DOI:
10.1039/C2RA01118B
(Paper)
RSC Adv., 2012,
2, 2493-2497
Synthesis, characterization and cytotoxicity study of magnetic (Fe3O4) nanoparticles and their drug conjugate
Received
17th November 2011
, Accepted 11th December 2011
First published on 2nd February 2012
Introduction
There has been steady advancement of nanoscience in the biomedical field over recent years.1 Considerable research has been aimed at achieving biocompatible, uniformly sized, high surface area and stable nanoparticles that can be used as diagnostic tools for various therapeutic applications.2 In recent times much attention has been focused on magnetic iron oxide nanoparticles (IONPs).3 Magnetic IONPs have potential application as magnetic drug carriers,4 magnetic resonance imaging (MRI)5 agents etc. However, in order to keep medical expenses low, diagnostic tools should be very cheap in price. Therefore it is necessary to have a simple, easily reproducible synthetic route to produce Fe3O4 nanoparticles that are specific to a target. In this paper we wish to disclose our initial results on the synthesis of magnetic IONPs, their uniformly dispersed surface area, loading of an anticancer drug on them and finally their cytotoxicity studies. Chemical co-precipitation has been employed as a cheap and convenient method for the preparation of IONPs. 5-Fluorouracil (5-FU) has been used as the only cytotoxic agent. Transmission electron microscopy and atomic force microscopy have been used to study the morphology of the uniform surface areas of the IONPs. FTIR studies have confirmed the loading of 5-FU onto the IONPs. Finally cell viability was measured by incubating IONPs and their drug conjugate using a MTT assay.
Experimental section
1 g (0.005 mol) FeCl2·4H2O was dissolved in 20 ml 1 (M) HCl and then 2.7 g (0.01 mol) FeCl3·6H2O was added to the solution before stirring for 30 min. Then 2 (M) NaOH solution was added until the pH became basic; immediately a black precipitate appeared. This black precipitate was then separated and washed four times with 20 ml of deionized and deoxygenated water. Then 20 ml of citric acid–sodium citrate buffer was added to disperse the Fe3O4 nanoparticles. Before conjugation with 5-FU the IONPs were washed by repeated cycles (3 times) of centrifugation and redispersion with deionized and deoxygenated water to remove citrate completely. Finally the IONPs were redispersed in deionized water under strong stirring conditions for another 30 min.
Synthesis of Fe3O4 nanoparticle–5-fluorouracil conjugates
For the conjugation of 5-fluorouracil (5-FU) onto nanoparticles: Fe3O4 nanoparticles (IONPs) and 5-FU in the ratio 1
:
1, were mixed (5.7 mg of 5-FU in 1 ml HPLC grade methanol was added to 5 ml of Fe3O4 nanoparticle solution). Then the mixture was stirred at 60 °C for 20 h. The unbound 5-FU was separated by centrifugation at 30,000 rpm for 30 min. A solid appeared and it was further washed 3 times with HPLC grade water and methanol and dried under vacuum. The 5-FU conjugated Fe3O4 nanoparticles were characterized by FTIR spectroscopy and AFM analysis.
Results and discussion
TEM
sample preparation and imaging7.
For TEM imaging, the sample was placed on a 300-mesh carbon coated copper grid (Allied Scientific Product, USA) (5 μl, 15 min) and excess sample was removed cautiously using tissue paper. It was finally dried and the images were recorded on a Tecnai G2 Spirit Bio TWIN (Type: FP5018/40) at an acceleration voltage of 80 kV. TEM images of the IONPs (Fig. 1 A and B) show that the size of the NPs is almost uniform in nature and most of them are approximately spherical with diameter 6 nm.
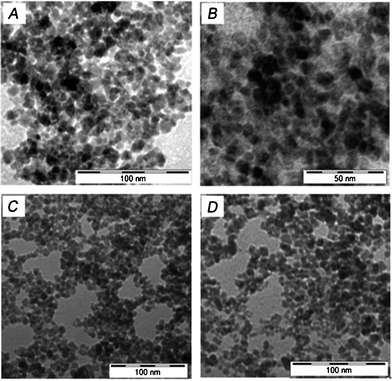 |
| Fig. 1 A and B show TEM images of IONPs; C and D show TEM images of 5-FU tagged IONPs. | |
AFM
sample preparation and imaging8.
For the AFM imaging of IONP samples, 10 μl of the sample were deposited onto freshly cleaved muscovite Ruby mica sheets (ASTM V1 Grade Ruby Mica from MICAFAB, Chennai) for 5–10 min. Mica sheets are basically negatively charged so Fe3O4 molecules bind strongly on the mica surface; after 5 min the sample was dried by using a vacuum dryer. AAC mode AFM was performed using a Pico plus 5500 AFM (Agilent Technologies USA) with a piezoscanner with a maximum range of 9 μm. Micro-fabricated silicon cantilevers of 225 μm in length with a nominal spring force constant of 21–98 N m−1 were used from Nano sensors. The cantilever oscillation frequency was tuned into the resonance frequency. The cantilever resonance frequency was 150–300 kHz. The images (512 × 512 pixels) were captured with a scan size of between 0.5 and 5 μm at a scan speed rate of 0.5 rpm. Images were processed by flattening, using Pico view software (Molecular Imaging Corporation, USA). All the images presented in this report were derived from the original data. Length, height and width of the nanomaterials were measured manually using Pico view software. In the AFM images (Fig. 2) the IONPs are nearly spherical with 6 nm diameter and having a tendency to aglomerization, whereas 5-FU conjugated Fe3O4 nanoparticles are oval shaped with nearly 10 nm diameter. It has been also observed that IONPs are prone to quick aglomerization. But to the contrary the 5-FU coated NPs are stable to coagulation because of their lack of free reactive surface for aglomerization.
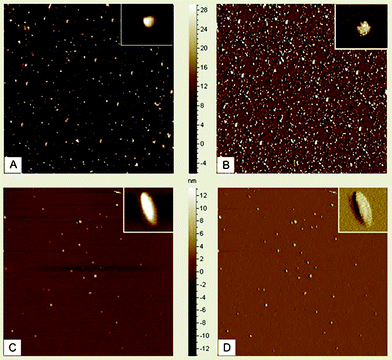 |
| Fig. 2 A and B show AFM images of IONPs; C and D show AFM images of 5-FU tagged IONPs. Inset pictures show enlarged views of IONPs and 5-FU tagged IONPs. | |
FT-IR experiment.
The FT-IR spectra of the samples were recorded on a JASCO FT/IR 4200 spectrometer using the (KBr) disc technique. The drug (5-FU/5-FU conjugated IONPs) was mixed with KBr in a clean glass pestle and mortar and compressed to obtain a pellet. The spectra were recorded from 400–4000 cm−1. Background spectra were obtained with a KBr pellet for each sample. JASCO software was used for data processing.
Dynamic light scattering (DLS)-based zeta-potential measurements10.
To obtain an idea about the size distributions of IONPs and 5-FU tagged IONPs, DLS experiments were carried out with their aqueous solutions and the results showed that the mean particle size of IONPs is 8.19 nm and of 5-FU-conjugated IONPs is 9.98 nm. Fig. 5 presents the zeta potential distributions of IONPs with a negative charge −39.1 mV and of 5-FU-conjugated IONPs with a negative charge −27.5 mV in water, which is sufficient to keep the particles from interacting with each other and therefore maintain a stable particle size of the sample. The resulting negative charges in IONPs and 5-FU tagged IONPs are attributed to negative surface charge in both IONPs and 5-FU tagged IONPs.
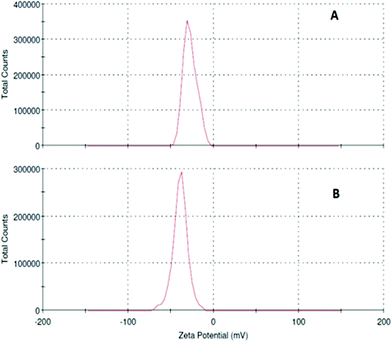 |
| Fig. 5 Zeta potentials of (A) 5-FU tagged IONPs and (B) IONPs. | |
X-Ray diffraction study11.
X-Ray diffraction studies of both IONPs and 5-FU tagged IONPs were carried out with a Scifert X-ray diffractometer (C 3000) using ‘Cu kα’ radiation. Fig.6 shows the X-ray diffraction patterns of IONPs and 5-FU tagged IONPs. The parameters of IONPs and 5-FU tagged IONPs, such as the positions and values of peaks were very close to those of the standard data of Fe3O4 alone in powder diffraction PDF card (JCPDS No.82n1533). The appearance of sample diffraction peaks at 2θ = 30.16°, 35.70°, 43.33°, 53.60°, 57.10°, and 62.9° corresponded to the (220), (311), (400), (422), (511) and (440) crystal planes of Fe3O4 respectively, which indicated that the resulting particles were Fe3O4, with structures of cubic crystal. From the X-ray diffraction data the calculated value of the lattice parameter, a0 = 8.3951 Å, which indicates that it is magnetite in nature.6a
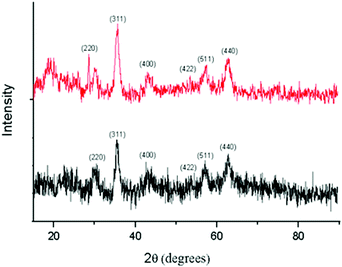 |
| Fig. 6
XRD patterns of IONPs (black) and 5-FU tagged IONPs (red). | |
BET surface area measurement12.
The surface area of IONPs was calculated by the BET method, assuming the particles to be solid with a smooth surface and of the same size. The BET surface area, SBET (in m2 g−1) = 6000/(ρXD), where D is the average diameter of the particles in nanometres; SBET represents the measured surface area of the powder; ρ is the theoretical density in g cm−3. The value of the BET surface area of the IONPs is 144 m2 g−1, considering the average equivalent particle size to be 8.1 nm (from DLS experiments).
CHN analysis
.
The chemical composition of 5-FU using CHN analysis reveals that it contains 36.44% carbon (36.93%), 2.09% hydrogen (2.32%), and 21.03% nitrogen (21.54%) where the figures in brackets represent the theoretical composition. And the chemical composition of 5-FU tagged IONPs using CHN analysis reveals that they contain 34.93% carbon, 2.04% hydrogen, and 20.01% nitrogen. This indicates that 5-FU tagged IONPs do not contain any citrate buffer.
Cell culture13.
Human hepatocellular carcinoma cell line HepG2 procured from the National Centre for Cell Sciences (NCCS, Pune, India) was grown in Dulbecco's modified Eagle medium (DMEM, Invitrogen, Life Technologies, USA) supplemented with 10% fetal bovine serum (FBS) and antibiotics (penicillin/streptomycin and gentamicin). Cells were cultured at 37 °C in 95% air and 5% CO2 humidified incubators. HepG2 cells were seeded at a density of 105/well plated in 96 well plates. Cells were typically grown to 60–70% confluence, rinsed in phosphate-buffered saline (PBS) and placed into serum-free medium overnight prior to treatments. After overnight incubation, the HepG2 cells were treated with IONPs, 5-FU and 5-FU tagged IONPs separately and cell viability was recorded by MTT assay.
Survival assay.
Viable cells were evaluated by MTT (3-(4,5-dimethylthiazol-2-yl)-2,5-diphenyltetrazolium bromide) assay, where the viability of cells was determined by the reduction of the yellow MTT into purple formazan product by mitochondrial dehydrogenase present in metabolically active cells. Cultured primary HepG2 were treated with IONPs, 5-FU and 5-FU tagged IONPs separately (1 μM, 2 μM, 4 μM and 6μM) for 24 h. After treatment the medium was removed and 50 μl of fresh medium was added along with 10 μl of MTT (5 mg ml−1). After 4 h, the MTT solution was slowly removed and the purple crystals were solubilised in 1.4 ml of DMSO. The absorbance was measured at a test wavelength of 590 nm in an Elisa plate reader. The absorbances obtained from treated cells were expressed as percentages of absorbance obtained from untreated cells and are reported as mean ± Sd (n = 2). Fig. 7 shows the cell viability after incubation of the control and with different concentrations of IONPs, 5-FU and 5-FU tagged IONPs. It was observed that IONPs did not induce any significant change in the proliferation with a concentration up to 6 μM with respect to the control, suggesting the absence of toxicity of the IONPs.
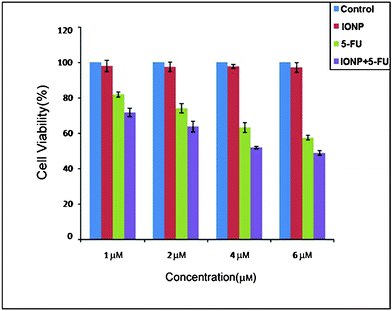 |
| Fig. 7 Cytotoxicity of control, IONPs, 5-FU and 5-FU tagged IONPs with different concentrations after 24 h incubation. | |
Subsequently the proliferation of HepG2 cells reduced significantly with 5-FU tagged IONPs and, at a dose of 6 μM, the cell proliferation was reduced by 50%. It is also noted that in all the concentrations the inhibition of cell proliferation by 5-FU tagged IONPs is more than that of 5-FU itself. This strongly indicates that when 5-FU is tagged with IONPs, it acts as a more potent anticancer drug than 5-FU alone.
Conclusion
This paper depicts a simple and convenient method to prepare biofunctionalized, highly water-soluble 5-FU tagged IONPs with uniform size. The synthetic method is cost effective, easy to scale up and reproducible. The presence of the large surface area of the nanoparticles facilitates incorporation of a substantial amount of anticancer drug (5-fluorouracil). Moreover we have shown that IONPs are non-toxic and highly biocompatible in nature. Thus the present study will serve as a first step towards attaching various bioactive agents such as anticancer drugs, biomarkersetc. and studying their properties and therapeutic potentials as well. As Fe3O4 nanoparticles are magnetic so they will have potential applications as a contrast enhancing agent in MRI and an active agent in drug delivery which we plan to study in the future.
Acknowledgements
S. P. thanks CSIR, New Delhi, India, for a project assistantship, S. M. thanks UGC, India, for financial support. We would also like to thank CSIR, India, for providing a research grant through the Network-project, NWP0035. The authors would also like to thank Dr Aparna Laskar and Mr Murganandan for recording TEM and AFM images respectively.
References
-
(a) S. Palchoudhury, W. An, Y. Xu, Y. Qin, Z. Zhang, N. Chopra, R. A. Holler, C. H. Turner and W. Bao, Synthesis and Growth Mechanism of Iron Oxide Nanowhiskers, Nano Lett., 2011, 11, 1141–1146 CrossRef CAS;
(b) C. R. Thomas, D. P. Ferris, J. H. Lee, E. Choi, M. H. Cho, E. S. Kim, J. F. Stoddart, J. S. Shin and J. I. J. C. Zink, Noninvasive Remote-Controlled Release of Drug Molecules In Vitro Using Magnetic Actuation of Mechanized Nanoparticles, J. Am. Chem. Soc., 2010, 132, 10623–10625 CrossRef CAS;
(c) E. Vucic, H. M. H. F. Sanders, F. Arena, E. Terreno, S. Aime, K. Nicolay, E. Leupold, M. Dathe, N. A. J. M. Sommerdijk, Z. A. Fayad and W. J. M. Mulder, Well-Defined, Multifunctional Nanostructures of a Paramagnetic Lipid and a Lipopeptide for Macrophage Imaging, J. Am. Chem. Soc., 2009, 131, 406–407 CrossRef CAS;
(d) P. Howes, M. Green, A. Bowers, D. Parker, G. Varma, M. Kallumadil, M. Hughes, A. Warley, A. Brain and R. Botnar, Magnetic Conjugated Polymer Nanoparticles as Bimodal Imaging Agents, J. Am. Chem. Soc., 2010, 132, 9833–9842 CrossRef CAS.
-
(a) M. Babi, D. Horák, M. Trchova, P. Jendelova, K. GlogarovA, P. Lesny, V. Herynek, M. Hájek and E. Sykova, Poly(L-lysine)-Modified Iron Oxide Nanoparticles for Stem Cell Labeling, Bioconjugate Chem., 2008, 19, 740–750 CrossRef;
(b) M. S. Bhojani, M. V. Dort, A. Rehemtulla and B. D. Ross, Targeted Imaging and Therapy of Brain Cancer Using Theranostic Nanoparticles, Mol. Pharmaceutics, 2010, 7, 1921–1929 CrossRef CAS;
(c) L. Berti, T. Woldeyesus, Y. Li and K. S. Lam, Maximization of Loading and Stability of ssDNA:Iron Oxide Nanoparticle Complexes Formed through Electrostatic Interaction, Langmuir, 2010, 26, 18293–18299 CrossRef CAS;
(d) D. Horák, M. Babič, P. Jendelová, V. Herynek, M. Trchová, Z. Pientka, E. Pollert, M. Hájek and E. Syková,
D-Mannose-Modified Iron Oxide Nanoparticles for Stem Cell Labeling, Bioconjugate Chem., 2007, 18, 635–644 CrossRef;
(e) M. Mahmoudi, M. A. Sahraian, M. A. Shokrgozar and S. Laurent, Superparamagnetic Iron Oxide Nanoparticles: Promises for Diagnosis and Treatment of Multiple Sclerosis, ACS Chem. Neurosci., 2011, 2, 118–140 CrossRef CAS.
-
(a)
J. Quinn, D. Elliott, O. S. Hara and A. Billow, Use of Nanoscale Iron and Bimetallic Particles for Environmental Remediation: A Review of Field-Scale Applications. Environmental Applications of Nanoscale and Microscale Reactive Metal Particles, 2010, ch. 15, pp. 263–283 Search PubMed;
(b) E. S. Bowles, W. Wu, T. Kowalewski, M. C. Schalnat, R. J. Davis, J. E. Pemberton, I. Shim, B. D. Korth and J. Pyun, Magnetic Assembly and Pyrolysis of Functional Ferromagnetic Colloids into One-Dimensional Carbon Nanostructures, J. Am. Chem. Soc., 2007, 129, 8694–8695 CrossRef;
(c) R. J. Hickey, A. S. Haynes, J. M. Kikkawa and S. J. Park, Controlling the Self-Assembly Structure of Magnetic Nanoparticles and Amphiphilic Block-Copolymers: From Micelles to Vesicles, J. Am. Chem. Soc., 2011, 133, 1517–1525 CrossRef CAS;
(d) N. G. Chalkias, P. Kahawong and E. P. Giannelis, Activity Increase of Horseradish Peroxidase in the Presence of Magnetic Particles, J. Am. Chem. Soc., 2008, 130, 2910–2911 CrossRef CAS;
(e) W. Wang, Y. Xu, D. I. C. Wang and Z. Li, Recyclable Nanobiocatalyst for Enantioselective Sulfoxidation: Facile Fabrication and High Performance of Chloroperoxidase-Coated Magnetic Nanoparticles
with Iron Oxide Core and Polymer Shell, J. Am. Chem. Soc., 2009, 131, 12892–12893 CrossRef CAS;
(f) G. A. Sotiriou, A. M. Hirt, P. Y. Lozach, A. Teleki, F. Krumeich and S. E. Pratsinis, Hybrid, Silica-Coated, Janus-Like Plasmonic-Magnetic Nanoparticles, Chem. Mater., 2011, 23, 1985–1992 CrossRef CAS.
-
(a)
J. Huang, P. Yao, A. Zhao, C. Kang, P. Pu and J. Chang, Construction of Multifunctional DDS:Transferrin-Mediated Tat and Drug-Loaded Magnetic Nanoparticles. New Delivery Systems for Controlled Drug Release from Naturally Occurring Materials, 2008, ch. 9, pp. 153–173 Search PubMed;
(b) J. L. Arias, L. H. Reddy, M. Othman, B. Gillet, D. Desmale, F. Zouhiri, F. Dosio, R. Gref and P. Couvreur, Squalene Based Nanocomposites: A New Platform for the Design of Multifunctional Pharmaceutical Theragnostics, ACS Nano, 2011, 5, 1513–1521 CrossRef CAS;
(c) P. S. Williams, F. Carpino and M. Zborowski, Magnetic Nanoparticle Drug Carriers and Their Study by Quadrupole Magnetic Field-Flow Fractionation, Mol. Pharmaceutics, 2009, 6, 1290–1306 CrossRef CAS;
(d) N. Kohler, C. Sun, J. Wang and M. Zhang, Methotrexate-Modified Superparamagnetic Nanoparticles and Their Intracellular Uptake into Human Cancer Cells, Langmuir, 2005, 21, 8858–8864 CrossRef CAS.
-
(a) L. L. Ma, M. D. Feldman, J. M. Tam, A. S. Paranjape, K. K. Cheruku, T. A. Larson, J. O. Tam, D. R. Ingram, V. Paramita, J. W. Villard, J. T. Jenkins, T. Wang, G. D. Clarke, R. Asmis, K. Sokolov, B. Chandrasekar, T. E. Milner and K. P. Johnston, Small Multifunctional Nanoclusters (Nanoroses) for Targeted Cellular Imaging and Therapy, ACS Nano, 2009, 3, 2686–2696 CrossRef CAS;
(b) Z. Ali, A. Z. Abbasi, F. Zhang, P. Arosio, A. Lascialfari, M. F. Casula, A. Wenk, W. Kreyling, R. Plapper, M. Seidel, R. Niessner, J. Knoll, A. Seubert and W. J. Parak, Multifunctional Nanoparticles for Dual Imaging, Anal. Chem., 2011, 83, 2877–2882 CrossRef CAS;
(c) F. Erogbogbo, K. T. Yong, R. Hu, W. C. Law, H. Ding, C. W. Chang, P. N. Prasad and M. T. Swihart, Biocompatible Magnetofluorescent Probes: Luminescent Silicon Quantum Dots Coupled with Superparamagnetic Iron(III) Oxide, ACS Nano, 2010, 4, 5131–5138 CrossRef CAS;
(d)
G. M. Lanza, P. M. Winter, M. S. Hughes, S. D. Caruthers, J. N. Marsh, A. M. Morawski, A. H. Schmieder, M. J. Scott, R. W. Fuhrhop, H. Zhang, G. Hu, E. K. Lacy, J. S. Allen and S. A. Wickline, Molecular Imaging and Therapy: New Paradigms for 21st Century Medicine Polymeric Drug Delivery I, 2006, ch. 21, pp. 295–311 Search PubMed.
-
(a) J. Sun, S. Zhou, P. Hou, Y. Yang, J. Weng, X. Li and M. Li, Synthesis and Characterization of Biocompatible Fe3O4 Nanoparticles, J. Biomed. Mater. Res., Part A, 2006, 80, 333–341 Search PubMed;
(b) H. Zeng and S. Sun, Syntheses, Properties, and Potential Applications of Multicomponent Magnetic Nanoparticles, Adv. Funct. Mater., 2008, 18, 391–400 CrossRef CAS;
(c) J. Xie, K. Chen, H. Y. Lee, C. Xu, A. R. Hsu, S. Peng, X. Chen and S. Sun, Ultrasmall c(RGDyK)-Coated Fe3O4 Nanoparticles and Their Specific Targeting to Integrin αvβ3-Rich Tumor Cells, J. Am. Chem. Soc., 2008, 130, 7542–7543 CrossRef CAS.
- S. Guha, M. G. B. Drew and A. Arindam Banerjee, Dipeptide Nanotubes, with N-Terminally Located ω-Amino Acid Residues, That are Stable Proteolytically, Thermally, and Over a Wide Range of pH, Chem. Mater., 2008, 20, 2282–2290 CrossRef CAS.
- T. Atanasijevic, M. Shusteff, P. Fam and A. Jasanoff, Calcium-sensitive MRI Contrast Agents Based on Superparamagnetic Iron Oxide Nanoparticles and Calmodulin, Proc. Natl. Acad. Sci. U. S. A., 2006, 103, 14707–14712 CrossRef CAS.
-
(a)
S. M. Baomi, A. A. Al-badar, Fluorouracil, in Analytical Profiles of Drug Substances, ed. K. Florey, Elsevier, New Delhi, 2005, vol. 18, pp. 599–632 Search PubMed;
(b) Y. Tsuchiya, T. Tamura, M. Fujii and M. Ito, J. Phys. Chem., 1988, 92, 1760–1765 CrossRef CAS.
- C. Chun, J. Joo, D. Kwon, C. S. Sup Kim, H. J. Cha, M-S. Chung and S. Jeon, Chem. Commun., 2011, 47, 11047–11049 RSC.
- D. Fu, P. G. Keech, X. Sun and J. C. Wren, Phys. Chem. Chem. Phys., 2011, 13, 18523–18529 RSC.
- H. Qiao, Z. Wei, H. Yang, L. Zhu and X. Yan, Preparation and Characterization of NiO Nanoparticles by Anodic Arc Plasma Method, Journal of Nanomaterials, 2009 DOI:10.1155/2009/795928 , 5 pages.
-
(a) J. G. Mabley, W. L. Suarez-Pinzon, G. Hasko, A. L. Salzman, A. Rabinovitch, E. Kun and C. Szabo, Inhibition of Poly(ADPribose) Synthetase by Gene Disruption or Inhibition with 5-Iodo-6-amino-1,2-benzopyrone Protects Mice from Multiple Low-Dose Streptozotocin-Induced Diabetes, Br. J. Pharmacol., 2001, 133, 909–919 CrossRef CAS;
(b) Y. Zhang, J. S. Li, M. Lang, X. Tang, L. Li and X. Shen, Folate-Functionalized Nanoparticles for Controlled 5-Fluorouracil Delivery, J. Colloid Interface Sci., 2011, 354, 202–209 CrossRef CAS.
|
This journal is © The Royal Society of Chemistry 2012 |
Click here to see how this site uses Cookies. View our privacy policy here.