DOI:
10.1039/C2RA21161K
(Paper)
RSC Adv., 2012,
2, 7724-7734
Formal total synthesis of (−)-exiguolide†
Received
11th June 2012
, Accepted 12th June 2012
First published on 22nd June 2012
Abstract
The formal total synthesis of (−)-exiguolide through the chiral-pool approach is described. The key reactions involved for formation of the macrocyclic core from two subunits are Julia–Kocienski olefination and Yamaguchi macrolactonization. The major methylene bis-tetrahydropyran fragment was achieved in a convergent manner from L-glutamic acid and L-aspartic acid involving the oxa-Michael reaction and an aldol-driven reductive etherification as key steps for the formation of a tetrahydropyran ring. The other sulfone subunit was prepared via an Evans aldol reaction.
Introduction
(−)-Exiguolide (1, Fig. 1) was isolated in 2006 by Ohta, Ikegami and co-workers from the marine sponge Geodia exigua Thiele (order Astrophorida, family Geodiidae).1 The structure, along with relative stereochemistry, has been determined by a combination of wide NMR study, conformational analysis based on nuclear Overhauser effect spectroscopy (NOESY) correlations and J-based configuration analysis. Structurally, it is a 20-membered macrolide with a methylene bis-tetrahydropyran (THP) subunit, five carbon–carbon double bonds and seven asymmetric carbons. The presence of the bispyran framework and the exocyclic enoate attached to one of the THP-rings are common motifs that can be found in marine antineoplastic agents, bryostatins.2 (−)-Exiguolide specifically inhibits the fertilization of sea urchin (Hemicentrotus pulcherrimus) gametes but not embryogenesis of the fertilized egg at higher concentrations. Based on these biological and structural features of (−)-1, an assumption has been made that (−)-exiguolide represents a structurally simplified analogue of the bryostatins.2b The limited supply and structural complexity of (−)-1 renders this molecule and its analogues important targets for chemical synthesis towards further evaluation of biological activities.
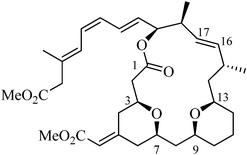 |
| Fig. 1 Structure of (−)-exiguolide (1). | |
In 2008, Lee and co-workers reported the total synthesis of (+)-exiguolide, an enantiomer of the natural product, using ring-closing metathesis, Prins cyclization, radical cyclization and Sonogashira coupling as the key steps.3 From this synthesis, they have determined the absolute stereochemistry of (−)-exiguolide. Later, three total syntheses of (−)-1 were published.4 In the year 2010, Fuwa and Sasaki reported the total synthesis of (−)-1via Julia–Kocienski olefination, reductive etherification, intramolecular oxa-conjugate addition and Suzuki–Miyayura coupling as the key reactions.4a In the same year, Roulland and co-workers accomplished (−)-1 using Yamaguchi macrolactonization, Trost's ruthenium-catalyzed ene–yne cross-coupling, reductive etherification and Sonogashira cross-coupling as steps.4b Recently, Scheidt and co-workers have described the enantioselective synthesis of (−)-exiguolide by dioxinone-directed Prins cyclizations to construct the THP-rings, as well as for macrocyclization and Leibeskind CuI-mediated coupling to stitch the side chain. Further, they have evaluated the ability of (−)-1 to inhibit cell growth on nine cancer cell lines and found significant antiproliferative activity against A549 lung cancer cells compared to others.4c Prior to this work, Fuwa et al. also elucidated the assessment of the growth inhibitory activity of synthetic (−)-exiguolide and its analogues against a panel of human cancer cell lines and observed that (−)-1 is an effective antiproliferative agent against the NCI-H460 human lung large carcinoma (log GI50 = −8.00) as well as the A549 human lung adenocarcinoma cell lines (log GI50 = −6.19).5 Notably (−)-1 has been found to have a 10 to 100-fold greater potency than bryostatin (log GI50 = −5.6 for NCI-H460, −5.4 for A549). They have also investigated the structure–activity relationships, which elucidated that the C5-methoxycarbonylmethylidene group and length of the side chain are important for biological activity.
Our interest in macrolides and THP-containing molecules6 coupled with the distinctive structure of (−)-exiguolide has encouraged us to attempt the synthesis of (−)-1. In this paper, we provide the full details of our work on the formal total synthesis of (−)-exiguolide.7 The retrosynthetic analysis of (−)-exiguolide is illustrated in Scheme 1. We envisioned that the side chain installation on iodo-macrocyclic core 2 would be at the final stage of the synthesis, which may be helpful for preparing new analogs. The macrocycle 2 could be realized from bis-THP subunit 3 and iodo-sulfone 4 through Julia–Kocienski olefination (C16–C17 double bond formation) followed by Yamaguchi macrolactonization. The synthesis of fragment 3 was planned in a convergent fashion from the THP-ketone 5 and aldehyde 6, utilizing an aldol-reaction followed by reductive etherification. Notably, THP-ketone 5 may in turn be obtained from L-glutamic acid using the oxa-Michael reaction, and aldehyde 6 could be derived from L-aspartic acid. The iodo-sulfone precursor 4 was envisaged from ethyl propiolate via iodo acrolein 7 by the Evans aldol approach.
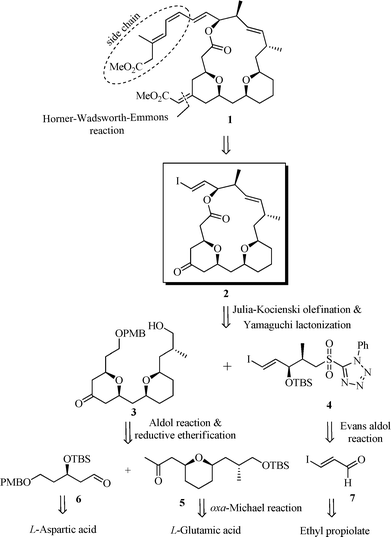 |
| Scheme 1 Retrosynthetic analysis of (−)-1. | |
Results and discussion
Synthesis of THP-ketone, C6–C16 fragment (5)
The synthesis of THP-ketone 5 was initiated by carrying out the preparation of the known tert-butyldiphenyl silyl protected (4S)-hydroxymethyl-4-butanolide 8 from L-glutamic acid using the literature method.8 In the first step, stereoselective methylation of 8 was carried out under LDA/MeI/−78 °C reaction conditions to get 9 in 78% yield. This reaction installed the C-15 methyl stereo center.9 The reductive opening of lactone 9 using lithium borohydride provided the 1,4-diol 10 in 99% yield. Subsequent protection of 1,4-diol 10 under 2,2-DMP/CSA reaction conditions afforded a seven-membered acetonide 11 in 90% yield. Then, the deprotection of the tert-butyldiphenyl silyl group of 11 was accomplished using tetrabutyl ammonium fluoride to give the alcohol 12 in 91% yield. In order to substitute the primary hydroxyl group with a four-carbon chain, a two-step procedure was planned. Accordingly, the alcohol 12 was tosylated (TsCl/Py, 96% yield) to 13, and subsequently tosylate 13 was subjected to a Schlosser copper-catalyzed Grignard coupling reaction with 3-butenyl magnesium bromide in the presence of dilithium tetrachloro cuprate, to give 14 in 88% yield.10 Synthesis of precursor 15 for the oxa-Michael reaction towards the pyran-ring formation was initially accomplished from 14 using a cross-metathesis reaction with methyl vinyl ketone in 56% yield.11 Alternatively, to get a better yield, olefin 14 was subjected to ozonolysis followed by a Wittig olefination (PPh3
CHCOMe), to provide α,β-unsaturated ketone 15 in 84% yield (over two steps). Hydrolysis of acetonide 15 and consequent oxa-Michael addition were accomplished through a p-TSA-catalyzed one-pot reaction to get pyran 16 in 89% yield.12 The NOE effect between H-9 and H-13 (2D NOESY) confirmed the relative syn-relationship of 2,6-disubstituted tetrahydropyran. The protection of the free-hydroxyl group of pyran 16 as tert-butyldimethyl silyl ether using TBSCl/imidazole gave the desired THP-ketone fragment 5 in 95% yield.
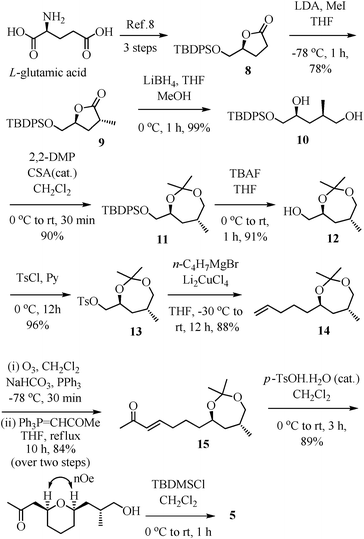 |
| Scheme 2 Synthesis of THP-ketone fragment 5. | |
Synthesis of aldehyde, C1–C5 fragment (6)
Synthesis of the C1–C5 subunit (Scheme 3) commenced with the preparation of epoxide 17 from commercially available L-aspartic acid using a known procedure.13 The conversion of the epoxide 17 to homoallylic alcohol 18 was accomplished through the copper(I)-catalyzed addition of vinyl magnesium bromide in 96% yield.14 Alcohol 18 was protected as tert-butyldimethyl silyl ether 19 in 95% yield (TBSCl/imidazole/DMF). It was then subjected to osmium-catalyzed dihydroxylation of the olefin followed by cleavage of the diol using sodium periodate to furnish aldehyde 6 in 84% yield.15
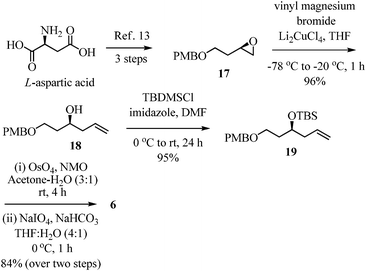 |
| Scheme 3 Synthesis of aldehyde fragment 6. | |
In an alternative approach to 6, a route via the opening of epoxide 17 with 1,3-dithiane was explored (Scheme 4). Thus, the treatment of epoxide 17 with 1,3-dithiane in the presence of n-butyl lithium gave the alcohol 20 in 82% yield. Protection of the hydroxyl group in 20 as tert-butyldimethyl silyl ether gave compound 21 in 86% yield (TBSOTf/2,6-lutidine/CH2Cl2) and subsequent deprotection of thioacetal using MeI/NaHCO3 in CH3CN/H2O afforded the aldehyde 6 in 83% yield.16
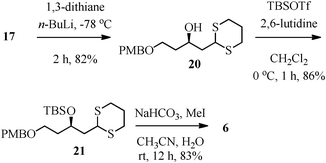 |
| Scheme 4 Alternative strategy for aldehyde 6. | |
Synthesis of sulfone fragment 4
As shown in Scheme 5, synthesis of the sulfone fragment 4 was based on known iodo acrolein 7, which was prepared from ethyl propiolate by a reported three-step sequence.17 For the required syn-propionate homologation, an Evans aldol reaction18 of 7 with the di-n-butylboron enolate of (R)-4-benzyl-3-propionyloxazolidin-2-one (22) was carried out to furnish the syn-product 23 in 74% yield. Exposure of 23 to TBSOTf/2,6-lutidine in CH2Cl2 gave the TBS-ether 24 in 90% yield. Compound 24 was treated with LiBH4 in THF–MeOH for the reductive removal of the auxiliary, to furnish alcohol 25. A Mitsunobu reaction19 of alcohol 25 with 1-phenyl-1H-tetrazole-5-thiol to thio tetrazole 26 (96% yield), followed by ammonium molybdate catalyzed oxidation20 of 26, completed the synthesis of sulfone 4 in 81% yield.
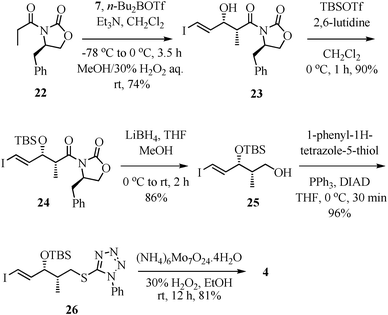 |
| Scheme 5 Synthesis of sulfone fragment 4. | |
Coupling of fragments
Having all the desired fragments 4, 5 and 6 in hand, we next proceeded to the synthesis of methylene bis-tetrahydropyran subunit 3 through an aldol driven reductive etherification strategy (Scheme 6). Hence, an aldol reaction of aldehyde 6 with the lithiated enolate of 5, derived using LDA, formed an aldol product as a inseparable mixture of diastereomers. This mixture was treated with Dess–Martin periodinane to give β-diketone 27 (71% yield over two steps), which entirely existed as the enol form (confirmed by 1H NMR). Deprotection of the TBS groups and cyclization was achieved in a one-pot operation by the reaction of 27 with HF (40% aq. solution) in CH3CN at room temperature, to furnish dihydropyranone 28 in 86% yield.21 Hydrogenation of 28 proceeded with the exclusive formation of cis-tetrahydropyranone 3 in 91% yield.22 The syn-relationship of both the THP rings was confirmed by 2D NOESY experiments.
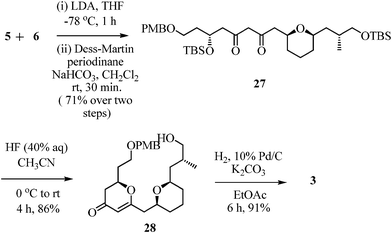 |
| Scheme 6 Synthesis of bis-THP subunit 3. | |
Next, the task was to couple the bis-THP subunit 3 with sulfone 4 by Julia–Kocienski reaction.23 Accordingly, oxidation of alcohol 3 with Dess–Martin periodinane gave the corresponding aldehyde (92% yield), but the following olefination of aldehyde with sulfone 4 using KHMDS as a base failed to give the desired product 29. Varying the reaction conditions (using different bases and solvent systems) also didn't help to get the desired product. These disappointing results prompted us to protect the keto-group present on the THP ring prior to the Julia–Kocienski reaction. Thus, exposure of 3 to trimethyl orthoformate/p-TSA·H2O (cat.) in MeOH afforded the ketal 30 in 82% yield. Now, the primary alcohol in 30 was oxidized to an aldehyde followed by Julia–Kocienski olefination with sulfone 4 using LiHMDS in THF
:
HMPA (4
:
1), affording 31 in 56% yield (80% brsm) with E-selectivity (>20
:
1) at the newly formed C16–C17 double bond. Unmasking the p-methoxybenzyl ether of 31 was achieved using 2,3-dichloro-5,6-dicyano-1,4-benzoquinone (DDQ) in CH2Cl2
:
pH 7 buffer (9
:
1) to provide 32 in 92% yield.24 To advance 32 towards a substrate suitable for the intramolecular Yamaguchi macrolactonization, the primary hydroxyl of 32 was oxidized to carboxylic acid under BAIB–TEMPO reaction25 conditions, followed by deprotection of the TBS-group with tetrabutylammonium fluoride (TBAF), to afford the hydroxy acid 33 in 91% yield (over two steps). Intramolecular macrolactonization of the hydroxy acid 33 under Yamaguchi reaction conditions26 gave the ketal protected macrolactone 34 in 63% yield. Further, hydrolysis of the ketal group of macrolide 34 with p-TSA·H2O (cat.) provided the desired macrocycle 2, whose spectral data matched exactly to that of the same compound prepared by Fuwa et al. for the synthesis of (−)-exiguolide (Scheme 7).4a
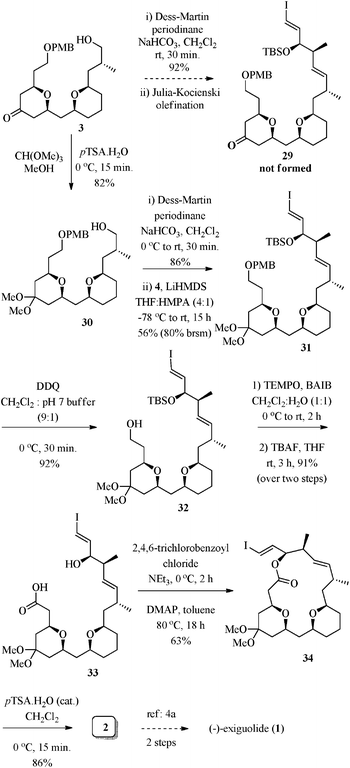 |
| Scheme 7 Synthesis of the macrocyclic core of (−)-exiguolide (1). | |
Conclusions
In summary, a flexible convergent synthesis of a macrocyclic precursor of (−)-exiguolide has been accomplished through a maximum-length linear sequence of 25 steps. The key reactions in the described route are the oxa-Michael reaction and an aldol driven reductive etherification for THP-ring formation, Julia–Kocienski olefination and Yamaguchi macrolactonization for macrocycle synthesis. We believe that the synthetic strategy developed is practical and scalable from inexpensive commercially available natural amino acids.
Experimental
General
Reactions were monitored by thin-layer chromatography carried out on silica plates (silica gel 60 F254, Merck) using UV-light and anisaldehyde or β-naphthol for visualization. Column chromatography was performed on silica gel (60–120 mesh) using hexanes, ethyl acetate, methanol and chloroform as the eluent. Evaporation of solvents was conducted under reduced pressure at temperatures less than 45 °C. IR spectra were recorded on a Perkin-Elmer 683, Nicolet Nexus 670 spectrometer. 1H NMR and 13C NMR spectra were recorded in CDCl3 solvent on a 300 MHz, 400 MHz and 500 MHz NMR spectrometer. Chemical shifts δ and coupling constants J are given in ppm (parts per million) and Hz (Hertz) respectively. Chemical shifts are reported relative to the residual solvent as an internal standard for 1H and 13C (CDCl3: δ 7.26 ppm for 1H and 77.0 ppm for 13C). Mass spectra were obtained on a Finnigan MAT1020B, micromass VG 70–70H or LC/MSD trapSL spectrometer operating at 70 eV using a direct inlet system.
(3R,5S)-5-((tert-Butyldiphenylsilyloxy)methyl)-3-methyldihydrofuran-2(3H)-one (9).
To a solution of diisopropyl amine (1.97 mL, 14 mmol) in dry THF (60 mL) at 0 °C was added n-BuLi (2.5 M in hexane, 5.6 mL, 14 mmol). After 30 min, the solution was cooled to −78 °C and a solution of 8 (5.0 g, 14 mmol) in dry THF (20 mL) was added dropwise. After 45 min at −78 °C, iodo methane (1.5 mL, 21 mmol) was added, and the solution was stirred for a further 1 h. The reaction was quenched with saturated aqueous NH4Cl (20 mL) and extracted with ether (2 × 50 mL). The combined organic layer was washed with brine (25 mL), dried over Na2SO4, filtered and evaporated to dryness under reduced pressure. The residue was purified by column chromatography (silica gel, hexanes
:
EtOAc = 81
:
15) to give 9 (4.0 g, 78%) as a colorless oil. Rf 0.40 (20% EtOAc in hexanes); IR (KBr): νmax 2931, 2858, 1760, 1191, 1109, 1024, 826, 703 cm−1; [α]20D = +32.0 (c = 1.20, CHCl3); 1H NMR (300 MHz, CDCl3): δ = 7.70–7.62 (m, 4H), 7.48–7.35 (m, 6H), 4.59–4.50 (m, 1H), 3.85 (dd, J = 11.3, 3.4 Hz, 1H), 3.66 (dd, J = 11.3, 3.2 Hz, 1H), 2.94–2.78 (m, 1H), 2.44 (ddd, J = 12.6, 9.6, 2.8 Hz, 1H), 2.07–1.89 (m, 1H), 1.29 (d, J = 7.3 Hz, 3H), 1.05 (s, 9H); 13C NMR (75 MHz, CDCl3): δ = 180.3, 135.4, 132.8, 132.3, 129.8, 127.8, 77.5, 65.4, 34.2, 32.1, 26.6, 19.1, 16.3; MS (ESI): m/z = 391 [M + Na]+ HRMS: calcd. for C22H32O3SiN (M + NH4)+ 386.2146; found 386.2160.
(2R,4S)-5-(tert-Butyldiphenylsilyloxy)-2-methylpentane-1,4-diol (10).
To a stirred solution of 9 (5.0 g, 13.6 mmol) in THF (65 mL) at 0 °C was sequentially added MeOH (0.65 mL, 16.32 mmol) and LiBH4 (414 mg, 19.02 mmol). After 1 h, the reaction was quenched with saturated aqueous NH4Cl (50 mL) and extracted with EtOAc (2 × 50 mL). The combined organic layer was dried over Na2SO4 and evaporated to dryness under reduced pressure. The residue was purified by flash column chromatography (silica gel, hexanes
:
EtOAc = 70
:
30) to give 10 (5.0 g, 99%) as a colorless oil. Rf 0.40 (30% EtOAc in hexanes); IR (KBr): νmax 3415, 2957, 2931, 2861, 1111, 758, 703 cm−1; [α]27D = +3.0 (c = 1.10, CHCl3); 1H NMR (300 MHz, CDCl3): δ 7.67–7.60 (m, 4H), 7.46–7.33 (m, 6H), 3.87 (m, 1H), 3.58 (dd, J = 10.0, 3.6 Hz, 1H), 3.55–3.39 (m, 3H), 2.81 (br s, 2H), 1.93–1.77 (m, 1H), 1.52–1.31 (m, 2H), 1.07 (s, 9H), 0.90 (d, J = 7.0 Hz, 3H); 13C NMR (75 MHz, CDCl3): δ = 135.4, 133.0, 129.8, 127.7, 69.2, 67.9, 67.5, 36.4, 32.2, 26.8, 19.1, 16.9; MS (ESI): m/z = 395 [M + Na]+; HRMS: calcd. for C22H32O3SiNa (M + Na)+ 395.2013; found 395.2029.
tert-Butyldiphenyl(((4S,6R)-2,2,6-trimethyl-1,3-dioxepan-4-yl)methoxy)silane (11).
To compound 10 (700 mg, 1.88 mmol) in CH2C12 (10 ml) was added camphorsulfonic acid (44 mg, 0.19 mmol) followed by 2,2-dimethoxypropane (0.58 mL, 4.70 mmol). After completion of the reaction (monitored by TLC), saturated NaHCO3 (10 mL) was added to the reaction mixture and extracted with CH2C12 (2 × 25 ml). The combined organic layer was dried over Na2SO4 and evaporated to dryness under reduced pressure. The residue was purified by column chromatography (silica gel, hexanes
:
EtOAc = 95
:
5) to give 11 (700 mg, 90%) as a colorless oil. Rf 0.50 (10% EtOAc in hexanes); IR (KBr): νmax 2934, 2861, 1760, 1219, 1110, 1080, 703 cm−1; [α]20D = −14.5 (c = 1.00, CHCl3); 1H NMR (300 MHz, CDCl3): δ = 7.70–7.60 (m, 4H), 7.42–7.30 (m, 6H), 3.93–3.81 (m, 2H), 3.59 (dd, J = 10.2, 6.6 Hz, 1H), 3.44 (dd, J = 10.2, 5.8 Hz, 1H), 3.36–3.28 (m, 1H), 1.86–1.74 (m, 1H), 1.54–1.36 (m, 2H), 1.28 (s, 3H), 1.22 (s, 3H), 1.04 (s, 9H), 1.02 (d, J = 7.0 Hz, 3H); 13C NMR (75 MHz, CDCl3): δ = 135.7, 135.6, 129.6, 127.6, 100.7, 68.8, 67.2, 65.9, 37.5, 31.0, 26.8, 25.0, 19.2, 17.1; MS (ESI): m/z = 435.1 [M + Na]+.
((4S,6R)-2,2,6-Trimethyl-1,3-dioxepan-4-yl)methanol (12).
A solution of 11 (1.20 g, 2.91 mmol) in THF (15 mL) was cooled to 0 °C and TBAF (4.37 mL, 4.37 mmol, 1.0 M solution in THF) was added dropwise. The resulting brown solution was stirred at room temperature for 2 h. The reaction was quenched with saturated aqueous NH4Cl (20 mL) and extracted with EtOAc (2 × 25 mL). The combined organic layer was washed with brine (15 mL), dried over Na2SO4 and evaporated to dryness under reduced pressure. The residue was purified by flash column chromatography (silica gel, hexanes
:
EtOAc = 80
:
20) to give 12 (460 mg, 91%) as a colorless oil. Rf 0.40 (30% EtOAc in hexane); IR (KBr): νmax 3367, 2927, 2873, 1375, 1219, 1045, 773 cm−1; [α]27D = +11.8 (c = 0.80, CHCl3); 1H NMR (300 MHz, CDCl3): δ = 4.00–3.90 (m, 1H), 3.87 (dd, J = 12.0, 1.5 Hz, 1H), 3.48–3.31 (m, 3H), 1.93–1.79 (m, 1H), 1.55–1.43 (m, 1H), 1.33 (s, 6H), 1.31–1.24 (m, 1H), 1.04 (d, J = 7.5 Hz, 3H); 13C NMR (75 MHz, CDCl3): δ = 108.8, 73.7, 69.6, 67.3, 36.9, 32.8, 26.8, 25.7, 16.7.
((4S,6R)-2,2,6-Trimethyl-1,3-dioxepan-4-yl)methyl 4-methylbenzenesulfonate (13).
Tosyl chloride (2.18 g, 11.4 mmol) was added to an ice cooled solution of 12 (1.81 g, 10.4 mmol) in dry pyridine (10 mL). The mixture was left to stand overnight in a refrigerator. It was then diluted with ice-water, and extracted with diethyl ether (2 × 25 ml). The combined organic layer was washed with water, dil. HCl, water, aq. NaHCO3 solution and brine, dried over Na2SO4, and concentrated to dryness under reduced pressure. The residue was purified by flash column chromatography (silica gel, hexanes
:
EtOAc = 90
:
10) to give 13 (3.6 g, 96%) as a colorless oil. Rf 0.50 (20% EtOAc in hexanes). IR (KBr): νmax 2983, 2922, 1362, 1218, 1177, 1063, 983, 812 cm−1; [α]20D = +3.18 (c = 0.88, CHCl3); 1H NMR (300 MHz, CDCl3): δ = 7.77 (d, J = 8.3 Hz, 2H), 7.32 (d, J = 8.3 Hz, 2H), 4.08–3.97 (m, 1H), 3.95–3.78 (m, 3H), 3.31 (dt, J = 12.0, 2.3 Hz, 1H), 2.46 (s, 3H), 1.88–1.76 (m, 1H), 1.47–1.34 (m, 2H), 1.24 (s, 6H), 1.01 (d, J = 7.5 Hz, 3H); 13C NMR (75 MHz, CDCl3): δ = 144.6, 133.0, 129.6, 127.7, 100.8, 72.4, 65.6, 65.5, 36.8, 30.5, 24.6, 24.5, 21.4, 16.8; MS (ESI): m/z = 351 [M + Na]+; HRMS: calcd. for C16H24O5SNa (M + Na)+ 351.1237; found 351.1236.
(4R,6R)-2,2,6-Trimethyl-4-(pent-4-enyl)-1,3-dioxepane (14).
A solution of 3-butenylmagnesium bromide (prepared from 4-bromo-1-butene (1.95 ml, 19.2 mmol) and Mg (634 mg, 26.4 mmol) in dry THF (25 mL) under nitrogen) was added dropwise to a stirred solution of 13 (3.15 g, 9.6 mmol) in dry THF (10 mL) at −78 °C. Subsequently, a solution of Li2CuCl4 (0.1 M in THF, 1 mL, 0.1 mmol) was added dropwise to the stirred mixture, which was left to stand overnight to gradually warm to room temperature. The reaction was quenched with saturated aqueous NH4Cl (20 mL) and extracted with diethyl ether (2 × 25 mL). The ether extract was washed with brine (20 mL), dried over Na2SO4 and concentrated in vacuo. The residue was purified by flash column chromatography (silica gel, hexanes
:
EtOAc = 95
:
5) to give olefin 14 (1.8 g, 88%) as a colorless oil. Rf 0.60 (10% EtOAc in hexanes); IR (KBr): νmax 2934, 1378, 1219, 1060 cm−1; [α]20D = −16.8 (c = 1.00, CHCl3); 1H NMR (300 MHz, CDCl3): δ = 5.88–5.72 (m, 1H), 5.04–4.91(m, 2H), 3.91 (dd, J = 12.0, 1.5 Hz, 1H), 3.87–3.77 (m, 1H), 3.36 (dt, J = 12.0, 3.0 Hz, 1H), 2.06 (dd, J = 14.3, 6.8 Hz, 2H), 1.85–1.73 (m, 1H), 1.59–1.35 (m, 6H), 1.34 (s, 3H), 1.32 (s, 3H), 1.04 (d, J = 6.8 Hz, 3H); 13C NMR (75 MHz, CDCl3): δ = 138.8, 114.3, 100.3, 67.8, 65.7, 41.7, 36.2, 33.8, 31.2, 25.7, 25.1, 17.1.
(E)-7-((4R,6R)-2,2,6-Trimethyl-1,3-dioxepan-4-yl)hept-3-en-2-one (15).
A solution of 14 (1.40 g, 6.54 mmol) and NaHCO3 (500 mg) in CH2Cl2 (65 mL) was treated at −78 °C with a stream of ozone until the starting material disappeared (monitored by TLC). The excess ozone was purged from the solution with a stream of oxygen and Ph3P (2.07 g, 7.85 mmol) was added. The reaction was allowed to warm slowly to room temperature, at which point it was diluted with water (25 mL). The subsequent mixture was extracted with CH2Cl2 (2 × 25 mL). The combined organic layers were washed with brine, dried over Na2SO4, filtered, and the filtrate was concentrated under reduced pressure to give the crude aldehyde, which was used for the next reaction without further purification.
To a solution of the above aldehyde in THF (30 mL) was added methyl-(triphenylphosphoranylidene)-2-propanone (3.12 g, 9.81 mmol) and the mixture was heated under reflux for 12 h. After cooling, the solvent was removed in vacuo, and the residue was purified by column chromatography (silica gel, hexanes
:
EtOAc = 90
:
10) to give enone 15 (1.4 g, 84%) as a colorless oil. Rf 0.50 (20% EtOAc in hexanes); IR (KBr): νmax 2931, 2864, 1712, 1673, 1358, 1218, 1072, 1039 cm−1; [α]26D = +2.6 (c = 1.05, CHCl3); 1H NMR (300 MHz, CDCl3): δ = 6.74 (dt, J = 15.8, 6.8 Hz, 1H), 6.03 (dt, J = 15.8, 1.4 Hz, 1H), 3.85 (dd, J = 12.0, 1.5 Hz, 1H), 3.82–3.72 (m, 1H), 3.31 (dt, J = 12.0, 2.3 Hz, 1H), 2.28–2.17 (m, 2H), 2.22 (s, 3H) 1.83–1.70 (m, 1H), 1.71–1.32 (m, 6H), 1.30 (s, 3H), 1.27 (s, 3H), 1.02 (d, J = 6.8 Hz, 3H); 13C NMR (75 MHz, CDCl3): δ = 198.6, 148.2, 131.3, 100.3, 67.6, 65.7, 41.7, 36.2, 32.4, 31.1, 26.8, 25.1, 25.0, 24.9, 17.1; MS (ESI): m/z = 277 [M + Na]+; HRMS: calcd. for C15H26O3Na (M + Na)+ 277.1774; found 277.1776.
1-((2S,6R)-6-((R)-3-Hydroxy-2-methylpropyl)tetrahydro-2H-pyran-2-yl)propan-2-one (16).
To compound 15 (450 mg, 1.77 mmol) in CH2C12 (10 ml) was added p-TSA·H2O (cat.). The mixture was stirred for 1 h at room temperature. After completion of the reaction (monitored by TLC), saturated NaHCO3 (10 mL) was added to the reaction mixture and extracted with CH2C12 (2 × 25 ml). The combined organic layer was dried over Na2SO4 and evaporated to dryness under reduced pressure. The residue was purified by column chromatography (silica gel, hexanes
:
EtOAc = 70
:
30) to give 16 (337 mg, 89%) as a colorless oil. Rf 0.40 (50% EtOAc in hexanes); IR (KBr): νmax 3433, 2927, 1709, 1361, 1195, 1037 cm−1; [α]28D = −3.3 (c = 0.90, CHCl3); 1H NMR (500 MHz, CDCl3): δ = 3.85–3.74 (m, 1H), 3.57–3.48 (m, 1H), 3.46 (dd, J = 10.5, 5.3 Hz, 1H), 3.34 (dd, J = 10.5, 6.8 Hz, 1H), 2.67 (dd, J = 15.8, 7.5 Hz, 1H), 2.39 (dd, J = 15.8, 5.3 Hz 1H), 2.15 (s, 3H), 1.93–1.78 (m, 2H), 1.65–1.37 (m, 5H), 1.31–1.12 (m, 3H), 0.9 (d, J = 6.8 Hz, 3H); 13C NMR (75 MHz, CDCl3): δ = 207.3, 75.5, 73.9, 67.6, 49.8, 40.1, 32.0, 31.1, 31.0, 30.9, 23.3, 17.2; MS (ESI): m/z = 237 [M + Na]+; HRMS: calcd. for C12H22O3Na (M + Na)+ 237.1461; found 237.1463.
1-((2S,6R)-6-((R)-3-(tert-Butyldimethylsilyloxy)-2-methylpropyl)tetrahydro-2H-pyran-2-yl)propan-2-one (5).
To a stirred solution of alcohol 16 (850 mg, 3.97 mmol) in CH2Cl2 (10 mL) was added imidazole (405 mg, 5.95 mmol) followed by tert-butyldimethylsilyl chloride (718 mg, 4.77 mmol) at 0 °C. The reaction mixture was allowed to warm to room temperature and stirred for 2 h. After completion of the reaction, the reaction mixture was diluted by the addition of water (20 mL) and the aqueous phase was extracted with CH2Cl2 (2 × 25 mL). The combined organic layer was washed with brine (20 mL), dried over Na2SO4 and the organic solvent evaporated under reduced pressure. The crude residue was purified by flash column chromatography (silica gel, hexanes
:
EtOAc = 95
:
5) to give 5 (1.24 g, 95%) as a colorless oil. Rf 0.30 (5% EtOAc in hexanes); IR (KBr): νmax 2930, 2856, 1717, 1360, 1252, 1086, 839, 775 cm−1; [α]27D = −3.2 (c = 1.20, CHCl3); 1H NMR (300 MHz, CDCl3): δ = 3.77–3.65 (m, 1H), 3.44–3.30 (m, 3H), 2.64 (dd, J = 15.1, 7.8 Hz, 1H), 2.35 (dd, J = 15.1, 4.8 Hz, 1H), 2.15 (s, 3H), 1.88–1.65 (m, 2H), 1.64–1.49 (m, 2H), 1.47–1.37 (m, 1H), 1.33–1.06 (m, 4H), 0.89 (s, 9H), 0.87 (d, J = 6.5 Hz, 3H), 0.03 (s, 6H); 13C NMR (75 MHz, CDCl3): δ = 207.7, 76.4, 74.3, 67.7, 50.3, 39.8, 32.3, 31.9, 31.5, 31.4, 31.0, 25.9, 23.5, 17.5, −5.4; MS (ESI): m/z = 351 [M + Na]+; HRMS: calcd. for C18H36O3SiNa (M + Na)+ 351.2326; found 351.2325.
(S)-1-(4-Methoxybenzyloxy)hex-5-en-3-ol (18)15.
To a stirred solution of epoxide 17 (2.40 g, 11.54 mmol) in dry THF (40 mL) was added Li2CuCl4 (5.80 mL of a 0.1M solution in THF, 0.58 mmol) followed by vinyl magnesium bromide (23.08 mL of a 1M solution in THF, 23.08 mmol) at −78 °C. The solution was allowed to warm to −20 °C and stirred at this temperature for 1 h. The reaction was quenched with saturated aqueous NH4Cl (20 mL) and extracted with ether (2 × 50 mL). The combined organic layer was dried over Na2SO4 and evaporated to dryness under reduced pressure. The residue was purified by flash column chromatography (silica gel, hexanes
:
EtOAc = 80
:
20) to give 18 (2.61 g, 96%) as a colorless oil. Rf 0.40 (30% EtOAc in hexanes); [α]20D = −4.6 (c = 1.00, CHCl3); 1H NMR (500 MHz, CDCl3): δ = 7.22 (d, J = 8.7 Hz, 2H), 6.85 (d, J = 8.7 Hz, 2H), 5.86–5.77 (m, 1H), 5.11–5.05 (m, 2H), 4.44 (s, 2H), 3.86–3.81 (m, 1H), 3.80 (s, 3H), 3.70–3.64 (m, 1H), 3.62–3.56 (m, 1H), 2.76 (br s, 1H), 2.22 (t, J = 6.8 Hz, 2H), 1.76–1.70 (m, 2H); 13C NMR (75 MHz, CDCl3): δ = 159.1, 134.8, 129.9, 129.2, 117.4, 113.7, 72.8, 70.3, 68.5, 55.2, 41.8, 35.7; MS (ESI): m/z = 259.2 [M + Na]+.
(S)-tert-Butyl(1-(4-methoxybenzyloxy)hex-5-en-3-yloxy)dimethylsilane (19)15.
To a stirred solution of alcohol 18 (1.0 g, 4.24 mmol) in dry DMF (5 mL) was added imidazole (432 mg, 6.36 mmol) followed by tert-butyldimethylsilyl chloride (766 mg, 5.08 mmol) at 0 °C. The reaction mixture was allowed to warm to room temperature and stirred for 12 h. After completion of the reaction the reaction mixture was diluted by the addition of ice cold water (20 mL) and the aqueous phase was extracted with ether (2 × 25 mL). The combined organic layer was washed with brine (20 mL), dried over Na2SO4 and the organic solvent evaporated under reduced pressure. The crude residue was purified by flash column chromatography (silica gel, hexanes
:
EtOAc = 95
:
05) to give 19 (1.51 g, 95%) as a colorless oil. Rf 0.70 (20% EtOAc in hexanes); [α]20D = +15.1 (c = 1.00, CHCl3); 1H NMR (300 MHz, CDCl3): δ 7.21 (d, J = 8.5 Hz, 2H), 6.83 (d, J = 8.5 Hz, 2H), 5.86–5.71 (m, 1H), 5.07–4.96 (m, 2H), 4.42 (d, J = 11.5 Hz, 1H), 4.35 (d, J = 11.5 Hz, 1H), 3.94–3.84 (m, 1H), 3.80 (s, 3H), 3.51–3.42 (m, 1H), 2.28–2.13 (m, 2H), 1.81–1.59 (m, 2H), 0.88 (s, 9H), 0.05 (s, 3H), 0.04 (s, 3H); 13C NMR (75 MHz, CDCl3): δ 159.0, 134.8, 130.5, 129.1, 116.8, 113.6, 72.5, 68.8, 66.6, 55.0, 42.2, 36.6, 25.8, 17.9, −4.4, −4.7; MS (ESI): m/z = 373.3 [M + Na]+.
(R)-3-(tert-Butyldimethylsilyloxy)-5-(4-methoxybenzyloxy)pentanal (6)15.
To a solution of silyl ether 19 (1.16 g, 3.31 mmol) in a 30 mL mixture of acetone–water (4
:
1) was added OsO4 (4.2 mL, 1 mg/1 mL toluene solution, 0.016 mmol) and NMO (465 mg, 3.98 mmol) at 25 °C and the mixture was stirred for 12 h. The solvent was evaporated and the residue was extracted with ethyl acetate (30 mL). The organic layers were washed with brine (20 mL), dried over Na2SO4 and concentrated in vacuo, affording an oil which was purified by flash column chromatography (silica gel, hexanes
:
EtOAc = 50
:
50) to give diol (1.2 g) as a colorless oil.
To a solution of the above diol (1.20 g, 3.12 mmol) in a 30 mL mixture of THF–H2O (4
:
1) was added NaHCO3 (446 mg, 5.30 mmol) and NaIO4 (2.0 g, 9.4 mmol). After stirring at 0 °C for 30 min, the solid was removed by filtration and the filtrate was extracted with ethyl acetate (2 × 30 mL). The combined organic layers were washed with brine (20 mL), dried with Na2SO4 and concentrated in vacuo. The crude aldehyde was purified by flash chromatography (10% ethyl acetate in hexanes) to give aldehyde 6 (0.98 g, 84%) as a colorless oil. Rf 0.60 (20% EtOAc in hexanes); IR (KBr): νmax 2954, 2931, 2857, 1726, 1613, 1513, 1465, 1250, 1098, 1037, 836, 777 cm−1; [α]20D = +5.2 (c = 1.00, CHCl3); 1H NMR (300 MHz, CDCl3): δ = 9.79 (s, 1H), 7.24 (d, J = 8.5 Hz, 2H), 6.88 (d, J = 8.5 Hz, 2H), 4.43 (d, J = 11.2 Hz, 1H), 4.38 (d, J = 11.3 Hz, 1H), 4.37–4.32 (m, 1H), 3.80 (s, 3H), 3.51 (t, J = 6.2 Hz, 2H), 2.59–2.51 (m, 2H), 1.9–1.76 (m, 2H), 0.86 (s, 9H), 0.07 (s, 3H), 0.05 (s, 3H); 13C NMR (75 MHz, CDCl3): δ = 202.1, 159.1, 130.3, 129.2, 113.7, 72.6, 65.9, 65.6, 55.2, 51.0, 37.5, 25.7, 17.9, −4.6, −4.7; MS (ESI): m/z = 375.2 [M + Na]+.
(R)-1-(1,3-Dithian-2-yl)-4-(4-methoxybenzyloxy)butan-2-ol (20).
To a stirred solution of 1,3-dithiane (434 mg, 3.6 mmol) in THF (20 mL) at −20 °C was added n-BuLi (1.6 M in hexanes, 2.25 mL, 3.6 mmol) dropwise. After 30 min, epoxide 17 (0.5 g, 2.40 mmol) in THF (5 mL) was added dropwise. After being stirred for 1 h at the same temperature, the reaction was quenched with saturated aqueous NH4Cl (15 mL) and the aqueous phase was extracted with ether (2 × 25 mL). The combined organic layer was washed with brine (20 mL), dried over Na2SO4 and the organic solvent evaporated under reduced pressure. The crude residue was purified by flash column chromatography (silica gel, hexanes
:
EtOAc = 80
:
20) to give 20 (645 mg, 82%) as a colorless oil. Rf 0.40 (20% EtOAc in hexanes); IR (KBr): νmax 3403, 2921, 2851, 1612, 1511, 1243, 1220, 772 cm−1; [α]20D = −20.7 (c = 0.88, CHCl3); 1H NMR (500 MHz, CDCl3): δ = 7.24 (d, J = 9.0 Hz, 2H), 6.87 (d, J = 9.0 Hz, 2H), 4.45 (s, 2H), 4.29 (dd, J = 9.0, 5.0 Hz, 1H), 4.17–4.09 (m, 1H), 3.80 (s, 3H), 3.72–3.67 (m, 1H), 3.66–3.60 (m, 1H), 3.08 (br s, 1H), 2.96–2.79 (m, 4H), 2.16–2.09 (m, 1H), 1.96–1.70 (m, 5H); 13C NMR (75 MHz, CDCl3): δ = 159.1, 129.7, 129.2, 113.7, 72.8, 68.4, 67.6, 55.1, 43.8, 42.7, 36.3, 30.3, 29.9, 25.8; MS (ESI): m/z = 329 [M + H]+; HRMS: calcd. for C16H25O3S2 (M + H)+ 329.1240; found 329.1230.
(R)-(1-(1,3-Dithian-2-yl)-4-(4-methoxybenzyloxy)butan-2-yloxy)(tert-butyl)dimethylsilane (21).
To a cooled (0 °C) solution of 20 (330 mg, 1.0 mmol) in CH2Cl2 (10 mL) was added 2,6-lutidine (0.23, 2.0 mmol) and TBSOTf (0.26 mL, 1.1 mmol). The mixture was stirred at the same temperature for 30 min and was then diluted with CH2Cl2 (10 mL). The organic phase was washed sequentially with a saturated aqueous solution of NaHCO3 (20 mL) and brine (15 mL), dried over Na2SO4 and evaporated to dryness. The residue was purified by flash chromatography (silica gel, 5% EtOAc in hexanes) to give the silyl ether 21 (380 mg, 86% yield) as a colorless oil. Rf 0.50 (10% EtOAc in hexanes); IR (KBr): νmax 2950, 2930, 2855, 1612, 1512, 1200, 1093, 833, 774 cm−1; [α]20D = −9.2 (c = 1.00, CHCl3); 1H NMR (300 MHz, CDCl3): δ = 7.27 (d, J = 8.3 Hz, 2H), 6.87 (d, J = 8.3 Hz, 2H), 4.42 (s, 2H), 4.14–4.05 (m, 1H), 3.81 (s, 3H), 3.50 (t, J = 6.8 Hz, 2H), 2.89–2.77 (m, 4H), 2.16–2.05 (m, 1H), 1.94–1.75 (m, 5H), 0.88 (s, 9H), 0.10 (s, 3H), 0.06 (s, 3H); 13C NMR (75 MHz, CDCl3): δ = 158.9, 130.4, 129.1, 113.6, 72.5, 66.3, 66.1, 55.1, 43.7, 42.8, 37.2, 30.3, 29.9, 25.8, 17.9, −4.6, −4.7; MS (ESI): m/z = 443.2 [M + H]+.
(R)-3-(tert-Butyldimethylsilyloxy)-5-(4-methoxybenzyloxy)pentanal (6).
To a stirred solution of 21 (1.2 g, 2.71 mmol) in CH3CN (27 mL) and H2O (7 mL) at room temperature was added NaHCO3 (1.14 g, 13.5 mmol) and MeI (3.4 mL, 54.2 mmol). The reaction mixture was stirred at 40 °C for 12 h. The resulting mixture was extracted with EtOAc (2 × 30 mL). The combined organic extracts were washed with saturated NaHCO3 (10 mL), washed with brine (10 mL), dried with Na2SO4 and concentrated in vacuo. The crude aldehyde was purified by flash chromatography (10% ethyl acetate in hexanes) to give aldehyde 6 (790 mg, 83%) as a colorless oil.
(R)-4-Benzyl-3-((2R,3R,E)-3-hydroxy-5-iodo-2-methylpent-4-enoyl)oxazolidin-2-one (23).
To a stirred solution of (R)-4-benzyl-3-propionyl oxazolidin-2-one 22 (1.0 g, 4.29 mmol) in 10 mL CH2Cl2 at −78 °C was added n-Bu2BOTf (5.15 mL of a 1M solution in CH2Cl2, 5.15 mmol) followed by NEt3 (0.84 mL, 6.0 mmol). The resulting solution was stirred at −78 °C for 1 h, then at 0 °C for 30 min. After cooling back to −78 °C, the aldehyde 7 (1.55 g, 8.58 mmol) in 10 mL CH2Cl2 solution was added dropwise. After stirring at −78 °C for 1 h, then at 0 °C for 1 h, the reaction was quenched with pH 7 buffer (5 mL), MeOH (17 mL) and 30% H2O2 (5 mL). The ice bath was removed, and stirring continued for 1 h. The resulting solution was concentrated, then extracted with CH2Cl2 (2 × 25 mL). The combined organic layers were washed with 20 mL saturated aq. NaHCO3 solution, dried over Na2SO4, filtered and concentrated. Purification by flash column chromatography (20% EtOAc–hexanes) afforded the aldol adduct 23 (1.32 g, 74%) as a viscous oil. Rf 0.40 (20% EtOAc in hexanes); IR (KBr): νmax 3470, 2922, 1776, 1692, 1385, 1215, 1110, 1011, 771, 703 cm−1; [α]20D = −31.4 (c = 1.16, CHCl3); 1H NMR (300 MHz, CDCl3): δ = 7.39–7.28 (m, 3H), 7.23–7.18 (m, 2H), 6.55 (dd, J = 15.1, 5.2 Hz, 1H), 6.46 (d, J = 15.1 Hz, 1H), 4.77–4.65 (m, 1H), 4.52–4.45 (m, 1H), 4.26 (dd, J = 9.0, 7.5 Hz, 1H), 4.21 (dd, J = 9.0, 3.0 Hz, 1H), 3.89–3.80 (m, 1H), 3.24 (dd, J = 13.6, 3.0 Hz, 1H), 3.06 (d, J = 3.0 Hz, 1H), 2.80 (dd, J = 13.6, 9.8 Hz, 1H), 1.26 (d, J = 7.5 Hz, 3H); 13C NMR (75 MHz, CDCl3): δ = 175.9, 152.9, 144.6, 134.8, 129.3, 128.9, 127.4, 78.6, 74.1, 66.2, 55.0, 41.9, 37.7, 11.1; MS (ESI): m/z = 438 [M + Na]+; HRMS: calcd. for C16H18INO4Na (M + Na)+ 438.0173; found 438.0141.
(R)-4-Benzyl-3-((2R,3R,E)-3-(tert-butyldimethylsilyloxy)-5-iodo-2-methylpent-4-enoyl)oxazolidin-2-one (24).
To a cooled (0 °C) solution of 23 (2.73 g, 6.58 mmol) in CH2Cl2 (25 mL) was added 2,6-lutidine (1.53 mL, 13.16 mmol) and TBSOTf (1.66 mL, 7.24 mmol). The mixture was stirred at 0 °C for 30 min and was then diluted with CH2Cl2 (15 mL). The organic phase was washed sequentially with a saturated aqueous solution of NaHCO3 (20 mL) and brine (15 mL), dried over Na2SO4 and evaporated to dryness. The residue was purified by flash chromatography (silica gel, 5% EtOAc in hexanes) to give 24 (3.13 g, 90% yield) as a colorless oil. Rf 0.50 (10% EtOAc in hexanes); IR (KBr): νmax 2929, 2856, 1782, 1698, 1381, 1212, 1108, 1072, 1015, 838, 776, 701 cm−1; [α]20D = −44.2 (c = 1.00, CHCl3); 1H NMR (300 MHz, CDCl3): δ = 7.39–7.28 (m, 3H), 7.24–7.18 (m, 2H), 6.59 (dd, J = 14.5, 6.8 Hz, 1H), 6.28 (d, J = 14.5 Hz, 1H), 4.68–4.55 (m, 1H), 4.29 (t, J = 6.8 Hz, 1H), 4.22–4.15 (m, 2H), 4.02–3.91 (m, 1H), 3.26 (dd, J = 13.4, 3.4 Hz, 1H), 2.78 (dd, J = 13.4, 9.6 Hz, 1H), 1.22 (d, J = 6.8 Hz, 3H), 0.88 (s, 9H), 0.02 (s, 3H), 0.01 (s, 3H); 13C NMR (75 MHz, CDCl3): δ = 174.0, 153.0, 146.7, 135.1, 129.3, 128.8, 127.3, 77.6, 76.5, 66.0, 55.5, 43.7, 37.7, 25.6, 18.0, 12.5, −4.5, −5.2; MS (ESI): m/z = 552.1 [M + Na]+; HRMS: calcd. for C22H32INO4SiNa (M + Na)+ 552.1037; found 552.0990.
(2S,3R,E)-3-(tert-Butyldimethylsilyloxy)-5-iodo-2-methylpent-4-en-1-ol (25).
To a stirred solution of 24 (1.52 g, 2.87 mmol) in THF (25 mL) at 0 °C was sequentially added MeOH (0.14 mL, 3.58 mmol) and LiBH4 (94 mg, 4.31 mmol). After 2h, the reaction was quenched with saturated aqueous NH4Cl (50 mL) and extracted with EtOAc (2 × 30 mL). The combined organic layer was dried over Na2SO4 and evaporated to dryness under reduced pressure. The residue was purified by flash column chromatography (silica gel, hexanes
:
EtOAc = 70
:
30) to give 25 (878 mg, 86%) as a colorless oil. Rf 0.40 (40% EtOAc in hexanes); IR (KBr): νmax 3378, 2930, 2858, 1606, 1466, 1362, 1255, 1170, 1117, 1038, 837, 776 cm−1; [α]20D = +38.8 (c = 1.12, CHCl3); 1H NMR (500 MHz, CDCl3): δ = 6.56 (dd, J = 14.3, 5.5 Hz, 1H), 6.25 (d, J = 14.3 Hz, 1H), 4.23 (t, J = 5.5 Hz, 1H), 3.59 (dd, J = 10.0, 8.8 Hz, 1H), 3.50–3.41 (m, 1H), 2.52 (br s, 1H), 1.91–1.82 (m, 1H), 0.88 (s, 9H), 0.81 (d, J = 6.6 Hz, 3H), 0.05 (s, 3H), 0.02 (s, 3H); 13C NMR (75 MHz, CDCl3): δ = 146.0, 77.5, 76.8, 65.0, 40.8, 25.7, 18.0, 11.7, −4.6, −5.2; MS (ESI): m/z = 379 [M + Na]+.
5-((2R,3R,E)-3-(tert-Butyldimethylsilyloxy)-5-iodo-2-methylpent-4-enylthio)-1-phenyl-1H-tetrazole (26)
DIAD (0.4 mL, 2.01 mmol) was slowly added at 0 °C to a solution of alcohol 25 (550 mg, 1.55 mmol), PPh3 (488 mg, 1.85 mmol) and 1-phenyl-1H-tetrazole- 5-thiol (330 mg, 1.85 mmol) in THF (20 mL). The mixture was stirred at room temperature for 1 h before the solvent was evaporated. The residue was purified by flash column chromatography (silica gel, hexanes
:
EtOAc = 90
:
10) to give 26 (768 mg, 96%) as a colorless oil. Rf 0.40 (10% EtOAc in hexanes); IR (KBr): νmax 2954, 2931, 2857, 1599, 1500, 1465, 1409, 1386, 1252, 1087, 1022, 838, 777 cm−1; [α]20D = +10.7 (c = 1.00, CHCl3); 1H NMR (300 MHz, CDCl3): δ = 7.63–7.50 (m, 5H), 6.54 (dd, J = 14.5, 6.2 Hz, 1H), 6.28 (dd, J = 14.5, 1.3 Hz, 1H), 4.21 (dddd, J = 6.0, 4.7, 3.6, 1.3 Hz, 1H), 3.48 (dd, J = 13.0, 6.6 Hz, 1H), 3.23 (dd, J = 13.0, 7.3 Hz, 1H), 2.14–2.05 (m, 1H), 1.02 (d, J = 6.9 Hz, 3H), 0.89 (s, 9H), 0.04 (s, 3H), 0.02 (s, 3H); 13C NMR (75 MHz, CDCl3): δ = 154.3, 146.2, 133.6, 130.0, 129.7, 123.7, 77.4, 76.9, 39.0, 36.2, 25.7, 18.0, 13.8, −4.4, −5.0; MS (ESI): m/z = 539 [M + Na]+; HRMS: calcd. for C19H30IN4OSSi (M + H)+ 517.0949; found 517.0904.
5-((2R,3R,E)-3-(tert-Butyldimethylsilyloxy)-5-iodo-2-methylpent-4-enylsulfonyl)-1-phenyl-1H-tetrazole (4).
A solution of (NH4)6Mo7O24·4H2O (0.868 g, 0.7 mmol) in aq. H2O2 (30% w/w, 3.5 mL) was stirred at 0 °C for 15 min before it was added to a solution of 26 (0.725 g, 1.40 mmol) in EtOH (14 mL) at 0 °C, and the mixture was allowed to warm up to room temperature. After 12 h, the reaction was diluted with water (20 mL) and the resulting mixture was extracted with EtOAc (2 × 25 mL). The organic layer was washed with saturated aqueous Na2S2O3 (20 mL) and brine (20 mL), then dried over Na2SO4. Concentration of the solvent in vacuo afforded a residue, which was purified by flash column chromatography (hexane–EtOAc, 90
:
10) to give 4 (621 mg, 81%) as a viscous oil. Rf 0.40 (10% EtOAc in hexanes); IR (KBr): νmax 2955, 2930, 2857, 1734, 1607, 1502, 1464, 1341, 1268, 1153, 1100, 761 cm−1; [α]20D = +6.3 (c = 1.00, CHCl3); 1H NMR (500 MHz, CDCl3): δ = 7.72–7.66 (m, 2H), 7.64–7.58 (m, 3H), 6.51 (dd, J = 14.0, 6.0 Hz, 1H), 6.36 (dd, J = 14.0, 6.0 Hz, 1H), 4.28 (t, J = 5.0 Hz, 1H), 4.02 (dd, J = 14.0, 4.0 Hz, 1H), 3.48 (dd, J = 14.0, 8.0 Hz, 1H), 2.52–2.41 (m, 1H), 1.15 (d, J = 7.0 Hz, 3H), 0.90 (s, 9H), 0.08 (s, 3H), 0.05 (s, 3H); 13C NMR (75 MHz, CDCl3): δ 153.8, 144.7, 132.9, 131.4, 129.6, 125.0, 78.6, 76.7, 57.8, 34.4, 25.7, 18.1, 14.7, −4.5, −5.0; MS (ESI): m/z = 571 [M + Na]+; HRMS: calcd. for C19H29IN4O3SSiNa (M + Na)+ 571.0667; found 571.0643.
(R)-6-(tert-Butyldimethylsilyloxy)-1-((2S,6S)-6-((R)-3-(tert-butyldimethylsilyloxy)-2-methylpropyl)tetrahydro-2H-pyran-2-yl)-8-(4-methoxybenzyloxy)octane-2,4-dione (27).
To a solution of diisopropyl amine (0.6 mL, 4.20 mmol) in dry THF (15 mL) at 0 °C was added n-BuLi (1.6 M in hexane, 2.5 mL, 4.02 mmol). After 30 min, the solution was cooled to −78 °C and a solution of ketone 5 (1.2 g, 3.65 mmol) in dry THF (5 mL) was added dropwise. After 1 h at −78 °C, a solution of aldehyde 6 (1.42 g, 4.02 mmol) in dry THF (10 mL) was added dropwise. The resulting mixture was stirred for 2 h at −78 °C, and was then quenched with saturated NH4Cl (15 mL). The aqueous phase was extracted with Et2O (3 × 25 mL). The combined organic phases were washed with brine, dried over Na2SO4, and concentrated in vacuo. The residue was purified by column chromatography (hexanes
:
EtOAc, 80
:
20) to give the aldol adduct (1.8 g, 74%) as a mixture of diastereomers.
To a stirred solution of the above aldol adduct (1.8 g, 2.64 mmol) in dry CH2Cl2 (20 mL) at room temperature was added NaHCO3 (665 mg, 7.92 mmol) and Dess–Martin periodinane (1.68 g, 3.96 mmol) in one portion. The mixture was stirred for 1 h at room temperature and quenched with saturated aqueous Na2S2O3 (10 mL). The layers were separated and the aqueous phase was extracted with ether (2 × 25 mL). The combined organic layers were washed with brine (10 mL), dried with Na2SO4 and concentrated in vacuo. The residue was purified by column chromatography (10% ethyl acetate in hexanes) to give diketone 27 (1.7 g, 96%) as a colorless oil. Rf 0.50 (20% EtOAc in hexanes); IR (KBr): νmax 2928, 2855, 1729, 1612, 1513, 1462, 1248, 1089, 834, 774 cm−1; [α]28D = −7.7 (c = 1.05, CHCl3); 1H NMR (300 MHz, CDCl3): δ = 7.20 (d, J = 9.0 Hz, 2H), 6.82 (d, J = 9.0 Hz, 2H), 5.55 (s, 1H), 4.41 (d, J = 11.3 Hz, 1H) 4.35 (d, J = 11.3 Hz, 1H), 4.30–4.20 (m, 1H), 3.80 (s, 3H), 3.72–3.62 (m, 1H), 3.47 (t, J = 6.8 Hz, 2H), 3.39 (d, J = 6.0 Hz, 2H), 3.38–3.29 (m, 1H), 2.48 (dd, J = 14.3, 6.7 Hz, 1H), 2.38 (d, J = 6.8 Hz, 2H), 2.28 (dd, J = 14.3, 6.0 Hz, 1H), 1.88–1.67 (m, 4H), 1.64–1.36 (m, 4H), 1.30–1.06 (m, 3H), 0.90 (s, 9H), 0.87 (d, J = 6.8 Hz, 3H), 0.85 (s, 9H), 0.04 (s, 3H), 0.02 (s, 6H), 0.00 (s, 3H); 13C NMR (75 MHz, CDCl3): δ = 192.6, 190.6, 159.1, 130.4, 129.2, 113.7, 102.1, 76.6, 74.6, 72.6, 67.8, 67.2, 66.2, 55.2, 46.5, 45.7, 39.7, 37.4, 32.4, 31.5, 31.4, 25.9, 25.8, 23.5, 17.4, −4.7, −5.3; MS (ESI): m/z = 701 [M + Na]+; HRMS: calcd. for C37H66O7Si2Na (M + Na)+ 701.4239; found 701.4234.
(S)-6-(((2S,6S)-6-((R)-3-Hydroxy-2-methylpropyl)tetrahydro-2H-pyran-2-yl)methyl)-2-(2-(4-methoxybenzyloxy)ethyl)-2H-pyran-4(3H)-one (28).
To a stirred solution of compound 27 (750 mg, 1.10 mmol) in MeCN (10 mL) was added HF (0.5 mL, 40% aq.) at room temperature. The resulting reaction mixture was stirred at room temperature for 4 h, and was then quenched by the addition of saturated NaHCO3 (15 mL). The resulting mixture was extracted with EtOAc (2 × 20 mL), and the combined organic phases were washed with brine, dried over Na2SO4, and concentrated in vacuo. The residue was purified by flash column chromatography (hexanes–EtOAc, 1
:
1) to give compound 28 (408 mg, 86%) as a colorless oil. Rf 0.20 (50% EtOAc in hexanes); IR (KBr): νmax 3451, 2927, 2858, 1658, 1606, 1247, 1091, 1034 cm−1; [α]20D = +69.4 (c = 0.50, CHCl3); 1H NMR (500 MHz, CDCl3): δ = 7.19 (d, J = 8.8 Hz, 2H), 6.82 (d, J = 8.8 Hz, 2H), 5.37 (s, 1H), 4.59–4.51 (m, 1H), 4.41 (s, 2H), 3.80 (s, 3H), 3.61–3.47 (m, 3H), 3.45–3.34 (m, 3H), 2.46–2.35 (m, 2H), 2.26 (dd, J = 14.7, 4.9 Hz, 1H), 1.95–1.80 (m, 3H) 1.60–1.44 (m, 4H), 1.41–1.34 (m, 1H), 1.30–1.17 (m, 3H), 0.90 (d, J = 6.8 Hz, 3H); 13C NMR (75 MHz, CDCl3): δ = 193.0, 174.2, 159.1, 130.0, 129.2, 113.7, 105.5, 75.6, 74.7, 72.7, 67.4, 65.1, 55.2, 41.5, 40.7, 40.0, 34.4, 32.2, 31.2, 31.1, 29.6, 23.4, 17.3; MS (ESI): m/z = 433.2 [M + H]+; HRMS: calcd. for C25H37O6 (M + H)+ 433.2585; found 433.2560.
(2S,6R)-2-(((2S,6S)-6-((R)-3-Hydroxy-2-methylpropyl)tetrahydro-2H-pyran-2-yl)methyl)-6-(2-(4-methoxybenzyloxy)ethyl)dihydro-2H-pyran-4(3H)-one (3).
Palladium on carbon (100 mg, 10% wet weight) was added to a solution of compound 28 (410 mg, 0.95 mmol) and K2CO3 (131 mg, 0.95 mmol) in EtOAc (5 mL). The reaction mixture was stirred overnight under a hydrogen atmosphere. After completion of the reaction, the mixture was filtered through celite and the resulting filtrate was concentrated in vacuo. Column chromatography of the residue on silica gel (40% ethyl acetate in hexanes) gave compound 3 (380 mg, 92%) as a colorless oil. Rf 0.40 (50% EtOAc in hexanes); IR (KBr): νmax 3433, 2931, 2859, 1716, 1606, 1512, 1253, 1088, 1033, 751 cm−1; [α]28D = +8.9 (c = 1.30, CHCl3); 1H NMR (400 MHz, CDCl3): δ = 7.18 (d, J = 8.5 Hz, 2H), 6.82 (d, J = 8.5 Hz, 2H), 4.39 (s, 2H), 3.80 (s, 3H), 3.78–3.68 (m, 2H), 3.57–3.37 (m, 6H), 2.36 (t, J = 13.1 Hz, 2H), 2.21 (td, J = 13.1, 5.4 Hz, 2H), 1.99–1.72 (m, 5H), 1.60–1.35 (m, 5H), 1.31–1.16 (m, 3H), 0.90 (d, J = 6.9 Hz, 3H); 13C NMR (75 MHz, CDCl3): δ = 207.8, 159.3, 130.4, 129.3, 113.9, 75.7, 74.5, 74.3, 74.0, 72.9, 67.7, 66.0, 55.4, 48.0, 47.7, 42.4, 40.5, 36.5, 32.3, 31.5, 31.2, 29.8, 23.7, 17.6; MS (ESI): m/z = 457 [M + Na]+; HRMS: calcd. for C25H38O6Na (M + Na)+ 457.2561; found 457.2571.
(R)-3-((2R,6S)-6-(((2S,6R)-4,4-Dimethoxy-6-(2-(4-ethoxybenzyloxy)ethyl)tetrahydro-2H-pyran-2-yl)methyl)tetrahydro-2H-pyran-2-yl)-2-methylpropan-1-ol (30).
To a stirred solution of 3 (141 mg, 0.32 mmol) in MeOH (2.4 mL) and (MeO)3CH (0.8 mL) at room temperature was added p-TSA·H2O (6.1 mg, 0.032 mmol). After being stirred for 15 min at room temperature, the reaction was quenched with saturated aqueous NaHCO3 (5 mL). The resulting mixture was extracted with CH2Cl2 (2 × 10 mL), and the combined organic layers were washed with brine (10 mL), dried over Na2SO4, filtered and concentrated under reduced pressure. The residue was purified by flash column chromatography (silica gel, hexanes
:
EtOAc =70
:
30) to give dimethyl ketal 30 (126 mg, 82%) as a colorless oil. Rf 0.50 (50% EtOAc in hexanes); IR (KBr): νmax 3452, 2927, 2858, 1610, 1512, 1428, 1358, 1223, 1151, 1033, 751 cm−1; [α]20D = +11.3 (c = 0.70, CHCl3); 1H NMR (500 MHz, CDCl3): δ = 7.25 (d, J = 8.9 Hz, 2H), 6.87 (d, J = 8.9 Hz, 2H), 4.42 (s, 2H), 3.80 (s, 3H), 3.66–3.39 (m, 8H), 3.20 (s, 3H), 3.15 (s, 3H), 2.01–1.89 (m, 2H), 1.88–1.66 (m, 3H), 1.65–1.37 (m, 6H), 1.33–1.12 (m, 6H), 0.92 (d, J = 7.2 Hz, 3H); 13C NMR (75 MHz, CDCl3): δ = 158.9, 130.4, 129.0, 113.6, 98.9, 75.0, 74.5, 72.5, 70.9, 70.5, 67.5, 66.4, 55.0, 47.5, 47.1, 41.8, 40.4, 38.5, 38.4, 35.8, 32.1, 30.9, 23.5, 17.5; MS (ESI): m/z = 503.3 [M + Na]+.
tert-Butyl((1E,3R,4S,5E,7R)-8-((2R,6S)-6-(((2S,6R)-4,4-dimethoxy-6-(2-(4-methoxybenzyloxy)ethyl)tetrahydro-2H-pyran-2-yl)methyl)tetrahydro-2H-pyran-2-yl)-1-iodo-4,7-dimethylocta-1,5-dien-3-yloxy)dimethylsilane (31).
To a stirred solution of 30 (85 mg, 0.18 mmol) in dry CH2Cl2 (4 mL) at 0 °C was added NaHCO3 (60 mg, 0.71 mmol) and Dess–Martin periodinane (115 mg, 0.27 mmol) in one portion. The mixture was stirred for 1 h at 0 °C and quenched with saturated aqueous Na2S2O3 (4 mL). The layers were separated and the aqueous phase was extracted with CH2Cl2 (2 × 5 mL). The combined organic layers were washed with brine (5 mL), dried over Na2SO4 and concentrated in vacuo. The residue was purified by flash column chromatography (30% EtOAc in hexanes) to give the aldehyde (73 mg, 86%) as a colorless oil, which was immediately used in the next reaction.
To a −78 °C solution of sulfone 4 (160 mg, 0.29 mmol) in a mixture of THF (0.8 mL) and HMPA (0.2 mL) was added LiHMDS (0.28 mL of a 1 M solution in THF, 0.28 mmol). After 30 min at that temperature, the above aldehyde (70 mg, 0.15 mmol) was added to a solution of THF (0.3 mL) and HMPA (0.1 mL). The reaction mixture was stirred for 2 h at −78 °C, after which it was slowly warm to room temperature over a period of 3 h and stirred at this temperature for 12 h. The mixture was subsequently diluted with ether (5 mL) and quenched by the addition of a saturated NH4Cl solution (2 mL). The organic phase was washed with water, brine, dried over Na2SO4, and concentrated in vacuo. The residue was purified by column chromatography (hexanes
:
EtOAc, 95
:
5 to 90
:
10) to give 31 (67 mg, 56%) and the aldehyde (20 mg, 28%). Rf 0.40 (20% EtOAc in hexanes); IR (KBr): νmax 2932, 2859, 1606, 1512, 1436, 1374, 1253, 1088, 1032, 751 cm−1; [α]20D = +9.17 (c = 0.60, CHCl3); 1H NMR (300 MHz, CDCl3): δ = 7.25 (d, J = 8.5 Hz, 2H), 6.82 (d, J = 8.5 Hz, 2H), 6.51 (dd, J = 14.5, 6.2 Hz, 1H), 6.14 (d, J = 14.5 Hz, 1H), 5.26–5.20 (m, 2H), 4.42 (s, 2H), 3.88–3.82 (m, 1H), 3.80 (s, 3H), 3.71–3.39 (m, 5H), 3.32–3.22 (m, 1H), 3.20 (s, 3H), 3.15 (s, 3H), 2.45–2.26 (m, 1H), 2.23–2.11 (m, 1H), 1.99 (t, J = 11.7 Hz, 2H), 1.91–1.67 (m, 2H), 1.64–1.37 (m, 4H), 1.33–1.12 (m, 8H), 0.97 (d, J = 6.6 Hz, 3H), 0.95 (d, J = 6.6 Hz, 3H), 0.89 (s, 9H), 0.03 (s, 3H), 0.00 (s, 3H); 13C NMR (75 MHz, CDCl3): δ = 159.0, 148.0, 137.1, 130.6, 129.9, 129.1, 113.7, 99.0, 79.1, 76.0, 75.5, 74.0, 72.6, 71.0, 70.8, 66.6, 55.2, 47.6, 47.3, 44.0, 43.5, 42.5, 38.8, 38.6, 36.0, 33.0, 32.1, 31.4, 29.6, 25.8, 23.6, 21.7, 18.2, 16.2, −4.4, −4.8; MS (ESI): m/z = 823.4 [M + Na]+; HRMS: calcd. for C39H65IO7SiNa (M + Na)+ 823.3436; found 823.3448.
2-((2R,6S)-6-(((2S,6R)-6-((2R,3E,5S,6R,7E)-6-(tert-Butyldimethylsilyloxy)-8-iodo-2,5-dimethylocta-3,7-dienyl)tetrahydro-2H-pyran-2-yl)methyl)-4,4 dimethoxytetrahydro-2H-pyran-2-yl)ethanol (32).
To a solution of 31 (60 mg, 0.075 mmol) in CH2Cl2 (0.9 mL) and pH 7 buffer solution (0.1 mL) was added DDQ (20.4 mg, 0.09 mmol) at 0 °C. The reaction mixture was stirred for 30 min, and then poured into water. The aqueous phase was extracted with CH2Cl2 (2 × 5 mL). The combined organic extracts were washed with aq. Na2S2O3 (5 mL), aq. NaHCO3 (5 mL), dried over anhydrous Na2SO4 and concentrated in vacuo. Flash chromatography (hexanes
:
EtOAc, 80
:
20) afforded alcohol 32 (47 mg, 92%) as a colorless oil. Rf 0.30 (20% EtOAc in hexanes); IR (KBr): νmax 3454, 2927, 2858, 1612, 1514, 1452, 1233, 1142, 1033, 772 cm−1; [α]20D = −1.8 (c = 0.50, CHCl3); 1H NMR (500 MHz, CDCl3): δ = 6.50 (dd, J = 14.9, 6.0 Hz, 1H), 6.15 (d, J = 14.9 Hz, 1H), 5.29–5.19 (m, 2H), 3.86 (t, J = 6.0 Hz, 1H), 3.81–3.68 (m, 4H), 3.44–3.35 (m, 1H), 3.29–3.22 (m, 1H), 3.21 (s, 3H), 3.16 (s, 3H), 2.72 (br s, 1H), 2.39–2.25 (m, 1H), 2.17 (dd, J = 13.0, 7.0 Hz, 1H), 2.02 (d, J = 13.0 Hz, 1H), 1.92 (d, J = 13.0 Hz, 1H), 1.86–1.62 (m, 3H), 1.60–1.41 (m, 4H), 1.38–1.11 (m, 7H), 0.97 (d, J = 7.0 Hz, 3H), 0.95 (d, J = 7.0 Hz, 3H), 0.89 (s, 9H), 0.04 (s, 3H), 0.01 (s, 3H); 13C NMR (75 MHz, CDCl3): δ = 148.0, 137.1, 130.0, 98.6, 79.0, 76.1, 75.6, 74.7, 74.1, 71.4, 61.6, 47.7, 47.3, 43.9, 43.5, 42.5, 38.6, 38.3, 37.5, 33.0, 31.9, 31.7, 29.6, 25.8, 23.5, 21.7, 18.1, 16.3, −4.4, −4.9; MS (ESI): m/z = 703 [M + Na]+; HRMS: calcd. for C31H57IO6Si (M + Na)+ 703.2861; found 703.2852.
2-((2S,6S)-6-(((2S,6R)-6-((2R,3E,5S,6R,7E)-6-Hydroxy-8-iodo-2,5-dimethylocta-3,7-dienyl)tetrahydro-2H-pyran-2-yl)methyl)-4,4-dimethoxytetrahydro-2H-pyran-2-yl)acetic acid (33).
To a solution of above alcohol 32 (60 mg, 0.09 mmol) in H2O–CH2Cl2 (1
:
1, 1 mL) were added TEMPO (4.0 mg, 0.026 mmol) and BAIB (87 mg, 0.27 mmol). After stirring at room temperature for 2 h, the reaction mixture was diluted with CH2Cl2 (5 mL) and then washed with saturated aqueous Na2S2O3 (5 mL). The organic layer was dried over Na2SO4, filtered, and the filtrate was concentrated under reduced pressure to give the crude carboxylic acid, which was used for the next reaction without further purification.
A solution of the above crude carboxylic acid in THF (2 mL) was cooled to 0 °C and TBAF (0.9 mL, 0.9 mmol, 1.0 M solution in THF) was added dropwise. The resulting brown solution was stirred at room temperature for 3 h. The reaction was quenched with saturated aqueous NH4Cl (2 mL) and extracted with EtOAc (2 × 5 mL). The combined organic layer was dried over Na2SO4 and evaporated to dryness under reduced pressure. The residue was purified by flash column chromatography (silica gel, CHCl3
:
MeOH = 98
:
2) to give seco acid 33 (47.5 mg, 91%) as a colorless oil. Rf 0.40 (5% MeOH in CHCl3); [α]20D = −4.6 (c = 1.00, CHCl3); 1H NMR (500 MHz, CDCl3): δ = 6.57 (dd, J = 14.0, 5.0 Hz, 1H), 6.33 (d, J = 14.0 Hz, 1H), 5.34–5.26 (m, 2H), 3.98 (t, J = 5.0 Hz, 1H), 3.93–3.85 (m, 1H), 3.73–3.65 (m, 1H), 3.43–3.35 (m, 1H), 3.28–3.22 (m, 1H), 3.21 (s, 3H), 3.17 (s, 3H), 2.56 (dd, J = 15.0, 8.0 Hz, 1H), 2.47 (dd, J = 15.0, 5.0 Hz, 1H), 2.44–2.37 (m, 1H), 2.35–2.23 (m, 1H), 2.07–1.94 (m, 2H), 1.89–1.74 (m, 2H), 1.64–1.40 (m, 6H), 1.38–1.10 (m, 4H), 1.00 (d, J = 7.0 Hz, 3H), 0.96 (d, J = 7.0 Hz, 3H); 13C NMR (75 MHz, CDCl3): δ = 174.9, 146.6, 138.0, 129.7, 98.6, 77.7, 77.5, 75.7, 74.3, 71.5, 70.5, 47.7, 47.4, 43.5, 42.4, 42.2, 40.6, 38.6, 37.8, 33.2, 31.9, 31.2, 29.6, 23.6, 21.6, 15.6; MS (ESI): m/z = 603.3 [M + Na]+.
Macrolactone (34).
To a solution of seco acid 33 (60 mg, 0.103 mmol) in THF (5 mL) at 0 °C were added Et3N (0.29 mL, 2.06 mmol) and 2,4,6-Cl3C6H2COCl (0.16 mL, 1.03 mmol). After being stirred at room temperature for 4 h, the reaction mixture was diluted with toluene (10 mL) and added dropwise to a solution of DMAP (503 mg, 4.12 mmol) in toluene (100 mL) at 80 °C over a period of 10 h. Upon complete addition, stirring was continued for an additional 12 h. After being cooled to room temperature, the mixture was concentrated to about 20 ml, diluted with EtOAc (15 mL), and the solution was successively washed with aqueous HCl, satd. aqueous NaHCO3, and brine, dried over Na2SO4, and concentrated in vacuo. The residue was purified by column chromatography (silica gel, hexanes
:
EtOAc = 95
:
05) providing macrolactone 34 (36.5 mg, 63%) as a colorless oil. Rf 0.40 (10% EtOAc in hexanes); IR (KBr): νmax 2926, 2854, 1739, 1660, 1457, 1373, 1282, 1256, 1185, 1098, 1045, 947 cm−1; [α]20D = −11.5 (c = 1.00, CHCl3); 1H NMR (300 MHz, CDCl3): δ = 6.50 (dd, J = 15.1, 6.0 Hz, 1H), 6.33 (d, J = 15.1 Hz, 1H), 5.47 (dd, J = 15.1, 9.0 Hz, 1H), 5.13 (dd, J = 7.5, 2.2 Hz, 1H), 5.07 (dd, J = 15.1, 9.8 Hz, 1H), 3.98–3.86 (m, 1H), 3.36–3.13 (m, 3H), 3.21 (s, 3H), 3.16 (s, 3H), 2.60–2.47 (m, 1H), 2.47–2.42 (m, 2H), 2.40–2.27 (m, 1H), 1.98 (dt, J = 12.8, 2.2 Hz, 1H), 1.86 (dt, J = 13.5, 2.2 Hz, 1H), 1.80–1.61 (m, 2H), 1.53–1.40 (m, 2H), 1.38–1.21 (m, 8H), 1.04 (d, J = 6.8 Hz, 3H), 0.93 (d, J = 6.8 Hz, 3H); 13C NMR (75 MHz, CDCl3): δ = 170.7, 142.8, 135.9, 131.7, 99.0, 79.4, 79.2, 75.9, 75.3, 71.3, 70.6, 47.6, 47.4, 43.9, 42.9, 41.0, 38.8, 37.6, 32.9, 32.3, 31.6, 29.6, 23.9, 21.7, 14.3. MS (ESI): m/z = 585 [M + Na]+; HRMS: calcd. for C25H39IO6Na (M + Na)+ 585.1684; found 585.1634.
Macrolactone (2)4a.
The above compound 34 (25 mg, 0.044 mmol) was dissolved in MeOH (1 mL) and the resulting solution was cooled to 0 °C and p-TSA·H2O (cat.) was added. The mixture was stirred for 15 min at 0 °C. And then solid NaHCO3 was added, and the solvents were removed under reduced pressure. Flash column chromatography (silica gel, 5→10% EtOAc in hexanes) furnished the desired compound 2 (19.5 mg, 86%) as a colorless oil. Rf 0.30 (10% EtOAc in hexanes); IR (KBr): νmax 2925, 2854, 1726, 1658, 1403, 1213, 1176, 1090, 975, 759 cm−1; [α]20D = −14.8 (c = 0.60, CHCl3); 1H NMR (300 MHz, CDCl3): δ = 6.51 (dd, J = 14.5, 6.4 Hz, 1H), 6.38 (d, J = 14.5 Hz, 1H), 5.45 (dd, J = 15.1, 9.4 Hz, 1H), 5.20 (dd, J = 6.0, 0.9 Hz, 1H), 5.11 (dd, J = 15.1, 9.4 Hz, 1H), 4.11–4.02 (m, 1H), 3.45 (t, J = 10.7 Hz, 1H), 3.31 (dd, J = 10.3, 8.7 Hz, 1H), 3.18 (t, J = 11.1 Hz, 1H), 2.62–2.55 (m, 2H), 2.55–2.46 (m, 1H), 2.45–2.29 (m, 5H), 1.88–1.73 (m, 2H), 1.66–1.36 (m, 5H), 1.29–1.10 (m, 3H), 1.06 (d, J = 7.0 Hz, 3H), 0.95 (d, J = 6.8 Hz, 3H); 13C NMR (75 MHz, CDCl3): δ = 205.9, 169.8, 142.5, 136.1, 131.5, 79.7, 79.5, 76.0, 74.9, 74.3, 73.0, 47.7, 46.5, 43.9, 43.0, 41.2, 41.0, 33.1, 32.4, 31.5, 23.8, 21.7, 14.2; MS (ESI): m/z = 539 [M + Na]+; HRMS: calcd. for C23H34IO5 (M + H)+ 517.1445; found 517.1427.
Acknowledgements
NNR thank the Council of Scientific and Industrial Research (CSIR), New Delhi, for the award of fellowship.
References
- S. Ohta, M. M. Uy, M. Yanai, E. Ohta, T. Hirata and S. Ikegami, Tetrahedron Lett., 2006, 47, 1957–1960 CrossRef CAS.
-
(a) G. R. Pettit, C. L. Herald, D. L. Doubek, D. L. Herald, E. Arnold and J. Clardy, J. Am. Chem. Soc., 1982, 104, 6846–6848 CrossRef CAS;
(b) J. Cossy, C. R. Chim., 2008, 11, 1477–1482 CrossRef CAS.
- M. S. Kwon, S. K. Woo, S. W. Na and E. Lee, Angew. Chem., Int. Ed., 2008, 47, 1733–1735 CrossRef CAS.
-
(a) H. Fuwa and M. Sasaki, Org. Lett., 2010, 12, 584–587 CrossRef CAS;
(b) C. Cook, X. Guinchard, F. Liron and E. Roulland, Org. Lett., 2010, 12, 744–747 CrossRef CAS;
(c) E. Crane, T. P. Zabava, R. L. Farmer and K. A. Scheidt, Angew. Chem., Int. Ed., 2011, 50, 9112–9115 CrossRef CAS.
- H. Fuwa, T. Suzuki, H. Kubo, T. Yamori and M. Sasaki, Chem.–Eur. J., 2011, 17, 2678–2688 CrossRef CAS.
-
(a) Ch. R. Reddy, D. Suman and N. N. Rao, Synlett, 2012, 23, 272–274 CrossRef CAS;
(b) Ch. R. Reddy and N. N. Rao, Eur. J. Org. Chem., 2012, 1819–1824 CrossRef;
(c) Ch. R. Reddy, N. N. Rao and B. Srikanth, Eur. J. Org. Chem., 2010, 345–351 CrossRef;
(d) Ch. R. Reddy, P. P. Madhavi and S. Chandrashekar, Tetrahedron: Asymmetry, 2010, 21, 103–105 CrossRef CAS;
(e) Ch. R. Reddy and B. Srikanth, Synlett, 2010, 1536–1538 CrossRef CAS;
(f) Ch. R. Reddy, G. Dharmapuri and N. N. Rao, Org. Lett., 2009, 11, 5730–5733 CrossRef CAS;
(g) Ch. R. Reddy and N. N. Rao, Tetrahedron Lett., 2009, 50, 2478–2480 CrossRef.
- For preliminary communication of our work see : Ch. R. Reddy and N. N. Rao, Tetrahedron Lett., 2010, 51, 5840–5842 CrossRef.
- Y. Shiro, K. Kato, M. Fujii, Y. Ida and H. Akita, Tetrahedron, 2006, 62, 8687–8695 CrossRef CAS.
- S. Hanessian, R. J. Roy, M. Petrini, P. J. Hodges, R. Di Fabio and G. J. Carganico, J. Org. Chem., 1990, 55, 5766–5777 CrossRef CAS.
- C. Fouquet and M. Schlosser, Angew. Chem., Int. Ed. Engl., 1974, 13, 82–83 CrossRef.
- Synthesis of 15 via cross-metathesis reaction.
.
- J. Liu, J. H. Yang, C. Ko and R. P. Hsung, Tetrahedron Lett., 2006, 47, 6121–6123 CrossRef CAS.
- J. A. Frick, J. B. Klassen, A. Bathe, J. M. Abramson and H. Rapoport, Synthesis, 1992, 7, 621–623 CrossRef.
- Improvement in the yield of the product was observed when Li2CuCl4 was used instead of CuI.
- Y. Wu, X. Liao, R. Wang, X.-S. Xie and J. K. De Brabander, J. Am. Chem. Soc., 2002, 124, 3245–3253 CrossRef CAS.
- The dithiane alternative route was accomplished using simple reagents (Scheme 4) by avoiding cuprate and osmium reagents (Scheme 3).
-
(a) I. Marek, C. Meyer and J-F. Normant, Org. Syn., 1997, 74, 194 CAS;
(b) B. M. Trost, M. U. Frederiksen, J. P. N. Papillon, P. E. Harrington, S. Shin and B. T. Shireman, J. Am. Chem. Soc., 2005, 127, 3666–3667 CrossRef CAS.
- D. A. Evans, J. Bartoli and T. L. Shih, J. Am. Chem. Soc., 1981, 103, 2127–2129 CrossRef CAS.
- O. Mitsunobu, Synthesis, 1981, 1–28 CrossRef CAS.
- H. S. Schultz, H. B. Freyermuth and S. R. Buc, J. Org. Chem., 1963, 28, 1140–1142 CrossRef CAS.
-
(a) R. F. Newton and D. P. Reynolds, Tetrahedron Lett., 1979, 20, 3981–3982 CrossRef;
(b) D.-R. Le, D.-H. Zhang, C.-Y. Sun, J.-W. Zhang, L. Yang, J. Chen, B. Liu, C. Su, W.-S. Zhou and G.-Q. Lin, Chem.–Eur. J., 2006, 12, 1185–1204 CrossRef.
- P. Wipf and J. T. Reeves, Chem. Commun., 2002, 2066–2067 RSC.
-
(a) P. R. Blakemore, W. J. Cole, P. J. Kocienski and A. Morley, Synlett, 1998, 26–28 CrossRef;
(b) P. R. Blakemore, J. Chem. Soc., Perkin Trans. 1, 2002, 2563–2585 RSC.
- Y. Oikawa, T. Yoshioka and O. Yonemitsu, Tetrahedron Lett., 1982, 23, 885–888 CrossRef CAS.
- H. Miyaoka, M. Yamanishi, A. Hoshino and A. Kinbara, Tetrahedron, 2006, 62, 4103–4109 CrossRef CAS.
- J. Inanaga, K. Hirata, H. Sacki, T. Hatsuki and M. Yamaguchi, Bull. Chem. Soc. Jpn., 1979, 52, 1989–1993 CrossRef CAS.
Footnote |
† Electronic supplementary information (ESI) available: Copies of 1H NMR and 13C NMR spectra of all the new compounds. See DOI: 10.1039/c2ra21161k. |
|
This journal is © The Royal Society of Chemistry 2012 |
Click here to see how this site uses Cookies. View our privacy policy here.