DOI:
10.1039/C2RA22529H
(Paper)
RSC Adv., 2012,
2, 12866-12869
One-pot synthesis of anhydrous silver carboxylates from silver(I) fluoride
Received
16th October 2012
, Accepted 17th October 2012
First published on 6th November 2012
Abstract
A new one-pot, water-free method for the synthesis of silver carboxylates is described. The preparation employs the direct reaction of AgF with the carboxylic acid in a non-aqueous solvent and isolated yields are above 70% for straight-chain and simple aromatic carboxylates and slightly lower for perfluorinated carboxylates. Disilver salts of dicarboxylic acids can also be prepared this way, but attempts to extend the reaction to other transition metals were successful only with copper.
Introduction
Transition metal carboxylates are some of the most common and well-studied coordination compounds.1 This is due to interest in their structural chemistry,2 varied properties and applications,3,4 and use as synthetic precursors.5 Dinuclear tetracarboxylate derivatives with the so-called ‘paddlewheel' structure are probably the most extended class among these complexes and probably among all the dinuclear coordination compounds in general.6,7 They have been described for several different transition metals from the first (Cr, Co, Cu, Ni), second (Mo, Ru, Rh) and third row (W, Re, Os, Ir), and many important studies of multiple metal–metal bonds, magnetic properties and liquid-crystallinity have originated from these studies. For example, such complexes of copper(II), rhodium(II), molybdenum(II), chromium(II) diruthenium(II, III) and ruthenium(III) are known to have liquid crystal properties,8 while rhodium(II) carboxylates are known, for example, as catalysts in organic reactions.9 To a lesser extent, carboxylates of rare-earth ions have also attracted some interest, basically due to the very rich structural diversity, optical and magnetic properties that these species are able to show.10–13
Silver(I) carboxylates have been studied thoroughly,14–22 and among their varied uses are some important applications in areas of applied chemistry. For instance, they have been employed in photothermographic imaging materials since the early 1960s23 and, more recently, as efficient precursors of silver nanoparticles generated by thermal decomposition and chemical vapour deposition.24–26 In particular, silver(I) perfluoroalkanoates have been found to be exceptional materials for the latter application due to their chemical and thermal stability.27–29 Consultation of the patent literature also confirms that silver carboxylates are materials of significance.30
The effective methods of synthesis of silver(I) carboxylates described in the literature are based on aqueous reactions. The most common path is a metathesis of a water-soluble silver salt, generally AgNO3, and the corresponding alkali metal carboxylate.31 Some variations involve the in situ preparation of Ag2CO3 and its reaction with the carboxylic acid,32 or the addition of freshly prepared Ag2O to a basic solution of the carboxylic acid.33 The yields are generally high when simple carboxylates are the target, such as small alkanoates or aromatic groups. However, the efficiency decreases significantly when larger organic groups or water-insoluble carboxylic acids are involved. Additionally, when the alkaline carboxylate is not available commercially, these methods imply either its preparation or the synthesis of silver carbonate or silver oxide.
Therefore, as pure, anhydrous materials are required for the applications described above, the fact that preparative methods predominantly employ water as a reaction solvent and that these methods are not always so readily applicable to a wide range of acids means that the availability of silver carboxylates is a little limited. For example, recently, we described the preparation of dinuclear complexes of PtIII obtained via oxidation of PtII using DMSO/H+, the structure is shown in Fig. 1, and it can be seen that they possess a bridging carboxylate function arising from the carboxylic acid used as the solvent.34 While acids, such as propionic and butyric acid work as solvents in these systems, longer chain acids are much less easy to deal with, rather limiting the range of materials that can be made. However, a subsequent paper from Anger et al.35 showed that the oxidation could be carried out equally as well using AgI and, indeed, early reports of related PtIII dimers had also used silver as an oxidant in the preparation.36,37 Thus, there was a need to have simple and effective routes into a range of silver carboxylates to facilitate further study of this system.
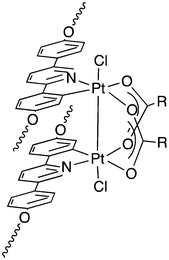 |
| Fig. 1 Structure of the liquid-crystalline complexes of PtIII containing bridging carboxylate ligands. | |
Results and discussion
Fluoride has long been recognised for its qualities as a base,38 something that is particularly evident in water-free environments. For example, Bu4NF is quite stable so long as it is slightly wet, but when dried at temperatures around 70–80 °C, an E2 reaction occurs leading to the formation of [F–H–F]−, Bu3N, and 1-butene according to:39
2 Bu4NF → (Bu4N)[FHF] + Bu3N + CH2 CH–CH2–CH3 |
However, at lower temperatures Bu4NF appears more stable39,40 and perhaps the purest samples were reported by Sun and DiMagno in an elegantly conceived reaction between Bu4N+CN− and C6F6.41
Yet as observed by Clark, F− is not a conventional Brønsted base in that it does not react with water, rather being solvated by it. Indeed, referring to Clark and Emsley,42 Clark went so far as to suggest that ‘the role of fluoride in base-assisted reactions should be differentiated from that of a simple proton acceptor'.38 Thus, for example, dissolution of KF in acetic acid leads to very strong F⋯H hydrogen bonds (estimated calorimetrically43 as >100 kJ mol−1) with a significant increase in the nucleophilicity of the carboxylate anion.
Despite what might be regarded as some equivocation about the basic nature of F−, it seemed that the fact that it does interact strongly with the hydrogens of carboxylic acids, and that it can be used in aprotic solvents was a sufficient reason to investigate its potential as a base in the form of AgF to form silver carboxylates. Thus, AgF is an easy-to-handle, non-hygroscopic salt, similar in price to AgNO3 and commonly available. Its reaction with RCO2H to give AgO2CR would, of course, generate HF but this does not present a serious hazard as it can easily be neutralised in a simple bubbling trap with a base, especially on the scale used here.
Thus, reactions were conducted between AgF and a series of carboxylic acids in THF at ambient temperature as indicated in Scheme 1. Thus, the AgF is suspended in THF and the carboxylic acid is added. The brown colour of the AgF gradually disappears concomitant with the appearance of a fine, colourless precipitate of the product. The product is obtained by simple filtration and washing, and there is no contamination from the residual AgF, which is attributed to its solubilisation via formation of a strong hydrogen bond with the unreacted acid, consistent with the observations described above. Support for the proposal that AgF is so solubilised comes from 19F NMR data obtained from a post-reaction solution, recorded in the THF solvent with a small amount of C6D6 added as a lock, which showed a number of species present.
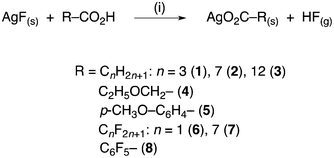 |
| Scheme 1 Silver(I) carboxylates synthesised in this work. Conditions: (i) THF–hexane, N2, r.t., 6 or 15 h. | |
The reaction was tested with a range of substrates, the best results obtained using two equivalents of the carboxylic acid and a solvent combination of tetrahydrofuran and hexane in a 2
:
1 ratio (see experimental for further details). Thanks to the entropic advantage accruing from the formation of hydrogen fluoride and a (generally) insoluble carboxylate, the effectiveness of the reaction is high and the reaction times are relatively short (6 h or occasionally overnight at room temperature, see Table 1). Furthermore, an advantage of the reaction is that as it is under water-free conditions, the anhydrous silver carboxylates obtained do not require further purification. Thus, very good results were obtained with aliphatic and aromatic substrates (1–5: 72–86%), while slightly lower yields were observed for perfluorinated derivatives (6–8: 55–66%). Nonetheless, it is re-emphasised that the materials were obtained in just one step directly from the commercial starting materials.
Table 1 Characterisation data for complexes 1–12
Compound |
R–COO |
T (h) |
Yield (%) |
v
(COO)/cm−1 |
Analytical data calculated (Found) |
C |
H |
1
|
C3H7 |
6 |
86 |
1516, 1407 |
24.6 (24.2) |
3.6 (3.4) |
2
|
C7H15 |
6 |
85 |
1514, 1415 |
38.3 (37.87) |
6.0 (5.9) |
3
|
C12H25 |
15 |
72 |
1516, 1423 |
48.6 (48.2) |
7.9 (7.7) |
4
|
C2H5OCH2 |
6 |
76 |
1568, 1396, 1376 |
22.8 (22.5) |
3.3 (3.1) |
5
|
4-CH3O–C6H4 |
6 |
83 |
1601, 1512, 1390 |
37.1 (37.5) |
2.7 (2.8) |
6
|
CF3 |
15 |
66 |
1617, 1460 |
10.9 (11.2) |
|
7
|
C7F15 |
15 |
58 |
1650, 1455 |
18.5 (18.1) |
|
8
|
C6F5 |
15 |
55 |
1610, 1492 |
26.4 (26.0) |
|
9
|
O2C–CH2 |
15 |
36 |
1558, 1408 |
11.3 (10.9) |
0.6 (0.6) |
10
|
O2C–(CH2)4 |
15 |
39 |
1514, 1428 |
20.0 (19.3) |
2.2 (2.0) |
11
|
O2C–Ph |
15 |
35 |
1630, 1504 |
25.3 (44.9) |
1.1 (2.7) |
12 (Cu) |
C4H9 |
6 |
23 |
1585, 1463 |
40.4 (39.9) |
5.9 (5.6) |
The integrity of the complexes was proven by elemental analysis and infrared spectroscopy, recorded as nujol mulls in polyethylene sheets. The IR spectra showed the usual absorptions due to the stretching modes,
(COO) of the carboxylic group between 1601 and 1390 cm−1 for the compounds 1–5, and at higher frequencies (1650–1455 cm−1) for the perfluorinated derivatives 6–8, indicating, as expected, a monodentate coordination to the silver centre.44,45 Note also, that very intense
(C–F) stretching bands were also observed in the region 1291–1150 cm−1 of the spectra of the perfluoroalkanoates 6–8.
Polycarboxylates are also substrates of great interest as they can act as bridging ligands for the construction of framework architectures with a wide range of structural diversity.46–50 Regarding this and with the same aim of evaluating the efficacy of AgF, the reactivity of three different dicarboxylic acids was assessed (Scheme 2). Thus, disilver salts of malonate (9, R = CH2), adipate (10, R = (CH2)4) and terephthalate (11, R = C6H4) were synthesised and their integrity investigated by elemental analysis and IR spectroscopy. In these reactions, the ratio of carboxylic acid to silver fluoride needed to be adjusted for the reactions to 1
:
2 in order to minimise the formation of the monometallic polymeric {Ag(O2CRCO2)}n. That some of this polymer is undoubtedly formed during the reaction, despite the different ratio of reagents, is almost certainly responsible for the generally lower yields obtained in these reactions when compared to the preparation of the salts of the simple mono carboxylic acids. Indeed, in the case of terephthalic acid, the polymer is evidently highly insoluble, as it was not possible to obtain an analytically pure sample of the disilver salt by this method.
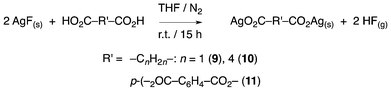 |
| Scheme 2 Synthesis of the dinuclear compounds Ag2(O2C–R′–CO2–) 9–11. | |
Then, in order to see if this method might be more generally applicable, attempts were made to extend the reaction to other transition metal fluorides. Thus, the reaction of butanoic acid was attempted with the fluorides of copper(II), iron(III), cobalt(III) and cobalt(II). Unfortunately, only one of these was successful, namely the preparation of copper(II) tetrabutanoate (12), which was obtained in a rather low yield. In the other cases, no evidence of the formation of the metal carboxylate was observed and most of the starting metal fluoride was recovered, even after two days under reflux.
Conclusions
A simple, one-pot, anhydrous and high-yielding preparation of silver carboxylates is reported starting from silver fluoride and the carboxylic acid, using THF or THF–hexane as the solvent. The HF produced helps drive the reaction entropically and is readily trapped. Disilver salts of dicarboxylic acids may also be obtained this way, but in a lower yield. Preparation of other metal carboxylates starting from the metal fluoride was successful only for copper(II).
Experimental
Schlenk- and vacuum-line techniques were employed for all manipulations. All the reactants were used as purchased without further purification. The infrared absorption spectra were recorded in a Thermo Nicolet FTIR Avatar 370 spectrometer, from nujol mull samples within two thin polyethylene layers. Elemental analysis was carried out on an Exeter Analytical Inc CE 440 Elemental Analyser and a Sartorius SE2 analytical balance at the University of York. It should be noted that elemental analysis for the perfluorinated compounds 7 and 8 was not straightforward as combustion under conventional conditions gave low carbon values and finite nitrogen values. Samples were therefore analysed at combustion temperatures above 1000 °C in tin capsules and using Pb3O4 as a combustion additive.
Synthesis of silver carboxylates 1–5
Silver(I) fluoride (0.40 g, 3.15 mmol) was suspended in a mixture of anhydrous tetrahydrofuran (35 cm3) and hexane (15 cm3) in a three-necked, round-bottom flask under a N2 atmosphere in the dark. A bubbling trap containing an aqueous solution of NaHCO3 (150 cm3, saturated) was connected to one of the necks while a small, continuous flow of N2 passed through the system. The relevant carboxylic acid (6.30 mmol, 2 mol equiv.) was added to the mixture. The N2 current remained throughout the entire reaction in order to remove HF from the system to be neutralised with the base in the trap. Progressively, a white solid appeared while the brownish AgF was consumed. After 6 h (1, 2, 4 and 5) or 15 h (3), the colourless, powdery solid was filtered, washed with diethyl ether (3 × 10 cm3) and air-dried. No further purification was needed.
Synthesis of silver perfluorocarboxylates 6–8
These reactions were carried out as described above for salts 1 to 5, except that in all cases the reaction was allowed to proceed overnight. The mixtures were then reduced to ca. 25% of their initial volume by rotary evaporation after which cold hexane (10 cm3) was added to precipitate the product as a colourless solid, which was recovered by filtration, washed with more cold hexane (3 × 5 cm3) and air-dried.
Synthesis of dinuclear silver carboxylates 9–11
Silver(I) fluoride (0.4 g, 3.15 mmol) was suspended in anhydrous THF (50 cm3) under a N2 atmosphere and in the dark. The corresponding dicarboxylic acid (1.57 mmol, 0.5 mol equiv.) was then added and the mixture was stirred at room temperature overnight under a gentle stream of nitrogen; a sodium bicarbonate bubble trap once more collected the HF evolved. The final mixture consisted of brown, unreacted AgF and a light, colourless solid that was the product. The two were separated by allowing the mixture to stand, after which all of the AgF had settled at the bottom of the flask while the product remained suspended. The product was recovered by decanting the mixture carefully and then twice more adding the solvent, allowing the mixture to stand and then decanting. The solid from the decanted fractions was then recovered by filtration, washed with diethyl ether (3 × 10 cm3) and air-dried.
Synthesis of [Cu2(μ2-O2CC3H7)4] 12
Butyric acid (1.08 cm3, 11.82 mmol) was added to a copper(II) fluoride (0.40 g, 3.94 mmol) suspended in a mixture of THF and hexane (50 cm3, ca 2
:
1). The reaction was stirred for 6 h at room temperature, during which the initially colourless suspension turned into a blue mixture. The colourless solid (unreacted CuF2) was filtered off and the deep blue solution was concentrated (∼2 cm3) by evaporation of the solvent, after which it was treated with cold hexane (10 cm3) to induce the precipitation of the carboxylate. The blue solid was filtered, washed with cold hexane (3 × 5 cm3) and air-dried.
Acknowledgements
We are grateful to the Comunidad Autonoma de la Rioja for funding to JT, the CNPq for funding to PAT and to Graeme McAllister (York) for his efforts with the combustion analysis of the perfluorinated compounds.
References
-
A. D. Pomogailo, G. I. Dzherdimalieva and V. N. Kestelman, Macromolecular Metal Carboxylates and their Nanocomposites, Springer, Berlin, 2008 Search PubMed
.
- L. Brammer, M. D. Burgard, C. S. Rodger, J. K. Swearingen and N. P. Rath, Chem. Commun., 2001, 2468 RSC
.
- S. Mishra, S. Daniele and L. G. Hubert-Pfalzgraf, Chem. Soc. Rev., 2007, 36, 1770 RSC
.
- B. V. Harbuzaru, A. Corma, F. Rey, J. L. Jorda, D. Ananias, L. D. Carlos and J. Rocha, Angew. Chem., Int. Ed., 2009, 48, 6476 CrossRef CAS
.
-
(a) R. Noguchi, A. Hara, A. Sugie and K. Nomiya, Inorg. Chem. Commun., 2006, 9, 355 CrossRef CAS
;
(b) E. Anger, M. Rudolph, L. Norel, S. Zrig, C. Shen, N. Vanthuyne, L. Toupet, J. A. G. Williams, C. Roussel, J. Autschbach, J. Crassous and R. Réau, Chem.–Eur. J., 2011, 17, 14178 CrossRef CAS
.
-
(a) M. A. S. Aquino, Coord. Chem. Rev., 1998, 170, 141 CrossRef CAS
;
(b) M. A. S. Aquino, Coord. Chem. Rev., 2004, 248, 1025 CrossRef CAS
.
- E. B. Boyar and S. D. Robinson, Coord. Chem. Rev., 1983, 50, 109 CrossRef CAS
.
- For a general discussion, see section 7.9.9.3 in: B. Donnio, D. Guillon, R. Deschenaux, D. W. Bruce, J. A. McCleverty, T. J. Meyer, in Comprehensive Coordination Chemistry II, Pergamon, Oxford, 2003, pp. 357 Search PubMed
.
-
(a) M. A. S. Aquino, Coord. Chem. Rev., 1998, 170, 141 CrossRef CAS
;
(b) M. A. S. Aquino, Coord. Chem. Rev., 2004, 248, 1025 CrossRef CAS
.
- A. Ouchi, Y. Suzuki, Y. Ohki and Y. Koizumi, Coord. Chem. Rev., 1988, 92, 29 CrossRef CAS
.
- L. Sun, G. Z. Li, M. H. Xu, X. J. Li, J. R. Li and H. Deng, Eur. J. Inorg. Chem., 2012, 1764 Search PubMed
.
- Z. J. Lin, B. Xu, T. F. Liu, M. N. Cao, J. A. Lu and R. Cao, Eur. J. Inorg. Chem., 2010, 3842 CrossRef CAS
.
- K. Binnemans, B. Heinrich, D. Guillon and D. W. Bruce, Liq. Cryst., 1999, 26, 1717 CrossRef CAS
.
- S. K. Adams, D. A. Edwards and R. Richards, Inorg. Chim. Acta, 1975, 12, 163 CrossRef CAS
.
- D. A. Edwards, M. Longley and J. Inorg, J. Inorg. Nucl. Chem., 1978, 40, 1599 Search PubMed
.
- E. Szlyk, I. Lakomska and A. Grodzicki, Thermochim. Acta, 1993, 223, 207 CrossRef CAS
.
- H. L. Zhu, X. M. Zhang, X. Y. Liu, X. J. Wang, G. F. Liu, A. Usman and H. K. Fun, Inorg. Chem. Commun., 2003, 6, 1113 CrossRef CAS
.
- K. Vehlow, K. Kohler, S. Blechert, S. Dechert and F. Meyer, Eur. J. Inorg. Chem., 2005, 2727 CrossRef CAS
.
- L. P. Olson, D. R. Whitcomb, M. Rajeswaran, T. N. Blanton and B. J. Stwertka, Chem. Mater., 2006, 18, 1667 CrossRef CAS
.
- J. S. Dong, D. R. Whitcomb, A. V. McCormick and H. T. Davis, Langmuir, 2007, 23, 7963 Search PubMed
.
- D. Sun, Z. H. Wei, D. F. Wang, N. Zhang, R. B. Huang and L. S. Zheng, Cryst. Growth Des., 2011, 11, 1427 CrossRef CAS
.
- P. N. Nelson and H. A. Ellis, Dalton Trans., 2012, 41, 2632 RSC
.
-
J. P. Cowdery-Corvan and D. R. Whitcomb, Handbook of Imaging Materials, Marcel-Dekker, New York, 2002 Search PubMed
.
- S. Mishra, S. Daniele and L. G. Hubert-Pfalzgraf, Chem. Soc. Rev., 2007, 36, 1770 RSC
.
- A. Grodzicki, I. Łakomska, P. Piszczek, I. Szymańska and E. Szłyk, Coord. Chem. Rev., 2005, 249, 2232 CrossRef CAS
.
- I. K. Shim, Y. I. Lee, K. J. Lee and J. Joung, Mater. Chem. Phys., 2008, 110, 316 CrossRef CAS
.
- E. Szlyk, I. Lakomska and A. Grodzicki, Thermochim. Acta, 1993, 223, 207 CrossRef CAS
.
- E. Szłyk, R. Szczesny and A. Wojtczak, Dalton Trans., 2010, 39, 1823 RSC
.
- S. J. Lee, S. W. Han and K. Kim, Chem. Commun., 2002, 442 RSC
.
-
(a)
D. R. Whitcomb,EU Pat., 0710877 B1, Eastman Kodak Company, 2001
;
(b)
Y. Li and J. S. C. Lee, H. Pan, P. F. Smith, H. K. Mahabadi, US Pat. 2009/0148600 A1, Xerox Corporation, 2009
;
(c)
S. Tholon, F. Luc, J. Barrault, S. Valange, E. Guelou, M. Daturi, F. Can, US Pat. 7648942, Commissariat a l'Energie Atomique, Centre National de la Reserche Scientifique, Universite de Poitiers , 2010 Search PubMed
.
- P. N. Nelson and H. A. Ellis, Dalton Trans., 2012, 41, 2632 RSC
.
- E. Szlyk, I. Lakomska and A. Grodzicki, Thermochim. Acta, 1993, 223, 207 CrossRef CAS
.
- H. L. Zhu, X. M. Zhang, X. Y. Liu, X. J. Wang, G. F. Liu, A. Usman and H. K. Fun, Inorg. Chem. Commun., 2003, 6, 1113 CrossRef CAS
.
- A. Santoro, M. Wegrzyn, A. C. Whitwood, B. Donnio and D. W. Bruce, J. Am. Chem. Soc., 2010, 132, 10689 CrossRef CAS
.
- E. Anger, M. Rudolph, C. Shen, N. Vanthuyne, L. Toupet, C. Roussel, J. Autschbach, J. Crassous and R. Réau, J. Am. Chem. Soc., 2011, 133, 3800 CrossRef CAS
.
- J. Kuyper and K. Vrieze, Transition Met. Chem., 1976, 1, 208 Search PubMed
.
- B. R. Steele and K. Vrieze, Transition Met. Chem., 1977, 2, 169 Search PubMed
.
- J. H. Clark, Chem. Rev., 1980, 80, 429 CrossRef CAS
.
- R. K. Sharma and J. L. Fry, J. Org. Chem., 1983, 48, 2112 CrossRef CAS
.
- D. P. Cox, J. Terpinski and W. Lawrynowicz, J. Org. Chem., 1984, 49, 3216 CrossRef CAS
.
- H. Sun and S. G. DiMagno, J. Am. Chem. Soc., 2005, 127, 2050 CrossRef CAS
.
- J. H. Clark and J. Emsley, J. Chem. Soc., Dalton Trans., 1975, 2129 RSC
.
- J. Emsley, J. Chem. Soc. A, 1971, 2702 RSC
.
- E. Szlyk, I. Lakomska and A. Grodzicki, Thermochim. Acta, 1993, 223, 207 CrossRef CAS
.
- P. N. Nelson and H. A. Ellis, Dalton Trans., 2012, 41, 2632 RSC
.
- L. Sun, G. Z. Li, M. H. Xu, X. J. Li, J. R. Li and H. Deng, Eur. J. Inorg. Chem., 2012, 1764 Search PubMed
.
- Z. J. Lin, B. Xu, T. F. Liu, M. N. Cao, J. A. Lu and R. Cao, Eur. J. Inorg. Chem., 2010, 3842 CrossRef CAS
.
- D. Sun, Z. H. Wei, D. F. Wang, N. Zhang, R. B. Huang and L. S. Zheng, Cryst. Growth Des., 2011, 11, 1427 CrossRef CAS
.
- B. H. Ye, M. L. Tong and X. M. Chen, Coord. Chem. Rev., 2005, 249, 545 CrossRef CAS
.
- H. El Bakkali, D. Choquesillo-Lazarte, A. Dominguez-Martin, M. D. Brandi-Blanco, A. Castineiras and J. Niclos-Gutierrez, Polyhedron, 2012, 31, 463 Search PubMed
.
|
This journal is © The Royal Society of Chemistry 2012 |
Click here to see how this site uses Cookies. View our privacy policy here.