DOI:
10.1039/C2FO30133D
(Paper)
Food Funct., 2013,
4, 74-82
Omega-3 fatty acids in ileal effluent after consuming different foods containing microencapsulated fish oil powder – an ileostomy study
Received
14th June 2012
, Accepted 2nd September 2012
First published on 4th September 2012
Abstract
The intestinal absorption of omega-3 long chain polyunsaturated fatty acids (ω3 LCPUFA), [eicosapentaenoic acid (EPA) + docosahexaenoic acid (DHA)], after consuming fish oil gelatine capsules or different food products fortified with microencapsulated fish oil, was determined using human ileostomates. The total amount of ω3 LCPUFA consumed per dose of fish oil capsule was 266 mg while that for fortified orange juice, yoghurt and cereal bar was 284 mg per serving of food product. In a time course experiment ω3 LCPUFA was measured in ileal effluent over 24 h post ingestion. Only 0.58–0.73% of the total ω3 LCPUFA dose was recovered in the ileal effluent irrespective of whether the fish oil was delivered in a gelatine capsule or in the form of a microencapsulated powder incorporated into fortified foods. Excretion of ω3 LCPUFA was detected in the 2–18 h effluent collections with none detected at 0 h or 24 h. post ingestion. The transit time of the minimal amount of ω3 LCPUFA that remained in the ileal effluent was dependent on the method of delivery of the fish oil. The ω3 LCPUFA content in the ileal effluent peaked at 2–8 h and declined after 10 h after consumption of fish oil capsules and fortified orange juice. In contrast, two peaks in ω3 LCPUFA content were observed in the ileal effluent, first at 2–8 h and again at 14–16 h, after consumption of fortified yoghurt and cereal bar. The highest recovery of the small amount of ω3 LCPUFA in the ileal effluent at 14–16 h was obtained when fortified cereal bar was consumed. The results suggest that the delivery of fish oil through food products fortified with microencapsulated fish oil does not compromise the bioavailability of the ω3 LCPUFA as evidence by no statistical differences detected in the remaining portion of ω3 LCPUFA in the ileal effluent (p = 0.58). However, the food matrix in which the microencapsulated oil was delivered may alter the transit kinetics of the ω3 LCPUFA through the small intestine.
Introduction
Functional food ingredients have biological benefits beyond normal nutrition and have the potential to improve human health. These ingredients are available as dietary supplements or may be incorporated into foods. The largest market sector for functional ingredients added to food and beverages today is omega-3 long-chain polyunsaturated fatty acids (ω3 LCPUFA), particularly eicosapentaenoic acid (EPA) and docosahexaenoic acid (DHA). The popularity of these ω3 LCPUFA is due to their potential health benefits in infant and child nutrition,1,2 and their effects on reducing the risk of cardiovascular disease,3 inflammation, rheumatoid arthritis, inflammatory bowel diseases4,5 and colon cancer.6 Research into the potential beneficial effects of ω3 LCPUFA in obesity7–9 and ageing10 is also increasing.
Obtaining adequate amounts of ω3 LCPUFA in the diet can be achieved by eating oily fish regularly, taking supplements (fish/algal oil in gelatine capsules) or including functional foods and beverages that are enriched with ω3 LCPUFA. Recommended intake levels for EPA and DHA range from 160–1600 mg per day.11 Consuming functional foods enriched with ω3 LCPUFA boosts the intake of ω3 LCPUFA by consumers who do not like eating fish or swallowing multiple capsules regularly. Traditionally functional food ingredients were added directly into foods. However there are issues with direct addition of some sensitive functional ingredients, such as ω3 LCPUFA as they are prone to oxidation and the development of off-flavours and off-odours, thus compromising the sensory properties of the food and loss of bioactivity.12–17 Today, microencapsulation strategies are often employed to stabilise LCPUFA oils and this has enabled their successful delivery through food.18,19 In encapsulation, the active ingredients (i.e. core) are surrounded by a secondary material (i.e. encapsulant material) which protects the active ingredient from the environment until a trigger stimulates the release of the active ingredient.20,21 It is important to ensure that the LCPUFA oil delivered through foods retain their biological potency until they are released at their site of action within the body to impart their health benefits. This is irrespective of whether the nutrient is retained within the naturally occurring structure in the food, directly incorporated as the neat nutrient into the food formulation during processing or added as microencapsulated ingredients.
There are many microencapsulation strategies that have been developed for stabilising ω3 LCPUFA.22–30 Where microencapsulated ingredients are used, they may be supplied as a stabilised formulation or powder that is blended with a food product just prior to consumption or they may be added during the manufacture of the processed food in the factory.
For the delivery of nutrients through food it is essential that the encapsulating materials used for preparing microencapsulated bioactive food ingredients are food grade components having GRAS (generally regarded as safe) status. Typically emulsion-based formulations are used for the microencapsulation of oils and oil-soluble functional ingredients. A variety of food grade components such as low molecular weight surface-active components (e.g. phospholipids), proteins (e.g. milk and soy proteins), sugars (e.g. glucose, sucrose, lactose), maltodextrins, starch including emulsifying starches, and gums (e.g. gum arabic, chitosan and pectin) have been used for formulating emulsions containing the lipophilic bioactive.27,31,32 The emulsions containing functional oils or oil-soluble ingredients may be spray dried and delivered as powdered ingredients that may be easily incorporated into formulated foods.33,34
Many studies on the digestibility of microencapsulated oil have been carried out in vitro.35–38 These studies have shown that the lipolysis and release of the encapsulated oil is influenced by the encapsulation formulation and the structure of the emulsion.39 The factors influencing the digestion of emulsion-based delivery systems and the cellular uptake of lipophilic components have been reviewed.40 Lipolysis occurs at the interface and lipase has to adsorb at the oil–water interface before it can hydrolyse the triacylglycerols into monoacylglycerols and free fatty acids. The attachment of lipase at the interface may be inhibited by strongly surface active components, such as polar lipids which compete with physiological surfactants which facilitate attachment of pancreatic lipase to the oil–water interface. Hence, the presence of these components in the digestive milieu can influence lipolysis.40
Microencapsulation of ω3 LCPUFA oils within matrices of heated mixtures of protein and carbohydrate has been shown to protect ω3 LCPUFA during processing and storage.41 The in vitro digestibility of the microencapsulated oil capsules30,42,43 and the transit time and kinetics of the uptake of ω3 LCPUFA (EPA and DHA) into the plasma of rats in vivo was influenced by the encapsulant material (heated or unheated protein–carbohydrate mixture) used for stabilisation of the microencapsulated fish oil or fish oil in combination with other bioactives and feeding pattern.44–46 It is also known that the microstructure of foods alters the bioavailability of nutrient lipids.47 Therefore it might be expected that the choice of the food-grade encapsulating materials, the formulation and the processing of the microencapsulated bioactive ingredients may all influence the bioavailability of the encapsulated oil. Further the food vehicle through which the microcapsules are delivered may also modify ω3 LCPUFA bioavailability. We have also recently shown that the in vitro digestibility of microencapsulated oil powder, stabilised by a heated mixture of sodium caseinate, glucose and a physically modified resistant starch, depended on the food vehicle used to carry the microcapsule.36
The aim of the present study was to examine the effect of the food matrix (used as food vehicle) on the release of ω3 LCPUFA from the microcapsules, during passage through the human small intestinal tract. The food matrices selected to deliver the microencapsulated fish oil powder were orange juice, yoghurt and a cereal bar. These were chosen because they represent liquid, semi-solid and solid food matrices respectively. The small intestinal transit in humans of ω3 LCPUFA delivered as foods fortified with microencapsulated fish oil powder was compared to that for the same oil delivered in gelatine capsules in volunteers who have previously had an ileostomy procedure, but are otherwise healthy. The use of ileostomates in a clinical trial provides a unique opportunity to measure the amount of ω3 LCPUFA remaining in the ileal effluent collected from ileostomy bags over time after ingestion. To our knowledge, no other studies had been done using ileostomates to examine the digestibility and transit of microencapsulated ω3 LCPUFA. The information obtained from this study is valuable for someone considering the choice of food matrix that will protect and deliver the microencapsulated omega-3 ingredients to the body, aimed at delivering its benefits to the consumers.
Materials and methods
Materials
Tuna oil soft gelatine capsules (fish oil capsule) were used as the control in this study and were provided by Nu-Mega Ingredients Pty Ltd, Australia. Microencapsulated fish oil powder [25% w/w Hi-DHA tuna oil (Nu-Mega Ingredients Pty Ltd., Australia w/w oil)] was prepared in the pilot plant of CSIRO Food and Nutritional Sciences as previously described.36 The matrix used for stabilising the oil-in-water emulsion was a heated protein–glucose–pre-processed starch mixture comprised of equal proportions of sodium caseinate (Myopure, Petersham, NSW), dextrose monohydrate (Penford, Australia) and Hylon VII (National Starch Food Innovation, Australia). The pre-processed starch was prepared by physical modification of the Hylon VII by heat and microfluidisation before combining with the other encapsulant materials. The tuna oil was added into the aqueous encapsulant matrix and homogenised at 35 MPa (1st stage) and 10 MPa (2nd stage). The homogenised emulsion was then spray dried into powder.36
The food vehicles used to deliver the microencapsulated oil powder were (i) orange juice (Just Juice™, National Foods, Australia), (ii) peach-mango flavoured diet yoghurt (Nestle™, Australia) and (iii) a cereal bar prepared from ingredients obtained from a local supermarket as previously described.36 The microencapsulated oil powder was mixed with the orange juice (4 g powder per 200 ml serve) and yoghurt (4 g powder per 200 ml serve) just prior to consumption whereas in the case of the cereal bar (4 g powder per 60 g serve), the microencapsulated powder was mixed with other ingredients during preparation.36 This represents the inclusion of 1000 mg tuna oil or 284 mg ω3 LCPUFA (50 mg EPA and 234 mg DHA) per serve of food.
Study volunteers
Human volunteers (n = 6) were recruited to this study. Inclusion and exclusion criteria were as follows: volunteers must (i) have had minimal terminal ileum removed and have a conventional and well-functioning permanent ileostomy; (ii) not be receiving any medication that could interfere with the study and no medications likely to modulate small intestinal function; (iii) not participate in a study with any experimental drug within 30 days of commencement of the study; (iv) absence of gastrointestinal, renal, hepatic disease or intestinal inflammation; and (v) age 20–82 years and willing to comply with alcohol and study diet restrictions. Before the study commenced, volunteers either visited CSIRO Animal, Food and Health Sciences, or were visited at home by project staff, where they were given detailed instructions about the study. Volunteers carried out each study in their own homes and the test foods were supplied on the morning of the test. The study protocols were approved by CSIRO's Human Research Ethics Committee.
Study design and diet
The study was conducted over two consecutive weeks. There was a control day (i.e. no sample consumed) included in each week of the study. Six volunteers completed the study. The volunteers were on habitual diets except that foods or supplements known to contain omega-3 oils were not permitted. The amount of ω3 LCPUFA per dose was based on a recommendation of 250 mg of ω3 LCPUFA per day to meet long chain omega-3 requirement.48 ω3 LCPUFA oils were delivered orally in three food delivery formats fortified with 1000 mg fish oil containing 284 mg ω3 LCPUFA (50 mg EPA and 234 mg DHA) per serving. The food serving consisted of 200 ml orange juice, 200 ml yoghurt or 60 g cereal bar. ω3 LCPUFA oils were also delivered in gelatine capsules at a dose of 1× 1000 mg fish oil capsule containing 266 mg ω3 LCPUFA (48 mg EPA and 218 mg DHA) taken with water.
The study was a randomised cross-over design and the treatments were administered in random order. The volunteers were given the four different samples and were instructed to consume one, on each of the four separate days. These days were arranged at the convenience of the participants. Following overnight fasting, the volunteers consumed one of the test samples over a maximum period of 20 min. After a further 60 min, the volunteers were allowed to resume their normal diet while avoiding a list of foods which contains omega-3. The volunteers were also asked to fill in a record of food consumed during the trial. The ileal effluent were collected by the volunteers at t = 0, 2, 4, 6, 8, 10, 12, 14, 16, 24 h. The samples were collected at these time points to capture the amount of undigested or unabsorbed ω3 LCPUFA soon after they exit the ileum, and to minimise possible degradation of ω3 LCPUFA due to prolonged exposure to bacterial action in the ileostomy bag. The volunteers changed their ileostomy bag at each time point and placed them on ice, and connected a new bag. Samples were transported to the laboratory immediately following collection. On arrival at the laboratory, the wet weight of each sample was recorded, then homogenised using a Stomacher homogeniser for 60 s. The homogenised samples were then divided into a number of smaller sub-samples and stored at-20 °C until ready for analysis.
Analysis of ileal effluent
Verification of oil extraction method.
To verify the recovery of oil in the effluent, the ileal effluent was spiked with bulk tuna oil and microencapsulated tuna oil powder at three levels (0.11, 0.34 and 1.02 mg tuna oil per g effluent; 0.45, 1.36 and 4.09 mg powder per g effluent). The lowest concentration used for spiking (0.11 mg oil per g effluent) corresponded to 10% of dose while the highest concentration (1.02 mg tuna oil per g effluent) corresponded to 100% of dose delivered.
Extraction of oil from ileal effluent.
One gram (±0.00001 g) of ileal effluent was weighed into a screw-capped 15 ml culture tube. Then, 1.5 ml water and 4 ml isopropanol were added and the tube capped prior to mixing at 60 rpm for 2 min using a suspension mixer (Ratex Instruments Pty ltd, Boronia, Victoria, Australia). A further 4 ml of hexane was added into the sample mixture and vortexing was carried out. The mixed solution was centrifuged at 2000 rpm for 15 min and the top (hexane) layer was withdrawn. Another 5 ml of hexane was added to the residue and the mixture vortexed before centrifugation and collection of the hexane layer. The solvent extracts were pooled and evaporated to dryness over nitrogen. Triplicate extractions of oil from each ileal effluent sample were carried out.
Preparation of methyl esters
Two ml of internal standard solutions (methyl tricosanoate in isooctane at levels of 0.014 and 0.14 mg ml−1) were added to the dried oil extract from the ileal effluent, followed by addition of 2 ml tetrahydrofuran (THF) and 2 ml 4.5% (v/v) H2SO4 in methanol and mixed well. The mixture was purged with nitrogen before the tube was capped and placed in a water bath (Julabo, SW23, John Morris Scientific, NSW, Australia) maintained at 50 °C and shaken at 100 rpm at overnight. Three ml of 5% (w/v) sodium chloride in water was added and the tube was shaken vigorously for 30 s. The upper organic phase was withdrawn and washed with 4 ml of aqueous potassium bicarbonate (2%, w/v) and dried over anhydrous sodium sulphate. The fatty acid methyl ester (FAME) solution was analysed by GC without further concentration as previously described by Shen et al.49
ω3 LCPUFA analysis of samples from ileal effluent
Initially the amount of ω3 LCPUFA (DHA and EPA) were analysed from each sample collected at every time points from two volunteers. As DHA and EPA were not detected at t = 0 and 24 h, further analysis was carried out on samples collected between 2 h and 16 h. To reduce the number of samples to be analysed, the individual samples from 2 h up to 16 h were pooled as follows into (2 + 4) h, (6 + 8) h, (10 + 12) h and (14 + 16) h sample collection for further analysis.
The total amount of ω3 LCPUFA (DHA and EPA) from the ileal effluents were analysed to determine the percentage of the added omega-3 oil that was protected from small intestinal digestion in order to provide an indication of how much ω3 LCPUFA was released, digested and absorbed in the upper GI tract.
Statistics
Power calculations suggested that 6 volunteers were more than adequate to provide an 80% chance of detecting a 20-fold difference in the degree of ω3 LCPUFA levels between treatments at an alpha of 0.05. Data were analysed using R version 2.14. A generalised linear model was used to determine whether there were significant differences between delivery formats, volunteers and time points in the remaining portion of total ω3 LCPUFA. This analysis is equivalent to an unbalanced ANOVA. It was unbalanced because there was a missing datum. A linear mixed model treating volunteers as a random effect was used to more accurately assess significant differences between delivery formats in the total remaining LCPUFA.
Box plots were plotted to summarise the data, showing five-number summaries: the smallest observation (sample minimum), lower quartile (Q1), median (Q2), upper quartile (Q3), and the highest observation still within 1.5 of the inter quartile range of the upper quartile (Q3–Q2). Outliers outside this range are plotted as circles.
Results
Fatty acid composition of feed material
The commercial fish oil capsules each contained 48 mg EPA and 218 mg DHA per gram oil (i.e. ratio of DHA
:
EPA = 4.54). The fish oil that was used for the manufacture of microencapsulated powder contained 50 mg EPA and 234 mg DHA per gram oil (i.e. ratio of DHA
:
EPA = 4.68). Previous experiments in our laboratory had shown that EPA and DHA are not oxidised during the manufacture of the microencapsulated oil powders (data not shown). The small difference in EPA and DHA between the capsule and the fish oil is typical batch to batch variation in the fish oil.
Extraction efficiency of EPA and DHA from spiked ileal effluent
ω3 LCPUFA recoveries from spiked ileal effluent samples varied from 71 to 98% and were dependent on the amount of oil spiked, the form in which the oil was presented (i.e. free oil or microencapsulated powder) and the particular fatty acid being recovered (Table 1). Generally better recoveries were obtained at higher spiking levels. Recovery of DHA was higher than for EPA, and more oil was recovered when spiking was with neat oil as compared to the microencapsulated oil powder. The ratio of recovered DHA
:
EPA varied with the level of spiking (Table 1), ranging from 3.4–5.1 compared with 4.68 for the original oil. Incomplete extraction, differential extraction efficiencies and/or differential oxidation of ω3 LCPUFA during the extraction procedure may have accounted for these differences. The results from the lowest spiking level were used as the correction factor in the calculation of ω3 LCPUFA recoveries from ileal effluents in the clinical study.
Table 1 ω3 LCPUFA recovery from blank ileal effluent spiked with tuna oil or microencapsulated tuna oil powder
Amount oil added to effluent (mg g−1 effluent) |
Format of ω3 LCPUFA delivery |
EPA recovery (%) |
DHA recovery (%) |
Ratio DHA : EPAa |
EPA and DHA concentrations in the fresh tuna oil were 50 and 234 mg g−1 tuna oil (i.e. ratio DHA : EPA = 4.68).
|
0.11 |
Neat oil |
81 |
73 |
3.4 |
Microencapsulated powder |
72 |
77 |
3.9 |
0.34 |
Neat oil |
71 |
77 |
4.6 |
Microencapsulated powder |
77 |
77 |
4.3 |
1.02 |
Neat oil |
89 |
98 |
5.1 |
Microencapsulated powder |
78 |
85 |
5.1 |
ω3 LCPUFA content in ileal effluent collected from volunteers
The total amounts of ω3 LCPUFA was 284 mg (50 mg EPA and 234 mg DHA) per dose when delivered as a microencapsulated powder in orange juice, yogurt or a cereal bar, and 266 mg (48 mg EPA and 218 mg DHA) when delivered as fish oil in a gelatine capsule. ω3 LCPUFA were not detected in the ileal effluent at 0 or 24 h. Only 0.58–0.73% of the total ω3 LCPUFA administered was recovered in the ileal effluent between 2–16 h showing that there was efficient digestion and absorption in the small intestine when fish oil was delivered as gelatine capsules or in different foods fortified with microencapsulated fish oil (Table 2). Also DHA was absorbed more than EPA in the upper gastro intestinal (GI) tract with only 0.54–0.63% (DHA) compared to 0.79–1.20% (EPA) of the administered dose exiting the ileum.
Table 2 Total amount (μg) and % of dose administrated of ω3 LCPUFA recovered from ileal effluent collected from 4 different delivery formats over 18 h (average of triplicate extraction ± STDEV of 6 volunteers). The total ω3 LCPUFA delivered were 50 mg EPA and 234 mg DHA in orange juice, yogurt and cereal bar and 48 mg EPA and 218 mg DHA in fish oil capsule. Correction factors for EPA and DHA from spiking analysis (at the lowest spiking level) were applied to the calculation
|
EPA content (μg) in ileal effluent |
EPA % of dose administered |
DHA content (μg) in ileal effluent |
DHA % of dose administered |
(EPA + DHA) content (μg) in ileal effluent |
(EPA + DHA) % of dose administered |
Fish oil capsule |
448 ± 167 |
0.93 |
1218 ± 717 |
0.56 |
1665 |
0.63 |
Orange juice |
598 ± 337 |
1.20 |
1283 ± 865 |
0.55 |
1881 |
0.66 |
Yogurt |
394 ± 251 |
0.79 |
1258 ± 1136 |
0.54 |
1652 |
0.58 |
Cereal bar |
585 ± 193 |
1.17 |
1481 ± 860 |
0.63 |
2067 |
0.73 |
The total of ω3 LCPUFA recovered in the ileal effluent (as % of dose delivered) from each volunteer between 2 and 16 h are given in Fig. 1. The results show a large variation in the total amounts recovered between volunteers for each of the four types of delivery vehicles. Volunteer 5 and 2 had higher amounts (p < 0.001) of ω3 LCPUFA recovered from the ileal effluents while volunteers 1, 4 and 6 had much lower amounts (Fig. 1).
![Amount of ω3 LCPUFA recovered from ileal effluent (as % of dose delivered) collected from each of the volunteer [1 (white), 2 (yellow), 3 (light blue), 4 (grey), 5 (red), 6 (dark blue)] for each of the 4 types of delivery formats (fish oil capsule; orange juice; yoghurt; cereal bar). Recovery amount from each volunteer at every time point was calculated as: ω3 LCPUFA per gram effluent × total collected effluent (g) at the time point. Correction factors for EPA and DHA from spiking analysis (at the lowest spiking level) were applied to the calculation.](/image/article/2013/FO/c2fo30133d/c2fo30133d-f1.gif) |
| Fig. 1 Amount of ω3 LCPUFA recovered from ileal effluent (as % of dose delivered) collected from each of the volunteer [1 (white), 2 (yellow), 3 (light blue), 4 (grey), 5 (red), 6 (dark blue)] for each of the 4 types of delivery formats (fish oil capsule; orange juice; yoghurt; cereal bar). Recovery amount from each volunteer at every time point was calculated as: ω3 LCPUFA per gram effluent × total collected effluent (g) at the time point. Correction factors for EPA and DHA from spiking analysis (at the lowest spiking level) were applied to the calculation. | |
The amounts of ω3 LCPUFA recovered from the ileal effluents (as % of dose delivered) at the four different time points (2 + 4 h, 6 + 8 h, 10 + 12 h, 14 + 16 h) after administration of microencapsulated oil through different food matrices are shown in Fig. 2. The amount of unabsorbed ω3 LCPUFA recovered from the effluent at 2 + 4 h was highest with orange juice, while the highest amount of ω3 LCPUFA recovered at 14 + 16 h was with cereal bar (Fig. 2).
![Amount of ω3 LCPUFA recovered from ileal effluent (as % of dose delivered) collected at the 4 different time points [2 + 4 h (white), 6 + 8 h (yellow), 10 + 12 h (grey), 14 + 16 h (blue)] from the 4 types of delivery formats. Recovery amount from each volunteer at every time point was calculated as: LCPUFA of per gram effluent × total collected effluent (g) at the time point. Correction factors for EPA and DHA from spiking analysis (at the lowest spiking level) were applied to the calculation.](/image/article/2013/FO/c2fo30133d/c2fo30133d-f2.gif) |
| Fig. 2 Amount of ω3 LCPUFA recovered from ileal effluent (as % of dose delivered) collected at the 4 different time points [2 + 4 h (white), 6 + 8 h (yellow), 10 + 12 h (grey), 14 + 16 h (blue)] from the 4 types of delivery formats. Recovery amount from each volunteer at every time point was calculated as: LCPUFA of per gram effluent × total collected effluent (g) at the time point. Correction factors for EPA and DHA from spiking analysis (at the lowest spiking level) were applied to the calculation. | |
The time for the small amount of unabsorbed ω3 LCPUFA to appear in the ileal effluent was dependent on the delivery vehicle in which the fish oil was delivered. When fish oil was consumed in the form of gelatine capsules or delivered as microencapsulated powder in yoghurt, the ω3 LCPUFA recovered in the effluent first peaked at 6 + 8 h and declined at 10 + 12 h, except that with the yoghurt a slight increase appeared at 14 + 16 h as second peak (Fig. 2). When fish oil was delivered as microencapsulated powder added into orange juice the amount of ω3 LCPUFA recovered from the effluents peaked at 2 + 4 h and declined at 6 + 8 h which further declined at 12 + 14 h, then increased at 14 + 16 h as a second peak (Fig. 2). When fish oil was delivered as microencapsulated powder added into cereal bar, a very low amount of ω3 LCPUFA was detected between 2–12 h with a significant increase in amount recovered at 14 + 16 h, being the highest amount recovered in any time point from all the different food vehicles.
The amounts of individual fatty acids EPA and DHA recovered from the ileal effluent collected at 4 different time points of each of the different delivery formats is shown in Fig. 3. The EPA and DHA amounts recovered were generally statistically significantly lower at 10 + 12 h compared to other time points (p < 0.05) for all delivery formats. Recovery of EPA from ileal effluent for capsule and yoghurt is low at all time points, but slightly higher in orange juice at 2–8 h and in cereal bar at 14 + 16 h. The recovery of DHA from ileal effluent was slightly higher between 2–8 h for all the delivery formats. For both EPA and DHA recovery, a second peak is observed at 14 + 16 h for yoghurt and cereal bar (Fig. 3) suggesting that these food matrices may have slower transit through the GI tract compared to orange juice and capsules.
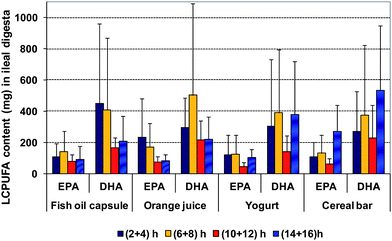 |
| Fig. 3 Amount (μg) of EPA and DHA recovered from ileal effluent collected at the 4 different time points from the 4 types of delivery formats (average of triplicate extraction ± STDEV of 6 volunteers). Recovery amount from each volunteer at every time point was calculated as: ω3 LCPUFA of per gram effluent × total collected effluent (g) at the time point. Correction factors for EPA and DHA from spiking analysis (at the lowest spiking level) were applied to the calculation. | |
No statistical significant differences were observed between delivery formats with respect to the percentage remaining ω3 LCPUFA (p = 0.36).
Discussion
The results clearly demonstrated that >98% of the ω3 LCPUFA administered was absorbed by the human small intestine during transit. This suggests that, at the level of fish oil used in this study, digestion and absorption of ω3 LCPUFA through the GI tract was similar and almost complete 16 h after consumption, irrespective of whether the fish oil was delivered in gelatine capsules or through foods fortified with microencapsulated fish oil powder. The time for the ω3 LCPUFA to appear in the effluent appears to have some relationship with the total solids of the food delivery vehicle, with the highest amount detected at 2–4 h in orange juice (lowest total solids) and the highest amount detected at 14–16 h was in cereal bar (highest total solids). The early appearance of ω3 LCPUFA in the effluent indicates fast transit through the GI tract, while late appearance indicates slower transit through the GI tract. These results suggest that the transit time and release of ω3 LCPUFA for absorption in the GI tract are influenced by the total solids content in the food matrix used as delivery vehicle.
This study also suggested that a much higher level of lipid digestion and absorption occurs in humans (>98%) than is observed using in vitro models of digestion (73.2–78.6% released) involving sequential exposure to simulated gastric and intestinal fluids of orange juice, yoghurt and cereal bar fortified with microencapsulated fish oil powders.36 A similar comparison between in vitro digestibility and in vivo studies, albeit in different encapsulation and feeding systems, suggested that while coating of emulsified lipids with chitosan inhibited lipolysis in vitro,50 chitosan did not inhibit lipolysis in vivo in mice.51 While in vitro models for examining digestibility of emulsion-based delivery systems are useful to the extent that they provide a rapid and cost effective screening method for comparing the digestibility and bioaccessibility of lipids within different microencapsulated formulations, the differences observed between in vitro and in vivo trials demonstrate the importance of complementing in vitro assessments of lead microencapsulated formulations with feeding studies in vivo.
Our present results in human ileostomates confirm that delivery of microencapsulated fish oil powder (where the oil is encapsulated by a heated caseinate–glucose–processed resistant starch encapsulant) within the various food matrices examined, do not compromise the bioavailability of the fish oil compared to capsular gelatine formats. Our results also corroborate previous studies in healthy human volunteers which show that oil delivered as microencapsulated powder formulations are bioavailable and have similar bioavailability as that delivered as supplements as judged usually by ω3 LCPUFA levels in the plasma. For example, consumption of breads containing microencapsulated tuna oil52 (10% protein and 54% carbohydrate) raised the ω3 LCPUFA levels in the plasma of human subjects.53 Similarly consumption of a luncheon meat formulation enriched with microencapsulated fish oil (formulation details not provided) significantly raised EPA and DHA levels in plasma in humans.54 The equivalent bioavailability of microencapsulated oils delivered within food matrices was also confirmed by the following studies. There were no significant differences in plasma ω3 LCPUFA levels in human subjects when ω3 LCPUFA was delivered as a fish oil capsule or as microencapsulated oil formulation in a milkshake.55 Studies in healthy human subjects have also demonstrated the bioequivalence of ethyl esters of EPA and DHA delivered in soft gel capsules and milk shake containing a microencapsulated oil powder stabilised by a complex coacervate of gelatine and polyphosphate cross-linked with transglutaminase enzyme.56 In their study, bioavailability was demonstrated by the significant increases in the serum phospholipid levels of EPA and DHA.56 While the food matrix did not significantly influence the bioavailability of the ω3 LCPUFA, Schram et al.57 reported that fish oil directly incorporated into food products (e.g. yoghurt and health bar) were absorbed differently from those simply administered as supplements (capsules) over 6 h in a single meal study in young healthy male subjects. They found that yoghurt was the best matrix providing fast lipid absorption compared to a health bar and a fish oil capsule in young males.57 However, fortification of snack bars with algal oil found that the ω3 LCPUFA delivery through this food vehicle resulted in the same bioavailability as that delivered in gelatine capsules.58 While these published studies suggest a bioequivalence of ω3 LCPUFA presented in gelatine capsules or as a microencapsulated formulation in food, it is difficult to estimate the proportion of the administered dose that has been digested and absorbed through the GI tract using this approach. This first ever study with human ileostomates provides this important complementary information. Here we show that in excess of 98% of administered ω3 LCPUFA is digested and absorbed in the small intestine, regardless of whether the fish oil is delivered in a gelatine capsule or a microcapsule formulation and in a fashion that is largely independent of the food matrix.
Whilst it is generally accepted that native food structure may have an impact on the bioavailability of some nutrients as they occur in nature or in formulated foods,59 it appears that humans are very efficient at extracting lipids from meals. The evidence to date suggests that the mode of delivery of fish oil through food in a capsule or a microencapsulated fish oil powder stabilised in a complex coacervate of protein and polyphosphate cross-linked by transglutaminase56 or in a heated protein–carbohydrate matrix (this work) does not have a significant effect on the bioavailability of the ω3 LCPUFA. This suggests that surface active components in emulsions, containing a protein–polyphosphate complex coacervate or a heated protein–carbohydrate mixture that are at the interface of a microencapsulated oil droplet can be digested or displaced by bile salts, enabling the attachment of pancreatic lipase to the interface and subsequent lipolysis. The fact that only 0.59–0.73% of the total EPA and DHA consumed was present in the ileal effluent (in this study) suggests that once lipolysis occurs, trans-mucosal uptake of the products of lipolysis is rapid and efficient.
Conclusion
This study showed that delivery of omega-3 oils (microencapsulated in heated protein–carbohydrate matrices) through different food products is equally bioavailable as capsular delivery. The results also suggest that the rate of digestion and release of ω3 LCPUFA through the GI tract could be manipulated by selecting the structure of the food matrix. Further clinical studies in healthy subjects are recommended to confirm bioavailability, absorption and accumulation of ω3 LCPUFA in the blood between a capsule and food as delivery vehicle for microencapsulated omega-3 powder.
Acknowledgements
The authors gratefully acknowledge the contributions made by Dr Tony Bird (overall management of the study), Jenny McInerney (organisation and running of the study with the volunteers), and to Caroline Cooke, Michael Mullar and Sharyn Zrna for their assistance in running the ileostomy study. Technical assistance from Li Jiang Cheng and Wayne Beattie in the production and physical characterisation of microcapsules is also acknowledged.
References
- E. E. Birch, S. E. Carlson, D. R. Hoffman, K. M. Fitzgerald-Gustafson, V. L. Fu, J. R. Drover, Y. S. Castaneda, L. Minns, D. K. Wheaton, D. Mundy, L. Marunycz and D. A. Ersen-Schade, The DIAMOND (DHA Intake AND Measurement of Neural Development) study: a double-masked, randomised controlled clinical trial of the maturation of infant visual acuity as a function of the dietary level of docosahexaenoic acid, Am. J. Clin. Nutr., 2010, 91, 848–859 CrossRef CAS.
- S. M. Madden, C. F. Garrioch and B. J. Holub, Direct diet quantification indicates low intakes of (n-3) fatty acids in children 4 to 8 years old, J. Nutr., 2009, 139, 528–532 CrossRef CAS.
- A. Leaf, Y. F. Xiao, J. X. Kang and G. E. Billman, Prevention of sudden cardiac death by n-3 polyunsaturated fatty acids, Pharmacol. Ther., 2003, 98, 355–377 CrossRef CAS.
- P. C. Calder, Inflammatory processes and rheumatoid arthritis, Proc. Nutr. Soc., 2008, 676, 409–418 CrossRef.
- P. C. Calder, Polyunsaturated fatty acids, inflammatory processes and inflammatory bowel diseases, Mol. Nutr. Food Res., 2008, 52, 885–897 CAS.
- C. E. Roynette, P. C. Calder, Y. M. Dupertuis and C. Pichard, N-3 polyunsaturated fatty acids and colon cancer prevention, Clin. Nutr., 2004, 23, 139–151 CrossRef CAS.
- J. P. Despres, A. Golay and L. Sjostrom, Effects of rimonabat on metabolic risk factors in overweight patients with dyslipidemia, N. Engl. J. Med., 2005, 353, 2121–2134 CrossRef CAS.
- I. Thorsdottir, H. Tomasson, I. Gunnarsdottir, E. Gisladottir, M. Kiely, M. D. Parra, N. M. Bandarra, G. Schaafsma and J. A. Martinez, Randomized trial of weight-loss-diets for young adults varying in fish and fish oil content, Int. J. Obes., 2007, 31, 1560–1566 CrossRef CAS.
- D. Parra, A. Ramel, N. Bandarra, M. Kiely, J. A. Martinez and I. Thorsdottir, A diet rich in long chain omega-3 fatty acids modulates satiety in overweight and obese volunteers during weight loss, Appetite, 2008, 51, 676–680 CrossRef CAS.
- R. Farzaneh-Far, J. Lin, E. Epel, K. Lapham, E. Blackburn and M. A. Whooley, Telomere length trajectory and its determinants in persons with coronary artery disease: longitudinal findings from the heart and soul study, PLoS One, 2010, 5(1), e8612, DOI:10.1371/journal.pone.0008612.
- B. J. Meyer, N. J. Mann, J. L. Lewis, G. C. Milligan, A. J. Sinclair and P. R. C. Howe, Dietary intakes and food sources of omega-6 and omega-3 polyunsaturated fatty acids, Lipids, 2003, 38, 391–398 CrossRef CAS.
-
L. Sanguansri and M. A. Augustin, Microencapsulation in Functional Food Product Development, in Functional Food Product Development, ed. J. Smith and E. Charter, Wiley Blackwell Publishing Ltd., 2010, pp. 3–23 Search PubMed.
- A. Ye, J. Cui, A. Taneja, X. Zhu and H. Singh, Evaluation of processed cheese fortified with fish oil emulsion, Food Res. Int., 2009, 42, 1093–1098 CrossRef CAS.
- M. A. Augustin and L. Sanguansri, Polyunsaturated fatty acids: delivery, innovation and incorporation into foods, Food Aust., 2003, 55(7), 294–296 CAS.
- M. B. Let, C. Jacobsen, E. N. Frankel and A. S. Meyer, Oxidative flavour deterioration of fish oil enriched milk. Effects of type of oil and calcium disodium ethylenediaminetetraacetate (EDTA), Eur. J. Lipid Sci. Technol., 2003, 105, 518–528 CrossRef CAS.
- A. Kamal-Eldin and N. V. Yanishlieva, N-3 fatty acids for human nutrition: stability considerations, Eur. J. Lipid Sci. Technol., 2002, 104, 825–836 CrossRef CAS.
- F. Shahidi and Y. Zhong, Lipid oxidation and improving the oxidative stability, Chem. Soc. Rev., 2010, 39, 4067–4079 RSC.
-
L. Sanguansri and M. A. Augustin. Microencapsulation and Delivery of Omega-3 Fatty Acids, in Functional Food Engineering Technologies and Processing, ed. J. Shi, CRC Press, Taylor & Francis Group, 2006, pp. 297–327 Search PubMed.
- C. J. Barrow, C. Nolan and Y. Jin, Stabilization of highly unsaturated fatty acids and delivery into foods, Lipid Technol., 2007, 19(5), 108–111 CrossRef CAS.
- P. de Vos, M. M. Faas, M. Spasojevic and J. Sikkema, Encapsulation for preservation of functionality and targeted delivery of bioactive food components, Int. Dairy J., 2010, 20(4), 292–302 CrossRef CAS.
- M. A. Augustin and Y. Hemar, Nano- and micro-structured assemblies for encapsulation of food ingredients, Chem. Soc. Rev., 2009, 38, 902–912 RSC.
-
M. A. Augustin and L. Sanguansri, Use of Encapsulation to Inhibit Lipid Oxidation, in Oxidation in Foods and Beverages and Antioxidant Applications, ed. E. Decker, R. Elias and D. J. McClements, Woodhead Publishing Ltd., 2010, pp. 479–495 Search PubMed.
- S. Drusch and S. Mannino, Patent-based review on industrial approaches for the microencapsulation of oils rich in polyunsaturated fatty acids, Trends Food Sci. Technol., 2009, 20(6–7), 237–244 CrossRef CAS.
- Y. Serfert, S. Drusch and K. Schwarz, Chemical stabilisation of oils rich in long-chain polyunsaturated fatty acids during homogenisation, microencapsulation and storage, Food Chem., 2009, 113, 1106–1112 CrossRef CAS.
-
M. Tanouchi, K. Takahashi, K. Fukunaga and K. Murakawa, Functional material and functional food comprising useful phospholipid composition, WO 2007/046386A1, 2007.
- L. A. Shaw, D. J. McClements and E. A. Decker, Spray dried multilayered emulsions as a delivery method for w-3 fatty acids into food systems, J. Agric. Food Chem., 2007, 55, 3112–3119 CrossRef CAS.
- D. J. McClements, E. A. Decker and J. Weiss, Emulsion based delivery systems for lipophilic bioactive components, J. Food Sci., 2007, 72, R109–R124 CrossRef CAS.
- U. Klinkersorn, P. Sophanodora, P. Chinachoti, E. A. Decker and D. J. McClements, Encapsulation of emulsified tuna oil in two-layered interfacial membranes prepared using electrostatic layer-by-layer deposition, Food Hydrocolloids, 2005, 19, 1044–1053 CrossRef.
- K. G. Wu, X. H. Chai and Y. Chen, Microencapsulation of fish oil by simple coacervation of hydroxypropyl methylcellulose, Chin. J. Chem., 2005, 23, 1569–1572 CrossRef CAS.
-
L. Sanguansri and M. A. Augustin, Encapsulation of food ingredients, WO 01/74175A1, 2001.
- A. F. Horn, N. S. Nielsen, U. Andersen, L. H. Søgaard, A. Horsewell and C. Jacobsen, Oxidative stability of 70% fish oil-in-water emulsions: impact of emulsifiers and pH, Eur. J. Lipid Sci. Technol., 2011, 113, 1243–1257 CrossRef CAS.
-
E. Shimoni. Starch as an Encapsulation Material to Control Digestion Rate in the Delivery of Active Food Components, in Delivery and Controlled Release of Bioactives in Foods and Nutraceuticals, ed. N. Garti, Woodhead, Cambridge, UK, 2008, pp. 279–293 Search PubMed.
- J. L. Chavez-Servin, A. I. Castellote and M. C. Lopez-Sabater, Evolution of available lysine and lactose contents in supplemented microencapsulated fish oil infant formula powder during storage, Int. J. Food Sci. Technol., 2008, 43, 1121–1128 CrossRef CAS.
- W. Kolanowski, D. Jaworska, G. Laufenberg and J. Weiβbrodt, Evaluation of sensory quality of instant foods fortified with omega-3 PUFA by addition of fish oil powder, Eur. Food Res. Technol., 2007, 225(5–6), 715–721 CrossRef CAS.
- U. Lesmes and D. J. McClements, Controlling lipid digestibility: response of lipid droplets coated by B-lactoglobulin-dextran Maillard conjugates to simulated gastrointestinal conditions, Food Hydrocolloids, 2012, 26(1), 221–230 CrossRef CAS.
- Z. Shen, C. Apriani, R. Weerakkody, L. Sanguansri and M. A. Augustin, Food matrix effects on in vitro digestion of microencapsulated tuna oil powder, J. Agric. Food Chem., 2011, 59, 8442–8449 CrossRef CAS.
- S. L. Kosaraju, R. Weerakkody and M. A. Augustin,
In vitro evaluation of hydrocolloid-based encapsulated fish oil, Food Hydrocolloids, 2009, 23(5), 1413–1419 CrossRef CAS.
- S. Mun, E. A. Decker and D. J. McClements, Influence of emulsifier type on in vitro digestibility of lipid droplets by pancreatic lipase, Food Res. Int., 2007, 40(6), 770–781 CrossRef CAS.
- D. J. McClements and Y. Li, Structured emulsion-based delivery systems: controlling the digestion and release of lipophilic food components, Adv. Colloid Interface Sci., 2010, 159(2), 213–228 CrossRef CAS.
- P. J. Wilde and B. S. Chu, Interfacial & colloidal aspects of lipid digestion, Adv. Colloid Interface Sci., 2011, 165(1), 14–22 CrossRef CAS.
- M. A. Augustin, L. Sanguansri and O. Bode, Maillard reaction products as encapsulants for fish oil powders, J. Food Sci., 2006, 71(2), E25–E32 CrossRef CAS.
- C. Chung, L. Sanguansri and M. A. Augustin,
In vitro lipolysis of fish oil microcapsules containing protein and resistant starch, Food Chem., 2011, 124, 1480–1489 CrossRef CAS.
- M. I. Burgar, P. Hoobin, R. Weerakkody, L. Sanguansri and M. A. Augustin, NMR of microencapsulated fish oil samples during in vitro digestion, Food Biophys., 2009, 4, 32–41 CrossRef.
- G. S. Patten, M. A. Augustin, M. A. Sanguansri, R. J. Head and M. Y. Abeywardena, Site specific delivery of microencapsulated fish oil to the gastrointestinal tract of the rat, Dig. Dis. Sci., 2009, 54, 511–521 CrossRef CAS.
- M. A. Augustin, M. Y. Abeywardena, G. Patten, R. Head, T. Lockett, A. DeLuca and L. Sanguansri, Effects of microencapsulation on the gastrointestinal transit and tissue distribution of a bioactive mixture of fish oil, tributyrin and resveratrol, J. Funct. Foods, 2011, 3, 25–37 CrossRef CAS.
- M. A. Augustin, G. Patten, A. De Luca, M. Y. Abeywardena, T. Lockett, R. Head and L. Sanguansri, Feeding and format of oil delivery affects intestinal passage of fish oil in rats, Food Funct., 2011, 2, 684–696 CAS.
- H. Sing, A. Ye and D. Horne, Structuring food emulsions in the gastrointestinal tract to modify lipid digestion, Prog. Lipid Res., 2009, 48(2), 92–100 CrossRef.
- Report of the dietary guidelines advisory committee on the dietary guidelines for Americas, 2010, Part D, Section 3, http://www.cnpp.usda.gov/DGAs2010-DGACReport.htm.
- Z. Shen, M. A. Augustin, L. Sanguansri and L. J. Cheng, Oxidative stability of microencapsulated fish oil powders stabilized by blends of chitosan, modified starch and glucose, J. Agric. Food Chem., 2010, 58, 4487–4493 CrossRef CAS.
- S. Mun, E. A. Decker and D. J. McClements, Effect of molecular weight and degree of deacetylation of chitosan on the formation of oil-in-water emulsions stabilised by surfactant-chitosan membranes, J. Colloid Interface Sci., 2006, 296(2), 581–590 CrossRef CAS.
- G. Y. Park, S. Mun, Y. Park, S. Rhee, E. A. Decker, J. Weiss, D. J. McClements and Y. Park, Influence of encapsulation of emulsified lipids with chitosan on their in vivo digestibility, Food Chem., 2007, 104(2), 761–767 CrossRef CAS.
- Driphorm Powder Specification, l Appendix II. Product Data Sheets, www.fda.gov/ohrms/./95s-0316-rpt0130-08-Appendix-II-vol90.pd, accessed 17 February 2012.
- Y. L. Yep, D. Li, N. J. Mann, O. Bode and A. J. Sinclair, Bread enriched with microencapsulated tuna oil increases plasma docosahexaenoic acid and total omega-3 fatty acids in humans, Asia Pac. J. Clin. Nutr., 2002, 11(4), 285–291 CrossRef CAS.
- D. H. Volker, X. Weng and P. Quaggiotto, Bioavailability of long chain omega-3 polyunsaturated fatty acid enriched luncheon meats, Nutr. Diet., 2005, 62, 130–137 CrossRef.
- S. Higgins, Y. L. Carroll, N. M. O'Brien and P. A. Morrissey, Use of microencapsulated fish oil as a means of increasing n-3 polyunsaturated fatty acid intake, J. Hum. Nutr. Diet., 1999, 12, 265–271 CrossRef.
- C. J. Barrow, C. Nolan and B. J. Holub, Bioequivalence of encapsulated and microencapsulated fish-oil supplementation, J. Funct. Foods, 2009, 1, 38–43 CrossRef CAS.
- L. B. Schram, C. J. Nielsen, T. Porsgaard, N. S. Nielsen, R. Holm and H. Mu, Food matrices affect the bioavailability of (n-3) polyunsaturated fatty acids in a single meal study in humans, Food Res. Int., 2007, 40, 1062–1068 CrossRef CAS.
- L. M. Arterburn, H. A. Oken, J. P. Hoffman, E. Bailey-Hall, G. Chung, D. Rom, J. Hamersley and D. McCarthy, Bioequivalence of docosahexaenoic acid from different algal oils in capsules and in a DHA-fortified food, Lipids, 2007, 42, 1011–1024 CrossRef CAS.
- J. Parada and J. M. Aguilera, Food microstructure affects the bioavailability of several nutrients, J. Food Sci., 2007, 72(2), R21–R32 CrossRef CAS.
|
This journal is © The Royal Society of Chemistry 2013 |
Click here to see how this site uses Cookies. View our privacy policy here.