DOI:
10.1039/C2RA21471G
(Paper)
RSC Adv., 2012,
2, 10869-10873
Received
17th July 2012
, Accepted 10th September 2012
First published on 12th September 2012
Abstract
The levulinate ester of 2-(benzothiazol-2-yl)phenol, 1, has been developed as a ratiometric fluorescent probe for identifying and quantitating sulfite anions. The mechanism of action is based on the sulfite-triggered intramolecular cleavage of the levulinate moiety to give 1 which, when excited with 310 nm light, decays to its ground state via an excited state intramolecular proton transfer (ESIPT) mediated pathway. We show that the intensity of the ESIPT fluorescent signal relative to that of 1 is proportional to sulfite concentration. This new probe shows good selectivity and high sensitivity for sulfite over other typically encountered anions (F−, Cl−, Br−, I−, HPO42−, SO42−, NO3−, AcO−, ClO4−, N3−, HCO3−) when measured in CH3CN/H2O (50
:
50, v/v) solution.
Introduction
Large quantities of mono- and divalent metal salts of the sulfite anion have been used in industry since the mid-1800's. The first major use of sodium sulfite was in the manufacture of wood pulp for the paper industry in 1867. Subsequently these materials have found wide use in the food industry1 where they are used as preservatives, anti-oxidants, bacteriostasis agents, and to control fruit and vegetable degradation caused by enzymatic or non-enzymatic reactions.2–4 In spite of their many beneficial uses, the willful or accidental release of large quantities of these chemicals into waste water streams can constitute a major environmental hazard. For example, sulfite contaminated waste water can seriously deplete the amount of oxygen in water to levels which are unsustainable to aquatic life forms.5 Moreover, sulfite can constitute a life-threatening hazard to allergy prone individuals; in-take of these anions by sensitized patients can cause acute breathing difficulties, asthma attacks, skin sensitivity and gastrointestinal reactions within minutes of ingestion.6,7 Additionally, their adverse effects can extend to general populations where they are known to be inimical to some vitamins and are suspected to be the cause of a variety of chronic health conditions.8 As a result, the presence of sulfite in the environment has aroused great concern and the use of sulfite in food stuffs and in waste water is strictly regulated in many countries. Recognition of these potential adverse effects has stimulated considerable interest in the development of methods to detect and quantify sulfite contaminants.
As a consequence of the concerns enumerated above, a variety of disparate schemes have been proposed for sulfite determination including electrochemistry,9–11 chromatography,12 chemiluminescence,13,14 electrochemical and enzymatic techniques.10,11 However, most of these conventional methods are either time-consuming, have poor selectivities, are expensive or can involve complicated procedures. Owing to operational simplicity and potential high sensitivity, fluorescent probes have been developed as alternative options for the detection of anions during the past decade.15–17 An important contribution in this regard has been made by Chang et al. who reported a turn-on type fluorescent probe for sulfite by chemical deprotection of resorufin levulinate.18 However, this intensity-based fluorescent sensor suffers from errors associated with the determination of probe concentration, environmental effects and instrument factors.19–24 Therefore, ratiometric fluorescent probes for sulfite detection are more desirable. So far, there is only one ratiometric probe for sulfite reported.25 The goal of the work reported herein was to develop a new ratiometric probe for sulfite that would allow us to more easily and accurately detect and quantitate this anionic species.
2-(Benzothiazol-2-yl)phenol and its derivatives26,27 are known to exhibit an excited state intramolecular proton transfer (ESIPT) from the phenol form to the keto form which results in an easily detectable fluorescence signal due to an accompanying large Stokes shift. To date, several derivatives of 2-(benzothiazol-2-yl)phenol have been reported as ratiometric fluorescent probes for F−, Hg2+, Zn2+, anionic species, pyrophosphate ions (PPi), and phosphates.28–33
These reports, coupled with Chang's findings that sulfite is capable of cleaving levulinate-protected phenol moieties selectively under mild and neutral conditions, inspired us to develop a novel ratiometric fluorescent probe 1,18,34 levulinate of 2-(benzothiazol-2-yl)phenol, for the detection of sulfite anions. Probe 1 was synthesized by the reaction of 2-(benzothiazol- 2-yl)phenol with levulinyl chloride in 65% yield, as shown in Scheme 1. Ester 1 shows a blue-violet fluorescence centered at 373 nm. Upon addition of sulfite, 1 is converted into phenolic dye 2 which, because of its ESIPT-mediated emission, exhibits a significant red-shifted fluorescent band centered at 468 nm which corresponds to a 95 nm red shift. The possible sulfite-selective signaling mechanism is shown in Scheme 2.
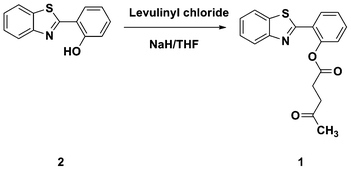 |
| Scheme 1 Synthesis of ratiometric probe 1 for sulfite. | |
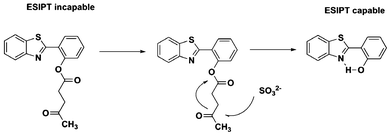 |
| Scheme 2 Possible sulfite-selective signaling mechanism. | |
Experimental
Materials and equipments
All titrations were carried out in HEPES buffer solution (10 mM, pH = 7.4, 50% acetonitrile, v/v). Unless otherwise noted, all chemicals obtained from commercial suppliers are analytical reagent grade and used as received. The fluorescence emission spectra are measured after 60 min of mixing. THF were refluxed with sodium and distilled under an atmospheric pressure. TLC plates and silica gel (200–300 mesh) were purchased from Qingdao Ocean Chemicals, China. The UV-Vis absorption spectra and fluorescence emission spectra were measured using a Shimadzu UV-2450 spectrophotometer and a HITACHI F-4600 spectrometer, respectively. 1H NMR spectra in CDCl3 were recorded using a Bruker 400 M AVANCE-III spectrometer with chemical shifts reported as ppm (TMS as the internal standard). Mass spectra were measured on a Shimadzu LC-MS-IT-TOF mass spectrometer.
Synthesis
2-(Benzothiazol-2-yl)phenol 2 and levulinyl chloride were synthesized according to the literature methods.35,36 Probe 1 was prepared as follows: to a stirred mixture of 2-(benzothiazol-2-yl)phenol 2 (227 mg, 1.0 mmol) and NaH (31 mg, 1.3 mmol) in 10 mL dry THF under an argon atmosphere, was added in a dropwise manner levulinyl chloride (174 mg, 1.3 mmol) at room temperature. The resulting reaction mixture was allowed to stir at room temperature overnight. The reaction was quenched with 1 mL of water and the solvent was evaporated under vacuum. The resulting solid product was extracted twice with 50 mL portions of dichloromethane. After drying over anhydrous Na2SO4, the organic solvent was filtered and removed by distillation to yield a yellow solid. Product 1 was purified by column chromatography on silica gel (3
:
1 hexane/ethyl acetate as eluent) and isolated as a pale yellow solid (210 mg, 65%). 1H NMR (400 MHz, CDCl3): δ (ppm) = 8.30 (d, J = 7.6 Hz, 1H), 8.09 (d, J = 8.4 Hz, 1H), 7.94 (d, J = 7.6 Hz, 1H), 7.51 (t, J = 7.6 Hz, 2H), 7.43–7.38 (m, 2H), 7.28 (s, 1H), 3.06 (t, J = 6.4 Hz, 2H), 2.93 (t, J = 6.4 Hz, 2H), 2.23 (s, 3H). m/z (LCMS-IT-TOF) M+ + 1: calculated, 326.0851; found, 326.0861.
Results and discussion
The absorption and fluorescence spectra of probe 1 and dye 2 are illustrated in Fig. 1. Due to the ESIPT process, the dye 2 displayed an emission band centered at 468 nm which is attributable to its keto tautomer. Because a corresponding ESIPT mechanism is not possible for 1, only the enol-like emission at 373 nm was observed. After addition of sulfite to a solution of 1 in HEPES buffer (10 mM, pH = 7.4, 50% acetonitrile), the fluorescence undergoes a 95 nm red shift and the emission band at 373 nm steadily decreases with the appearance of the emission band at 468 nm (Fig. 2a). There is a clear isoemission point at 426 nm. The above results indicate that sulfite can easily induce cleavage of the levulinate protection group while concomitantly generating the phenol moiety for the ESIPT–mediated emission.
 |
| Fig. 1 The absorption and emission spectra (△) of probe 1 and dye 2 in H2O/CH3CN (50%, v/v). Dashed line (a, c): 1; solid line (b, d): 2. | |
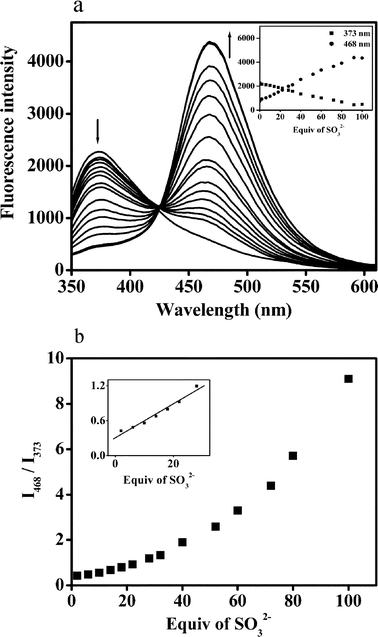 |
| Fig. 2 (a) The fluorescence emission spectra (λex = 310 nm) of probe 1 (10 μM) upon addition of sulfite (0–100 equiv.) in HEPES buffered (pH 7.4, 10 mM) H2O/CH3CN (50%, v/v). Inset: fluorescence intensity change based on the peak heights of 373 and 468 nm. (b) The fluorescence intensity ratiometric I468/I373 of probe 1 (10 μM) upon addition of sulfite (0–100 equiv.) in HEPES buffered (pH 7.4, 10 mM) H2O/CH3CN (50%, v/v). Inset: enlarged displays of the linear correlation between ratiometric I468/I373 and the low concentration of sulfite. | |
Inspection of Fig. 2b shows that the fluorescence intensity ratio (I468/I373) changes from 0.37 to 9.2 (25-fold) when the concentration of sulfite increases from 0 to 1000 μM with good linearity, R2 = 0.99208, occurring in the range of 0–320 μM. Specifically, the detection limit of probe 1 to sulfite was calculated to be 5 μM.
To evaluate the selectivity of probe 1, other typically encountered anions, including F−, Cl−, Br−, I−, HPO42−, SO42−, NO3−, AcO−, ClO4−, N3− and HCO3−, were added to the solution of probe 1 and the fluorescence response was measured (Fig. 3). Our results show that within this family of anions, sulfite is the only one that significantly causes the transformation of Probe 1 into phenol 2. The result demonstrates that probe 1 can be used to detect sulfite with good selectivity over other anions.
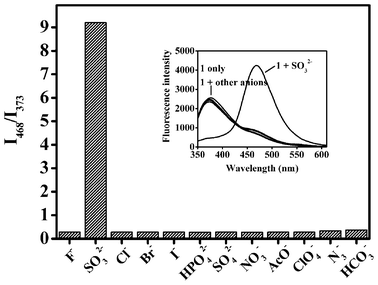 |
| Fig. 3 Anion selectivity in the fluorescence intensity ratiometric I468/I373 of probe 1. Inset: The fluorescence spectra (λex = 310 nm) of probe 1 (10 μM) upon addition of 100 equiv. of various anions in HEPES buffered (pH 7.4, 10 mM) H2O/CH3CN (50%, v/v). | |
In order to check the practical ability of the probe 1 to act as a selective fluorescent probe for sulfite, we conducted competitive experiments in the presence of sulfite at 100 equiv. mixed with F−, Cl−, Br−, I−, HPO42−, SO42−, NO3−, AcO−, ClO4−, N3− and HCO3− (200 equiv. each). The results, shown in Fig. 4a and Fig. 4b, indicate that the addition of these potential interfering anions, even at higher concentrations, does not adversely affect the fluorescence response of 1.
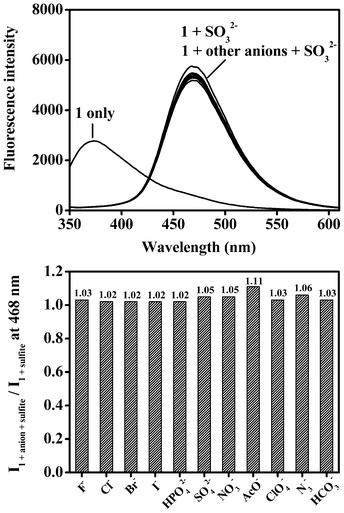 |
| Fig. 4 (a) The fluorescence spectra (λex = 310 nm) of probe 1 (10 μM) upon addition of sulfite (100 equiv.) and in the presence of interfering anions (200 equiv.) in HEPES buffered (pH 7.4, 10 mM) H2O/CH3CN (50%, v/v). (b) The fluorescence intensity ratio (I1+anion+sulfite/I1+sulfite) of probe 1 (10 μM) at 468 nm upon addition of sulfite (100 equiv.) and in the presence of interfering anions (200 equiv.) in HEPES buffered (pH 7.4, 10 mM) H2O/CH3CN (50%, v/v). | |
We further investigated the influence of pH on the fluorescence of probe 1 and the parent dye 2, respectively. As for probe 1, the fluorescence intensity ratio (I468/I373) was barely affected when the pH ranged from 4.0 to 10.0, while the decomposition of probe 1 was observed from the fluorescence change when the pH was over 10 (shown in Fig. 5a). The pH has a slight effect on the fluorescence of the parent dye 2, as shown in Fig. 5b. The data suggests that probe 1 can be used for the detection of sulfite at a wide pH range.
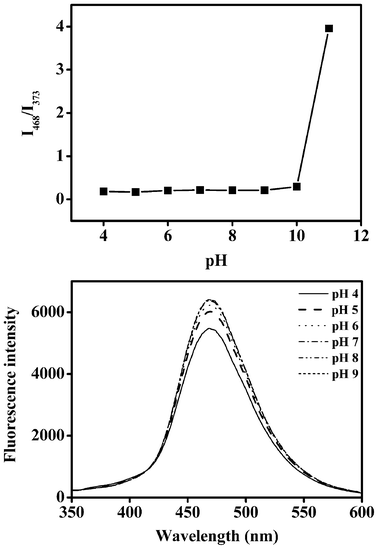 |
| Fig. 5 (a) The fluorescence intensity ratio (I468/I373) of probe 1 (10 μM) at various pH values in H2O/CH3CN (50%, v/v) solution. (b) Fluorescence spectra (λex = 310 nm) of compound 2 (10 μM) in H2O/CH3CN (50%, v/v) solution at different pH conditions. | |
Conclusions
In summary, we have developed a novel ESIPT-based, ratiometric fluorescent probe (1) which can detect sulfite qualitatively and quantitatively. It displays a 95 nm red-shift of fluorescence emission as a result of the sulfite-selective deprotection of levulinate. Moreover, this probe shows good selectivity and high sensitivity for sulfite over other anions in a wide working pH range. This work also suggests that O-functionalized 2-(benzothiazol-2-yl)phenols can potentially be used for the design of ratiometric fluorescent probes for other ions.
Acknowledgements
The research was supported by NSF of China (No. 21072235) and the Program for New Century Excellent Talents in University (No. NCET-10-0793).
References
- F. Moore, Contributions of the chemist to the pulp and paper industry, Ind. Eng. Chem., 1915, 7, 292–3 CrossRef CAS.
- C. Fernandez-Pereira, Application of ion chromatography to the determination of inorganic anions in foodstuffs, J. Chromatogr., 1992, 624, 457 CrossRef CAS.
- T. J. Cardwell and M. J. Christophersen, Determination of sulfur dioxide and ascorbic acid in beverages using a dual channel flow injection electrochemical detection system, Anal. Chim. Acta, 2000, 416, 105–10 CrossRef CAS.
- R. McFeeters, Use and removal of sulfite by conversion to sulfate in the preservation of salt-free cucumbers, J. Food Prot., 1998, 61, 885–90 CAS.
- M. Kuratli, M. Badertscher, B. Rusterholz and W. Simon, Bisulfite addition reaction as the basis for a hydrogensulfite bulk optode, Anal. Chem., 1993, 65, 3473–9 CrossRef CAS.
- K. R. B. Silva, I. M. Raimundo Jr, I. F. Gimenez and O. L. Alves, Optical sensor for sulfur dioxide determination in wines, J. Agric. Food Chem., 2006, 54, 8697–701 CrossRef CAS.
- A. N. Araujo, C. M. C. M. Couto, J. L. F. C. Lima and M. C. Montenegro, Determination of SO2 in wines using a flow injection analysis system with potentiometric detection, J. Agric. Food Chem., 1998, 46, 168–72 CrossRef CAS.
- L. Pizzoferrato, G. Di Lullo and E. Quattrucci, Determination of free, bound and total sulphites in foods by indirect photometry-HPLC, Food Chem., 1998, 63, 275–9 CrossRef CAS.
- D. Lowinsohn and M. Bertotti, Determination of sulphite in wine by coulometric titration, Food Addit. Contam., 2001, 18, 773–7 CAS.
- A. A. Ensafi and H. Karimi-Maleh, Ferrocenedicarboxylic acid modified multiwall carbon nanotubes paste electrode for voltammetric determination of sulfite, Int. J. Electrochem. Sci., 2010, 5, 392–406 CAS.
- V. J. Smith, Determination of sulfite using a sulfite oxidase enzyme electrode, Anal. Chem., 1987, 59, 2256–9 CrossRef CAS.
- S. Theisen, R. Hansch, L. Kothe, U. Leist and R. Galensa, A fast and sensitive HPLC method for sulfite analysis in food based on a plant sulfite oxidase biosensor, Biosens. Bioelectron., 2010, 26, 175–81 CrossRef CAS.
- Y. Huang, C. Zhang, X. Zhang and Z. Zhang, Chemiluminescence of sulfite based on auto-oxidation sensitized by rhodamine 6G, Anal. Chim. Acta, 1999, 391, 95–100 CrossRef CAS.
- D. A. Paulls and A. Townshend, Sensitized determination of sulfite using flow injection with chemiluminescent detection, Analyst, 1995, 120, 467–9 RSC.
- A. P. De Silva, H. Q. N. Gunaratne, T. Gunnlaugsson, A. J. M. Huxley, C. P. McCoy, J. T. Rademacher and T. E. Rice, Signaling recognition events with fluorescent sensors and switches, Chem. Rev., 1997, 97, 1515–66 CrossRef CAS.
- R. N. Martínez-Máñez and F. l. Sancenón, Fluorogenic and chromogenic chemosensors and reagents for anions, Chem. Rev., 2003, 103, 4419–76 CrossRef.
- M. E. Jun, B. Roy and K. H. Ahn, “Turn-on” fluorescent sensing with “reactive” probes., Chem. Commun., 2011, 47, 7583–601 RSC.
- M. G. Choi, J. Hwang, S. Eor and S. K. Chang, Chromogenic and fluorogenic signaling of sulfite by selective deprotection of resorufin levulinate, Org. Lett., 2010, 12, 5624–7 CrossRef CAS.
- L. Yuan, W. Lin and J. Song, Ratiometric fluorescent detection of intracellular hydroxyl radicals based on a hybrid coumarin-cyanine platform, Chem. Commun., 2010, 46, 7930–2 RSC.
- Y. Bao, B. Liu, H. Wang, J. Tian and R. Bai, A “naked eye” and ratiometric fluorescent chemosensor for rapid detection of F− based on combination of desilylation reaction and excited-state proton transfer, Chem. Commun., 2011, 47, 3957–9 RSC.
- J. F. Zhang, C. S. Lim, S. Bhuniya, B. R. Cho and J. S. Kim, A highly selective colorimetric and ratiometric two-photon fluorescent
probe for fluoride ion detection, Org. Lett., 2011, 13, 1190–3 CrossRef CAS.
- Z. Xu, K. H. Baek, H. N. Kim, J. Cui, X. Qian, D. R. Spring, I. Shin and J. Yoon, Zn2+-triggered amide tautomerization produces a highly Zn2+-selective, cell-permeable, and ratiometric fluorescent sensor, J. Am. Chem. Soc., 2009, 132, 601–10 CrossRef.
- W. Lin, L. Long, L. Yuan, Z. Cao, B. Chen and W. Tan, A ratiometric fluorescent probe for cysteine and homocysteine displaying a large emission shift, Org. Lett., 2008, 10, 5577–80 CrossRef CAS.
- J. Jiang, H. Jiang, W. Liu, X. Tang, X. Zhou and R. Liu, A colorimetric and ratiometric fluorescent probe for palladium, Org. Lett., 2011, 13, 4922–5 CrossRef CAS.
- X. F. Gu, C. H. Liu, Y. Zhu and Y. Z. C.; Zhu, A boron-dipyrromethene-based fluorescent probe for colorimetric and ratiometric detection of sulfite, J. Agric. Food Chem., 2011, 59, 11935–9 CrossRef CAS.
- R. Sarma, B. Nath, A. Ghritlahre and J. B. Baruah, Study on changes in optical properties of phenylbenzothiazole derivatives on metal ion binding, Spectrochim. Acta, Part A, 2010, 77, 126–9 CrossRef.
- C. C. Hsieh, C. M. Jiang and P. T. Chou, Recent experimental advances on excited-state intramolecular proton coupled electron transfer reaction, Acc. Chem. Res., 2010, 43, 1364–74 CrossRef CAS.
- R. Hu, J. Feng, D. Hu, S. Wang, S. Li, Y. Li and G. Yang, A rapid aqueous fluoride ion sensor with dual output modes, Angew. Chem., 2010, 122, 5035–8 CrossRef.
- M. Santra, B. Roy and K. H. Ahn, A “reactive” ratiometric fluorescent probe for mercury species, Org. Lett., 2011, 13, 3422–5 CrossRef CAS.
- Y. Xu, Q. Liu, B. Dou, B. Wright, J. Wang and Y. Pang, Zn2+ binding-enabled excited state intramolecular proton transfer: a step toward new near-infrared fluorescent probes for imaging applications, Adv. Healthcare Mater., 2012, 1, 485–92 CrossRef CAS.
- Q. Chu, D. Medvetz and Y. Pang, A polymeric colorimetric sensor with excited-state intramolecular proton transfer for anionic species, Chem. Mater., 2007, 19, 6421–9 CrossRef CAS.
- W. H. Chen, Y. Xing and Y. Pang, A highly selective pyrophosphate sensor based on ESIPT turn-on in water, Org. Lett., 2011, 13, 1190–3 CrossRef.
- T. I. Kim, H. J. Kang, G. Han, S. J. Chung and Y. Kim, A highly selective fluorescent ESIPT probe for the dual specificity phosphatase MKP-6., Chem. Commun., 2009, 39, 5895–7 RSC.
- M. Ono and I. Itoh, A new deprotection method for levulinyl protecting groups under neutral conditions, Chem. Lett., 1988, 585–8 Search PubMed.
- H. Y. Guo, J. C. Li and Y. L. Shang, A simple and efficient synthesis of 2-substituted benzothiazoles catalyzed by H2O2/HCl, Chin. Chem. Lett., 2009, 20, 1408–10 CrossRef CAS.
- D. P. Langlois and H. Wolff, Pseudo esters of levulinic acid, J. Am. Chem. Soc., 1948, 70, 2624–6 CrossRef CAS.
Footnote |
† Electronic Supplementary Information (ESI) available. See DOI: 10.1039/c2ra21471g |
|
This journal is © The Royal Society of Chemistry 2012 |
Click here to see how this site uses Cookies. View our privacy policy here.