DOI:
10.1039/C2GC36900A
(Communication)
Green Chem., 2013,
15, 596-600
Evaluation of alternative solvents in common amide coupling reactions: replacement of dichloromethane and N,N-dimethylformamide†
Received
27th November 2012
, Accepted 19th December 2012
First published on 19th December 2012
The amide bond is one of the most fundamental functional group linkages and underpins the connectivity of basic biomolecules (e.g., proteins) as well as being commonly found in many widely used materials (e.g., nylons) and key pharmaceuticals (e.g., penicillin, Lipitor®).1,2 In this latter context, amide bond formation is the single most common synthetic transformation used within medicinal chemistry. Indeed, several studies have demonstrated the prevalence of this particular transformation within the pharmaceutical sector: MacDonald's analysis of the GlaxoSmithKline (GSK) Respiratory Centre of Excellence for Drug Discovery revealed that 17% of all reaction types conducted in array (focused library) format were to prepare amide or sulfonamide moieties.3 Similarly, Roughley's analysis of the most common reactions used within synthetic medicinal chemistry research across three of the largest pharmaceutical companies (GSK, AstraZeneca, and Pfizer) indicated that N-acylation to prepare amides ranked 1st for frequency of use, accounting for 16% of all reactions performed and with the amide linkage present in 54% of the compound set analysed.4
While carboxamide formation is formally a condensation between a suitable carboxylic acid and amine combination that can be achieved simply by heating the requisite components together,5 this is typically not an efficient or particularly useful method due to the formation of an unreactive carboxylate–ammonium salt which commonly leads to poor yields, lengthy reaction times, or substrate compatibility issues.6 As such, carboxamide formation is often more conveniently achieved through application of a suitable coupling agent.7 Over the years, amide bond coupling reagents have been the subject of some considerable development to the stage that a bank of these reagents are available for deployment and guides have been devised to assist the practitioner in selecting the most effective reagent for a desired amidation.8
Throughout this area, however, the most widespread solvents employed are those with major regulatory issues such as chlorinated (dichloromethane, 1,2-dichloroethane) or N,N-dimethylformamide (DMF). For example, a survey of amidation reactions using SciFinder revealed that 83% of approximately 680
000 amidation reactions employed either CH2Cl2 (36%) or DMF (47%) as the reaction media.9 By contrast, the emerging green solvent 2-methyltetrahydrofuran accounted for only 0.04% of this reaction set.9 Despite their utility, CH2Cl2 and DMF are clearly not compatible with the current drive towards more sustainable and environmentally cognisant medicinal chemistry processes.10
As part of a programme focused on enabling sustainable medicinal chemistry practices, we have been interested in addressing solvent selection within both reaction and purification scenarios.11 Herein, we describe our evaluation of a selection of alternative solvents for use within amidation reactions using common amide coupling agents with a view to identifying suitable replacements for CH2Cl2 and DMF.
Results and discussion
Methods
For our study, we elected to use five of the most common amide coupling reagents or reagent combinations: (1-cyano-2-ethoxy-2-oxoethylidenaminooxy)dimethylamino-morpholino-carbenium hexafluorophosphate (COMU),12N,N′-diisopropylcarbodiimide/hydroxybenzotriazole (DIC/HOBt),13N-[(dimethylamino)-1H-1,2,3-triazolo-[4,5-b]pyridin-1-ylmethylene]-N-methylmethanaminium hexafluorophosphate N-oxide (HATU),14 (benzotriazol-1-yloxy)tripyrrolidinophosphonium hexafluorophosphate (PyBOP),15 and n-propylphosphonic anhydride (T3P®)16 (Fig. 1). In addition, we aimed to evaluate these reagents within the amidation reactions of representative examples of both aryl and alkyl acids and amines in order to probe alkyl–alkyl, aryl–aryl, and alkyl–aryl couplings (Fig. 1). In terms of solvent selection, to compare directly with CH2Cl2 and DMF, we selected emerging or existing solvents including tert-butyl methyl ether (TBME), cyclopentylmethyl ether (CPME), dimethylcarbonate (DMC), ethyl acetate (EtOAc), iso-propyl alcohol (IPA), and 2-methyltetrahydrofuran (2-MeTHF) (Fig. 1).17 Other potential alternative solvents such as MeOH, EtOH, and acetone were discounted based on unfavourable potential side reactions (esterification and condensation pathways). Overall, we elected to evaluate 160 different sets of reaction conditions in this initial stage of our survey.
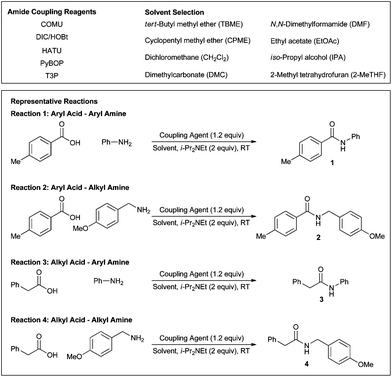 |
| Fig. 1 Coupling agents, solvents, and representative reactions for the amidation survey.† | |
To ascertain the reaction performance of the alternative solvents, we analysed each reaction by HPLC at a range of time points (0 h, 1 h, 2 h, 3 h, 4 h, 5 h, 6 h, 8 h, and 24 h) to give a conversion curve, which could be directly compared across the solvent selection. Reactions and aliquots were repeated and analysed up to a maximum of four times to ensure reliability.†
Analysis
An illustrative example chart of conversion vs. time for Reaction 3 using HATU is shown in Fig. 2. The data indicated that this amidation proceeded effectively in most solvents and was generally complete after 4 h with the exception of TBME (8 h) and CPME (24 h – data point not shown). An alternative view of a section of the generated data is obtained from analysis of coupling agents for a particular reaction in a given solvent. For example, Fig. 3 displays the conversion vs. time data for Reaction 3 in 2-MeTHF using the five coupling agents.†
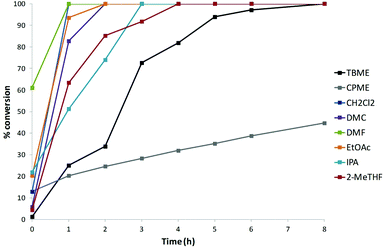 |
| Fig. 2 Representative example of conversion data: amidation Reaction 3 using HATU with the range of solvents.† | |
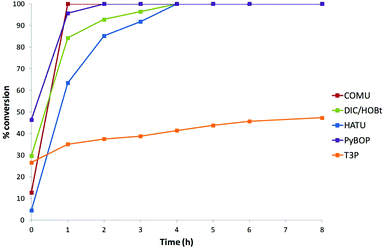 |
| Fig. 3 Representative example of conversion data: amidation Reaction 3 with the range of coupling agents in 2-MeTHF.† | |
From consideration of the overall data set (see ESI†), a series of general observations could be made. Firstly, unsurprisingly, aryl–aryl couplings (Reaction 1) tended to be the slowest processes in general while alkyl–alkyl couplings (Reaction 4) were faster overall. Most reactions (81% of the dataset) proceeded to 100% conversion, 74% were complete within 4 h, and 36% were complete within 1 h. In terms of general trends observed for the array of solvents: (i) TBME was generally good for aryl–aryl (Reaction 1) and alkyl–alkyl (Reaction 4) couplings and poor for aryl–alkyl (Reaction 2) and alkyl–aryl (Reaction 3) couplings. (ii) CPME was generally poor across the spectrum of reaction conditions examined with the exception of the more reactive alkyl–alkyl coupling (Reaction 4), Reaction 1 using HATU, PyBOP, and T3P, and Reaction 2 using T3P. (iii) As expected, CH2Cl2 and DMF were generally very good for all reactions. (iv) Pleasingly, DMC, EtOAc, and 2-MeTHF were found to be generally very good for all reactions. (v) Somewhat surprisingly, IPA performed very well with only a few exceptions (particularly for Reaction 3). An additional observation relating to TBME and CPME is that reactions tended to become heterogeneous as time progressed. This may help to explain why these reactions were generally less successful than the equivalent reactions in the other solvents. Lastly, all coupling agents were effective in each class of reaction (depending on solvent as discussed above) with the exception of T3P for alkyl acid–aryl amine couplings (Reaction 3) which were generally very poor. An illustrative summary of the overall analysis is provided in Table 1.
Table 1 Illustrative representation of the amidation dataseta
Key: Red = <50% conv., orange = 50–70% conv., green = >70% conv.; * Indicates 100% conv. within 4 h. ** Indicates 100% conv. within 1 h.
|
|
Based on all of this, it was evident that DMC, EtOAc, and 2-MeTHF were the alternative solvents which offered the greatest potential as replacements for CH2Cl2/DMF. Additionally, COMU has emerged as an effective and greener amide coupling agent which operated efficiently within the benchmark reaction survey and, indeed, in several cases more effectively than the ‘gold standard’2n reagent HATU. As such, we decided to further evaluate DMC, EtOAc, and 2-MeTHF as the reaction solvent alongside CH2Cl2 and DMF for comparison utilising COMU as the coupling agent over a broader range of substrates possessing increased functionality (Table 2). Based on observations from the screening process that reactions using COMU in DMC, EtOAc, and 2-MeTHF were generally complete within 4 h, the reactions in Table 2 were analysed at 0 h (effectively within 5 min), 4 h and 24 h. In addition to increased functionality, the acids and amines were selected on the basis that they would deliver amide products that had a physicochemical profile that was consistent with being lead-like (H-Bond Acceptors, 2–7; H-Bond Donors, 0–2; Rotatable Bonds, 3–10; Polar Surface Area, 20–93 Å2; Molecular Weight, 231–332; Xlog P, 0–2.4).18
Table 2 Assessment of promising solvents in a range of amidations using COMU as the preferred coupling agenta

|
Entry |
Product |
Completion time b |
CH2Cl2 |
DMF |
DMC |
EtOAc |
2-MeTHF |
Reaction conditions: acid (1 equiv., 0.2 mmol), amine (1.2 equiv., 0.24 mmol), i-Pr2NEt (2 equiv., 0.4 mmol), COMU (1.5 equiv., 0.3 mmol), solvent (1 mL, 0.2 M), RT.
Determined by HPLC analysis. See ESI.
Taken at 0 h time point, represents first data point sampled.
|
1 |
|
4 h |
4 h |
4 h |
4 h |
4 h |
2 |
|
4 h |
4 h |
4 h |
4 h |
4 h |
3 |
|
4 h |
4 h |
4 h |
4 h |
4 h |
4 |
|
24 h |
24 h |
4 h |
4 h |
4 h |
5 |
|
4 h |
5 minc |
24 h |
4 h |
48 h |
6 |
|
24 h |
24 h |
4 h |
4 h |
4 h |
7 |
|
5 minc |
4 h |
4 h |
5 minc |
5 minc |
8 |
|
24 h |
24 h |
4 h |
4 h |
4 h |
9 |
|
4 h |
5 minc |
4 h |
4 h |
4 h |
10 |
|
4 h |
4 h |
4 h |
24 h |
4 h |
As can be seen from Table 2, the amidation reactions were generally complete within the first four hours and comparable to CH2Cl2 and DMF in the majority of reactions. Indeed, there were very few cases where CH2Cl2 or DMF were shown to outperform the alternative solvents over the substrate range evaluated under these reaction conditions. Overall, these results suggest that DMC, EtOAc, and 2-MeTHF would be effective replacements for CH2Cl2 and DMF for similar amidation processes. In addition, and with specific regard to DMF, an additional practical value of employing DMC, EtOAc, or 2-MeTHF as alternatives is that they would simplify the work up procedure involved prior to any purification as both the boiling points and the water miscibility of these solvents are considerably lower than that of DMF,17 leading to more effective aqueous work up and concentration processes, where appropriate.
Conclusions
In summary, we have evaluated several alternative solvents as potential replacements for CH2Cl2 and DMF as the medium for four benchmark amide bond forming reactions using common amide coupling reagents or reagent combinations. These studies revealed that CH2Cl2 and DMF could potentially be readily replaced with more environmentally acceptable and sustainable alternatives. We subsequently applied three of these solvents in a range of amidation reactions using a variety of carboxylic acids and amines with functionality frequently encountered within Medicinal Chemistry programmes, employing COMU as the preferred coupling agent. This assessment demonstrated that the general rates of reaction using DMC, EtOAc, and 2-MeTHF were broadly comparable to those observed using CH2Cl2 or DMF and delivered equally high levels of conversion to product. Overall, we believe that DMC, EtOAc, and 2-MeTHF would be practical alternatives to conventionally-used media for routine amide coupling processes and would be highly beneficial for more environmentally benign synthesis programmes in both academia and industry.
Acknowledgements
We are grateful to GlaxoSmithKline and Sigma Aldrich for the kind donation of consumables. DSM wishes to thank the University of Strathclyde Knowledge Transfer Account for postdoctoral funding. Graham Inglis and Dr Simon MacDonald (GSK) are thanked for helpful discussions.
Notes and references
-
(a)
A. Greenberg, C. M. Breneman and J. F. Liebman, The Amide Linkage: Selected Structural Aspects in Chemistry, Biochemistry, and Material Science, Wiley, New York, 2000 Search PubMed
;
(b)
N. Sewald and H.-D. Jakubke, Peptides: Chemistry and Biology, Wiley-VCH Verlag GmbH, Weinheim, 2002 Search PubMed
;
(c) M. A. Fischbach and C. T. Walsh, Chem. Rev., 2006, 106, 3468 CrossRef CAS
;
(d) M. Funabashi, Z. Yang, K. Nonaka, M. Hosobuchi, Y. Fujita, T. Shibata, X. Chi and S. G. Van Lanen, Nat. Chem. Biol., 2010, 6, 581 CrossRef CAS
;
(e) M. Simonovic and T. A. Steitz, Biochim. Biophys. Acta, 2009, 1789, 612 CAS
.
- For reviews of amide coupling processes, see:
(a)
P. D. Bailey, I. D. Collier and K. M. Morgan, In Comprehensive Organic Functional Group Transformations, ed. A. R. Katritzky, O. Meth-Cohn and C. W. Rees, Pergamon, Cambridge, 1995, vol. 5, ch. 6 Search PubMed
;
(b) L. A. Carpino, M. Beyermann, H. Wenschuh and M. Bienert, Acc. Chem. Res., 1996, 29, 268 CrossRef CAS
;
(c) J. M. Humphrey and A. R. Chemberlin, Chem. Rev., 1997, 97, 2243 CrossRef CAS
;
(d) Y. S. Klausner and M. Bodansky, Synthesis, 1972, 453 CrossRef
;
(e) F. Albericio, R. Chinchilla, D. J. Dodsworth and C. Nájera, Org. Prep. Proced. Int., 2001, 33, 203 CAS
;
(f) P. Li and J. C. Xu, J. Pept. Res., 2001, 58, 129 CrossRef CAS
;
(g) D. T. Elmore, Amino Acids, Pept. Proteins, 2002, 33, 83 Search PubMed
;
(h) F. Albericio, Curr. Opin. Chem. Biol., 2004, 8, 211 CrossRef CAS
;
(i) S.-Y. Han and Y.-A. Kim, Tetrahedron, 2004, 60, 2447 CrossRef CAS
;
(j) C. A. G. N. Montalbetti and V. Falque, Tetrahedron, 2005, 61, 10827 CrossRef CAS
;
(k)
P. D. Bailey, T. J. Mills, R. Pettercrew and R. A. Price, in Comprehensive Organic Functional Group Transformations II, ed. A. R. Katritzky and R. J. K. Taylor, Elsevier, Oxford, 2005, vol. 5, ch. 7 Search PubMed
;
(l) J. W. Bode, Curr. Opin. Drug. Discovery. Dev., 2006, 9, 765 CAS
;
(m) E. Valeur and M. Bradley, Chem. Soc. Rev., 2009, 38, 606 RSC
;
(n) A. El-Faham and F. Albericio, Chem. Rev., 2011, 111, 6557 CrossRef CAS
;
(o) V. R. Pattabiraman and J. W. Bode, Nature, 2011, 480, 471 CrossRef CAS
.
- T. W. J. Cooper, I. B. Campbell and S. J. F. MacDonald, Angew. Chem., Int. Ed., 2010, 49, 8082 CrossRef CAS
.
- S. D. Roughley and A. M. Jordan, J. Med. Chem., 2011, 54, 3451 CrossRef CAS
.
- For example, see:
(a) J. A. Mitchell and E. E. Reid, J. Am. Chem. Soc., 1931, 53, 1879 CrossRef CAS
and references therein; ;
(b) B. S. Jursie and Z. Zdravkovski, Synth. Commun., 1993, 23, 2761 CAS
.
- For example, see: L. Perreux, A. Loupy and F. Volatron, Tetrahedron, 2002, 58, 2155 Search PubMed
.
- It should be noted that catalytic amide bond formation techniques have also been extensively developed. For general examples, see ref. 2. For examples of boronic acid-catalysed processes, see:
(a) H. Charville, D. Jackson, G. Hodges and A. Whiting, Chem. Commun., 2010, 46, 1813 RSC
;
(b) T. Maki, K. Ishihara and H. Yamamoto, Tetrahedron, 2007, 63, 8645 CrossRef CAS
;
(c) K. Ishihara, S. Ohara and H. Yamamoto, J. Org. Chem., 1996, 61, 4196 CrossRef CAS
;
(d) P. Tang, Org. Synth., 2005, 81, 262 CAS
;
(e) K. Arnold, B. Davies, R. L. Giles, C. Grosjean, G. E. Smith and A. Whiting, Adv. Synth. Catal., 2006, 348, 813 CrossRef CAS
;
(f) K. Arnold, A. S. Batsanov, B. Davies and A. Whiting, Green Chem., 2008, 10, 124 RSC
;
(g) R. M. Al-Zoubi, O. Marion and D. G. Hall, Angew. Chem., Int. Ed., 2008, 47, 2876 CrossRef CAS
;
(h) K. Ishihara, Tetrahedron, 2009, 65, 1085 CrossRef CAS
;
(i) T. Marcelli, Angew. Chem., Int. Ed., 2010, 49, 6840 CrossRef CAS
. For boronic ester-based processes, see: ;
(j) P. Starkov and T. D. Sheppard, Org. Biomol. Chem., 2011, 9, 1320 RSC
. Catalytic amidation of alcohols and amines is also highly effective, see: ;
(k) C. Gunanathan, Y. Ben-David and D. Milstein, Science, 2007, 317, 790 CrossRef CAS
;
(l) L. U. Nordstrøm, H. Vogt and R. Madsen, J. Am. Chem. Soc., 2008, 130, 17672 CrossRef CAS
;
(m) A. J. A. Watson, A. C. Maxwell and J. M. J. Williams, Org. Lett., 2009, 11, 2667 CrossRef CAS
;
(n) S. C. Ghosh, S. Muthaiah, Y. Zhang, X. Xu and S. H. Hong, Adv. Synth. Catal., 2009, 351, 2643 CrossRef CAS
.
- For example, see ref. 2j and 2k.
- SciFinder, American Chemical Society, Search conducted November 19th 2012, 2012.
-
(a) R. K. Henderson, C. Jiménez-González, D. J. C. Constable, S. R. Alston, G. G. A. Inglis, G. Fisher, J. Sherwood, S. P. Binks and A. D. Curzons, Green Chem., 2011, 13, 854 RSC
;
(b) D. J. C. Constable, C. Jiménez-González and R. K. Henderson, Org. Process Res. Dev., 2007, 11, 133 Search PubMed
;
(c) K. Alfonsi, J. Colberg, P. J. Dunn, T. Fevig, S. Jennings, T. A. Johnson, H. P. Kleine, C. Knight, M. A. Nagy, D. A. Perry and M. Stefaniak, Green Chem., 2008, 10, 31 RSC
;
(d) B. W. Cue and J. Zhang, Green Chem. Lett. Rev., 2009, 2, 193 Search PubMed
.
- D. S. MacMillan, J. Murray, H. F. Sneddon, C. Jamieson and A. J. B. Watson, Green Chem., 2012, 14, 3016 RSC
.
-
(a) A. El-Faham, R. Subirós-Funosas, R. Prohens and F. Albericio, Chem.–Eur. J., 2009, 15, 9404 CrossRef CAS
. See also: ;
(b) A. El-Faham and F. Albericio, J. Org. Chem., 2008, 73, 27 CrossRef CAS
;
(c) R. Subirós-Funosas, R. Prohens, R. Barbas, A. El-Faham and F. Albericio, Chem.–Eur. J., 2009, 15, 9394 CrossRef CAS
;
(d)
http://www.sigmaaldrich.com/chemistry/chemical-synthesis/technology-spotlights/comu.html
;
(e) M. Junkers, Aldrich ChemFiles, 2010, 10, 1 Search PubMed
.
-
(a) N. L. Benoiton and F. M. F. Chen, J. Chem. Soc., Chem. Commun., 1981, 543 RSC
;
(b) M. Junkers, Aldrich ChemFiles, 2007, 7.2, 5 Search PubMed
.
-
(a) I. Abdelmoty, F. Albericio, L. A. Carpino, B. M. Forman and S. A. Kates, Lett. Pept. Sci., 1994, 1, 57 Search PubMed
;
(b) M. Junkers, Aldrich ChemFiles, 2007, 7.2, 9 Search PubMed
.
-
(a) J. Coste, D. Le Nguyen and B. Castro, Tetrahedron Lett., 1990, 31, 205 CrossRef
;
(b) M. Junkers, Aldrich ChemFiles, 2007, 7.2, 8 Search PubMed
.
-
(a) H. Wissmann and H.-J. Kleiner, Angew. Chem., Int. Ed. Engl., 1980, 19, 133 CrossRef
;
(b) R. Escher and P. Bünning, Angew. Chem., Int. Ed. Engl., 1986, 25, 277 CrossRef
;
(c)
http://www.archimica.com/pdf/archimica_t3p_brochure.pdf
;
(d)
http://www.archimica.com/pdf/archimica_t3p_datasheet.pdf
;
(e) M. Junkers, Aldrich ChemFiles, 2007, 7.2, 11 Search PubMed
.
- For selected further information relating to the solvents used in this study, see:
(a)
http://www.sigmaaldrich.com
;
(b) K. Watanabe, N. Yamagiwa and Y. Torisawa, Org. Process Res. Dev., 2007, 11, 251 Search PubMed
;
(c)
http://www.zeon.co.jp
;
(d)
R. Aul and B. Comanita, Manufacturing Chemist, May 2007, 33;
(e) J. Bian, M. Xiao, S. Wang, Y. Lu and Y. Meng, Catal. Commun., 2009, 10, 1142 CrossRef CAS
;
(f)
M. Winterberg, E. Schulte-Korne, U. Peters and F. Nierlich, Methyl tert-butyl ether, in Ullmann's Encyclopedia of Industrial Chemistry, Wiley-VCH, 2010 Search PubMed
;
(g) V. Antonucci, J. Coleman, J. B. Ferry, N. Johnson, M. Mathe, J. P. Scott and J. Xu, Org. Process Res. Dev., 2011, 15, 939 Search PubMed
;
(h) M. Nielsen, H. Junge, A. Kammer and M. Beller, Angew. Chem., Int. Ed., 2012, 51, 5711 Search PubMed
.
- The KNIME program (http://www.knime.org) was used to determine the relevant physicochemical parameters. For further information, see the ESI.†.
Footnote |
† Electronic supplementary information (ESI) available: Experimental procedures, analytical data, charts of conversion vs. time for all substrates in all solvents and for all coupling agents. See DOI: 10.1039/c2gc36900a |
|
This journal is © The Royal Society of Chemistry 2013 |
Click here to see how this site uses Cookies. View our privacy policy here.