DOI:
10.1039/C2RA20989F
(Paper)
RSC Adv., 2012,
2, 7772-7780
Preparation and characterization of pH-sensitive hydrogel for drug delivery system
Received
19th April 2012
, Accepted 27th June 2012
First published on 27th June 2012
Abstract
In this paper, a new kind of pH-sensitive hydrogel based on poly(lactic acid) (PLA), methoxyl poly(ethylene glycol) (MPEG) and itaconic acid (IA) was prepared by heat-initiated free radical polymerization method without any organic solvent. The obtained macromonomers and hydrogels were characterized by 1H NMR and FTIR. Detailed swelling behavior of hydrogels under variational pH-conditions revealed that the dynamic sensitivity of P(MPEG-PLA-co-IA-MEGMA) hydrogels [P(LE-IA-MEG) hydrogels] as well as the diffusional mechanisms might be causing the network expansion and collapse. In vitro drug release behavior was investigated using VB12 and paclitaxel (PTX) as model drugs, revealing that the P(LE-IA-MEG) hydrogel could control the diffusion of the drug. The prepared new kind of pH-sensitive hydrogel with great pH-responsiveness and good properties for drug delivery might have great potential application in smart drug delivery systems.
1. Introduction
Polymeric hydrogels are of considerable importance because of their potential applications as biomaterials, such as soft contact lenses, artificial corneas, soft tissue substitutes and burn dressings.1 They have a great interest for pharmaceutical and medical applications. They are three-dimensional polymeric networks that swell quickly by imbibing a large amount of water or de-swell in response to changes in their external environment.2–5 The water uptake by hydrogels is sensitive to the external environment, including temperature, ionic strength, pH and the electric field.6–8 In more recent years, hydrogels have become popular carriers for drug delivery applications, especially for oral drug delivery owing to their biocompatibility and resemblance to biological tissues.9–10 For hydrogels, there are many advantages, including simple drug formulations and administration procedures, a sustained drug release behavior, no organic solvent, less systemic toxicity and, for some of them, a stimuli-responsive behavior.11–16 As advanced smart drug delivery systems, pH-sensitive hydrogels have a very important role. Various pH-sensitive hydrogels had been investigated widely and swelling behavior is affected by variation of the natural pH environment of the gastrointestinal tract in the human body, which varies from acidic in the stomach to neutral in the intestine.17 Thus, they allow the release of drugs at a specific site and are considered as great candidates for site-specific drug delivery carriers.
Several FDA approved products have been used in many biomedical materials containing MPEG and PLA that are both non-cytotoxic. PLA is biodegradable and non-cytotoxic with great permeability.18–21 In previous work,22 a kind of amphiphilic block copolymer containing the hydrophilic MPEG and the hydrophobic PLA was successfully synthesized, and the structure of the copolymer played an important role in the properties of the pH-sensitive hydrogel.
Owing to the fact that pH-sensitive hydrogels can conveniently change their volume in response to the environmental stimuli of different pH values, they have been extensively investigated as drug carriers.23 This has led to the fact that the diffusion and permeation of drug molecules from the hydrogels can be controlled by external pH. Compared to previous work,24 IA was chosen here to prepare a new kind of pH-sensitive hydrogel due to its two carboxyl groups in one molecule, which should achieve a satisfactory pH-responsive ability. In this study, a new kind of pH-sensitive P(LE-IA-MEG) hydrogel was successfully synthesized through a low temperature, heating-initiated free radical polymerization method without any organic solvent. This method is simple and practical compared with the UV photo-initiated free radical polymerization method that has been chosen to synthesise pH-sensitive hydrogels by many researchers. The solvent free method greatly reduced the toxicity of prepared hydrogels. Meanwhile, the pharmaceutical activity of many light-sensitive drugs, especially protein and peptide drugs was protected through heating-initiated free radical polymerization. Detailed investigation was carried out to reveal that the hydrogel has favorable water absorption, and that its pH-responsiveness was greatly affected by the pH value of the surrounding environment. Subsequently, in vitro degradation behavior and drug release behavior were also studied in this paper.
2. Materials and methods
2.1 Materials
MPEG (Mn = 2000), N,N′-Methylene-bis-acrylamide (MBAA), itaconic acid (IA), poly(ethylene glycol) methyl ether methacrylate (MPEGMA, MEGMA, Mn = 300), tin(II) 2-etheylhexanoate, acryloyl chloride (97%), ammonium persulfate (98%) (APS) and sodium hydrogensulfite (NaHSO3) were all analytical grade, and were purchased from Aldrich Company, USA. Vitamine B12 (VB12) and PTX (Sigma, USA) were used without further purification. D,L-lactide was synthesized in our lab. All the other reagents were also analytic grade and used as received.
2.2 Synthesis and purification of the P(LE-IA-MEG) hydrogel
MPEG-PLA block polymer was synthesized by ring-opening polymerization (ROP) of D,L-lactide initiated by MPEG2000 using tin(II) 2-etheylhexanoate as the catalyst.25 Then, the toluene solvent was concentrated using a rotary evaporator. The resultant copolymer was precipitated from cold petroleum ether three times, and dried in vacuum at 25 °C. After polymerization, the MPEG-PLA macromonomer was first dissolved in analytical reagent (AR) grade dichloromethane (DCM), with a considerable amount of triethylamine, and reacted with acryloyl chloride at 40 °C, allowing reflux for 4 h according to Scheme 1. The products were purified by repeated dissolution into DCM and precipitation by cold petroleum ether and were dried in vacuum at 25 °C. In the following text, the MPEG-PLA-AC macromonomer is denoted as PLE-AC for simplification.
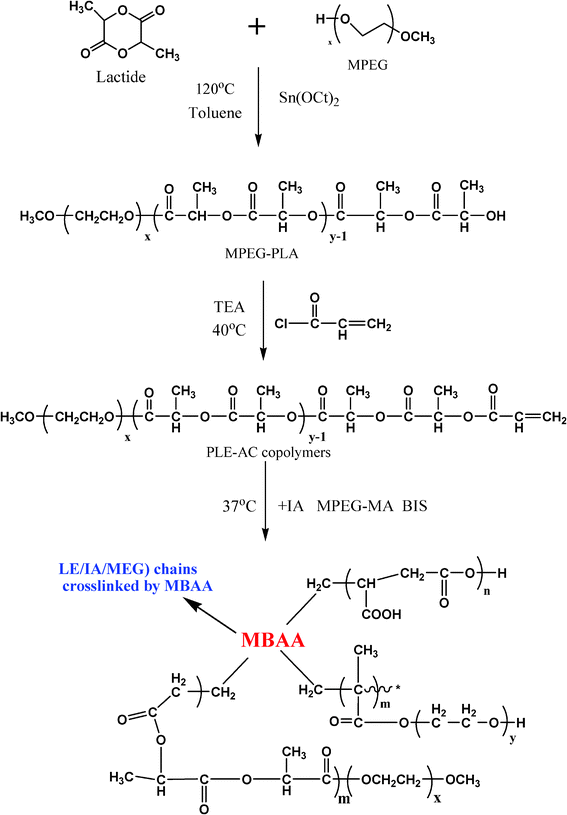 |
| Scheme 1 The synthesis of the P(LE-IA-MEG) hydrogel. | |
The P(LE-IA-MEG) hydrogel was synthesized by heat-initiated free radical polymerization with APS as the heat-initiator and MBAA as the cross-linker. Predetermined amounts of the PLE-AC macromonomer (0.8 g), IA (0.6 g), MPEG-MA (0.6 g), MBAA (6% w/w of the total monomers) and the redox initiator system of APS and NaHSO3 (3% w/w of the total monomers) were dissolved in water (6 ml), then the mixture was poured into a weighing bottle and heated to 37 °C with nitrogen for about 1 h according to Scheme 1 and Fig. 1. The just-obtained P(LE-IA-MEG) hydrogel was immersed in distilled water for 1 day in order to remove excess unreacted reagents. The purified hydrogels were first dried at room temperature for 1 day, and then dried at 40 °C under vacuum for 3 days. Then they were kept in airtight bags prior to use. The obtained samples in this work are shown in Table 1.
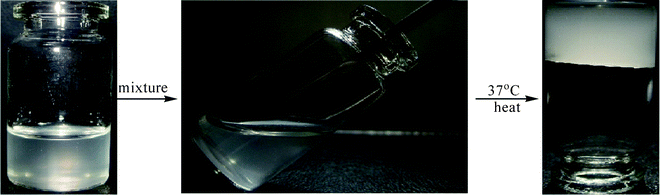 |
| Fig. 1 A photo of the formation of the P(LE-IA-MEG) hydrogel: the fluid mixture became a hydrogel after heating at 37 °C in the water bath. | |
Table 1 The hydrogels prepared in this paper
Sample |
LE : IA : MEG |
Redox initiator content (wt%) |
Gel content (wt%) |
RI
|
S-1 |
40 : 30 : 30 |
6 |
98 |
31.4 |
S-2 |
40 : 40 : 20 |
6 |
95.3 |
40.6 |
S-3 |
40 : 50 : 10 |
6 |
92.4 |
56.5 |
2.3 Structural characterization of the P(LE-IA-MEG) hydrogel
1H NMR spectra (in CDCl3) were recorded on a Varian 400 spectrometer (Varian, USA) at 400 MHz using tetramethysilane (TMS) as the internal standard.
FTIR (KBr) spectra of the hydrogel sample and the macromonomers were detected on a NICOLET 200SXV Infrared Spectrophotometer (Nicolet) at room temperature. All samples were cast on a KBr pallet before measurement.
2.4 Gel content of the P(LE-IA-MEG) hydrogel
PLA and MPEG homopolymers could be dissolved in chloroform, but when crosslinked by MBAA, the obtained P(LE-IA-MEG) hydrogel could not be dissolved in chloroform. So, in this work, the gel content was determined as follows:
The as-polymerized hydrogels (unpurified samples) were first dried in a vacuum oven at 50 °C for 3 days, then were immersed in chloroform for 24 h, and finally were vacuum dried at 50 °C for another 3 days. The gel content was calculated as follows:
Gel content (%) = Wt/W0 × 100
Where W0 and Wt are the dry weight of hydrogel before and after immersion in chloroform, respectively. The gel content of hydrogel samples are also shown in Table 1.
2.5 Morphological analysis
The morphological characterization of an internal section of hydrogel was performed by JEOL SEM (JSM-5900LV, JEOL, and Japan). The hydrogels were immersed in solutions of pH 1.2 and pH 6.8, and they were frozen in liquid nitrogen for 1 h. Afterwards, the hydrogels were sputtered with gold before observation.
2.6 Swelling study of the hydrogel
HCl/H2O solution (pH 1.2, 5.3 ml of concentrated hydrochloric acid was fully melted in 1000 ml water) and phosphate buffered saline (PBS: pH 6.8, 250 ml of 0.2 M KH2PO4, 118 ml of 0.2 M NaOH) were first prepared. Water absorption of the hydrogel was detected by weighing the hydrogel pieces after placing in different pH values (pH = 1.2 and 6.8) at 37 °C for different periods. Finally, the hydrogels were taken out and the surplus surface water was removed by filter paper. The percentages of water absorption can be determined by the following eqn (1): | Swelling ratio (SR) = Wt/W0 × 100% | (1) |
Where W0 is the initial dry weight and Wt is the wet weight of the hydrogel at time t. This experiment was performed in triplicate.
2.7 Dynamic swelling/deswelling kinetics
About 0.1 g of the dried hydrogels (S-1) were first immersed in aqueous medium at pH 1.2 for pre-determined times of 10 min, 20 min, 40 min, 60 min, 80 min, 100 min and 120 min. The fully swollen hydrogel was briefly removed from the media at certain time intervals and blotted with filter paper to remove any excess surface moisture before weighing. Afterwards, the hydrogel was put in another aqueous medium at pH 6.8, and the swelling ratios at different time points were measured too. This experiment was performed in triplicate.
In previous work,26 we defined a responsive index (RI), which was used to analyse pH sensitivity of a hydrogel. RI was calculated by following eqn (2):
| RI = SR(pH 6.8), t=120 − SR(pH 1.2), t=180 | (2) |
where SR(pH 6.8), t=120 and SR(pH 1.2), t=180 are the swelling ratios at t = 120 min (pH 6.8) and t = 180 min (pH 1.2), respectively. RI was defined as the difference between equilibrium swelling at pH 6.8 and pH 1.2. With an increase in RI, the difference between equilibrium swelling at pH 6.8 and 1.2 enhanced, which indicated that pH sensitivity of the hydrogel increased accordingly. The results are listed in Table 1.
2.8
In vitro hydrolytic degradation behavior of the hydrogel
In vitro hydrolytic degradation tests were carried out as follows: pre-weighed, dried hydrogels were put in aqueous media with pH = 1.2 and 6.8 at 37 °C and the aqueous media were refreshed every week. Samples were recovered periodically, washed thoroughly with distilled water and then dried in a vacuum desiccator for 6 days at 50 °C. The degree of degradation was calculated by weight loss according to eqn (3): | Weight loss (%) = (W0 − Wt)/W0 × 100%. | (3) |
W
0 is the dry weight before degradation and Wt is the dry weight at time t. This experiment was performed in triplicate.
2.9
In vitro drug release behavior of the hydrogel
2.9.1 Release of hydrophilic small-molecular-weight drugs.
In vitro release of the hydrophilic, small-molecular-weight drug VB12 from the P(LE-IA-MEG) hydrogel was determined. Briefly, 1 ml VB12 solution (5 mg ml−1) was carefully dropped into 0.1 g of gel with a syringe. After that, the gels were immersed in 2 ml of PBS (pH 6.8) or HCl/H2O solution (pH 1.2), and then they were shaken at 100 rpm at 37 °C. At specific time intervals, all of release media were removed and replaced by fresh release media. After centrifugation at 13
000 rpm for 10 min, the supernatant of the removed release media was collected and stored at −20 °C before analysis. The released drugs from the hydrogels were analyzed by an ultraviolet spectrophotometer (Shimazu, UV-2450, Japan) at 360 nm.27
2.9.2 Release of hydrophobic small-molecular-weight drugs.
Freshly prepared paclitaxel loaded P(LE-IA-MEG) hydrogel complexes were used to assay the in vitro release behavior of hydrophobic drugs. Briefly, 0.1 g of PTX/ P(LE-IA-MEG) hydrogel complexes containing 1 mg PTX were immersed in 2 ml of PBS (pH 6.8) or HCl/H2O solution (pH 1.2), and then they were shaken at 100 rpm at 37 °C. The concentration of PTX was detected by HPLC (Waters Alliance 2695). Chromatographic separations were performed on a reversed phase C18 column (4.6 mm × 250 mm − 5 μm, Apollo column). Acetonitrile/water (60/40 v/v) was used as the eluent at a flow rate of 1 ml min−1. Each experiment was repeated 3–4 times, and the results were expressed as mean value ± SD.
3. Results and discussion
3.1 PLE-AC monopolymer and P(LE-IA-MEG) hydrogel characterization
Over the past decades, pH-sensitive hydrogels for controlled drug delivery systems have received great attention owing to the unique formation of pH-sensitive hydrogels. Previous studies of pH sensitive hydrogels for oral drug delivery were concentrated mainly on anionic hydrogels that do not swell at gastric pH and swell significantly at intestinal pH. Since the first time a series of block copolymers consisting of MPEG and PLA segments was prepared, these di-block copolymers containing PLA blocks and MPEG blocks have been widely investigated. In this paper, by ring-opening copolymerization of lactide on MPEG, and a new kind of pH-sensitive hydrogel based on a di-block MPEG-PLA copolymer, IA and MPEG-MA was developed. Fig. 1 shows that the formation of the P(LE-IA-MEG) hydrogel. 1H NMR, FT-IR and SEM results indicated that the P(LE-IA-MEG) hydrogel was successfully prepared.
The MPEG-PLA copolymer was synthesized by a melting method through lactone rings in D,L-lactide initiated by MPEG. Simultaneously, the hydrogel was synthesized by a heating-initiated, free radial polymerization method. Table 1 summarizes the factors influencing the hydrogel, including PLE content, APS content, gel content and responsive index.
The chemical structure of the PLE-AC macromonomer was confirmed by 1H NMR as presented in Fig. 2a. The characteristic peaks of the product are marked in this figure. The signals at 5.8, 6.1 and 6.4 ppm correspond to protons of the carbon-carbon double bonds, and the presence of methane (CH) and methyl (CH3) protons in PLA were observed at around 5.1 ppm and 1.4 ppm, respectively. The methane protons in CH2 groups of MPEG were recorded at around 3.6 ppm. These results indicated that the terminal hydroxyl groups in the MPEG precursor were linked with PLA and then the PLA chain was further converted to acrylate groups.
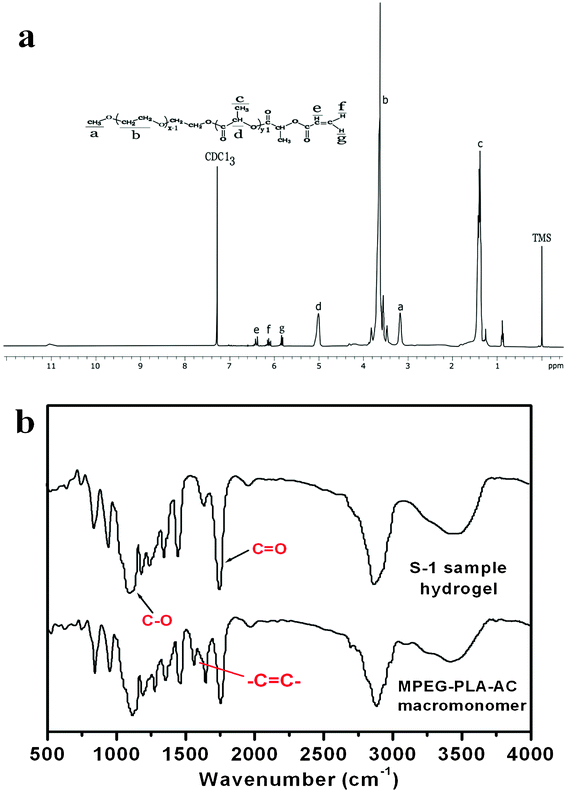 |
| Fig. 2 Structural characterization: 1H NMR spectrum of the PLE-AC macromonomer (in CDCl3) (a); FTIR spectra of the PLE-AC macromonomer and he S-1 hydrogel sample (b). | |
According to the characteristic spectra of the copolymer and hydrogel in Fig. 2b, an attempt was made to determine the eventual presence of interactions between them. In general, the absorption bands of 1146 and 1749 cm−1 were attributed to ether (C–O) and ester (C
O) bond-stretching peaks, respectively. The peaks at 1127 and 1454 cm−1 belonged to the stretching vibrations of CH2CH2O from the MPEG block. The peak at 1631 cm−1 was attributed to C
C stretching of the PLE-AC macromonomer, which disappeared in the S-1 sample hydrogel, which indicated that during the formation of hydrogel, the end carbon-carbon double bonds were converted to carbon-carbon single bonds completely to form the main chain.
Careful examination of representative hydrogels of each formulation using SEM microscopy revealed more detailed information regarding the internal and external morphological features. The cross-sectional morphology of the blank and drug loaded P(LE-IA-MEG) hydrogel in different aqueous media at pH 1.2 and 6.8 are presented in Fig. 3. According to Fig. 3, both the cross-sectional morphologies of the blank and drug loaded hydrogel at pH 1.2 are very dense, and the mesh sizes are small. To the contrary, the mesh sizes increased a lot, and the cross-sectional morphology became loose and the crack-network got larger than that when the pH was raised to 6.8.
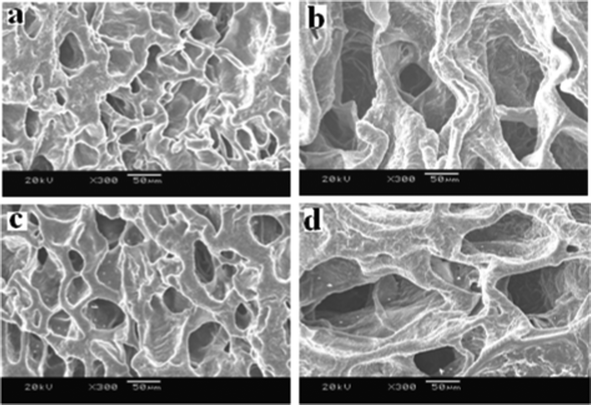 |
| Fig. 3 SEM observation of (a) the blank hydrogel at pH 1.2; (b) the blank hydrogel at pH 6.8; (c) the PTX loaded hydrogel at pH 1.2 and; (d) the PTX loaded hydrogel at pH 6.8. | |
Gel contents of the obtained hydrogels are listed in Table 1. For each sample (S-1, S-2, S-3), with the increase in IA content, the gel content decreased significantly. Meanwhile, the gel content decreased significantly according to the decrease of MPEG-MA content.
3.2 Water adsorption of hydrogels
The ability of a hydrogel to preserve water is one of the most important aspects for drug delivery systems, especially for oral drug delivery systems. Generally, the permeability of networks significantly increases with increasing water content. The water retained in the hydrogel network was considerable, determined by the hydrophilic and hydrophobic segments of this hydrogel. In Table 1 and Fig. 4a, the factors influencing the network structures are indicated, and we could find that the equilibrium swelling ratios of this hydrogel in pH 6.8 buffer solution were much higher than those in acidic solution (pH 1.2). The effect of pH value on the swelling ratio was determined in buffer media of varying pH from 1.2 to 6.8. In all compositions, a lesser extent of swelling was presented at pH 1.2 and maximum extents of swelling were reached at pH 6.8. The influence of pH values of the buffer solution on the equilibrium swelling behavior of hydrogels at 37 °C is observed in Fig. 4a. At low pH (pH 1.2), due to the existence of dissociative carboxylic acid groups in the hydrogel, there were a lot of hydrogen bonds and, consequently, this hydrogen-bonded complex restricted the movement or relaxation of network chains. After the pH value was raised to 6.8, these free carboxylic acid groups were partially ionized, and a great many hydrogen bonds were broken, generating electrostatic repulsion among polymer chains, leading to the swelling of the hydrogel. IA was chosen to prepare this kind of hydrogel owing to its special composition, which contains two carboxylic acid groups per molecule, causing water binding affinity to be extensively enlarged and pH-responsibility of hydrogels to be greatly enhanced. The swelling ratio increased with the increase of IA content in the hydrogel. The higher the content of IA in the hydrogel, the larger the ability of the hydrogels to attach to water. As can be seen in Fig. 4a, the swelling ratio of S-3 is larger than that of S-1 and S-2. From Fig. 3, we could find that the cross-sectional morphology of the hydrogel at pH 1.2 is very dense, and the mesh size is small. Conversely, it becomes loose and its mesh size increased a lot when the pH is elevated to 6.8. These data and phenomenon mentioned above indicate the great effect of pH value on these hydrogels.
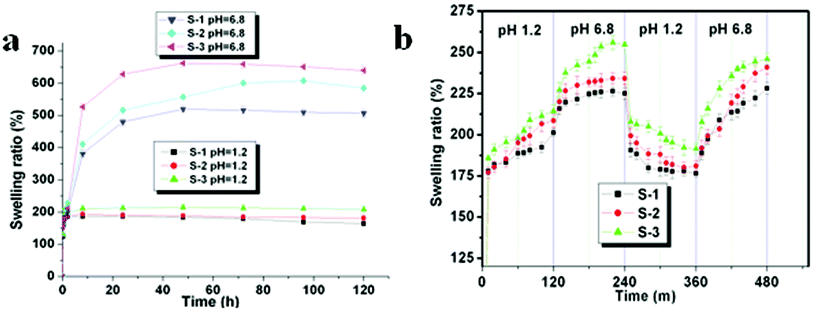 |
| Fig. 4 Swelling characterization of hydrogels in aqueous medium at different pH values (pH = 1.2 and pH = 6.8) at 37 °C for up to 120 h (a). Dynamic swelling/deswelling behavior of hydrogels in aqueous medium with pH 1.2 and pH 6.8 at an interval of 120 min (37 °C). P < 0.05, significant differences of S-3 as compared with S-1 and S-2 at the same time point (b). Data are shown as mean ± SD for n = 3 − 4. | |
3.3 pH-sensitivity of the hydrogels
The dynamic swelling/deswelling behavior (pH-sensitivity) of P(LE-IA-MEG) hydrogels in buffer solutions with different pH values at room temperature is shown in Fig. 4b. pH is a key factor in oral drug delivery systems, which influences the swelling ratio of pH-sensitive hydrogels. In Fig. 4b, these data showed the better pH-sensitive characterization of P(LE-IA-MEG) hydrogels. The environmental pH value has a large effect on the swelling behavior of the P(LE-IA-MEG) hydrogels. To evaluate the dynamic swelling/deswelling behavior (pH-sensitivity) of P(LE-IA-MEG) hydrogels, the equilibrium reswelling behavior of the hydrogels was studied in this work, and the results are presented in Fig. 4b. In the initial stage of hydration, a gradual increase in swelling ratios from pH 1.2 to pH 6.8 at a determined interval of time is illustrated in Fig. 4b. Then the sharp decreased in swelling ratio is obviously observed from pH 6.8 to pH 1.2. Under low pH conditions (pH 1.2), anionic carboxylate groups are protonated, leading to the collapse of the polymeric network. At about pH 4.5, the carboxylic acid component comes into action as well. The main reason for that is the free carboxylic acid groups of the hydrogel tend to dissociate at a pH > 4.5.28,29 However, with further increase of pH up to 6.8, ionization of carboxylic acid groups happens, and the hydrogen bonds break. Then, the repulsive action between these groups promotes the swelling of the hydrogel and causes a higher swelling ratio.30
3.4
In vitro hydrolytic degradation behavior of the hydrogel
The hydrolytic degradation behavior of P(LE-IA-MEG) hydrogels in buffer solution was also studied in this paper, and the results are presented in Fig. 5. In order to access the effect of IA content on the hydrolytic degradation, specimens were degraded in pH 1.2 and pH 6.8 media for a period of seven weeks. The results show that the degradation rate of hydrogel was greatly depended on the content of IA. The greater the content of IA, the faster the degradation rate of hydrogel. The hydrolytic degradation behavior of P(LE-IA-MEG) hydrogels in different pH buffer solutions was also studied in detail. According to Seppälä's opinion,31 the hydrolytic degradation behavior of semicrystalline polymers is determined by chemical composition, degree of crystallinity, hydrophilicity and the pH value of the degradation medium. The specimens were swollen in the buffer solution and degraded in pH 1.2 and pH 6.8 for a period of time and the mass loss was detected. Simultaneously, we found that the chemical composition changed. The possible reason for that is during the accelerated degradation, PLA, accompanying cleavage of the ester bond, could indeed be hydrolyzed, leading to the decrease in weight loss. The degradation rate might be determined by the content of IA. The more the IA content is, the larger the water binding affinity, leading to accelerated cleavage of the ester bond in the PLA segment and the increased degradation rate of hydrogels. Moreover, the hydrolytic degradation rate was indeed increased with increasing in IA content. The weight loss, observed in Fig. 5, was in agreement with the conclusion mentioned above.
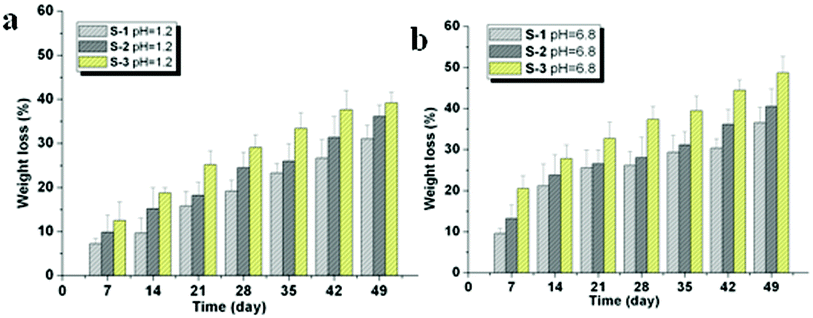 |
| Fig. 5
In vitro hydrolytic degradation behavior of hydrogels. (a) Mass loss of the heatcrosslinked P(LE-IA-MEG) hydrogel incubated in pH 1.2 for 49 days. (b) Mass loss of the heatcrosslinked P(LE-IA-MEG) hydrogel incubated in PBS (pH 6.8, 37 °C) for up to 49 days. | |
3.5
In vitro drug release from the P(LE-IA-MEG) hydrogel
3.5.1 Release of hydrophilic small-molecular-weight drugs.
In vitro release of VB12 from the VB12/P(LE-IA-MEG) hydrogel at pH 1.2 and 6.8 are shown in Fig. 6a. As shown in Fig. 6a, VB12 was released from VB12/P(LE-IA-MEG) for a determined period of time. It is clear that the drug release rate at pH 1.2 is more slow than that at pH 6.8. At lower pH (pH 1.2), an initial burst release of 21.4% from the VB12 loaded hydrogel occurred in the first 1 h, followed by the release of 65.2% in four days, whereas at higher pH (pH 6.8), the cumulative release rates for 1 h and 4 days were 32.7% and 92.9%, respectively.
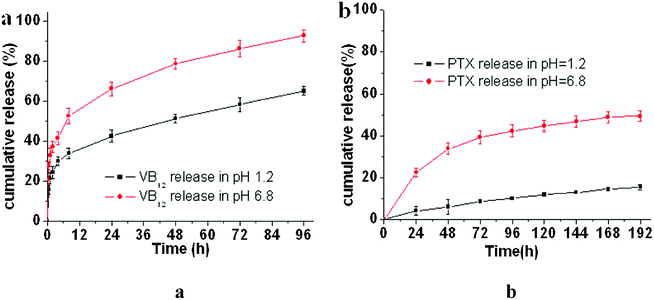 |
| Fig. 6
In vitro release behavior of VB12 (a) and PTX (b) from drug/P(LE-IA-MEG) hydrogel complexes at different pH values of 1.2 and 6.8. Results are the mean value ± SD of 3–4 independent experiments. | |
3.5.2 Release of hydrophobic small-molecular-weight drugs.
In vitro release of PTX from the PTX/P(LE-IA-MEG) hydrogel in PBS is shown in Fig. 6b. According to Fig. 6b, PTX was released from this hydrogel for a sustained period of time. From Fig. 6b, we could find that pH value has a great effect on the release behavior of PTX from this hydrogel. It is clear that the cumulative release rate of PTX increased dramatically from 15.39% (pH 1.2) to 49.46% (pH 6.8) in an 8-day period. The burst releases of PTX were approximately 4.19% and 22.42% in the first 24 h. After that, PTX could be released steadily from the hydrogel, which finally reached 15.39% and 49.46% of cumulative drug release rates in next 7 days.
In vitro drug release behavior of the P(LE-IA-MEG) hydrogel at different pH (pH 1.2 and 6.8) was investigated and its cumulative release profile is displayed in Fig. 6. From Fig. 6, we could find that pH value has a great effect on the release behavior of drugs, including hydrophilic and hydrophobic drugs, from the hydrogel. In neutral medium (pH 6.8), either PTX or VB12 was released faster, and the cumulative release was higher than that in acidic medium. The hydrophilic drug was almost completely released from the P(LE-IA-MEG) hydrogel in a few days with a high release rate (>90% in 96 h) and a high initial burst rate (about 21.4% in 1 h). Conversely, the hydrophobic drug could be released slowly in a longer period with a lower cumulative release rate. Generally, drug release behavior from the hydrogel was driven by two forces: diffusion effect and degradation or erosion of the hydrogel.32 For hydrophobic drugs, a low diffusion rate in water and strong intermolecular interactions with the hydrogel dominated the drug release profile, which resulted in a lower release rate. For the pH-sensitive hydrogel, the permeability and release rate of the drugs was influenced by the type of releasing agent and the water content in the hydrogel. At pH 1.2, carboxylic acid groups are protonated, thus the network de-swells, leading to the lowest swelling ratio. As a result, the drug release from the hydrogel network is strongly retarded. When the pH value is further increased to 6.8, the carboxylic acid groups from the hydrogels become progressively ionized. Meanwhile, the hydrogel swells more significantly owing to a large swelling force created by the electrostatic repulsion among the ionized groups. It causes the large water content of the hydrogel network, which leads to the fast release of drug. In this study, swelling behavior has been investigated, and we could find a larger water content absorption at high pH values than that at low pH values. In vitro drug release behavior from the P(LE-IA-MEG) hydrogel at different pH values is presented in Fig. 6.
Conclusions
A series of pH-sensitive P(LE-IA-MEG) hydrogels with no organic solvent were successfully prepared by a low temperature, heat-initiated, free radical polymerization method. This hydrogel is biodegradable and has apparent pH responsiveness. The swelling ratio increased accordingly when the pH value changed from 1.2 to 6.8. In addition, dynamic swelling/de-swelling behavior has been investigated in this study. In vitro drug release behavior, including hydrophilic and hydrophobic drugs, was studied in detail. Owing to the convenient and solvent free method of preparation of this hydrogel, and its great pH-sensitivity, it would be beneficial for some drugs delivery systems, and has an especially large potential application in the oral administration of protein and peptide drugs.
Acknowledgements
This work was financially supported by National Science Foundation of China (81173024).
References
- B. Vkquez, M. Gurruchaga, I. GoAi and E. Narvarte, Polymer, 1995, 36, 3327–3333 CrossRef.
- H. Chaoliang, K. Sung Wan and L. Doo Sung., J. Controlled Release, 2008, 127, 189–207 CrossRef.
- T. Qunwei, W. Jihuai and L. Jianming, Carbohydr. Polym., 2008, 73, 315–321 CrossRef.
- I. Francesca, S. U. Gianfranco, P. Francesco, M. Rita, T. Sonia, C. Roberta, L. Sonia and P. Nevio, Int. J. Pharm., 2006, 312, 151–157 CrossRef.
- X. Yuling, X. Wenjin, Z. Qifeng, Y. Bingfang, Y. Dongfang, Y. Jin, H. Xianran, L. Shucai and H. Xianming, Carbohydr. Polym., 2009, 77, 612–620 CrossRef.
- Y. Yumei, S. Xiang and W. Pixin, Eur. Polym. J., 2009, 45, 309–315 CrossRef.
- I. Colinet, V. Dulong, G. Mocanu, L. Picton and D. Le Cerf., Eur. J. Pharm. Biopharm., 2009, 73, 345–350 CrossRef CAS.
- G. Baolin and G. Qingyu, Carbohydr. Res., 2007, 342, 2416–2422 CrossRef.
- L. Yuyang, F. W. Xiaodong, Borong, S. Qingfa, C. Weixing and S. Le, Int. J. Pharm., 2006, 308, 205–209 CrossRef.
- W. Ke, X. Xu, L. TingTing, F. ShaoZhi, G. Gang, G. YingChun, L. Feng, Z. Xia, W. YuQuan and Q. ZhiYong, Carbohydr. Polym., 2010, 79, 755–761 CrossRef.
- K. Bumsang and P. Nicholas, Polymer, 2003, 44, 3701–3707 CrossRef.
- Z. Yeli and C. Chih Chang, J. Biomed. Mater. Res., 2001, 54, 1–11 CrossRef.
- M. Takashi, U. Tadashi and N. Katsuhiko, Adv. Drug Delivery Rev., 2002, 54, 79–98 CrossRef.
- H. C. Chiu, A. T. Wu and Y. F. Lin, Polymer, 2001, 42, 1471–1479 CrossRef CAS.
- J. E. Elliott, M. Macdonald, J. Nie and C. N. Bowman, Polymer, 2004, 45, 1503–1510 CrossRef CAS.
- S. Horiike, K. Yumoto, S. Matsuzawa and K. Yamaura, Polym. Adv. Technol., 2003, 14, 422–427 CrossRef CAS.
- L. Changhua, C. Yanqing and C. Jianguang, Carbohydr. Polym., 2010, 79, 500–506 CrossRef.
- N. C. Kalarickal, S. Rimmer, P. Sarker and J. C. Leroux, Macromolecules, 2007, 40, 1874–1880 CrossRef CAS.
- W. J. E. M. Habraken, J. G. C. Wolke and J. A. Jansen, Adv. Drug Delivery Rev., 2007, 59, 234–248 CrossRef CAS.
- M. Sasatsu, H. Onishi and Y. Machida, Int. J. Pharm., 2005, 294, 233–245 CrossRef CAS.
- L. Feng, L. Suming and M. Vert, Macromol. Biosci. DOI:10.1002/mabi.200500143.
- Z. XiuLing, K. Bing, G. MaLing, F. ShaoZhi, Z. Juan, M. Ke, C. LiJuan, L. Feng, Z. YingLan, Z. Xia, W. YuQuan and Q. ZhiYong, Int. J. Pharm., 2010, 386, 262–267 CrossRef.
- L. YuYang, F. XiaoDong, W. BoRong, S. QingFa, C. WeiXing and S. Le, Int. J. Pharm., 2006, 308, 205–209 CrossRef.
- W. Ke, X. Xu, W. YuJun, Y. Xi, G. Gang, H. MeiJuan, L. Feng, Z. Xia, W. YuQuan and Q. ZhiYong, Int. J. Pharm., 2010, 389, 130–138 CrossRef.
- C. GuoTao, Q. ZhiYong, H. MeiJuan, K. Bin, G. YingChun, G. ChangYang, Y. JinLiang, W. Ke, D. Mei, L. XingYi, G. MaLing, T. MingJing and W. YuQuan, J Biomed Mater Res A, 2008, 85(A), 36–46 Search PubMed.
- J. Wen Juan, L. Jian Gang, Z. Yang De, W. Ji Wei and J. Wang, J. Drug Targeting, 2007, 15, 140–145 CrossRef.
- H. Yihong, Y. Huiqun and X. Chaobo, Carbohydr. Polym., 2007, 69, 774–783 CrossRef.
- S. K. Bajpai and S. Singh, React. Funct. Polym., 2006, 66, 431–440 CrossRef CAS.
- L. Serra, J. Doménech and N. A. Peppas, Eur. J. Pharm. Biopharm., 2006, 63, 11–18 CrossRef CAS.
- L. E. Bromberg and E. S. Ron, Adv. Drug Delivery Rev., 1998, 31, 197–221 CrossRef CAS.
- M. Malin, M. Hiljanen-Vainio, T. Karjalainen and J. Seppälä, J. Appl. Polym. Sci., 1996, 59, 1289–1298 CrossRef CAS.
- G. Changyang, S. Shuai, D. Pengwei, K. Bing, G. Maling, W. Xianhuo, L. Xingyi, L. Feng, Z. Xia and W. Yuquan, Int. J. Pharm., 2009, 365, 89–99 CrossRef.
|
This journal is © The Royal Society of Chemistry 2012 |