DOI:
10.1039/C3FO60380F
(Paper)
Food Funct., 2014,
5, 65-73
Responsiveness of emulsions stabilized by lactoferrin nano-particles to simulated intestinal conditions
Received
2nd September 2013
, Accepted 27th October 2013
First published on 28th October 2013
Abstract
There is an upsurge of interest in the use of nano-particles to fabricate emulsions and modulate their functionality, with particular emphasis on modulating emulsion digestive fate. Food grade nano-particles formed through controlled processing and electrostatic biopolymer interactions are yet to be systematically studied for their ability to stabilize emulsions and modulate emulsion digestibility. This study focused on the responsiveness of emulsions stabilized by lactoferrin (LF) nano-particles (NPs) and dietary fibers to key digestive parameters. Compared to native LF, LF-NPs comprised emulsion exhibited elevated creaming rates as evident from accelerated stability tests performed by analytical centrifugation. The electrostatic deposition of alginate or carrageenan onto the LF-NPs significantly improved the stability of the corresponding emulsions. Further, the use of various nano-particles showed to have both beneficial and deleterious effects on emulsion responsiveness to pH (2.0 < pH < 10.0), CaCl2 (0–40 mM) and bile (0–25 mg mL−1). Simulated pH-stat lipolysis experiments show that the use of LF or LF-NPs had no marked effect on lipolysis. Intriguingly, the use of LF-NPs and alginate reduced emulsion lipolysis by 14% while the use of LF-NPs and carrageenan increased lipolysis by 10%. Microscopy images as well as droplet characterization in terms of size and charge indicate that the altered emulsion responsiveness may be due to physical differences in emulsion properties (e.g. droplet size) and overall organization during digestion (e.g. aggregation vs. coalescence). Overall, this study's insights could prospectively be used to harness protein nano-particles to tweak emulsion behavior during digestion.
1. Introduction
Functional foods are a vivid field of research seeking to nutritionally intervene, alleviate or prevent modern morbidity, such as obesity, coronary heart disease and diabetes. In recent years, there has been an upsurge of interest in rational design and product engineering to incorporate more bioactive ingredients into our diet, to maximize the advantages of food processing and enhance food's contribution to human health exceeding its nutritional value.1 Emulsions are extremely common in foods and have been enthusiastically studied for their potential to serve as efficient and cost effective delivery systems for lipophilic bioactive components.1–5 However, emulsions' inherent thermodynamic instability renders the use of emulsifiers and/or stabilizers a practical necessity in the manufacturing of kinetically stable emulsions.5 Thus, the modification of emulsifiers and of droplet interfacial properties has been documented to affect emulsions' susceptibility to various stresses and even to manipulate their digestive fate.3,5–8
The ever existing need to overcome the limitations of current emulsion formulations maintains a constant need for innovations in the field. Recently, the use of solid colloids to form and stabilize emulsions, also termed Pickering emulsions, has been increasingly described in the literature.9,10 These unique colloidal systems offer the possibility to fabricate emulsions with distinctly improved physical and chemical stability, even from food-complaint colloids, such as polysaccharide nano-particles (NPs).9,11–13 Such emulsions are uniquely stabilized by colloids practically irreversibly adsorbed onto the droplet interface providing marked steric hindrance.14 The effectiveness of colloids to generate such unique physical barriers depends on various parameters such as oil type, particle size, inter-particle interactions, and particle wettability.9,15 Various food compliant hydrocolloids have recently been described in the literature, including starch granules, chitin nano-crystals, cellulose nano-crystals, solid lipid nanoparticles and fibrils.9–13,16–20
Protein–polysaccharide complex NPs formed through electrostatic biopolymer interactions have also been recently described as potential Pickering emulsion stabilizers.21 The formation and stability of such structured food NPs have even been recently reviewed.22 Moreover, the incorporation of proteins into emulsion systems is thought to potentially offer additional health benefits, as some alimentary proteins have been demonstrated to have specific bioactivities, such as antimicrobial, antioxidant, antihypertensive or immune-modulating activities.23,24 In addition, certain polysaccharides have also been shown to possess bioactivity. For example, indigestible carbohydrates classified as dietary fibers are associated with many potential health benefits, including modulation of colon flora, prevention of some cancer types and are even thought of as therapeutic targets tackling obesity and metabolic syndromes.25,26 Thus, various bioactive proteins and carbohydrates in emulsions can be used to form multifunctional emulsions in which the lipid droplet may deliver lipophilic bioactives and the formulation stabilizers may provide additional bioactive components to the formulation. Further, the integration of proteins and polysaccharides into emulsion formulations can be efficient tools in modulating the droplet interfacial composition and properties and consequently modulating emulsion digestibility in the human gastrointestinal tract (GIT).27–29 Still, little is known on the role of protein-based NPs in stabilizing emulsions and modulating emulsion responsiveness to the conditions of the GIT which govern the emulsion digestive fate.
Recent studies have demonstrated the conditions and properties of different lactoferrin nano-particles formed via thermal processing and pH treatments, based on the high isoelectric point of lactoferrin (pI > 8.0).30–32 Such LF-based NPs have also been shown to exhibit altered susceptibility to gastric proteolysis.29 Most recently, we have demonstrated that emulsions stabilized by certain LF-based NPs may exhibit altered responsiveness to artificial saliva and in vitro gastric digestion.21 Overall, these studies have shown that certain polysaccharides can interfere with gastrointestinal proteolysis and at the same time be useful as emulsifiers yielding emulsions with varying functionalities and susceptibility to oral and gastric conditions. Thus, the present study was dedicated to expand our understanding on the responsiveness of emulsions stabilized by lactoferrin–dietary fiber NPs to key physiological parameters of the small intestine.
2. Materials and methods
2.1. Materials
Food grade bovine lactoferrin (Vivinal lactoferrin FD, 95.6% protein, <120 ppm minerals) was kindly donated by DMV International (Delhi, NY, USA); the polysaccharides (PS) used were of GENUGEL® iota-carrageenan type CJ kindly donated by CPKelco. Olive oil was purchased from a local supermarket. CaCl2, alginic acid (sodium salt) from brown algae and type II porcine pancreatic lipase (≥100 units per mg protein) were purchased from Sigma-Aldrich (Rehovot, Israel) whereas bile extract from porcine was purchased from Chem Cruz (Santa Cruz Biotechnology INC). All reagents and chemicals used were of analytical grade.
2.2. Production of NPs and NP stabilized emulsions
Fabrication of lactoferrin nano-particles.
Particulate production was done through controlled heat treatment (90 °C, 20 min) followed by pH control (elevation from pH = 7.0 to pH = 8.0) to attain three types of NPs (np): (a) LF NPs (LFnp), (b) LF NPs with alginate (LF–ALG), and (c) LF NPs with iota-carrageenan (LF–CAR), as previously described in detail.29,32 In brief, 0.4% (w/w) LF solutions were heated and pH treated to form NPs and then mixed with equal volumes of 0.4% (w/w) polysaccharide solutions to yield 0.2% (w/w) NP solutions used to produce the subject emulsions. Unprocessed native LF was used as the control.
Production of emulsions stabilized by NPs.
Emulsification of olive oil with NP suspensions was done through two processing stages: first a premix was formed through high shear blending and then emulsions were produced through high pressure homogenization of the premix. Premix samples were produced via high shear homogenization of water–oil mixtures (olive oil 2% w/w–LF/LFnp 0.2% w/w in double distilled water (DDW) at pH 7.0) for 1 min at 35
000 RPM using a hand blender (PRO 200, BIOGEN series pro scientific, Oxford CT, USA). Emulsions were produced by passing premix samples 4 times through a high pressure homogenizer (Micro DeBEE air operated, BEE International, MA, USA) operated at 103 MPa. This production scheme has also been illustrated and described in detail in a recent publication.21 Emulsions were subjected to various analyses within less than three days from production and were stored in a refrigerator at 4 °C until used.
2.3. Characterization of emulsion properties
Emulsion characterization focused on physical stability, size, charge and overall appearance. The physical stability of emulsions to creaming was monitored using an analytical centrifugal analyzer (LUMisizer, L.U.M. GmbH, Berlin, Germany). For this purpose, rectangular cells were loaded with emulsion samples whose time and space-resolved transmission extinction profiles were monitored by analytical centrifugation. This has been applied to evaluate emulsion creaming velocities, as previously described.21,33 Practically, 400 μL of non-diluted emulsions were loaded into rectangular cells whose transmission extinction profiles were recorded under 2000 RPM at 23 °C for 8.5 hours.
Laser scattering characterization of emulsions.
Static laser light scattering (Malvern Mastersizer 2000, Malvern Instruments, Worcestershire, UK) was used to characterize emulsions' droplet sizes. Refractive indices used for the water and olive oil phases were 1.333 and 1.549, respectively, and the size information was given by the mean volume-weighed droplet diameter (
, where ni is the number of droplets of diameter di) and particle size distributions were based on droplet volumes. Dynamic laser scattering coupled with capillary electrophoresis (Zetasizer Nano ZS series, Malvern Instruments, Worcestershire, UK) was applied to determine the electrophoretic mobility of emulsion droplets. These values were translated into electrokinetic charge (ζ-potential) using the Smoluchowski model, as described in a previous study.6,34,35 In practice, emulsion samples were diluted in DDW in pH 7.0 at a ratio of 1
:
50 (v/v) and then filled into the test cell. After 60 seconds of equilibration in the instrument, the data were collected from at least 10 sequential readings per sample and the ζ-potential was calculated using the instrument.
Direct observation of emulsions.
Emulsions were visually inspected using a Cell Observer-Zeiss Axiovert 200 inverted microscope. The lipid phase of the emulsions was dyed using Nile red (0.1 mg mL−1 Nile red in ethanol which is diluted 1
:
100 before use with the tested emulsion) and incubated for 10 min before being placed on glass microscope slides and covered. Images were processed using the AxioVision (Zeiss) image analysis software for acquisition and image processing. Additionally, direct images of emulsions were obtained using a Nikon camera (COOLPIX P500).
2.4. Responsiveness to an ionic environment
During digestion pH and CaCl2 are amongst the effectors of emulsion stability.36–38 The emulsion stability to pH was evaluated between 2.0 and 10.0 by overnight incubation of emulsions at ambient temperature following pH adjustment to various pH levels (2, 4, 6, 8, and 10). The adjustment of pH was attained using controlled volumes of HCl and NaOH ranging from 0.05 M to 1 M until reaching the desired value. Similarly, the emulsion stability to CaCl2 was pursued through addition of controlled volumes of a 400 mM CaCl2 stock solution to reach final sample conditions of 1.8% (w/w) oil and CaCl2 concentrations of 0–40 mM. Following an overnight equilibration period, samples were tested for size, ζ-potential and physical stability.
2.5. Responsiveness to bile and lipolysis
The role of bile and pancreatic lipases is detrimental to the emulsion stability during digestion.6,38,39 As emulsifier displacement by bile plays a role in lipid digestion,34,40,41 emulsion properties were examined under various bile concentrations (0–25 mg mL−1), even exceeding physiological levels. Emulsion responsiveness to these conditions was assessed based on measuring the changes in the ζ-potential, as has recently been done.34,40
Monitoring the lipolysis rate and extent using a pH stat methodology.
A pH STAT protocol was carried out using an auto-titration unit (Titrando 902, Metrohm, Switzerland) in a heated jacketed reactor (maintained at 37 °C), continuously stirred (230 RPM) with pH held constant at pH = 7.00. This was done using “TIAMO 2.3” software (Metrohm, Switzerland) and controlled volumes of 50 mM NaOH, based on previous reports with minor adjustments.34,37,42–44 Emulsion samples were diluted with pH 7.0 double distilled water (their continuous phase) to a final volume of 30 mL containing 0.5% oil. The solution was re-adjusted to pH 7.00 and heated for 10 minutes to 37 °C. Then 4 mL of bile solution containing 187.5 mg bile extract (final concentration of 5 mg mL−1) and 1 mL CaCl2 solution calculated to give a final concentration of 20 mM were added to the reactor. All mentioned solutions added were dissolved in 5 mM phosphate buffer pH 7.0 and pre-incubated for 10 minutes in a 37 °C water bath. Eventually, a freshly prepared 2.5 mL lipase solution containing 297 mg lipase (590 units per mL in final solution) was added. The pH was adjusted using minimal volumes of HCl and NaOH solutions using 1 M, 0.5 M and 0.05 M, as needed. Once the lipase solution was added to the reactor the pH stat control program was initiated. The percentage of free fatty acids (FFAs) released during pH stat lipolysis was determined through eqn (1): | 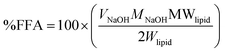 | (1) |
where VNaOH is the volume of NaOH required for neutralizing the released FFA (in mL), MNaOH is the molarity of NaOH (mole per liter), MWlipid is the molecular weight of the oil and Wlipid is the initial weight of oil in the reactor.
2.6. Experimental design and analysis
All experiments were carried out in triplicate with each sample measured thrice, and results are presented as the calculated mean and standard deviation. Statistical analyses were performed using Microsoft Excel 2010 data analysis tool pack and mainly relied on t-tests assuming equal variances and ANOVA single factors.
3. Results and discussion
Considerable advances have been made with respect to emulsion design and the tailoring of emulsion digestive fate.3,5 Pickering emulsions stabilized by nano-and micro-particles exhibit unique behavior and potential applications to foods.10,45 However, scarce reports have addressed the responsiveness of such colloid-stabilized emulsions to the physiological conditions of the human GIT.21,42 This study aimed to extend the understanding of Pickering emulsions stabilized by proteinaceous nano-particles in relation to their stability and responsiveness to physiological levels of pH, CaCl2, bile and intestinal lipolysis.
3.1. Emulsion susceptibility to pH
Due to the marked changes in pH during digestion, initial experiments evaluated the responsiveness of the various emulsions to a range of pH values. Following an equilibration period, the particle size distribution curves of the emulsions were calculated and are presented in Fig. 1 along with direct observations of samples collected through fluorescent microscopy (Fig. 2).
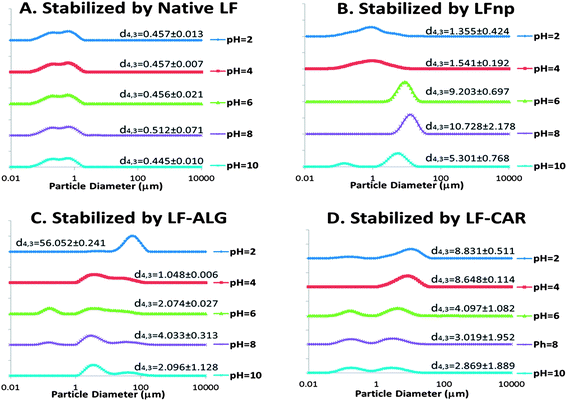 |
| Fig. 1 Particle size distribution (PSD) curves of different emulsions under various pH values. [A] Emulsion stabilized by native lactoferrin (LF). [B] Emulsion stabilized by LF nano-particles (LFnp). [C] Emulsion stabilized by lactoferrin nano-particles and alginate (LF–ALG). [D] Emulsion stabilized by lactoferrin nano-particles and carrageenan (LF–CAR). | |
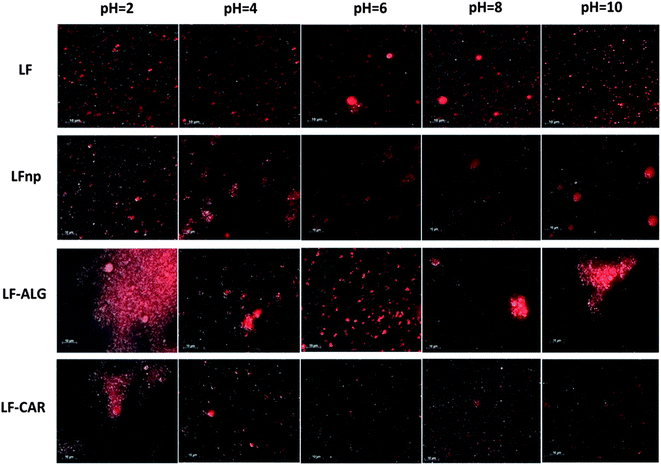 |
| Fig. 2 Fluorescent microscopy images (scale bar size 10 μm) of different emulsions under various pH values. Emulsions stabilized by native lactoferrin (LF), LF nano-particles (LFnp), lactoferrin nano-particles and alginate (LF–ALG) or lactoferrin nano-particles and carrageenan (LF–CAR). | |
These experiments gave rise to two major observations. First, the processing of LF into LF-based nano-particles was found to hamper its emulsifying properties, yielding emulsions with increased droplet sizes and overall poorer physical stability to changes in pH (Fig. 1A vs. B) and concurring with recent findings.21 The addition of alginate or iota-carrageenan to the NPs was found to improve the pH stability of emulsions at 6 ≤ pH ≤ 10, as deemed from the smaller droplets found in these emulsions (Fig. 1C and 1D). However, at low pH values of four or below, the addition of alginate and iota-carrageenan to the NPs resulted in emulsions with poorer physical stability and increased droplet sizes compared to corresponding emulsions stabilized merely by LF NPs (Fig. 1B). Moreover, the direct observation of the emulsions showed that emulsion instability in these formulations was due to droplet aggregation (Fig. 2). These microscopy images corroborated that the nature of this flocculation behavior was pH dependent and that the extent of the instability varied between formulations.
The stability of protein-stabilized emulsions is commonly attributed to stem from electrostatic repulsion. Moreover, the stability of the NPs is governed by electrostatic biopolymer interactions.22 Hence, the ζ-potential of emulsions was measured to determine the droplet surface charge under various conditions (Fig. 3). According to these findings emulsions stabilized by LF or LFnp had diminished ζ-potentials at 6 ≤ pH ≤ 10 where emulsions were found to have poor physical stability. Under the same environmental conditions, emulsions stabilized by LFnp and dietary fibers were found to have significantly charged droplets (ζ < −40 mV) which may explain the improved physical stability of these emulsions. Concomitantly, under acidic conditions (2 ≤ pH ≤ 4) the addition of dietary fibers did not yield substantial ζ-potentials, hence providing a possible explanation to the observed poor physical stability of the emulsions under these conditions. Studies show the pKa of the carboxylic acids on the two monomers comprising alginate, D-mannuronic and L-gulluronic to be 3.38 and 3.65, respectively, and may explain the formation of soft gels or precipitates.46,47 This suggests that under similar acidic conditions alginate is more likely to be protonated thereby abolishing its electrostatic interactions with other charged species such as LF. Similarly, the reported pKa of the two sulfate groups of iota-carrageenan is around pH = 2.0,48 which could explain the improved stability of the LF–CAR complex compared to the LF–ALG complex, hence providing emulsions with a slightly improved physical stability, as evident from the size analyses (Fig. 1D), and general organization directly observed (Fig. 2). The alterations in the electrostatic interactions within the emulsion formulations may also explain the observed flocculation which could be due to bridging flocculation.
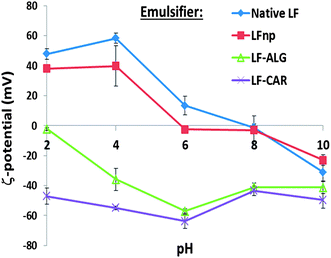 |
| Fig. 3
ζ-Potential values under pH = 2–10 of emulsions stabilized by native lactoferrin (LF), LF nano-particles (LFnp), lactoferrin nano-particles and alginate (LF–ALG) or lactoferrin nano-particles and carrageenan (LF–CAR). | |
To better quantitate and compare emulsion stability, the physical stability of non-diluted samples was monitored by analytical centrifugation which enabled calculation of sample creaming velocities (Fig. 4). These experiments showed that the use of LF NPs significantly (p < 0.05) hampered the emulsion stability and increased creaming rates compared to emulsions stabilized by native LF and that dietary fiber addition improved emulsion stability, as has been recently shown.21 These results (Fig. 4) further support the observations made regarding the physical stability of emulsions to pH (Fig. 1 and 2). Particularly, this analysis shows that emulsions stabilized by LF–ALG at pH = 2.0 had a marked increase in size (Fig. 1C), flocculation (Fig. 2), drop in ζ-potential values (Fig. 3) and accelerated creaming (Fig. 4). Overall, these experiments provide yet another quantitative indication showing that the various NPs can have varying effects on the physical stability of emulsions. Such differences could prove useful in prospective attempts to tweak emulsion physical stability.
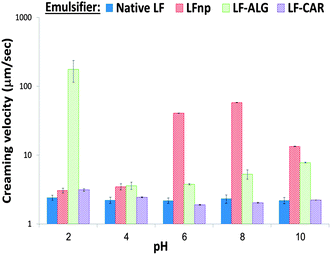 |
| Fig. 4 Calculated creaming velocity of emulsions stabilized by native lactoferrin (LF), LF nano-particles (LFnp), lactoferrin nano-particles and alginate (LF–ALG) or lactoferrin nano-particles and carrageenan (LF–CAR) based on time and space resolved transmission extinction profiles by analytical centrifugation. Data are presented as triplicate means ± SD. | |
3.2. Emulsion susceptibility to CaCl2
The presence of divalent calcium ions is known to affect emulsion stability during storage and digestion.49,50 In particular, some studies show that emulsions stabilized by LF are affected by the presence of calcium ions at levels exceeding 30 mM of CaCl2.34,51,52 The observed changes in emulsion droplet size (Fig. 5A), creaming velocity (Fig. 5B) and ζ-potential (Fig. 5C) under various CaCl2 concentrations (0–40 mM) are presented in Fig. 5. As can be seen, native LF stabilized emulsions remained relatively stable at 0–20 mM CaCl2 in terms of size and creaming velocity, with a minor increase at 40 mM (Fig. 5A and B). Previous studies demonstrated a significant elevation in d4,3 of LF stabilized emulsions after overnight exposure to at least 50 mM CaCl2.51,53 Surprisingly, LFnp stabilized emulsions were highly susceptible to Ca2+ ions exceeding 2 mM as evident from the pronounced rise in droplet size (p < 0.0003) (Fig. 5A) and creaming velocity (p < 0.005) (Fig. 5B). The corresponding measurements of ζ-potentials (Fig. 5C) provide further insight into these observations. While LF-stabilized emulsion had a ζ-potential between 20 mV and 31 mV supporting the electrostatic stabilization of the emulsion, emulsions stabilized by LFnp had reduced charge (between 13 mV and 20 mV) providing a possible explanation for the reduced physical stability observed. These differences may arise from the thermal processing of LF and its controlled aggregation into LFnp which alter the protein structure and consequently its interactions with its surroundings. Altogether, CaCl2 appears to similarly change the droplet charge, however, due to the different starting points for these changes the impact on emulsion stability differs.
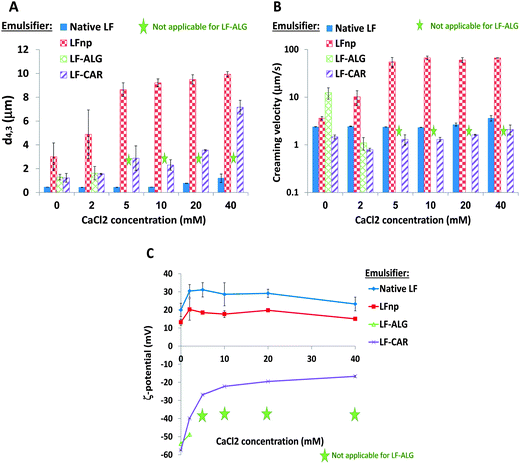 |
| Fig. 5 Properties of emulsions stabilized by native lactoferrin (LF), LF nano-particles (LFnp), lactoferrin nano-particles and alginate (LF–ALG) or lactoferrin nano-particles and carrageenan (LF–CAR) at different concentrations of CaCl2. [A] Mean droplet size (d4,3). [B] Calculated creaming velocity and [C] ζ-potentials. Results are presented as triplicate means and samples marked in green asterisks (*) are for LF–ALG stabilized emulsions that could not be determined due to extensive instability. | |
In the case of alginate, emulsions stabilized by LF–ALG could only be taken into account for low concentrations of CaCl2 as at concentrations exceeding 5 mM CaCl2 samples tended to gel and sediment, as also noted by others.54,55 Therefore, the results received from CaCl2 concentrations of 5–40 mM for those emulsions were excluded and are not presented. In contrast, the use of carrageenan enabled the formation of LF–CAR stabilized emulsions with pronounced negative ζ-potentials and so they are likely to attract Ca2+ ions and reduce the droplet surface charge. This could explain the observed increase in size and in ζ-potential with an increase of CaCl2 concentration (Fig. 5A and C). Moreover, iota-carrageenan tends to gel in the presence of Ca2+ due to cross-linking through structural spirals containing adjacent sulphate groups.56 This could explain the observed physical stability of the emulsion to phase separation (Fig. 5B).
3.3. Emulsion susceptibility to bile
One of the key physiological events during foam and emulsion digestion is their interaction with bile, held to be elemental in the process of emulsifier displacement and the interfacial adsorption of lipases during lipolysis.39,57 This part of the work sought to evaluate the effect of bile on different emulsion formulations, particularly as it pertained to the emulsion droplet charge indicative of the droplet interfacial composition and competitive displacement of the emulsifier by bile, as previously shown.34Fig. 6 summarizes the effect of increasing bile concentrations (0–25 mg mL−1) on the ζ-potential of the investigated Pickering emulsions. First, the addition of 5 mg mL−1 or more of bile was found to lead to droplet charge reversal for emulsions stabilized LF or LFnp, without any pronounced differences between these emulsions. This supports the notion that bile successfully altered the droplet interfacial properties and that LF particulation into NPs did not affect this process. Another possible explanation may be the formation of a secondary layer of negatively charged bile on top of the positively charged LF/LFnp emulsifier. When applying protein–polysaccharide NPs to stabilize emulsions slight changes in ζ-potentials were recorded and droplets became less negatively charged. In this case, bile displacement seems to be the most likely mechanism explaining these findings, particularly as all of the tested emulsions were found to have a similar droplet charge when adding high levels of bile. Thus, the collected evidence suggests that the various nano-particles did not significantly alter the susceptibility of emulsions to bile.
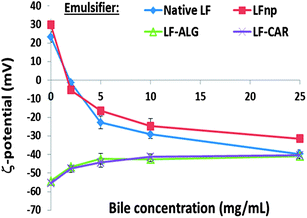 |
| Fig. 6
ζ-Potential values of emulsions stabilized by native lactoferrin (LF), LF nano-particles (LFnp), lactoferrin nano-particles and alginate (LF–ALG) or lactoferrin nano-particles and carrageenan (LF–CAR) at different bile concentrations. | |
3.4. Emulsion responsiveness to lipolysis
Alimentary lipids entail high caloric values and affect satiety; thus, efforts are being made to modulate digestive lipolysis of hydrocolloid stabilized systems through various chemical and physical strategies.3,39,58,59 To date, it has been shown that the rate and extent of fat digestion in emulsions is highly dependent on physicochemical characteristics of the droplet interfacial layer.7,60 In this respect, monitoring the FFA release during simulated small intestinal digestion under a pH-stat methodology offers an opportunity to investigate the rate and extent of emulsion lipolysis.37 Accordingly, the FFA release profiles obtained during the lipolysis of various emulsions are presented in Fig. 7 which also includes light microscopy images of fully digested samples. As can be seen, no significant differences could be noted between the lipolysis of emulsions stabilized by native LF to emulsions stabilized by LF nano-particles. However, direct microscopy images indicate that emulsions were liable to both coalescence and aggregation with emulsions stabilized by LFnp being more prone to aggregation during the experiments without significant ramifications to the rate and extent of lipolysis.
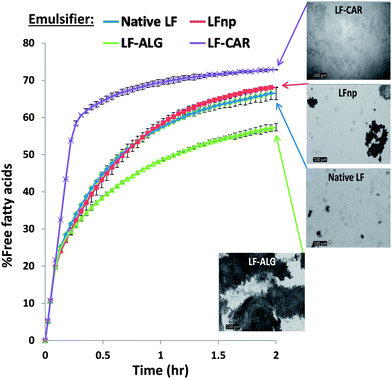 |
| Fig. 7 Free fatty acid (FFA) release profiles during pH-stat lipolysis of emulsions stabilized by native lactoferrin (LF), LF nano-particles (LFnp), lactoferrin nano-particles and alginate (LF–ALG) or lactoferrin nano-particles and carrageenan (LF–CAR). Inset: concomitant light microscopy of fully digested emulsion samples. | |
Interestingly, the combination of LFnp with dietary fibers to stabilize emulsions showed to have pronounced effects on the rate and extent of lipolysis. These emulsions differed from emulsions solely stabilized by LF or LFnp. On one hand, the use of alginate in the emulsion formulation significantly reduced the rate and extent of lipolysis by ∼14%. This finding can be explained by the physical seclusion of the lipid by alginate physical cross-linking in the presence of physiological levels of CaCl2. This is also supported by the findings of others showing that alginate encapsulation of emulsion droplets can deter their digestion.37 Microscopy images (Fig. 7 – inset) further show that lipolysis entailed considerable droplet aggregation which significantly reduce the droplet surface
:
volume ratio. This could very well explain the reduced extent of lipolysis which has been well documented to be a reaction occurring at the droplet interface.39 On the other hand, the use of iota-carrageenan elevated the rate and extent of lipolysis by ∼10%. Concomitant light microscopy images (Fig. 7 – inset) show that LF–CAR stabilized emulsions were prone to mild aggregation with no evidence supporting coalescence, unlike emulsions stabilized by LF–ALG. Thus, it seems that the effects of dietary fiber addition on emulsion lipolysis can be explained by physical changes in emulsion properties (e.g. droplet size) and overall organization (e.g. aggregation vs. coalescence). Altogether, such differences lead to variations in the lipid surface area available for lipolytic attack and further substantiate that these emulsions could have different digestibilities in the human GIT. However, further experiments are required to ascertain the additive effect of preceding digestive events, i.e. mastication and gastric digestion.
4. Conclusion
Oil in water emulsions stabilized by LF nano-particles have recently been shown to have different behaviors when exposed to artificial saliva and simulated gastric conditions.21 The findings of this study show that these emulsions have altered responsiveness to pH, CaCl2, bile and lipolysis, yet evidence is needed to establish the combined effects of digestive events more closely mirroring the serial events of human digestion. It seems that the formation of LF nano-particles hampers the emulsifying properties of the protein for sub-micron sized droplets but the addition of alginate or carrageenan further modifies the emulsion responsiveness with both beneficial and deleterious effects, e.g. delayed or accelerated lipolysis. Overall, this work provides evidence that could prospectively facilitate the use of protein nano-particles to modulate emulsion behavior during digestion.
Acknowledgements
This research was supported by the Technion funding through the Russell Berrie Nanotechnology Institute and the Rubin Scientific and Medical Research Fund. Ms Meshulam would like to thank Ms Gal Shimoni and Dr Nitzan Dahan for their technical assistance.
References
- R. C. Benshitrit, C. S. Levi and S. L. Tal,
et al., Development of oral food-grade delivery systems: current knowledge and future challenges, Food Funct., 2012, 3, 10–21 CAS.
- D. J. McClements, E. A. Decker and J. Weiss, Emulsion-based delivery systems for lipophilic bioactive components, J. Food Sci., 2007, 72(8), R109–R124 CrossRef CAS PubMed.
- H. Singh, A. Q. Ye and D. Horne, Structuring food emulsions in the gastrointestinal tract to modify lipid digestion, Prog. Lipid Res., 2009, 48(2), 92–100 CrossRef CAS PubMed.
- K. P. Velikov and E. Pelan, Colloidal delivery systems for micronutrients and nutraceuticals, Soft Matter, 2008, 4(10), 1964–1980 RSC.
-
D. J. McClements, Emulsion Design to Improve the Delivery of Functional Lipophilic Components, in Annual Review of Food Science and Technology, Annual Reviews, Palo Alto, 2010, vol. 1, 241–269 Search PubMed.
- U. Lesmes and D. J. McClements, Controlling lipid digestibility: Response of lipid droplets coated by [beta]-lactoglobulin-dextran Maillard conjugates to simulated gastrointestinal conditions, Food Hydrocolloids, 2012, 26(1), 221–230 CrossRef CAS PubMed.
- D. J. McClements and Y. Li, Structured emulsion-based delivery systems: Controlling the digestion and release of lipophilic food components, Adv. Colloid Interface Sci., 2010, 159(2), 213–228 CrossRef CAS PubMed.
- C. M. Oliver, M. A. Augustin and L. Sanguansri, Maillard-based casein–carbohydrate microcapsules for the delivery of fish oil: emulsion stability during in vitro digestion, Aust. J. Dairy Technol., 2009, 64(1), 80–83 CAS.
- E. Dickinson, Food emulsions and foams: Stabilization by particles, Curr. Opin. Colloid Interface Sci., 2010, 15(1–2), 40–49 CrossRef CAS PubMed.
- E. Dickinson, Use of nanoparticles and microparticles in the formation and stabilization of food emulsions, Trends Food Sci. Technol., 2012, 12(1), 4–12 CrossRef PubMed.
- M. Kargar, K. Fayazmanesh and M. Alavi,
et al., Investigation into the potential ability of Pickering emulsions (food-grade particles) to enhance the oxidative stability of oil-in-water emulsions, J. Colloid Interface Sci., 2012, 366(1), 209–215 CrossRef CAS PubMed.
- M. V. Tzoumaki, T. Moschakis and V. Kiosseoglou,
et al., Oil-in-water emulsions stabilized by chitin nanocrystal particles, Food Hydrocolloids, 2011, 25(6), 1521–1529 CrossRef CAS PubMed.
- M. Rayner, A. Timgren and M. Sjoo,
et al., Quinoa starch granules: a candidate for stabilising food-grade Pickering emulsions, J. Sci. Food Agric., 2012, 92(9), 1841–1847 CrossRef CAS PubMed.
- R. Aveyard, B. P. Binks and J. H. Clint, Emulsions stabilised solely by colloidal particles, Adv. Colloid Interface Sci., 2003, 100, 503–546 CrossRef.
- J. Giermanska-Kahn, V. Schmitt and B. P. Binks,
et al., A new method to prepare monodisperse Pickering emulsions, Langmuir, 2002, 18(7), 2515–2518 CrossRef CAS.
- R. Gupta and D. Rousseau, Surface-active solid lipid nanoparticles as Pickering stabilizers for oil-in-water emulsions, Food Funct., 2012, 3(3), 302–311 CAS.
- S. Frasch-Melnik, I. T. Norton and F. Spyropoulos, Fat-crystal stabilised w/o emulsions for controlled salt release, J. Food Eng., 2010, 98(4), 437–442 CrossRef CAS PubMed.
- S. Wang, Y. J. He and Y. Zou, Study of Pickering emulsions stabilized by mixed particles of silica and calcite, Particuology, 2010, 8(4), 390–393 CrossRef CAS PubMed.
- H. Adelmann, B. P. Binks and R. Mezzenga, Oil Powders and Gels from Particle-Stabilized Emulsions, Langmuir, 2012, 28(3), 1694–1697 CrossRef CAS PubMed.
- K. N. P. Humblet-Hua, G. Scheltens and E. van der Linden,
et al., Encapsulation systems based on ovalbumin fibrils and high methoxyl pectin, Food Hydrocolloids, 2011, 25(3), 569–576 CrossRef CAS PubMed.
- G. Shimoni, C. Shani Levi and S. Levi Tal,
et al., Emulsions stabilization by lactoferrin nano-particles under in vitro digestion conditions, Food Hydrocolloids, 2013, 33(2), 264–272 CrossRef CAS PubMed.
- O. G. Jones and D. J. McClements, Recent progress in biopolymer nanoparticle and microparticle formation by heat-treating electrostatic protein–polysaccharide complexes, Adv. Colloid Interface Sci., 2011, 167(1–2), 49–62 CrossRef CAS PubMed.
- D. Agyei and M. K. Danquah, Rethinking food-derived bioactive peptides for antimicrobial and immunomodulatory activities, Trends Food Sci. Technol., 2012, 23(2), 62–69 CrossRef CAS PubMed.
- R. Nagpal, P. Behare and R. Rana,
et al., Bioactive peptides derived from milk proteins and their health beneficial potentials: an update, Food Funct., 2011, 2(1), 18–27 CAS.
-
A. M. Elad and U. Lesmes, Nutritional Programming of Probiotics to Promote Health and Well-Being, in Probiotics, ed. E. C. Rigobelo, InTech, 2012 Search PubMed.
- H. A. Grabitske and J. L. Slavin, Gastrointestinal effects of low-digestible carbohydrates, Crit. Rev. Food Sci. Nutr., 2009, 49(4), 327–360 CrossRef CAS PubMed.
- L. Y. Chen, G. E. Remondetto and M. Subirade, Food protein-based materials as nutraceutical delivery systems, Trends Food Sci. Technol., 2006, 17(5), 272–283 CrossRef CAS PubMed.
- J. Parada and J. M. Aguilera, Food microstructure affects the bioavailability of several nutrients, J. Food Sci., 2007, 72(2), R21–R32 CrossRef CAS PubMed.
- T. David-Birman, A. Mackie and U. Lesmes, Impact of dietary fibers on the properties and proteolytic digestibility of lactoferrin nano-particles, Food Hydrocolloids, 2013, 31(1), 33–41 CrossRef CAS PubMed.
- C. Bengoechea, O. G. Jones and A. Guerrero,
et al., Formation and characterization of lactoferrin/pectin electrostatic complexes: Impact of composition, pH and thermal treatment, Food Hydrocolloids, 2011, 25(5), 1227–1232 CrossRef CAS PubMed.
- C. Bengoechea, I. Peinado and D. J. McClements, Formation of protein nanoparticles by controlled heat treatment of lactoferrin: Factors affecting particle characteristics, Food Hydrocolloids, 2011, 25(5), 1354–1360 CrossRef CAS PubMed.
- I. Peinado, U. Lesmes and A. Andres,
et al., Fabrication and Morphological Characterization of Biopolymer Particles Formed by Electrostatic Complexation of Heat Treated Lactoferrin and Anionic Polysaccharides, Langmuir, 2010, 26(12), 9827–9834 CrossRef CAS PubMed.
- D. Lerche and T. Sobisch, Direct and Accelerated Characterization of Formulation Stability, J. Dispersion Sci. Technol., 2011, 32(12), 1799–1811 CrossRef CAS.
- U. Lesmes, P. Baudot and D. J. McClements, Impact of Interfacial Composition on Physical Stability and In Vitro Lipase Digestibility of Triacylglycerol Oil Droplets Coated with Lactoferrin and/or Caseinate, J. Agric. Food Chem., 2010, 58(13), 7962–7969 CrossRef CAS PubMed.
- T. Schmelz, U. Lesmes and J. Weiss,
et al., Modulation of physicochemical properties of lipid droplets using [beta]-lactoglobulin and/or lactoferrin interfacial coatings, Food Hydrocolloids, 2011, 25, 1181–1189 CrossRef CAS PubMed.
- M. Hu, Y. Li and E. A. Decker,
et al., Role of calcium and calcium-binding agents on the lipase digestibility of emulsified lipids using an in vitro digestion model, Food Hydrocolloids, 2010, 24(8), 719–725 CrossRef CAS PubMed.
- Y. Li, M. Hu and D. J. McClements, Factors affecting lipase digestibility of emulsified lipids using an in vitro digestion model: Proposal for a standardised pH-stat method, Food Chem., 2011, 126(2), 498–505 CrossRef CAS PubMed.
- H. Singh and A. Sarkar, Behaviour of protein-stabilised emulsions under various physiological conditions, Adv. Colloid Interface Sci., 2011, 165(1), 47–57 CrossRef CAS PubMed.
- P. J. Wilde and B. S. Chu, Interfacial & colloidal aspects of lipid digestion, Adv. Colloid Interface Sci., 2011, 165(1), 14–22 CrossRef CAS PubMed.
- A. Sarkar, D. S. Horne and H. Singh, Interactions of milk protein-stabilized oil-in-water emulsions with bile salts in a simulated upper intestinal model, Food Hydrocolloids, 2010, 24(2–3), 142–151 CrossRef CAS PubMed.
- U. Lesmes and D. J. McClements, Controlling lipid digestibility: Response of lipid droplets coated by beta-lactoglobulin-dextran Maillard conjugates to simulated gastrointestinal conditions, Food Hydrocolloids, 2012, 26(1), 221–230 CrossRef CAS PubMed.
- M. V. Tzoumaki, T. Moschakis and E. Scholten,
et al., In vitro lipid digestion of chitin nanocrystal stabilized o/w emulsions, Food Funct., 2013, 4(1), 121–129 CAS.
- S. Sandra, E. A. Decker and D. J. McClements, Effect of interfacial protein cross-linking on the in vitro digestibility of emulsified corn oil by pancreatic lipase, J. Agric. Food Chem., 2008, 56(16), 7488–7494 CrossRef CAS PubMed.
- N. H. Zangenberg, A. Mullertz and H. G. Kristensen,
et al., A dynamic in vitro lipolysis model I. Controlling the rate of lipolysis by continuous addition of calcium, Eur. J. Pharm. Sci., 2001, 14(2), 115–122 CrossRef CAS.
- B. S. Murray, K. Durga and A. Yusoff,
et al., Stabilization of foams and emulsions by mixtures of surface active food-grade particles and proteins, Food Hydrocolloids, 2011, 25(4), 627–638 CrossRef CAS PubMed.
- K. I. Draget, G. S. Braek and O. Smidsrod, Alginic Acid Gels – the Effect of Alginate Chemical-Composition and Molecular-Weight, Carbohydr. Polym., 1994, 25(1), 31–38 CrossRef CAS.
-
K. I. Draget, O. A. Smidsrød and G. Skjåk-Bræk, Alginates from Algae, in Biopolymers Online, Wiley-VCH Verlag GmbH & Co. KGaA, 2005 Search PubMed.
- Y. S. Gu, E. A. Decker and D. J. McClements, Influence of pH and iota-carrageenan concentration on physicochemical properties and stability of beta-lactoglobulin-stabilized oil-in-water emulsions, J. Agric. Food Chem., 2004, 52(11), 3626–3632 CrossRef CAS PubMed.
- A. Q. Ye, J. Cui and X. Q. Zhu,
et al., Effect of calcium on the kinetics of free fatty acid release during in vitro lipid digestion in model emulsions, Food Chem., 2013, 139(1–4), 681–688 CrossRef CAS PubMed.
- K. D. Cashman, Calcium intake, calcium bioavailability and bone health, Br. J. Nutr., 2002, 87, S169–S177 CrossRef CAS PubMed.
- T. Tokle and D. J. McClements, Physicochemical properties of lactoferrin stabilized oil-in-water emulsions: Effects of pH, salt and heating, Food Hydrocolloids, 2011, 25(5), 976–982 CrossRef CAS PubMed.
- N. A. McCarthy, A. L. Kelly, J. A. O'Mahony and M. A. Fenelon, Sensitivity of emulsions stabilised by bovine β-casein and lactoferrin to heat and CaCl2, Food Hydrocolloids, 2014, 35, 420–428 CrossRef CAS PubMed.
- U. Lesmes,
et al., Impact of Surface Deposition of Lactoferrin on Physical and Chemical Stability of Omega-3 Rich Lipid Droplets Stabilized by Caseinate, Food Chem., 2010, 123(1), 99–106 CrossRef CAS PubMed.
- N. E. Simpson, C. L. Stabler and C. P. Simpson,
et al., The role of the CaCl2–guluronic acid interaction on alginate encapsulated beta TC3 cells, Biomaterials, 2004, 25(13), 2603–2610 CrossRef CAS PubMed.
- U. Schlemmer, Studies of the Binding of Copper, Zinc and Calcium to Pectin, Alginate, Carrageenan and Gum Guar in HCO3–CO2 Buffer, Food Chem., 1989, 32(3), 223–234 CrossRef CAS.
- V. L. Campo, D. F. Kawano and D. B. da Silva,
et al., Carrageenans: Biological properties, chemical modifications and structural analysis – A review, Carbohydr. Polym., 2009, 77(2), 167–180 CrossRef CAS PubMed.
- J. Maldonado-Valderrama, N. C. Woodward and A. P. Gunning,
et al., Interfacial characterization of beta-lactoglobulin networks: Displacement by bile salts, Langmuir, 2008, 24(13), 6759–6767 CrossRef CAS PubMed.
- I. Norton, S. Moore and P. Fryer, Understanding food structuring and breakdown: engineering approaches to obesity, Obes. Rev., 2007, 8, 83–88 CrossRef PubMed.
- D. J. McClements, E. A. Decker and Y. Park, Controlling Lipid Bioavailability through Physicochemical and Structural Approaches, Crit. Rev. Food Sci. Nutr., 2009, 49(1), 48–67 CrossRef PubMed.
- B. S. Chu, G. T. Rich and M. J. Ridout,
et al., Modulating Pancreatic Lipase Activity with Galactolipids: Effects of Emulsion Interfacial Composition, Langmuir, 2009, 25(16), 9352–9360 CrossRef CAS PubMed.
|
This journal is © The Royal Society of Chemistry 2014 |
Click here to see how this site uses Cookies. View our privacy policy here.