DOI:
10.1039/C2AN35974J
(Paper)
Analyst, 2013,
138, 118-122
2p or not 2p: tuppence-based SERS for the detection of illicit materials†
Received
18th July 2012
, Accepted 10th October 2012
First published on 11th October 2012
Abstract
Deposition of silver onto British 2p coins has been demonstrated as an efficient and cost effective approach to producing substrates capable of promoting surface enhanced Raman scattering (SERS). Silver application to the copper coins is undemanding taking just 20 s, and results in the formation of multiple hierarchial dendritic structures. To demonstrate that the silver deposition sites were capable of SERS the highly fluorescent Rhodamine 6G (R6G) probe was used. Analyses indicated that Raman enhancement only occurs at the silver deposition sites and not from the roughened copper surface. The robustness of the substrate in the identification and discrimination of illegal and legal drugs of abuse was then explored. Application of the drugs to the substrates was carried out using spotting and soaking methodologies. Whilst little or no SERS spectra of the drugs were generated upon spotting, soaking of the substrate in a methanolic solution of the drugs yielded a vast amount of spectral information. Excellent reproducibility of the SERS method and classification of three of the drugs, 4-methylmethcathinone (mephedrone), 5,6-methylenedioxy-2-aminoindane (MDAI) and 3,4-methylenedioxy-N-methylamphetamine (MDMA) were demonstrated using principal components analysis and partial least squares.
Introduction
Raman has long been acknowledged as a vibrational spectroscopic technique capable of exquisite analyte discrimination. It is because of the information-rich spectra produced that Raman spectroscopy is a renowned technique in the detection and identification of illegal and legal substances of abuse.1,2 Raman scattering has been used for the detection of MDMA,3,4 cocaine,5 benzodiazepines,6,7 mephamphetamine,8 pharmaceutically active drugs9 and precursors.10 Although a wealth of knowledge has been ascertained about these chemicals using Raman it is undeniable that the technique has its flaws. These include a very weak scattering phenomenon making the detection of analytes present at low concentrations difficult and the masking of Raman spectra in the presence of fluorescent sample impurities. One way of overcoming these problems is to use surface enhanced Raman scattering (SERS). In SERS the analyte of interest is generally brought into close proximity to a roughened metallic surface, which is usually composed of either silver or gold.11 Both SERS12,13 and hyphenated SERS14 systems have been used in drug detection. However, the technique has never been applied to legal drug derivatives also known as ‘designer drugs’. Recently an increased usage of these drugs has been witnessed worldwide and it is thought that this is most likely due to the drugs being freely available and offered at a low price. Although many of these drugs exploit loopholes in the law to maintain their legal status, the potential health risk of these substances should not be dismissed, as users and academics alike have little access to information regarding the drug activity or dosage. Designer drugs include MDAI,15 and also the infamous mephedrone, a member of the cathinone family which adopted an illegal status at the beginning of 2010.16 Classification of illegal drugs within the UK is carried out on a scale of A–C. Drugs in category A are deemed the most harmful whilst drugs in category C are thought to be the least harmful. Mephedrone is categorised as a class B drug.
SERS can be facilitated in either solution or using a thin roughened film. The use of solid-state SERS substrates bypasses the difficulty involved in optimising experimental parameters in solution, but the synthesis of surfaces can vary in complexity with the most complex often requiring specialised equipment and labour intensive manufacture. Commercially available thin films are generally expensive to purchase, so we sought to develop a methodology for the production of a solid state SERS substrate that was cheap, facile and accessible, providing a robust surface that can be used by non-specialist groups.
An attractive synthetic route to substrate formation is through the exploitation of simple redox chemistry via the use of galvanic replacement.17 Here the reaction is used to galvanise British 2p coins with silver, the reaction proceeds as the main component of the coins is copper, which has a lower standard electrode potential than the silver solution. This results in the replacement of copper atoms with silver atoms and causes the deposition of a silver target onto the coin. This displacement reaction involving silver has been used a number of times to produce SERS active substrates with the oxidised metal most commonly being either copper,18 or zinc.19 The morphologies that result from the deposition are dependent on a number of factors such as oxidant concentration, deposition temperature and time.20 SERS substrates have previously been produced on US pennies21 but their elemental composition differs from bronze British coins and it is this difference in metallic composition that can ultimately affect the morphology of deposited silver and resultant SERS enhancement factors.
Experimental
Materials
Silver nitrate (99.999%) and Rhodamine 6G were purchased from Sigma Aldrich (Dorset, U.K.). British 2p coins were sorted into pre-1992 and post-1992 categories with only the pre-1992 coins being used for analysis. The drugs, amphetamine, MDMA, ketamine and cocaine were analysed with Home Office license approval. Mephedrone was acquired online from www.meowmeow.com a site which has since closed due to the substance being categorised as a class B drug. MDAI advertised under the name Sparkle was purchased from a head shop (Dr Hermans, Manchester, U.K.). Purity of the mephedrone was verified using accurate mass spectrometry, 1H and 13C nuclear magnetic resonance spectroscopy (NMR) as well as elemental analysis. The purity MDAI was assessed using accurate mass spectrometry, 1H and 13C nuclear magnetic resonance spectroscopy (NMR) and melting point tests. Each analytical method confirmed the drugs to be of high purity, and did not highlight the presence of any starting reagents, structural precursors, derivatives or excipients, therefore the drugs were used as supplied. All solvents used throughout the synthesis were of analytical grade.
Methods
Synthesis of the silver on copper (SoC) substrate
To ensure a high copper content of the coins pre-1992 2p bronze coins were selected; the composition of which is 97% copper, 2.5% zinc and 0.5% tin. By contrast post-1992 2p coins are composed of copper-plated steel, so due to their unclearly defined composition these coins were not used for R6G or drug analysis. However, we are also able to deposit silver targets on these surfaces via galvanic replacement (Fig. S1†). Before silver deposition was carried out the coins were scrubbed using a soapy solution and then sonicated in 1% methanolic acetic acid for 30 min to remove oxides and copper scale. After rinsing in copious amounts of methanol the coins were dried under a stream of nitrogen. Upon completion of these steps the coins were rendered clean. The silver deposition site was created by spotting 10 μL of a 0.1 M silver nitrate solution onto the coin's surface at room temperature (23 °C). The reaction was left to proceed for 20 s before the excess silver nitrate and copper nitrate solutions were gently removed using a sequential wash with water and methanol. The coins and resultant silver deposition sites were thoroughly dried using a stream of nitrogen. All silver surfaces were used within an hour of synthesis, thus avoiding oxidation. The average diameter across 5 replicate silver targets was calculated to be 5 mm. It is also important to mention that the application of silver to the coins surface does not deface the coin because the silver crystalline deposits can be easily removed upon rubbing with a damp paper towel.
SEM analysis of the SoC substrates was carried out using a Zeiss Supra 40 VP field-emission gun scanning electron microscope (FEG-SEM; Carl Zeiss SMT GmBH, Oberkochen, Germany) operating at a voltage of 1 kV.
Raman mapping
SERS analyses were undertaken using a WITec Alpha 300R confocal Raman instrument (WITec GmbH, Ulm Germany) equipped with a piezo-driven XYZ scan stage and a 632.8 nm wavelength laser. The power at the sample was measured at ∼1.0 mW and the laser was focussed using an Olympus 100×/0.5 objective. Spectra were collected across an area of 20 μm × 20 μm using a step size of 1 μm meaning a total of 400 spectra were collected to generate a map. Each spectrum had an integration time of 0.1 s.
Application of R6G to the interface between the silver deposition site and the roughened copper surface – SERS testing
A 1 × 10−4 M methanolic R6G solution was prepared. Methanol was used to dissolve the R6G since its rapid evaporation when applied to the substrates surface does not result in the formation of a ‘coffee ring’.22 The high concentration of R6G is also vital in this proof of principle experiment whereby it must be ensured that the number of R6G molecules deposited on the interface between the silver target and copper surface is sufficient to test the SERS response of the two areas. To the edge of the silver site 0.5 μL of the rhodamine solution was spotted. It is estimated that through careful deposition the diameter of the methanolic R6G spot could be maintained at ∼2 mm. The coin was then subjected to a gentle stream of nitrogen to ensure all the methanol had evaporated from the surface. Once completely dry the coin was immediately interrogated using Raman.
Application of the illegal and legal drugs to the coins surface: spotting and soaking
Methanolic solutions of all the drugs were made up to a concentration of 1 × 10−4 M. This concentration was chosen because it is sufficiently low enough to enable the detection of drugs imported in solution.23 For application of the drugs to the surface via spotting, 1 μL of each drug solution was placed onto individually prepared silver targets and left to dry. It was estimated the drug spot diameter was maintained at ∼2 mm comparable to the R6G spots. Assuming that there is a homogenous layer of analyte cast over the surface when employing the spotting technique then a direct relationship can be derived from the spot size and the number of molecules in relation to the concentration of the analyte contained in solution. However, due to the rapid manner in which the methanol evaporates there may not be enough time for the analyte to orientate into a position which it forms a favourable interaction with the surface thus limiting its chances of being enhanced. Similarly, the inability to orientate in a reproducible way could result in the significant variation in spectra collected, making identification and discrimination of the drug extremely difficult. To overcome the problems that may be encountered using the spotting technique, soaking was used. For this individual substrate preparations were submerged in the drug solutions for 10 min then removed. This allows the drugs to form selective interactions with the substrate and orientate themselves in an ordered manner, reducing any difficulties in spectral assignment. It should be noted that soaking the substrates is independent of the original drug solution concentration. If the analyte chooses to interact favourably with the substrate it will do so regardless of the number of molecules contained in solution. Once the coins were dried SERS spectra were generated from around the silver deposition centre to avoid any bias that mapping at the target edge may generate.
Results and discussion
SEM analysis of the coins was carried out on the bare copper surface and the silver deposition site (Fig. 1). The surface of the copper is understandably roughened due to the period of time the coins had spent in circulation. However it has been readily reported that the morphology of the templating surface is crucial in defining the structural outcome of fractals synthesised using galvanic replacement.17 Visualisation of the silver deposition sites reveals the presence of silver microcrystalline domains which display complex hierarchical morphologies. Magnification of the fractals shows the presence of fern-like-structures, which exhibit secondary silver crystalline growth perpendicular to a primary silver backbone. Once successful deposition of the silver had been observed it was necessary to test whether the silver site was SERS active and also whether the roughened copper surface alone was capable of Raman enhancement. The analyte used for this purpose was R6G because in the absence of a SERS active substrate it exhibits a high level of fluorescence when illuminated with visible radiation. Chemical maps of the interface between the silver deposition site and copper surface were collected once the R6G had been deposited. For the purpose of identifying at which positions the SERS signal of R6G arose, a map of the intensity at 1368 cm−1 assignable to the C–C + C–N stretching vibrations was generated. Fig. 2A shows an image which illustrates the deposition boundary with the red box signifying the areas from which Raman/SERS spectra were collected. It can be seen that the peak at 1368 cm−1 is only detectable on the silver surface and not on the copper signified by the green and yellow dots respectively. Fig. 2B shows a 3D plot of the intensity, again spectra of R6G are only observed on the surface of the silver and not on the roughened copper. Once it was proven that the silver substrate was SERS active analysis of the drugs could take place. To ascertain whether the drugs were detectable on the surface of bare 2p coins, both methods of application; soaking and spotting were used. The spectra generated (example spectrum is shown in Fig. S2†) contained no bands, just background noise. This verified that identification of the drugs was not possible on the copper surface alone. Similarly, drug solutions which had been spotted onto the silver sites also contained no consistent bands assignable to the drugs. However, when the coins were soaked in the solutions SERS spectra were acquired. Fig. 3 shows example SERS spectra acquired from the drug solutions (MDMA, mephedrone and MDAI which were analysed more frequently than the other analytes). Spectra for the other drugs and tentative assignments for all drugs can be found in the ESI section (Fig. S3 and Tables S1–S6†).
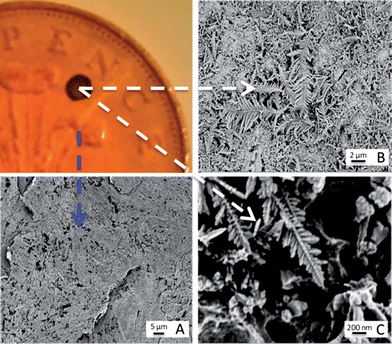 |
| Fig. 1 Characterisation of galvanic displacement. The optical image (top left) shows a clean British 2p coin, with silver deposited onto its surface. (A) shows an SEM of the rough surface of the tuppence after cleaning. The SEM in (B) shows the silver dendritic structures that are formed on the coins surface once 10 μL of AgNO3 was left to mature for 20 s at room temperature (23 °C). The fern like structures are magnified in (C) and show that secondary crystalline domains grow perpendicular from a primary silver backbone. | |
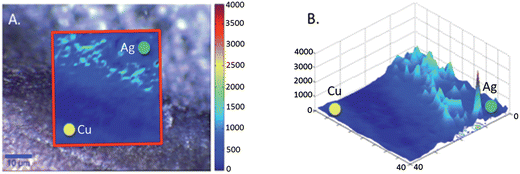 |
| Fig. 2 Chemical maps on Rhodamine 6G on the 2p coin surface. (A) Shows the Raman/SERS map generated using the peak intensity at 1368 cm−1 the spectra were acquired across the interface between the silver deposition site (green dot) and the copper coin (yellow dot) the map is overlaid onto an optical image of the interface. (B) Shows a 3D plot of the intensity observed on both the copper and silver sites. | |
Chemometric methods were then used to assess the reproducibility and discriminatory abilities of SERS. Prior to analysis the spectra were pre-processed (this process is shown in Fig. S4†). Initially the raw SERS spectra were filtered using Daubechies 5 wavelet function with 3 levels of decomposition, detail coefficients were replaced with 0 s while the approximation coefficients were kept unchanged. The spectra were then reconstructed using the wavelet coefficients to remove the high frequency noises and spikes. Data were then normalised using an extended multiplicative scatter correction with a bin size of 9. The spectra were then autoscaled, which mean-centres each value of the columns and then divides by the row entries of a column by the standard deviation calculated within the column. Initial principal components analysis (PCA) showed excellent reproducibility of the SERS method (Fig. S5†). Partial least squares (PLS) discriminant analysis was then used for classification of three of the drugs. Mephedrone, MDAI and MDMA were chosen as they were analysed the most (n = 201), and three separate PLS1 models were generated for each of the drugs. To assess the stability and predictive nature of the models bootstrap validation (1000 iterations) was carried out (see ESI† text for details). One output of PLS is the generation of a confusion matrix (Fig. S5A–C†), which shows the number of false positives (FP), true positives (TP), true negatives (TN) and false negatives (FN). These can be used to assess prediction sensitivity, specificity, precision and accuracy (calculations also detailed in ESI†). A summary of the PLS prediction capabilities is provided in Table 1. Overall it can be seen that the specificity, precision and accuracy for the three analytes is >0.95 meaning that the models generated were robust enough to predict the presence of the drug from its SERS spectra and this confirmed that these SERS-PLS1 models could generalize accurately. Whilst the sensitivity is lower for MDMA due to the high number of false negatives identified (Fig. S6A–C†), the models displayed an excellent sensitivity for MDAI and mephedrone. An additional output of PLS is loadings plots (Fig. S7A–C†) which allows the assignment of the most important features for each of the models and details which vibrational modes are discriminatory, the majority of which could readily be assigned to the three drugs.
Table 1 Summary of the results generated from PLS for each of the drugs analysed
Drugs |
FP |
TP |
TN |
FN |
Sensitivity |
Specificity |
Precision |
Accuracy |
MDMA (n = 36) |
1 |
26 |
164 |
10 |
0.72 |
0.99 |
0.96 |
0.95 |
MDAI (n = 109) |
0 |
105 |
92 |
4 |
0.96 |
1 |
1 |
0.98 |
Mephedrone (n = 56) |
0 |
51 |
145 |
5 |
0.91 |
1 |
1 |
0.98 |
Conclusion
In summary, a rapid and facile method of generating robust SERS substrates through the exploitation of standard electrode potentials has been exhibited. The silver deposited onto the copper surface has the ability to be used in the detection and discrimination of illegal and legal substances present in solution at low concentrations when combined with supervised multivariate analysis methods such as PLS. We believe that this robust approach demonstrated on 2p coins has general utility for the non-specialist to exploit SERS.
Acknowledgements
RG and SM thank the RSC and EPSRC for funding SM's Analytical Studentship.
Notes and references
-
S. J. Bell, S. P. Stewart and S. J. Speers, in Infrared and Raman Spectroscopy in Forensic Science, ed. J. M. Chalmers, H. G. M. Edwards and M. D. Hargreaves, John Wiley & Sons, West Sussex, 2012, ch. 6, p. 317 Search PubMed.
- M. J. West and M. J. Went, Drug. Test. Anal., 2010, 3, 532 CrossRef.
- S. E. J. Bell, D. T. Burns, A. C. Dennis, L. J. Matchett and J. S. Speers, Analyst, 2000, 125, 1811 RSC.
- S. E. J. Bell, D. T. Burns, A. C. Dennis and J. S. Speers, Analyst, 2000, 125, 541 RSC.
- A. G. Ryder, G. M. O'Connor and T. J. Glynn, J. Raman Spectrosc., 2000, 31, 221 CrossRef CAS.
- G. A. Neville, H. D. Beckstead and H. F. Shurvell, J. Pharm. Sci., 1994, 83, 143 CrossRef CAS.
- G. A. Neville, H. D. Beckstead, D. B. Black, B. A. Dawson and H. F. Shurvell, J. Pharm. Sci., 1994, 83, 1274 CrossRef CAS.
- H. Tsuchihashi, M. Katagi, M. Nishikawa, M. Tatsuno, H. Nishioka, A. Nara, E. Nishio and C. Petty, Appl. Spectrosc., 1997, 51, 1796 CrossRef CAS.
- J. P. Pestaner, F. G. Mullick and J. A. Centeno, J. Forensic Sci., 1996, 41, 1060 CAS.
- N. Milhazes, F. Borges, R. Calheiros, M. Paula and M. Marques, Analyst, 2004, 129, 1106 RSC.
- P. C. Lee and D. Meisel, J. Phys. Chem., 1982, 86, 3391 CrossRef CAS.
- K. Faulds, W. E. Smith, D. Graham and R. J. Lacey, Analyst, 2002, 127, 282 RSC.
- A. Ruperez, R. Montes and J. J. Laserna, Vib. Spectrosc., 1991, 2, 145 CrossRef CAS.
- G. Trachta, B. Schwarze, B. Sägmüller, G. Brehm and S. Schneider, J. Mol. Struct., 2004, 693, 175 CrossRef CAS.
- D. E. Nichols, W. K. Brewster, M. P. Johnson, R. Oberlender and R. M. Riggs, J. Med. Chem., 1990, 33, 703 CrossRef CAS.
- The Misuse of Drugs (Amendment) (England, Wales and Scotland) Regulation, 2010, 1144, 16th April 2010. Archived from original on 28th May 2012.
- C. M. Cobley and Y. Xia, Mater. Sci. Eng., R, 2010, 70, 44 CrossRef.
- J. Hao, Z. Xu, M.-J. Han, S. Xu and X. Meng, Colloids Surf., A, 2010, 366, 163 CrossRef CAS.
- S. Lv, H. Suo, X. Zhao, C. Wang, S. Jing, T. Zhou, Y. Xu and C. Zhao, Solid State Commun., 2009, 149, 1755 CrossRef CAS.
- S. Mabbott, I. A. larmour, V. Vishnyakov, Y. Xu, D. Graham and R. Goodacre, Analyst, 2012, 137, 2791 RSC.
- J. Betz, Y. Cheng and G. W. Rubloff, Analyst, 2012, 137, 826 RSC.
- X. Shen, C.-M. Ho and T.-S. Wong, J. Phys. Chem. B, 2010, 114, 5269 CrossRef CAS.
- C. Eliasson, N. A. Macleod and P. Matousek, Anal. Chim. Acta, 2008, 607, 50 CrossRef CAS.
Footnote |
† Electronic supplementary information (ESI) available: Tentative band assignments for all the drugs analysed, representative SERS spectra for the drugs ketamine, cocaine and amphetamine, details of SERS data pre-processing and resultant PCA plots of all processed SERS spectra, a guide to the PLS predictions of the drugs (including equations showing how sensitivity, specificity, precision and accuracy are calculated), PLS1 bootstrap results for MDMA, MDAI and mephedrone and the annotated PLS1 loadings plots for MDMA, MDAI and mephedrone. See DOI: 10.1039/c2an35974j |
|
This journal is © The Royal Society of Chemistry 2013 |