulting in the increase in ALT and amylase activities. Treatment with SeHLan at the dose of 10 mg Se kg−1 body weight led to significant increases in BUN and creatinine levels in mouse sera (Fig. 4b and c). This suggests that nephrotoxicity is induced by the accumulation of SeHLan in the kidneys. Although the Se concentration in the kidneys of mice treated with SeHLan at the dose of 1.0 mg Se kg−1 (1.99 μg Se g−1 tissue) was comparable to that in the liver (1.50 μg Se g−1 tissue) (Fig. 2), the toxicity of SeHLan was induced only in the kidneys. Hence, the kidneys are more vulnerable to SeHLan than the liver. The mechanisms underlying the nephrotoxicity of SeHLan are still unclear. The metabolism of SeHLan reached a plateau at the non-nephrotoxic dose, i.e., 1.0 mg Se kg−1 body weight. Thus, the metabolites of SeHLan did not seem to have any toxic effects on the kidneys. As most of the urinary Se species were detected as SeHLan at the toxic dose, SeHLan itself might induce the nephrotoxicity. The sulfur analogue of SeHLan, such as lanthionine or homolanthionine, is a component of peptide-containing antibiotics known as lantibiotics.30 This pharmacological activity of lantibiotics may attribute to the toxicity of SeHLan despite further elucidation should be awaited. On the other hand, no apparent nephrotoxicity was detected in the mice treated with SeMet at the high dose. The dose of 10 mg kg−1 body weight of inorganic Se is lethal to mice. However, no significant changes in serum biochemical indices were observed at the two lower doses of 0.1 and 1.0 mg kg−1 body weight.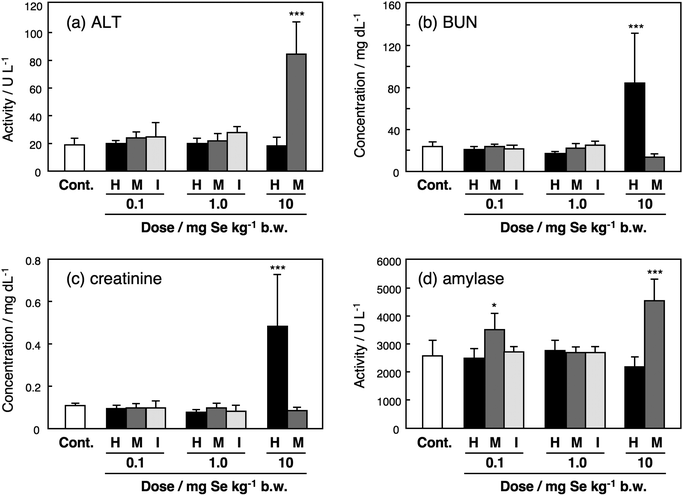 |
| Fig. 4 Serum biochemical indices of mice injected with Se compounds. Male ICR mice were intravenously injected with SeHLan (H), SeMet (M) or selenite (I) at the doses of 0.1, 1.0, and 10 mg Se kg−1 body weight. Selenite was not administered at the dose of 10 mg Se kg−1 body weight because that dose was lethal. Sera were collected 24 h after the injection and serum biochemical parameters were determined using an auto-analyzer and a kit. Columns and bars represent means ± standard deviation for 4 animals. Significant differences between control group and treated groups were indicated by *** at p < 0.001. | |
Protective effects of Se compounds against LPS toxicity
The survival rate of the mice was 0.1 at 72 h after the injection of LPS alone at the dose of 15 mg kg−1 body weight (Fig. 5). Because the highest dose (10 mg kg−1 body weight) showed toxicity as mentioned above, the intermediate dose (1.0 mg kg−1 body weight) was used for the treatment of LPS toxicity. It has been reported that sodium selenite ameliorates septic stress.31,32 Indeed, treatment with inorganic Se, i.e., sodium selenite, lessened the mortality caused by the LPS treatment (Fig. 5). SeMet and SeHLan were also effective in decreasing the mortality at that dose; in particular, SeHLan improved the survival rate from 0.1 to 0.5. Some mechanisms underlying the amelioration of septic stress by selenite were proposed. For instance, selenite acts as a redox modulator against oxidative damage induced by septic stress.33 Selenite may boost the immune system.34–36 Selenite recovers the amount of selenoprotein P (Sel P) that is reduced by septic stress.37 However, these mechanisms cannot convincingly explain the effect of SeHLan and SeMet on septic stress. SeHLan and SeMet are redox-stable compounds in organisms. Although the anti-oxidative effect of Sel P was proposed, the activity of Sel P as an anti-oxidant was lower than those of other Se-containing anti-oxidative enzymes, such as glutathione peroxidase and thioredoxin reductase.38 Thus, the actual biological function of Sel P is still controversial. Further studies are needed to clarify the precise mechanism of action of SeHLan and SeMet against septic stress.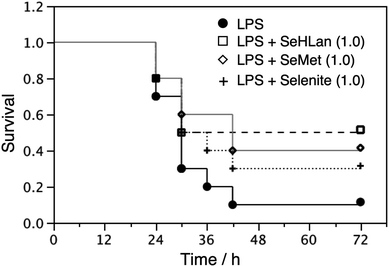 |
| Fig. 5 Protective role of Se compounds against LPS toxicity. Male ICR mice were intraperitoneally injected with LPS at the dose of 15 mg kg−1 body weight, and then intravenously injected with Se compounds at the dose of 1.0 mg Se kg−1 body weight. The number of surviving mice was counted every 6 h over a 72 h period. Numbers in parenthesis show the dose in mg Se kg−1 body weight. | |
In conclusion, SeMet was more preferably distributed to the liver than to the kidneys, whereas SeHLan was equally distributed to the liver and the kidneys. SeMet induced hepatic and pancreatic toxicities at the highest dose (10 mg Se kg−1 body weight). SeHLan induced toxicity in the kidneys at the highest dose. Although part of SeHLan was assimilated and transformed into the urinary Se metabolite, MeSeGalNAc, the major part of urinary Se species was intact SeHLan. SeHLan more effectively improved the survival rate lessened by the LPS-induced septic stress than selenite and SeMet at the non-toxic dose (1.0 mg Se kg−1 body weight). Overall, the results indicate that SeHLan can be used safely and effectively to treat sepsis in the clinical setting in place of selenite.
Acknowledgements
We would like to acknowledge Grants-in-Aid from the Ministry of Education, Culture, Sports, Science and Technology, Japan (Nos. 23390032 and 24659022), and the financial support from Otsuka Pharmaceutical Factory, Inc., Japan.References
- X. M. Xu, B. A. Carlson, Y. Zhang, H. Mix, G. V. Kryukov, R. S. Glass, M. J. Berry, V. N. Gladyshev and D. L. Hatfield, New developments in selenium biochemistry: selenocysteine biosynthesis in eukaryotes and archaea, Biol. Trace Elem. Res., 2007, 119, 234–241 CrossRef CAS.
- Y. Kobayashi, Y. Ogra, K. Ishiwata, H. Takayama, N. Aimi and K. T. Suzuki, Selenosugars are key and urinary metabolites for selenium excretion within the required to low-toxic range, Proc. Natl. Acad. Sci. U. S. A., 2002, 99, 15932–15936 CrossRef CAS.
- Y. Ogra and Y. Anan, Selenometabolomics: identification of selenometabolites and specification of their biological significance by complementary use of elemental and molecular mass spectrometry, J. Anal. At. Spectrom., 2009, 24, 1477–1488 RSC.
- T. G. Sors, D. R. Ellis and D. E. Salt, Selenium uptake, translocation, assimilation and metabolic fate in plants, Photosynth. Res., 2005, 86, 373–389 CrossRef CAS.
- E. Kápolna, M. Shah, J. A. Caruso and P. Fodor, Selenium speciation studies in Se-enriched chives (Allium schoenoprasum) by HPLC-ICP–MS, Food Chem., 2007, 101, 1398–1406 CrossRef.
- M. Kotrebai, J. F. Tyson, P. C. Uden, M. Birringer and E. Block, Identification of the principal selenium compounds in selenium-enriched natural sample extracts by ion-pair liquid chromatography with inductively coupled plasma- and electrospray ionization-mass spectrometric detection, Anal. Commun., 1999, 36, 249–252 RSC.
- Y. Ogra, K. Ishiwata, Y. Iwashita and K. T. Suzuki, Simultaneous speciation of selenium and sulfur species in selenized odorless garlic (Allium sativum L. Shiro) and shallot (Allium ascalonicum) by HPLC-inductively coupled plasma-(octopole reaction system)-mass spectrometry and electrospray ionization-tandem mass spectrometry, J. Chromatogr. A, 2005, 1093, 118–125 CrossRef CAS.
- E. Dumont, F. Vanhaecke and R. Cornelis, Selenium speciation from food source to metabolites: a critical review, Anal. Bioanal. Chem., 2006, 385, 1304–1323 CrossRef CAS.
- G. N. Schrauzer, Selenomethionine: a review of its nutritional significance, metabolism and toxicity, J. Nutr., 2000, 130, 1653–1656 CAS.
- M. Dernovics, T. García-Barrera, K. Bierła, H. Preud'homme and R. Lobiński, Standardless identification of selenocystathionine and its -glutamyl derivatives in monkeypot nuts by 3D liquid chromatography with ICP-MS detection followed by nanoHPLC–Q-TOF-MS/MS, Analyst, 2007, 132, 439–449 RSC.
- L. Tastet, D. Schaumloffel, B. Bouyssiere and R. Lobinski, Capillary HPLC-ICP MS mapping of selenocompounds in spots obtained from the 2-D gel electrophoresis of the water-soluble protein fraction of selenized yeast, Anal. Bioanal. Chem, 2006, 385, 948–953 CrossRef CAS.
- S. M. Lippman, E. A. Klein, P. J. Goodman, M. Scott Lucia, I. M. Thompson, L. G. Ford, H. L. Parnes, L. M. Minasian, J. Michael Gaziano, J. A. Hartline, J. Kellogg Parsons, J. D. Bearden III, E. David Crawford, G. E. Goodman, J. Claudio, E. Winquist, E. D. Cook, D. D. Karp, P. Walther, M. M. Lieber, A. R. Kristal, A. K. Darke, K. B. Arnold, P. A. Ganz, R. M. Santella, D. Albanes, P. R. Taylor, J. L. Probstfield, T. J. Jagpal, J. J. Crowley, F. L. Meyskens Jr, L. H. Baker and C. A. Coltman Jr, Effect of selenium and vitamin E on risk of prostate cancer and other cancers: the selenium and vitamin E cancer prevention trial (SELECT), J. Am. Med. Assoc., 2009, 301, 39–51 CrossRef CAS.
- Y. Ogra, T. Kitaguchi, K. Ishiwata, N. Suzuki, Y. Iwashita and K. T. Suzuki, Identification of selenohomolanthionine in selenium-enriched Japanese pungent radish, J. Anal. At. Spectrom., 2007, 22, 1390–1396 RSC.
- Y. Anan, T. Mikami, Y. Tsuji and Y. Ogra, Distribution and metabolism of selenohomolanthionine labeled with a stable isotope, Anal. Bioanal. Chem., 2011, 399, 1765–1772 CrossRef CAS.
- Y. Tsuji, T. Mikami, Y. Anan and Y. Ogra, Comparison of selenohomolanthionine and selenomethionine in terms of selenium distribution and toxicity in rats by bolus administration, Metallomics, 2010, 2, 412–418 RSC.
- X. Forceville, B. Laviolle, D. Annane, D. Vitoux, G. Bleichner, J. M. Korach, E. Cantais, H. Georges, J. L. Soubirou, A. Combes and E. Bellissant, Effects of high doses of selenium, as sodium selenite, in septic shock: a placebo-controlled, randomized, double-blind, phase II study, Crit. Care, 2007, 11, R73 CrossRef.
- X. Forceville, Effects of high doses of selenium, as sodium selenite, in septic shock patients a placebo-controlled, randomized, double-blind, multi-center phase II study – selenium and sepsis, J. Trace Elem. Med. Biol., 2007, 21(Suppl. 1), 62–65 CAS.
- Z. Wang, X. Forceville, P. Van Antwerpen, M. Piagnerelli, D. Ahishakiye, P. Macours, D. De Backer, J. Neve and J. L. Vincent, A large bolus, but not a continuous infusion, of sodium selenite improves outcome in peritonitis, Shock, 2008, 32, 140–146 CrossRef.
- Z. Wang, X. Forceville, P. Van Antwerpen, M. Piagnerelli, D. Ahishakiye, P. Macours, D. De Backer, J. Neve and J. L. Vincent, A large-bolus injection, but not continuous infusion of sodium selenite improves outcome in peritonitis., Shock, 2009, 32, 140–146 CrossRef CAS.
- Y. Ogra, T. Kitaguchi, N. Suzuki and K. T. Suzuki, In vitro translation with [34S]-labeled methionine, selenomethionine and telluromethionine, Anal. Bioanal. Chem., 2008, 390, 45–51 CrossRef CAS.
- K. T. Suzuki, K. Kurasaki, N. Okazaki and Y. Ogra, Selenosugar and trimethylselenonium among urinary Se metabolites: dose- and age-related changes, Toxicol. Appl. Pharmacol., 2005, 206, 1–8 CrossRef CAS.
- S. H. Zeisel, A. L. Ellis, X. F. Sun, E. A. Pomfret, B. T. Ting and M. Janghorbani, Dose–response relations in urinary excretion of trimethylselenonium in the rat, J. Nutr., 1987, 117, 1609–1614 CAS.
- M. Bueno and F. Pannier, Quantitative analysis of volatile selenium metabolites in normal urine by headspace solid phase microextraction gas chromatography-inductively coupled plasma mass spectrometry, Talanta, 2009, 78, 759–763 CrossRef CAS.
- D. Juresa, D. Kuehnelt and K. A. Francesconi, Consequences of vapor enhancement on selenium speciation analysis by HPLC/ICPMS, Anal. Chem., 2006, 78, 8569–8574 CrossRef CAS.
- Y. Ogra, Integrated strategies for identification of selenometabolites in animal and plant samples, Anal. Bioanal. Chem., 2008, 390, 1685–1689 CrossRef CAS.
- B. Gammelgaard, C. Gabel-Jensen, S. Stürup and H. R. Hansen, Complementary use of molecular and element-specific mass spectrometry for identification of selenium compounds related to human selenium metabolism, Anal. Bioanal. Chem., 2008, 390, 1691–1706 CrossRef CAS.
- K. T. Suzuki, L. Somekawa, K. Kurasaki and N. Suzuki, Simultaneous tracing of 76Se-selenite and 77Se-selenomethionine by absolute labeling and speciation, Toxicol. Appl. Pharmacol., 2006, 217, 43–50 CrossRef CAS.
- M. F. Cottrall and D. M. Taylor, [75Se]selenomethionine uptake by the pancreas, J. Nucl. Med., 1980, 21, 191–192 CAS.
- N. Krasner, M. J. Brodie, D. J. Sumner and T. J. Thomson, Tests of pancreatic function using 75Se-selenomethionine, Acta Hepatogastroenterol. (Stuttg), 1976, 23, 144–150 CAS.
- G. Bierbaum and H. G. Sahl, Lantibiotics: mode of action, biosynthesis and bioengineering, Curr. Pharm. Biotechnol., 2009, 10, 2–18 CAS.
- M. W. Angstwurm, L. Engelmann, T. Zimmermann, C. Lehmann, C. H. Spes, P. Abel, R. Strauss, A. Meier-Hellmann, R. Insel, J. Radke, J. Schüttler and R. Gärtner, Selenium in Intensive Care (SIC): results of a prospective randomized, placebo-controlled, multiple-center study in patients with severe systemic inflammatory response syndrome, sepsis, and septic shock, Crit. Care Med., 2007, 35, 118–126 CrossRef CAS.
- K. Renko, P. J. Hofmann, M. Stoedter, B. Hollenbach, T. Behrends, J. Kohrle, U. Schweizer and L. Schomburg, Down-regulation of the hepatic selenoprotein biosynthesis machinery impairs selenium metabolism during the acute phase response in mice, FASEB J., 2009, 23, 1758–1765 CrossRef CAS.
- X. Forceville, The need for precise dose information of sodium selenite in the SIC study and rectification of GPx-3 plasma concentration, Crit. Care Med., 2008, 36, 656–657 CrossRef.
- R. L. Norton and P. R. Hoffmann, Selenium and asthma, Mol. Aspects Med., 2012, 33, 98–106 CrossRef CAS.
- M. P. Rayman, Selenium and human health, Lancet, 2012, 379, 1256–1268 CrossRef CAS.
- Z. Huang, A. H. Rose and P. R. Hoffmann, The role of selenium in inflammation and immunity: from molecular mechanisms to therapeutic opportunities, Antioxid. Redox Signal, 2012, 16, 705–743 CrossRef CAS.
- B. Hollenbach, N. G. Morgenthaler, J. Struck, C. Alonso, A. Bergmann, J. Kohrle and L. Schomburg, New assay for the measurement of selenoprotein P as a sepsis biomarker from serum, J. Trace Elem. Med. Biol., 2008, 22, 24–32 CAS.
- G. Takebe, J. Yarimizu, Y. Saito, T. Hayashi, H. Nakamura, J. Yodoi, S. Nagasawa and K. Takahashi, A comparative study on the hydroperoxide and thiol specificity of the glutathione peroxidase family and selenoprotein P, J. Biol. Chem., 2002, 277, 41254–41258 CrossRef CAS.
Footnote |
† Electronic supplementary information (ESI) available. See DOI: 10.1039/c2tx20050c |
|
This journal is © The Royal Society of Chemistry 2013 |