DOI:
10.1039/C2RA01056A
(Review Article)
RSC Adv., 2012,
2, 4547-4592
Recent developments in solvent-free multicomponent reactions: a perfect synergy for eco-compatible organic synthesis
Received
10th November 2011
, Accepted 23rd January 2012
First published on 7th March 2012
Abstract
Multicomponent reactions have gained significant importance as a tool for the synthesis of a wide variety of useful compounds, including pharmaceuticals. In this context, the multiple component approach is especially appealing in view of the fact that products are formed in a single step, and the diversity can be readily achieved simply by varying the reacting components. The eco-friendly, solvent-free multicomponent approach opens up numerous possibilities for conducting rapid organic synthesis and functional group transformations more efficiently. Additionally, there are distinct advantages of these solvent-free protocols since they provide reduction or elimination of solvents thereby preventing pollution in organic synthesis “at source”. The chemo-, regio- or stereoselective synthesis of high-value chemical entities and parallel synthesis to generate a library of small molecules will add to the growth of multicomponent solvent-free reactions in the near future. In this review we summarized the results reported mainly within the last 10 years. It is quite clear from the growing number of emerging publications in this field that the possibility to utilize multicomponent technology allows reaction conditions to be accessed that are very valuable for organic synthesis. Therefore, diversity oriented synthesis (DOS) is rapidly becoming one of the paradigms in the process of modern drug discovery. This has spurred research in those fields of chemical investigation that lead to the rapid assembly of not only molecular diversity, but also molecular complexity. As a consequence multi-component as well as domino or related reactions are witnessing a new spring.
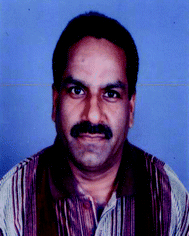 Maya Shankar Singh | Maya Shankar Singh was born in 1960 and earned his M. Sc. and Ph. D. degrees in Organic Chemistry from Banaras Hindu University, Varanasi, India in 1981 and 1986, respectively. After postdoctoral research, he joined the Vikram University Ujjain, as Assistant Professor in 1990, and moved to Gorakhpur University as Associate Professor in 1998, then to Banaras Hindu University in 2004, where he is currently Professor in Organic Chemistry since 2006. During his sabbatical, he visited University of Arizona, Tucson, USA and Nagoya Institute of Technology, Nagoya, Japan. His research interests are the development of novel building blocks, new synthetic methods, multicomponent reactions, and structural studies. 14 students have completed their Ph. D. degrees under his supervision and presently 12 are working. His research work has been documented in 100 papers in reputed journals. Additionally, he has authored two textbooks in organic chemistry published from Pearson-Education. |
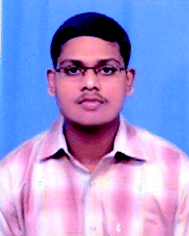 Sushobhan Chowdhury | Sushobhan Chowdhury was born in South 24 Parganas, West Bengal, India, in 1985. He received his B. Sc. degree from Ramkrishna Mission Residential College, Kolkata in 2007 and M. Sc. degree from Banaras Hindu University, Varanasi in 2010. Currently, he is pursuing his Ph. D. under the supervision of Professor Maya Shankar Singh at Banaras Hindu University. His major research interest is to develop new synthetic methodologies through multicomponent reactions to synthesize bioactive moieties. |
1 Introduction
The chemistry of organic synthesis attracts particular attention in science fundamentally because of its immense importance to life. The extreme flexibility of bonding at carbon is well-suited to the wide variety of structures required to support living systems. Organic synthesis is one of the most important and useful disciplines of chemistry, which mainly involves the construction and cleavage of carbon–carbon (C–C) and carbon–heteroatom (C–X) bond(s). The synthetic strategies regarding how to construct and cleave the above bonds represent the central theme in organic synthesis. Due to tremendous impact of the chemical industry in our society, there is constant pressure to reduce costs and consumable resources to have less detrimental impact to the environment. Present day organic synthesis requires and even demands certain regulations and guidelines in developing any operationally simple, useful, and practical strategy, keeping the protection of the environment as a major concern. The classical ways to improve synthetic reactions are either to invent a new reagent, to invent a new catalyst or to elucidate details about the mechanism that in turn enables one to judiciously modify conditions to increase the reaction's rate and/or yield. The continual upsurge in facile and non-polluting synthetic procedures urges chemists to increase tools of their arsenal. One approach to address this challenge involves the development of eco-compatible multicomponent procedures in organic synthesis.
The literature on multicomponent reactions (MCRs) has experienced exponential growth over the last decade and the literature of MCRs under solvent-free conditions has expanded enormously, making it very difficult to keep up with the research reported in this field. Therefore, review literature has become increasingly important to researchers who are trying to keep abreast of this field. The available reviews in the literature on solvent-free synthesis and/or multicomponent reactions deal mainly with the synthesis of certain categories of organic compounds. Yet no review in the literature is available covering the wide areas of organic compounds such as acyclic, carbocyclic, and heterocyclic through a multicomponent solvent-free approach. Thus, considering the lack of such a review in the literature and in continuation of our efforts on more sustainable syntheses under solvent-free conditions, here an overview of the open literature on the development of more sustainable methodologies for the synthesis of acyclic, carbocyclic, and heterocyclic compounds under solvent-free conditions is provided. Since the main goal of this review is to enable the readers to follow new methods towards the synthesis of carbocyclic and heterocyclic compounds, we have classified the cycles according to ring simplicity and then subdivided by the number of heteroatoms present in the ring. Bearing in mind that the major interest in heterocycles is the synthesis via multicomponent reactions under solvent-free conditions, we have arranged the materials systematically according to the size and number of heteroatoms. The nature of the heteroatoms, and their number and positions in the molecule are used as secondary discriminators. This way, any one searching for a solvent-free synthesis of an acyclic, carbocyclic or heterocyclic scaffold will find a whole range of useful protocols. The review begins with the solvent-free reactions of acyclic compounds followed by carbocyclic and heterocyclic systems. For clarification of ambiguity, we also have named the nucleus in the review according to the naming done by the authors in the respective journals. Thus, our aim is to provide a comprehensive and updated overview of recent developments in multicomponent approaches from 2000 to 2010 on the preparation of compounds with the emphasis on the rationale behind each synthetic procedure and the dependence of the results on a proper selection of reaction conditions.
1.1 Multicomponent reactions (MCRs)
Several methodologies such as the Merrifield synthesis, click processes, and multicomponent reactions (MCRs) are amenable to implementation as fast and efficient chemical syntheses suitable for automation. Of these, multicomponent processes have considerable economic and ecological interest as they address the fundamental principles of synthetic efficiency and reaction design. Multicomponent reactions1–3 have become important tools for the rapid generation of molecular complexity and diversity with predefined functionality in chemical biology and drug discovery.4–6 These reactions are often discovered by serendipity, but rational design strategies are now playing an increasing role because of their convergent nature, superior atom economy, and straightforward experimental procedures in the construction of target compounds by the introduction of several diversity elements in a single operation, resulting in substantial minimizations of waste, labor, time, and cost. Further, chemo- and stereoselectivities7 of MCRs have been widely accepted as a significant challenging task for synthetic organic chemists. MCRs, a powerful and virtually reliable target-guided synthetic approach, has extensively been used and applied for the rapid construction of molecular-level complex architectures, and interest from different branches of science is expanding exponentially.
In order to break down the complex notion of molecular diversity, we can distinguish three fundamental levels of diversity: (a) appendage diversity (combinatorial chemistry), (b) stereochemical diversity, and (c) scaffold diversity. Appendage diversity involves the introduction of different appendages to a common molecular skeleton, resulting in limited overall diversity. Stereochemical diversity involves the selective generation of as many stereoisomers of the same molecule as possible by changing the stereochemistry of the catalyst and/or chiral starting materials. Scaffold diversity is probably the most important element of diversity, which involves the generation of a collection of compounds with different molecular skeletons. This can be realized by changing the reagents added to a common substrate (reagent-based approach) or by transforming a collection of substrates with suitable pre-encoded skeletal information under similar reaction conditions (substrate-based approach). Unlike molecular diversity, which can be readily quantified on the basis of structural and physicochemical properties, molecular complexity is a less tangible property that is hard to quantify. It involves not only the number and types of atoms in the molecule, but also their connectivity. Therefore, new protocols in terms of efficiency, minimal environmental hazards, operational simplicity, and high selectivity are still demanded and would be of great relevance to both synthetic and medicinal chemists. Amongst numerous well-known multicomponent name reactions, the important ones are depicted in Fig. 1.
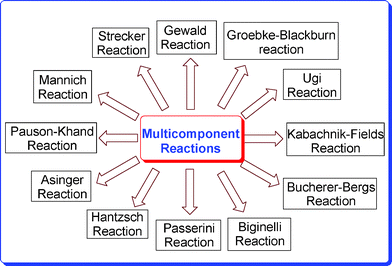 |
| Fig. 1 Different multicomponent reactions. | |
1.2 Solvent-free reactions
Because of the increasing concern of the harmful effects of organic solvents on the environment and human body, organic reactions that are operated with green solvents or without conventional organic solvents have aroused the attention of organic and medicinal chemists. In the past decade, interest in solvent-free MCRs has expanded and it now encompasses wide areas of the chemical enterprise. For reasons of economy and pollution prevention, solvent-free methods8 are used to modernize classical procedures by making them cleaner, safer, and easier to perform. The demand for both clean and efficient chemical syntheses is becoming more urgent.9 Among the proposed solutions, solvent-free conditions are becoming more popular and it is often claimed that the best solvent is no solvent.10
A pressing challenge facing organic chemists, therefore, is to advance new processes that are not only efficient, selective, and high yielding but also eco-compatible.11,12 Although steps toward sustainability can be made by reusing solvents, recycling is rarely accomplished with complete efficiency. An alternative strategy is to reduce the E factor (the E factor, introduced by Sheldon,13 is defined as the ratio of the weight of waste to the weight of product) of reactions and their impact on the environment is to conduct them under solvent-free conditions.14–17 The benefits of solvent-free processes are cost savings, decreased energy consumption, reduced reaction times, a large reduction in reactor size and capital investment. It has been an interesting observation that due to demerits associated with reactions carried out in conventional organic solvents, synthetic chemists are paying more attention to the development of new methodologies based on solvent-free reactions, clearly shown by the graphical representation of the growing number of solvent-free synthetic methodologies against years (Fig. 2).
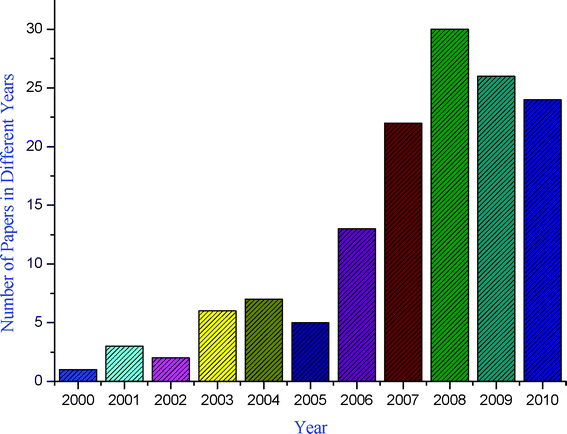 |
| Fig. 2 Number of publications dealing with MCRs under solvent-free conditions in the period 2000–2010. | |
2 Acyclic compounds
The synthesis of compounds occupies much of the effort of organic chemistry, and is the principal business of the chemical industry. The manufacture of drugs, pigments, and polymers entails the preparation of organic compounds on a scale of thousands to billions of kilograms per year, and there is constant research to develop new products and processes. Synthesis of new substances is carried out for many purposes beyond the goal of a commercial product. A compound of a specified structure may be needed to test a mechanistic proposal or evaluate a biochemical response such as the inhibition of an enzyme. Synthesis may provide a more dependable and less expensive source of a naturally occurring compound. Moreover, a synthetic approach permits variations in the structure that may lead to enhanced biological activity.
Three-component coupling of an aldehyde, alkyne, and amine (A3-coupling) is one of the best examples of acetylene–Mannich MCR and has received much attention in recent times. The resultant propargylamines are important building blocks for a variety of organic transformations and also valuable precursors for therapeutic drug molecules18,19 such as β-lactams, oxotremorine analogues, conformationally restricted peptides, isosteres, allylamines, oxazoles and other natural products. These compounds are synthesized by nucleophilic attack of lithium acetylides or Grignard reagents to imines or their derivatives20via a Mannich one-pot three-component coupling reaction of formaldehyde, secondary amines, and terminal alkynes.21 There has been continuing interest in developing transition-metal catalysts to accomplish the Mannich three-component reaction via C–H activation.22 Dax et al.23 reported the use of copper as a transition-metal catalyst for the solid-phase Mannich condensation of amines, aldehydes, and alkynes. This polymer-supported three-component Mannich reaction requires one of the reactants immobilized on a resin. A microwave-assisted Mannich three-component coupling reaction in the presence of Cu(I) on Al2O3 under solvent-free conditions has also been reported,24 and the enantioselective syntheses of propargylamines through a one-pot three-component coupling protocol have also been developed.25
Copper(I) immobilized on organic-inorganic hybrid materials such as silica behave as a very efficient heterogeneous catalyst in the three-component Mannich coupling reaction of terminal alkynes 1, aldehydes 2, and amines 3via C–H activation to afford the corresponding propargylamines 4 in high yields (82–99%) under solvent-free conditions (Scheme 1A).26 The microwave-enhanced Mannich condensation of 1, 2, and 3 on CuI-doped alumina in the absence of solvent has been reported by Kabalka et al.27 to produce the corresponding propargylamines 4 in good yields (Scheme 1B). The reaction has been further extended to produce 2-substituted benzo[b]furans in good yields (71–92%) via a Mannich condensation/cyclization sequence. Among the cuprous salts, cuprous iodide has been found to be most effective. Recently, our group has identified NiCl2 as a highly efficient and effective catalyst for a one-pot three-component (A3) coupling of 1, 2, and 3 to produce propargylamines 4 in nearly quantitative yields.28 Structurally divergent aldehydes (aromatic, heteroaromatic, and aliphatic) and amines were well tolerated under the reaction conditions.
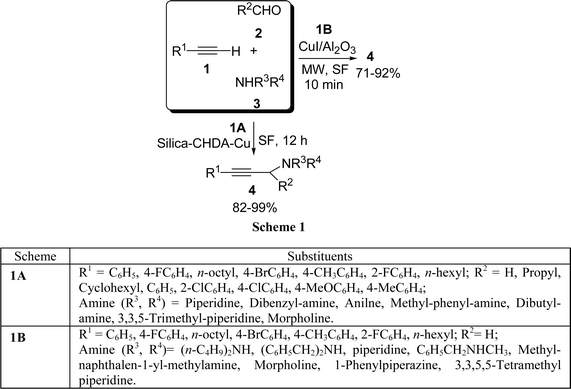 |
| Scheme 1 | |
β-Acetamido ketones and esters are versatile intermediates and exist in a number of biologically or pharmacologically important compounds.29 Various methods for the synthesis of β-acetamido ketones and esters by multicomponent coupling have been reported, involving aldehydes, enolisable ketones, acetyl chloride, and acetonitrile.30β-Acetylaminoketones have been synthesized using Cu(OTf)2, Zn(II), Bi(III), Sn(II), Sc(III)triflates, BF3, CuCl2, BiCl3, LaCl3, LiClO4, InCl3,31 H2SO4–SiO2,32 zeolite Hb,33 heteropolyacid,34 and K5CoW12O40·3H2O.35a Nagarapu et al.35b have described the results of SnCl2·2H2O-catalyzed one-pot synthesis of β-acetamidoketones and esters 9 with aryl aldehydes 5, enolisable ketones 6, acetyl chloride 7, and acetonitrile 8 under solvent-free conditions at room temperature in 76–92% yield (Scheme 2A). When methyl acetoacetate is involved in the reaction, diastereomeric mixtures can be obtained. The major diastereomer was anti in all the observed cases.
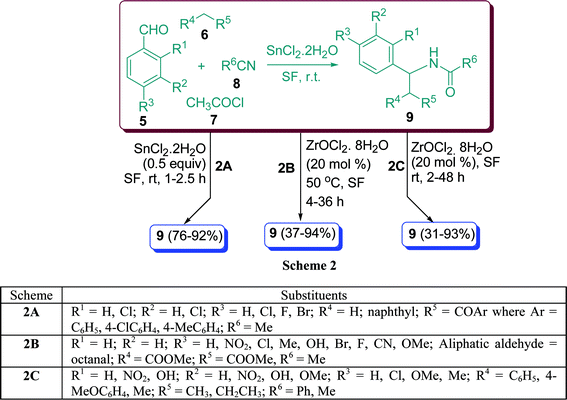 |
| Scheme 2 | |
The one-pot synthesis of β′-acetamido-β-dicarbonyl compound 9 from precursor compounds 5, 6, 7, and 8, catalyzed by 20 mol% ZrOCl2·8H2O in solvent as well as under solvent-free conditions was described by Ghosh et al.36 in high yields. Benzaldehyde generated the corresponding β-acetamido-β′-ketoester in 92% yield with moderate (1
:
3.7) diastereoselectivity using optimised solvent conditions. The selectivity can be well understood by incorporating a preferred chair like transition state. Under similar conditions, aromatic aldehydes containing electron-withdrawing groups in the ring gave good yields and selectivities. On the other hand, aromatic aldehydes containing electron-donating groups such as Cl, Me, OH, Br, and F in the ring also reacted equally well (Scheme 2B). β-Acetamido or β-amino ketones are also potential intermediates for the generation of β-amino alcohols,37 the common structural units in natural nucleoside antibiotics such as nikkomycins or neopolyoxins. Zr(IV) compounds, especially ZrCl4 have received considerable attention in various organic reactions38 because of their ready availability in the earth’s crust39 and low toxicity.40 However, the reported zirconium oxychloride based reactions are limited.41 It has been reported that a one-pot treatment of a mixture of 5, 6, 7, and 8 at ambient temperature in the presence of ZrOCl2·8H2O catalyst (20 mol%) furnished the corresponding β-acetamido ketones 9 in excellent yields (Scheme 2C).42α-Substituted enolizable ketones such as ethyl methyl ketone also reacted with benzaldehyde or p-methylbenzaldehyde producing poor to moderate diastereoselectivity, primarily forming the anti-isomer.
A highly efficient and eco-friendly protocol for the synthesis of β-aminoketone 13 by the coupling of aldehydes 10, amines 11, and ketones 12 using commercially available Fe(Cp)2PF6 as an efficient catalyst under solvent-free conditions has been developed (Scheme 3).43
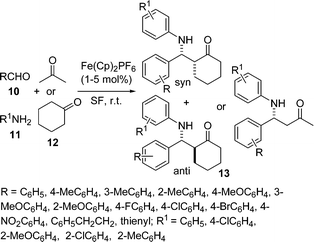 |
| Scheme 3 | |
α-Amino phosphonate derivatives are increasingly useful due to their importance in pharmaceuticals and industry. Thus, the development of simple, eco-benign, and low cost protocols for the synthesis of α-amino phosphonates are highly desirable. A facile and efficient synthesis of α-amino phosphonates 17via one-pot three-component coupling of aldehydes 14, amines 15, and diethyl phosphite 16 catalyzed by NBS or CBr4 (5 mol%) under solvent-free conditions at 50 °C has been carried out (Scheme 4).44
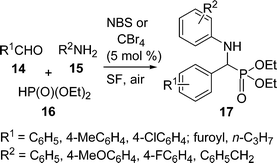 |
| Scheme 4 | |
β-Aryl-β-mercaptoketones are valuable synthetic scaffolds for both medicinal and synthetic organic chemists. They have been utilized as precursors for the synthesis of various biologically active compounds, such as thiochromans,45 thiopyrans,46 benzothiazapines,47 4,5-dihydroisoxazoles, 4,5-dihydropyrazoles48etc. Traditionally their synthesis has been performed by a sequence of two separate reaction steps, (i) synthesis of an α,β-unsaturated ketone via an aldol reaction, (ii) 1,4-conjugate addition of a thiol to an α,β-unsaturated ketone via thia-Michael addition. Michael addition of a thiol to chalcone is an efficient approach to prepare β-aryl-β-mercaptoketones. Zirconium chloride (40 mol%) efficiently catalyzes the one-pot three-component reaction of an aryl aldehyde 18, cyclic or acyclic enolizable ketones 19, and thiols 20 under solvent-free conditions at room temperature to afford the corresponding β-aryl-β-mercaptoketones 21via an aldol–Michael addition reaction (Schemes 5).49 Interestingly, when silica supported ZrCl4 was used, instead of exclusive formation of the desired product 21, the side product 22 was formed as the major one by the coupling of aldehyde and thiol (Scheme 6).
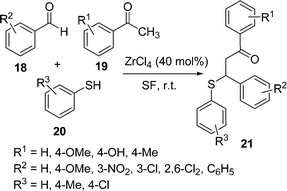 |
| Scheme 5 | |
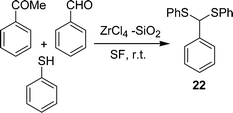 |
| Scheme 6 | |
α-Aminophosphonic acids have received significant attention in synthetic organic chemistry due to their structural analogy to natural α-amino acids, displaying biological importance either in themselves or as building blocks for peptides.50 Because of their versatile biological activities, a number of methods for the synthesis of α-aminophosphonic acids have been developed during the past two decades. Among the reported methods, three-component Mannich type reactions starting from aldehydes, amines, and phosphites catalyzed by Lewis acids such as InCl3,51 ZrCl4,52 lanthanide triflates,53 TaCl5–SiO2,54 SmI2,55 Mg(ClO4)2,56 I2 or acetyl chloride57 are common strategies. Ranu and Hajra58 have described a practical green approach for the synthesis of α-aminophosphonates by a three-component condensation of carbonyl compounds (aldehydes and ketones), amines, and diethyl phosphite at 75–80 °C in the absence of solvent and catalyst. Subsequently, Kumaraswamy and co-workers59 reported the synthesis of α-aminophosphonates in good yields. Recently, a simple and efficient three-component one-pot synthesis of α-amino phosphonates has been accomplished in good yields from aldehydes, amines, and diethyl phosphite using potassium hydrogen sulfate60a or super magnetic nano iron oxide60b as a catalyst under solvent-free conditions at ambient temperature. Zhang et al.61 synthesized α-aminoalkylphosphonate 26via the one-pot condensation of aldehydes/ketones 23, diethyl phosphoramidate 24, and cyclic trivalent chlorophosphite 25 at 50–60 °C in the absence of solvent and catalyst (Scheme 7). Various substituted aldehydes and ketones were tested and it was found that aromatic and heteroaromatic aldehydes produced better yields than ketones. However, only trace amounts of the α-aminoalkylphosphonates 26 were obtained when conjugated aliphatic aldehydes were used, and no expected products were obtained when aliphatic aldehydes and aliphatic ketones were utilized under similar reaction conditions.
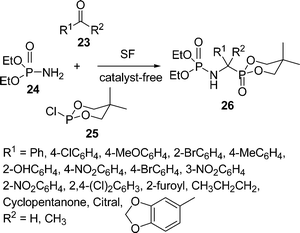 |
| Scheme 7 | |
Compounds with 1,3-amino oxygenated functional groups are present in a variety of biologically important natural products and in a number of nucleoside antibiotics and HIV protease inhibitors, such as ritonavir and lipinavir.62 1-Amidoalkyl naphthol can be easily hydrolyzed to 1-aminoalkyl naphthol, which shows biological activities such as hypotensive and bradycardiac effects.63 In addition, 1-aminoalkyl alcohol type ligands have been used for asymmetric synthesis and also as catalysts.64 Therefore, for easy access of 1-aminoalkyl alcohols, a simple and efficient protocol is very much desirable. Nandi et al.65 have reported an atom-efficient and environment-friendly approach for the synthesis of amidoalkyl naphthols 30 in excellent yields via a one-pot three-component reaction of 2-naphthol 27, aromatic aldehydes 28, and amides 29 promoted by P2O5 under solvent-free conditions at 60 °C (Scheme 8A). The reaction of 2-naphtol with aromatic aldehyde in the presence of a catalyst is known to provide a ortho-quinone methide (o-QMs) intermediate. The ortho-quinone methide intermediate was reacted with various amides to produce 1-amidoalkyl-2-naphtol derivatives.
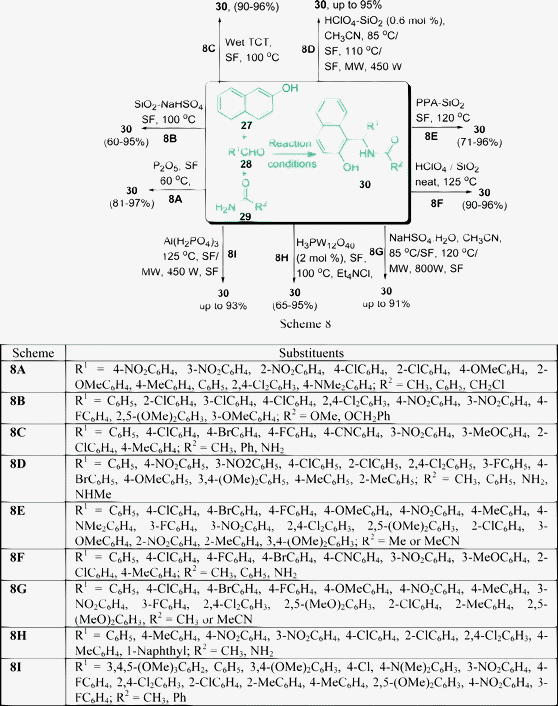 |
| Scheme 8 | |
Heterogeneous catalysts are generally less expensive, highly reactive, eco-friendly and are easy to handle. They reduce reaction times, impart greater selectivity and are simple to workup and the catalysts are simple to recover.66 Silica supported sodium hydrogen sulfates have gained much importance in recent years as heterogeneous catalysts due to economic and environmental considerations.67 The synthesis of 1-carbamatoalkyl-2-naphthol derivatives under solvent-free conditions have been described by Shaterian et al.68 They have described an efficient one-pot three-component condensation of 27, 28, and carbamates 29 in the presence of silica supported sodium hydrogen sulfate at 100 °C to give the corresponding product 30 (Scheme 8B). The method worked well with a variety of aryl aldehydes with both electron-withdrawing and electron-donating groups such as OMe, Cl, F and NO2. Under similar conditions, aliphatic aldehydes such as propionaldehyde or heptaldehyde and heterocyclic 2-pyridinecarbaldehyde did not give any desired product. A direct procedure has been developed for the preparation of amidoalkyl naphthols 30 in excellent yields by the one-pot condensation of 27,28 and 29, in the presence of 10 mol% wet-TCT as a catalyst under solvent-free media (Scheme 8C).69 Shaterian and coworkers70 have found that silica gel-supported polyphosphoric acid (PPA–SiO2) can also act as an efficient catalyst for the multicomponent condensation of 27, 28, and 29 to afford the corresponding 30 in good to excellent yields (Scheme 8E). HClO4–SiO271 has been utilized as a heterogeneous catalyst for the preparation of amidoalkyl naphthols by a one-pot condensation of aryl aldehydes, 2-naphthol, and urea or amides under solvent-free conditions (Scheme 8D).
Silica supported perchloric acid acts as a recyclable solid acid catalyst, which have been extensively used in organic reactions such as the protection of hydroxyl groups;72 the acetylation of phenols, thiols, alcohols, amines,73 and β-keto enol ethers;74 the Knoevenagel condensation, Michael addition and cyclo-dehydration;75 the synthesis of 14-aryl-14H-dibenzo[a,j]xanthenes;76 the Friedländer synthesis of quinolines;77 the synthesis of acylals from aldehydes;78 the synthesis of enaminones and enamino esters;79 the synthesis of quinoxalines and dihydropyrazines, and chemoselective carbon sulfur bond formation.80
Mahdavinia et al.81 have also used HClO4–SiO2 as a heterogeneous catalyst (1 mol%) for the preparation of amidoalkyl naphthols by a one-pot condensation of aryl aldehydes, 2-naphthol, and urea or amides in excellent yields under solvent-free conditions (Scheme 8F). The synthesis of amidoalkyl naphthols 30 using only sodium hydrogen sulfate (instead of supported by silica) as the heterogeneous catalyst has also been described (Scheme 8G).82 Another procedure has been developed for the preparation of amidoalkyl naphthols 30, the condensation of aryl aldehydes 27, β-naphthol 28 and urea or acetamide 29 in the presence of 12-tungstophosphoric acid as a heterogeneous catalyst under solvent-free conditions. A library of amidoalkyl naphthols were prepared by performing all the reactions for 80 min at 100 °C to give a 65–95% yield. Isobutyryl aldehyde did not take part in the reaction and therefore no other aliphatic aldehydes were examined in the protocol (Scheme 8H).83
A solvent-free synthesis of amidoalkyl naphthols 30 in the presence of Al(H2PO4)3 as a heterogeneous catalyst has also been described. The reaction was carried out under thermal conditions as well as under microwave irradiation. The main feature of the catalyst is its high stability and catalytic activity. It also can be recovered and recycled at least five times after washing with acetone and drying at 100 °C. The reaction time in the case of microwave irradiation was shortened notably compared to solvent-free thermal conditions. The yield of the product was also higher (55–93%) for microwave irradiation compared to thermal conditions (50–73%) (Scheme 8I).84 The one-pot, three-component condensation of the substrates 27, 28, and 29 also took place smoothly in the presence of cation-exchange resins (e.g. Indion-130, Indion-140, and Amberlyst-15) to afford the corresponding 1-amidoalkyl-2-naphthols 30 in good yields. Up to 90% yields of the products are obtained in 6–30 min at 110 °C under solvent-free conditions.85
A novel one-pot three-component Mannich condensation between an electron-rich aromatic compound such as 5-methyl-2-hydroxyphenyl sulfide 31, 2-aminopyrimidine 32, and aromatic aldehydes 33 for the preparation of a series of new unsymmetrical multidentate aminophenol ligands 34 has been described in high yields (75–90%) under solvent-free conditions at 125 °C (Scheme 9).86
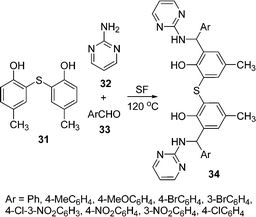 |
| Scheme 9 | |
The one-pot, three-component synthesis of 4-substituted 1-acylthiosemicarbazides 37 has been described by the condensation of 2-naphthol 28, aldehydes 35, and 1-acylthiosemicarbazides 36 catalysed by p-toluenesulfonic acid (p-TSA) in DCM at room temperature and under solvent-free conditions at 125 °C. Various aromatic aldehydes bearing electron-withdrawing and electron-releasing groups, aliphatic aldehydes, and heterocyclic aldehydes were well tolerated under the reaction conditions. Under solvent-free conditions, the reaction times were found to be much shorter than when using DCM, with almost parallel yields (Scheme 10).87
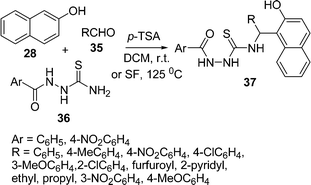 |
| Scheme 10 | |
Koszelewski et al.88 have studied the effects of the substrate structure and concentration on the yield of the Passerini reaction. They have developed a new and convenient solvent-free methodology for the preparation of α-acyloxyamides 41 by the coupling of 38, 39, and 40. The reaction was performed in solvent as well as under solvent-free conditions, but the yield of the product was greatly enhanced (86%) under solvent-free conditions compared to when using solvent (22%). When aromatic isocyanide was used, an increment of the yield (almost 50%) was observed under solvent-free conditions compared to the classical procedure. On the other hand, the products derived from aliphatic aldehydes were obtained in good yields (88–90%) when the reaction was performed in DCM. Reactions with p-anisaldehyde, p-methoxybenzoic acid, and isocyanoacetic acid ethyl ester did not give any desired product (Scheme 11).
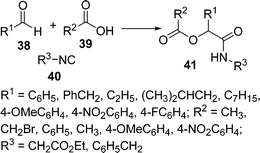 |
| Scheme 11 | |
Some novel substituted hydrazones 45 have been reported by El Kaïm and coworkers,89 by the coupling of hydrazones 42, aldehydes 43, and secondary amines 44 under solvent-free conditions (Scheme 12). The reaction remains confined to coupling of hydrazones possessing electron-withdrawing groups on the hydrazone (R1 = CO2Et, CN). Simple hydrazones (R1 = alkyl, aryl) did not react at all in ethanol, toluene or chlorobenzene, even after prolonged heating in the presence of several equivalents of amine and aldehyde.
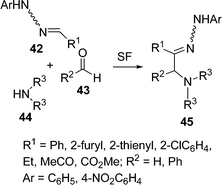 |
| Scheme 12 | |
Schiff bases owe their applications in metal ion complexation because they act as inhibitors of human β-thrombin and microbicides.90 Some new bis-Schiff bases 48 have been synthesized by the coupling of 5,5′-methylenebis(2-aminothiazole) 46 and aromatic aldehydes 47 under solvent and catalyst-free conditions (Scheme 13).91
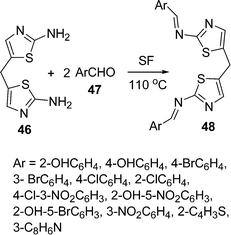 |
| Scheme 13 | |
Srihari et al.92 have developed a solvent-free synthetic method catalyzed by PMA-SiO2 (5 mol%) for the synthesis of 3-substituted indole derivatives 52 by the one-pot three-component coupling of aldehyde 49, N-methylaniline 50, and indoles 51 at room temperature. The reaction proceeded smoothly at a much faster rate under solvent-free conditions than when using solvent. The yields of the products were found to be good (85–95%) depending upon substituents (Scheme 14). Reactions involving cinnamaldehyde, butyraldehyde and n-octanal did not work under this protocol.
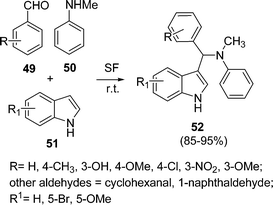 |
| Scheme 14 | |
Bhuyan et al.93 have synthesized some 3-alkylated indoles via the Michael addition of indoles to carbonyl compounds in the absence of catalyst. The one-pot three-component coupling of 2-substituted indoles 53, aldehydes 54, and N,N-dimethylbarbituric acid 55 under solvent-free conditions at 80–150 °C for 15–25 min resulted two products 56 and 57 in good yields (Scheme 15).
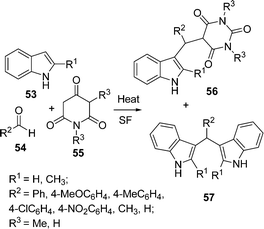 |
| Scheme 15 | |
Firouzabdi and co-workers94 have synthesized indole derivatives bis(indolyl)methanes 60via the electrophilic substitution of indoles with carbonyl compounds under solvent-free conditions in high yields. In this three-component condensation, the two molecules of indole 58 and one molecule of aldehyde/ketone 59 reacted to produce the desired bis(indolyl)methane in the presence of ZrOCl2·8H2O/Silica gel as a new efficient and highly water-soluble catalyst. Time required for the completion of the reaction and the yields of the products depend on Z, R1 and R2 (Scheme 16).
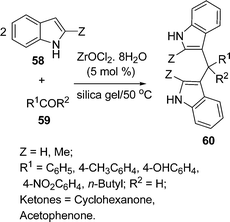 |
| Scheme 16 | |
Among the various indole derivatives, 3-substituted indoles, i.e. 3-[(N-heteroaryl)-(aryl)methyl]indoles 64, have recently been synthesized by the one-pot three-component domino coupling of indole 61, aromatic aldehydes 62, and heteroaryl amines 63 under solvent-free conditions at 80 °C in moderate to high yields (Scheme 17).95N-Substituted thioamides 68 have been synthesized from acyl halides 65 and amines 66 in the presence of recently developed thionating system H2O/PSCl367 (Scheme 18).96
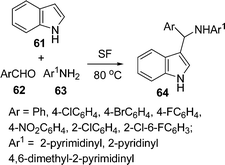 |
| Scheme 17 | |
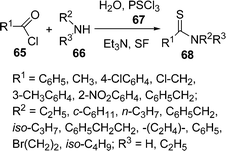 |
| Scheme 18 | |
O-Alkyl-2-methoxyethyl alkylphosphonates act as markers of nerve agents. A rapid and efficient surface mediated synthesis of O-alkyl-2-methoxyethyl alkylphosphonates from alkylphosphonic acids 69 and alcohols using DCC-Celite as a solid support under solvent-free condition is described (Scheme 19).97
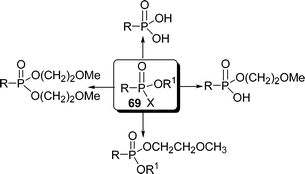 |
| Scheme 19 | |
The synthesis of S-allyl-N-aryl dithiocarbamates 73 through a one-pot coupling of amines 70, carbonyl sulfide 71, and alkyl halide or α,β-unsaturated compounds 72 using SnCl2 as a catalyst under solvent-free conditions is described (Scheme 20). Various substituted anilines, CS2, and allyl/crotyl bromide were treated under said conditions to yield the corresponding S-allyl-N-aryl dithiocarbamates.98
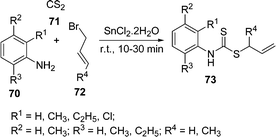 |
| Scheme 20 | |
3 Carbocyclic six-membered compounds
Biphenyls represent a key structural motif in a large number of compounds used as pharmaceuticals, agrochemicals, dyes, chiral ligands for metal catalysts, liquid crystals, organic semiconductors, and materials for molecular recognition devices.99a Furthermore, the biaryl subunit is present in an extensive range of natural products.99b The uncatalyzed synthesis of the biphenyl system has been scarcely investigated100b and solvent-free conditions have never been used. Considering the importance of this class of compounds, the development of a green approach for their synthesis has been attempted. Fringuelli et al.100a have described the synthesis of biphenyl-2-carbonitrile derivatives 76 by an uncatalyzed and solvent-free multicomponent process, starting from an aryl aldehyde and constructing the second aryl ring by (i) the Knoevenagel reaction, (ii) Diels–Alder cycloaddition, and (iii) the final aromatization process (Scheme 21). The synthesis has been carried out by the reaction of aryl aldehydes 74, active methylenes e.g. nitroacetonitrile, and 1,3-butadienes 75. The aromatization of adduct was performed at 60 °C, generally in the presence of 2.0 molar equiv. of DBU under O2 atmosphere (0.5 bar) and solvent-free conditions.
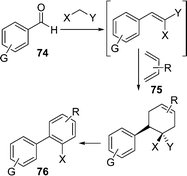 |
| Scheme 21 | |
Yanan Zhao and co-workers100b have developed a simple and green method for the synthesis of 1,3,5-triarylbenzenes 78 from three molecules of aryl ketone 77 catalyzed by P-TSA under solvent-free conditions. Acetophenones reacted smoothly to afford 1,3,5-arylbenzenes in good yields, irrespective of electron-donating or electron-withdrawing groups. When the para-substituents were chloro, bromo and iodo, increased yields were obtained due to their decreased electronegativity. Strong electron-donating groups such as NH2 and OCH3 provided lower yields than other groups such as alkyls or halogens. The reactions of para- or meta-substituted chloroacetophenones afforded slightly higher yields than sterically hindered ortho-substituted acetophenone, suggesting that the steric effect provides a significant contribution. Furthermore, water is the only byproduct of this reaction, which makes the present protocol environmentally benign (Scheme 22).
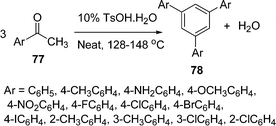 |
| Scheme 22 | |
An improved procedure for the three-component coupling reaction of aldehydes, amides, and dienophiles (AAD-reaction) has been developed by Strubing et al.101 Using microwave technology, an endo-selective synthesis of N-acyl cyclohexenylamines 82 by the coupling of aldehydes 79 and amides 80 followed by a Diels–Alder reaction with electron-deficient dienophiles 81 (Scheme 23) was performed. The reaction has been performed both in solvents and under solvent-free conditions and it has been observed that it is comparatively high yielding under solvent-free conditions. A versatile and efficient route to 3-amino-1-aryl-9H-fluorene-2,4-dicarbonitrile 86 the via one-pot coupling of 1-indanone 83, aromatic aldehydes 84, and malononitrile 85 under solvent-free conditions with NaOH as the catalyst is described in Scheme 24. The reaction has been performed at room temperature, which provided a 75-87% yield of the desired product within a very short time.102
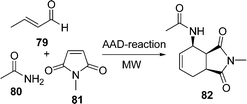 |
| Scheme 23 | |
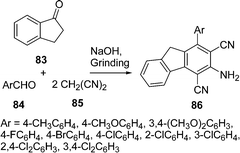 |
| Scheme 24 | |
A one-pot synthesis of 2-amino-5-nitro-4,6-diarylcyclohex-1-ene-1,3,3-tricarbonitriles 89 by the condensation of malononitrile 85, aromatic aldehyde 87, and nitromethane 88 has been carried out in the presence of Mg–Al hydrotalcite i.e. HTs (different Mg/Al ratios) and solid bases (Scheme 25). The reaction was performed in different solvents, such as DMF, H2O, and MeOH but the best results were obtained under solvent-free conditions. The catalyst can be easily separated and is also recyclable.103
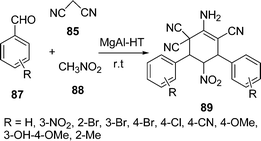 |
| Scheme 25 | |
4 Heterocyclic compounds
Heterocycles104,105 form the largest of the classical divisions of organic chemistry. Moreover, they are of immense importance not only both biologically and industrially, but to the functioning of any developed human society as well. Their participation in a wide range of areas can not be underestimated. The majority of pharmaceutical products that mimic natural products with biological activity are heterocycles. Other important practical applications of heterocycles can also be cited, for instance, additives and modifiers in a wide variety of industries including cosmetics, reprography, information storage, plastics, solvents, antioxidants, and vulcanization accelerators. Most of the significant advances against disease have been made by designing and testing new structures, which are often heteroaromatic derivatives. In addition, a number of pesticides, antibiotics, alkaloids, and cardiac glycosides are heterocyclic natural products of immense significance to human and animal health. Therefore, researchers are in continuous pursuit to design and produce better pharmaceuticals, pesticides, insecticides, rodenticides, and weed killers following natural models. These compounds play a major part in biochemical processes and are the side groups of the most typical and essential constituents of living cells. Finally, as an applied science, heterocyclic chemistry is an inexhaustible resource of novel compounds. A huge number of combinations of carbon, hydrogen, and heteroatoms can be designed, providing compounds with the most diverse physical, chemical, and biological properties. In fact, in the CMC database, more than 67% of the compounds listed contain heterocyclic rings, and nonaromatic heterocycles are twice as abundant as heteroaromatics. It is therefore easy to understand why both the development of new methods and the strategic deployment of known methods for the synthesis of complex heterocyclic compounds continue to drive the field of synthetic organic chemistry. Organic chemists have been engaged in extensive efforts to produce these heterocyclic compounds by developing new and efficient synthetic transformations. Among the new synthetic transformations, cyclocondensation reactions are among the most attractive methodologies for synthesizing heterocyclic compounds, and the need for improved cyclocondensation reactions is very much desirable.
4.1 Multicomponent reactions for the synthesis of heterocycles
Multicomponent reactions in general are cyclic or acyclic condensation reactions. The cyclocondensation reaction can be defined as a kind of annulation reaction involving the formation of a ring from one or several acyclic precursors. In multicomponent reactions, coupling occurs between three, four or more components, incorporating almost all the components to give the desired product with the elimination of some small molecule(s). The condensation reactions leading to heterocycles were carried out with a range of different components and reactions types and the functional groups can react as either the electrophiles e.g. E1, E2, and E3 or nucleophiles e.g. Nu1, Nu2, and Nu3. The electrophiles are primarily functional groups containing carbon atoms bonded with other heteroatoms such as, O, N, S, halogen atoms etc. forming carbonyl, imine, nitrile, thiocarbonyl, unsaturated ketones, ethylcyanoacetate, mono or dihalosubstituted carbons, acetal and orthoester carbons; and the nucleophiles are either carbon atoms joined adjacent to groups such as aldehydes, ketones, enols, or enamines, or heteroatoms such as nitrogen, oxygen, and sulfur. We therefore have given the different mode of condensations schematically in Fig. 3.106
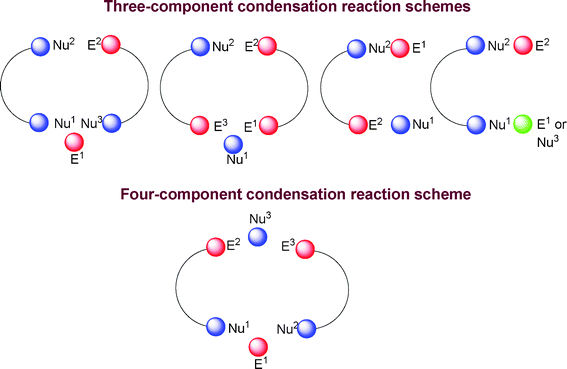 |
| Fig. 3 Different condensation schemes of multicomponent reactions. | |
4.2 Building blocks for the formation of heterocycles
The reactions mentioned in this review leading to the formation of heterocycles are mainly based on certain building blocks (Table 1). The building blocks incorporate the characteristics of electrophiles and nucleophiles, and are consequently coupled in different ways (as shown in Fig. 3) to form the corresponding heterocycles.
Table 1
Building blocks for three-component reactions |
No. of components |
Building blocks |
Products |
[3 + 2 + 1] |
[CCN + CC + C] |
acridines |
[3 + 2 + 1] |
[CCO + CC + C] |
chromenes/chromones |
[3 + 2 + 1] |
[CCS + CC + C] |
thiochromenes/thiochromones |
[4 + 2 + 1] |
[NCCN + CC + C] |
diazepines |
[3 + 1 + 1] |
[NCN + C + C] |
imidazoles |
[3 + 2 + 1] |
[CCN + CC + C] |
isoquinolines |
[2 + 2 + 1] |
[CC + CC + N] |
pyrroles |
[2 + 2 + 1] |
[NN + CC + C] |
phthalazines |
[3 + 2 + 1] |
[CCO + CC + C] |
pyrans/chromenes/xanthenes |
[3 + 2 + 1] |
[CCC + CC + N] |
pyridines |
[3 + 2 + 1] |
[CCN + CC + C] |
pyridinones |
[3 + 2 + 1] |
[NCN + CC + C] |
pyrimidines |
[3 + 2 + 1] |
[NCN + CC + C] |
pyrimidinones/pyrimidinethiones |
[3 + 2 + 1] |
[CCN + CC + C] |
quinolines |
[4 + 1 + 1] |
[CCCN + C + N] |
quinazolines/quinazolinones |
[3 + 1 + 2] |
[NCS + C + CC] |
thiazines |
[2 + 1 + 1] |
[CCN + C +S] |
thiazolines |
[2 + 1 + 2] |
[CC + N + CS] |
thiazolines |
[3 + 2 + 1] |
[NCS + CC + C] |
thiazines |
Building blocks for four-component reactions |
[2 + 1 + 2 + 1] |
[CC + C + CC + N] |
acridines |
[1 + 1 + 1 + 1] |
[CC + N + C + N] |
imidazoles |
[2 + 2 + 1 + 1] |
[CC + CC + C + N] |
pyridines |
[3 + 1 + 1 + 1] |
[CCC + N + C + N] |
pyrimidines |
[2 + 2 + 1 + 1] |
[CC + CC + C + N] |
pyridinones |
[2 + 2 + 1 + 1] |
[CC + CC + C + N] |
quinolines |
4.3 Five-membered heterocycles
4.3.1 Containing one heteroatom.
4.3.1.1 Pyrroles.
Pyrrole derivatives are very important heterocycles from many points of view including medicinal, pharmaceutical, and materials science; and are common structural motifs in various biologically active molecules and pharmaceutical substances for their antioxidant, antibacterial, antitumor, anti-inflammatory, and antifungal properties. The pyrrole nucleus is the key structural motif of heme and chlorophyll, the pigments essential for life. Multicomponent reactions are one of the most interesting concepts in modern synthetic chemistry to provide an attractive entry into pyrrole derivatives.107a Recently, a solvent-free and catalyst-free synthesis of pentasubstituted pyrroles 93 has been developed in good yields (70–85%) via the one-pot three-component coupling of primary amines 90, alkyl acetoacetates 91, and fumaryl chloride 92 (Scheme 26).107b
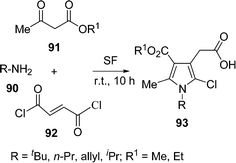 |
| Scheme 26 | |
Ranu et al.108a have synthesized substituted pyrroles 97 (Scheme 27) by coupling aldehydes/ketones/conjugated carbonyls 94, amine 95, and a simple or α,β-unsaturated nitroalkane 96 on the surface of a silica gel by microwave irradiation in good yields. The above method was also modified using N,N-disubstituted thiobarbituric acids in place of the α,β-unsaturated aldehyde, providing pyrrolo[2,3-d] pyrimidines108b in good yields. Furthermore, the synthesis of highly substituted and fused pyrroles 101 have also been reported by coupling acyclic/cyclic carbonyl compounds 98, an amine 99, and α,β-unsaturated nitro compounds 100 on an alumina surface by Ranu and co-workers (Scheme 28).108a,c
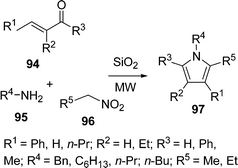 |
| Scheme 27 | |
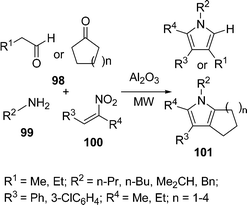 |
| Scheme 28 | |
Polysubstituted pyrroles 105 with complete control of pathway selectivity have been synthesized by a sequential one-pot three-component reaction of primary aliphatic amines 102, active methylene compounds 104, and 1,2-diaza-1,3-dienes 103 under solvent-free conditions without a catalyst (Scheme 29). Various primary aliphatic amines such as 4-methoxybenzylamine, n-propylamine, n-butylamine, allylamine, 1-amino-2-propanol, 1-amino-2-acetaldehyde diethyl acetal, and cyclohexylamine were used and effectively converted into the corresponding substituted pyrroles, which are otherwise not easy to synthesize. Pyrroles substituted with electron-withdrawing functional groups such as carboxylic acid derivatives (both symmetrical and unsymmetrical dicarboxylate scaffold, ester, amide, and/or thioester), sulfone, and phosphonate moiety at the C-3 and C-4 positions of the heterocyclic ring can be directly obtained, indicating the fitness of the scheme to all kinds of components.109
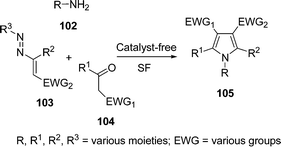 |
| Scheme 29 | |
4.3.1.2 Indoles.
The substituted indole nucleus is a structural component of a vast number of biologically active natural and unnatural compounds. The indole nucleus is without doubt a privileged structure in medicinal chemistry and also very abundant in nature. Substituted indoles possess several biological activities such as antioxidant, antibacterial, and insecticidal properties. They also act as colon cancer cell and tumor growth inhibitors and are employed as valuable antibiotics. The synthesis and functionalization of indoles has been the object of research for over 100 years, and a variety of well-established classical methods are now available. To name a few of them: the Fisher indole synthesis, the Gassman synthesis of indoles from N-haloanilines, the Madelung cyclization of N-acyl-o-toluidines, the Bischler indole synthesis, the Batcho–Leimgruber synthesis of indoles from o-nitrotoluenes and dimethylformamide acetals, and the reductive cyclization of o-nitrobenzyl ketones.
Recently, a three-component solvent-free synthesis of a 5-hydroxy-benzo[g]indole scaffold 109 through a Lewis acid-catalyzed one-pot reaction of naphthaquinone 106, ketones 107, and urea 108 under microwave irradiation has been devised by Borthakur and co-workers110 (Scheme 30). The key step in the synthesis is a Michael addition followed by in situ aza cyclization. Urea has been used as an environmentally benign source of ammonia. This synthetic route tolerates a significant substrate variation to deliver a broad range of substituted products in 50–88% yields.
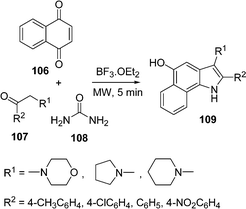 |
| Scheme 30 | |
4.3.1.3 Furans.
Furans are among the most important five-membered heterocycles in organic and pharmaceutical chemistry. They are not only significant as key motifs in many natural products, but also as versatile building blocks for the construction of highly complex target structures in numerous total syntheses. As an alternative to classical furan syntheses, when the mixture of o-ethynylphenol 110, secondary amines 111, and para-formaldehyde 112 were subjected to microwave irradiation of 30% power for 5 min under solvent-free conditions in the presence of cuprous iodide doped alumina, it underwent Mannich condensation followed by cyclization to give 2-(dialkylaminomethyl)-benzo[b]furans 113 in low to moderate yields (38–70%) (Scheme 31).111 Interestingly, when o-ethynylphenol (2 equiv) was subjected to react with para-formaldehyde (excess) and piperazine (1 equiv), bis-Mannich condensation-cyclization product was formed in 40% yield.
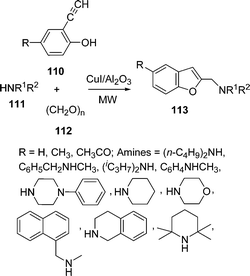 |
| Scheme 31 | |
4.3.1.4 Thiophenes.
Thiophene derivatives have emerged as a class of important heterocycles because of their presence in a broad spectrum of natural and synthetic organic molecules with diverse biological properties and utility in organic synthesis as versatile intermediates. Over the years, thiophene-based materials have emerged as an important class of electrically conducting organic materials. A facile and efficient one-pot synthesis of highly substituted thiophenes 117 has been developed by Karthikeyan et al.112via the condensation of pyrrolidine or piperidine 114, 5-aryldihydro-3(2H)-thiophenone 115, and an aromatic aldehyde 116 under MW irradiation (Scheme 32). Moderate to good yields of thienylpyrrolidines (45–60%) were obtained by a one-pot tandem process. In the case of reactions with piperidine, the yields are lower (30–35%).
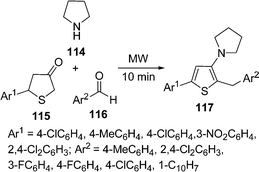 |
| Scheme 32 | |
4.3.1.5 Lactams.
2-Azetidinones, commonly known as β-lactams, are the key structural motifs in the most widely used class of antibiotics, i.e., β-lactam antibiotics, such as penicillins, cephalosporins, carbapenems, etc. The development of novel synthetic methodologies for the preparation of functionalized β-lactams and the screening of their biological activity has occupied a pivotal position in medicinal chemistry for almost a century now. Deprez et al.113 have synthesized five- and six-membered lactams 121via a 4-center three-component Ugi reaction by combining amines 118, isocyanides 119, and ketoacids 120 under solvent-free microwave irradiation conditions (Scheme 33).
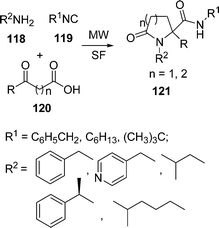 |
| Scheme 33 | |
4.3.2 Containing two heteroatoms.
4.3.2.1 Pyrazoles.
Pyrazoles are rarely available in natural products, but they represent an important motif of man-made biologically active compounds. Many synthetic pyrazoles show anti-hyperglycemic, anti-inflammatory, anticancer, antipyretic, antibacterial, analgesic, sedative, and hypnotic activity. Some of these compounds have emerged as potent and selective γ-aminobutyric acid (GABA)-gated chloride channel antagonists, novel ligands for oestrogen receptors, and agrochemicals of economic importance. The 1,3,5-tri- and 1,3,4,5-tetrasubstituted pyrazoles constitute the core structures of commercial drugs. Among the reported syntheses of pyrazoles, widely applicable methods are the dipolar [3 + 2] cycloadditions between CN2 and C2 moiety or the classical cyclocondensation of a monosubstituted hydrazine with a 1,3-dicarbonyl compound or surrogates thereof.
Among the various reported methods of pyranopyrazoles,114–117 a three-component condensation118 of N-methylpiperidone, pyrazolin-5-one, and malononitrile in absolute ethanol, and a two-component reaction119 between pyran derivatives and hydrazine hydrate are superior. Preparation of 1,4-dihydropyrano[2,3-c]pyrazoles include synthesis in aqueous media,120 the use of piperidine as a base in water,121N-methylmorpholine in ethanol,122 microwave irradiation123 and solvent-free conditions.124–126 A green protocol has been developed with per-6-ABCD, which acts simultaneously as a supramolecular host as well as an efficient solid base catalyst for the solvent-free syntheses of various dihydropyrano[2,3-c]pyrazole derivatives 125 involving a four-component reaction of 122, 123, 124, and 85 (Scheme 34). This atom-economical protocol also applies for ketones and resulted in near quantitative yields. The catalyst can be reused at least six times without any change in its catalytic activity.127
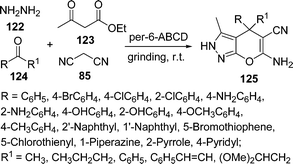 |
| Scheme 34 | |
4.3.2.2 Imidazoles.
Substituted imidazoles represent a common scaffold in numerous bioactive compounds and have a number of pharmacological properties. Imidazoles are also widely used as precursors of N-heterocyclic carbenes, organocatalysts, and ionic liquids. Due to the wide importance of this class of compounds their synthesis and functionalization reactions have been intensively studied in the field of organic synthesis. Despite many synthetic processes,128 Khmelnitsky et al.129 have produced a solvent-free microwave assisted synthesis of 2,4,5-trisubstituted and 1,2,4,5-tetrasubstituted imidazoles 130 by the condensation of 1,2-dicarbonyl compounds 126, aldehyde 127, and amine 128 using acidic alumina impregnated with ammonium acetate 129 as the solid support in up to 80% yields (Scheme 35A). Kantevari et al.130 have developed a one-pot four-component synthesis of 1,2,4,5-tetrasubstituted imidazoles 130 by the cyclocondensation of the components using perchloric acid adsorbed on silica. The reaction was performed in different solvents using different catalysts but the highest yielding conditions was a solvent-free environment with HClO4/SiO2 (Scheme 35B). Depending upon different substituents, moderate to high yields (56–98%) of the products were obtained.
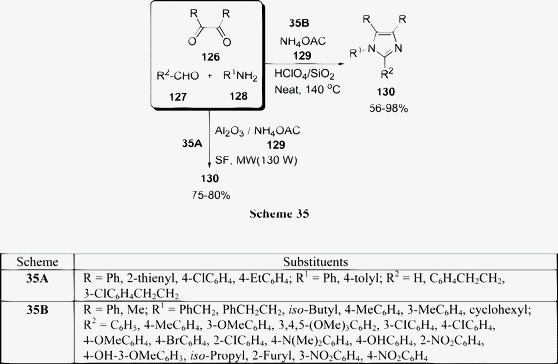 |
| Scheme 35 | |
Adib and co-workers131 synthesized 1,2,4-trisubstituted-1H-imidazoles 134via a one-pot four-component reaction of ammonium acetate 129, 2-bromoacetophenone 131, aldehyde 132, and primary amines 133 under solvent-free conditions (Scheme 36). Different aryl aldehydes, bromoacetophenones and alkyl amines have been used and were stable in this protocol. Depending upon the substituents, 80–95% yields of the product was obtained in 2 h.
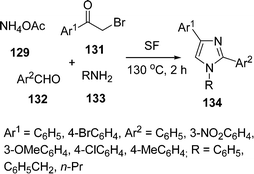 |
| Scheme 36 | |
Silica-supported titanium tetrachloride has been prepared and employed as a novel catalyst for the rapid and efficient synthesis of 2,4,5-trisubstituted imidazoles 137 in excellent yields by a three-component one-pot condensation of aryl aldehydes 135, 1,2-diketones 136, and ammonium acetate 129 under solvent-free conditions using conventional heating or microwave irradiation (Scheme 37).132
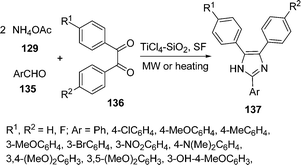 |
| Scheme 37 | |
4.3.2.3 Thiazoles.
Thiazoles and their derivatives are found to be associated with various biological activities. In addition, thiazoles are also synthetic intermediates and common substructures in numerous biologically active compounds. Thus, the thiazole nucleus has been much studied in the fields of organic and medicinal chemistry. Among the many synthetic procedures of thiazole or thiazole derivatives, Dawane et al.133 have developed a method for the synthesis of thiazole derivatives 141 through the coupling of substituted o-hydroxy benzaldehydes 138, substituted-α-haloketones 139, and thiourea 140 under solvent-free conditions in 85–95% yields (Scheme 38). Anderluh and co-workers134 have described a one-pot three-component reaction among 142, 143, and 144 leading to the formation of 2-amino-5-alkylidine-thiazol-4-ones 145 involving conventional heating under solvent-free conditions or microwave irradiation in low to moderate yields (Scheme 39).
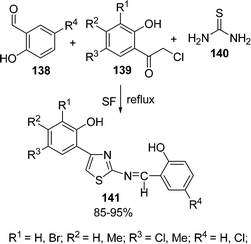 |
| Scheme 38 | |
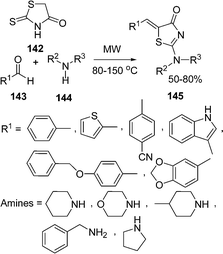 |
| Scheme 39 | |
An efficient procedure for the synthesis of thiazole derivatives 148 by a three-component one-pot reaction of thiourea 140, α-haloketone 146, and substituted pyrazolones 147 under environmentally solvent-free conditions in good yields has been developed (Scheme 40).135
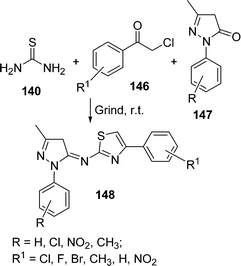 |
| Scheme 40 | |
4.3.2.4 Thiazolines.
Thiazoline and its derivatives are important scaffolds for drug candidates due to their anticonvulsant, sedative, antidepressant, anti-inflammatory, antihypertensive, antihistaminic, and antiarthritic activities. Min Xia and co-workers136 have synthesized 2-acylimino-3-aryl-thiazolines 152via a three-component one-pot reaction of amines 149, isothiocyanate 150, and α-halocarbonyl compounds 151. The reaction is accelerated by microwave irradiation under solvent-free conditions, resulting in the desired compounds in 83–98% yields (Scheme 41).
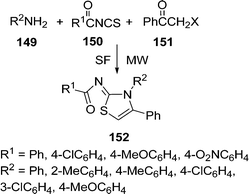 |
| Scheme 41 | |
2-Thiazolines 156 have been synthesized by reaction of carboxylic acids 153 and 1,2-aminoalcohol 154 in the presence of Lawesson's Reagent 155 by microwave irradiation under solvent-free conditions.137 The reaction was carried out using molar ratio of the reactants in 1.0
:
1.5
:
0.75 at 150 °C for 4–8 min. If the substituents in amino alcohol, R2, R3 = H or R2 = Me and R3 = H, the yield of the product increased significantly, while with substituents R2 = H, R3 = Ph resulted in poor yields. The overall yield is 21–86% depending upon substituents (Scheme 42). The reaction is also compatible with heteroaromatic acids, e.g pyridyl, furyl and thienyl derivatives. Aliphatic acids, such as heptanoic and decanoic acids led to the corresponding 2-thiazolines in good yields.
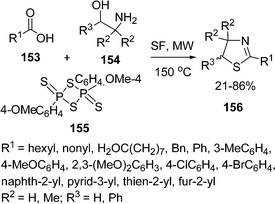 |
| Scheme 42 | |
4.3.2.5 Thiazolidinones.
Thiazolidin-4-ones are applied pharmaceutically as antimicrobial agents. Kasmi-Mir et al.138 have developed an efficient one-pot three-component solvent-free synthesis of thiazolidine-4-one by the coupling of substituted thiourea 157, chloroacetic acid 158, and aldehyde 159 (1.0
:
1.2
:
1.0 molar ratio) under microwave irradiation at 90–110 °C for 10–20 min. The desired product 5-arylidene-2-iminothiazolidin-4-ones 160 have been obtained in 61–89% yields (Scheme 43). Yavari and co-workers139 have synthesized thiazolidine-4-ones 164 in 70–83% yields through one-pot three-component reaction under solvent-free conditions at room temperature. Various 4-phenylthiosemicarbazides 161, DMAD 162, and aldehydes or ketones 163 were tolerated well under solvent-free conditions (Scheme 44).
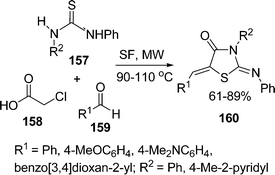 |
| Scheme 43 | |
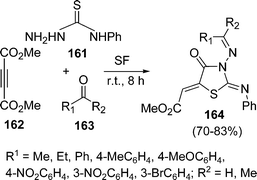 |
| Scheme 44 | |
4.3.2.6 Phthalazines.
Phthalazine (also called benzoorthodiazine or benzopyridazine) is a heterocyclic organic compound isomeric with quinoxaline, cinnoline, and quinazoline. Like others members of the isomeric diazine series, phthalazines have found wide applications as therapeutic agents, as ligands in transition metal catalysis, as chemiluminescent materials, and for optical applications. Heterocycles with a phthalazine moiety exhibit various pharmacological and biological activities,140 such as anticonvulsant,141 cardiotonic,142 and vasorelaxant143 properties. In view of its wide range of applications in synthetic and medicinal chemistry, various methods are reported in the literature for the synthesis of phthalazine derivatives.144 Sayyafi et al.145 have derived 2H-indazolo[2,1-b]phthalazine-1,6,11(13H)-trione derivatives 168 from the condensation of phthalhydrazide 165, dimedone 166, and aromatic aldehydes 167 under solvent-free conditions in excellent yields (80–93%) within 10–20 min (Scheme 45A).
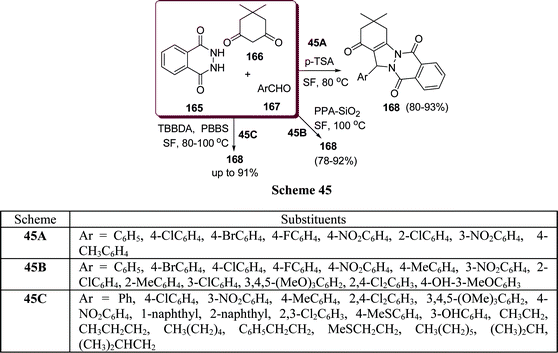 |
| Scheme 45 | |
Similarly, 2H-indazolo[2,1-b]phthalazine-1,6,11(13H)-trione derivatives 168 have been synthesized through the three-component condensation reaction of 165, 166, and 167 under solvent-free conditions in the presence of reusable silica supported polyphosphoric acid (PPA–SiO2) as the heterogeneous acid catalyst. It was found that a catalyst loading of 0.05 mmol at 100 °C provided the desired products in 78–93% yields, depending upon the substituents on the starting materials. The catalyst was reused five times without any significant loss in its catalytic activity (Scheme 45B).146 The N,N,N′,N′-Tetrabromobenzene-1,3-disulfonamide (TBBDA) and poly N-bromo-N-ethylbenzene-1,3-disulfonamide (PBBS) catalyzed one-pot synthesis of aliphatic and aromatic 2H-indazolo[2,1-b]phthalazinetriones 168 from various aliphatic and aromatic aldehydes 167, 165, and 166 at 80–100 °C under solvent-free conditions has been developed by Ghorbani-Vaghei et al. (Scheme 45C).147a Very recently, a rapid and efficient one-pot three-component protocol for the synthesis of 2H-indazolo[2,1-b]phthalazine-1,6,11-triones and 1H-pyrazolo[1,2-b]phthalazine-5,10-diones has been developed by our group147bvia domino coupling of phthalhydrazide, 1,3-diketones, and aldehydes under solvent-free conditions at 80 °C as well as under solvent-free ultrasound irradiation at room temperature promoted by (S)-camphorsulfonic acid.
4.3.3 Containing four heteroatoms.
4.3.3.1 Tetrazoles.
Among tetrazoles, 1-substituted tetrazoles have been used in a variety of synthetic and medicinal chemistry applications as well as in materials science, including propellants and explosives. They are also regarded as the biological equivalent to the carboxylic acid group. Kundu and co-workers148 have developed a synthetic route towards the synthesis of 1-substituted-1H-1,2,3,4-tetrazoles 172 in excellent yields via a three-component condensation of amine 169, trimethyl orthoformate 170, and sodium azide 171 in the presence of a catalytic amount of indium triflate (5 mol%) under solvent-free conditions. The reaction proceeds smoothly to generate the corresponding 1-substituted tetrazoles in 70–92% yields upon heating at 100 °C. The reaction tolerated a wide range of anilines (containing electron-withdrawing as well as electron-donating groups) and heterocyclic amines such as 2-amino pyridine and furfurylamine furnishing the corresponding products (Scheme 46).
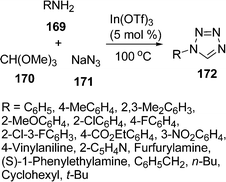 |
| Scheme 46 | |
Recently click chemistry has been much focused where condensation of alkynes with azides occurs to yield the triazoles. Similarly, methods for addition of cyanides with azides to yield tetrazoles have been explored by Srihari et al.149 The desired tetrazoles 176 have been synthesized by the coupling of Baylis Hillman acetate 173, TMS azide 174, and arylnitrile 175 under solvent-free conditions in the presence of TBAF as catalyst. Some of these tetrazoles were found to be potential TNF-α inhibitors. They have found that E-isomer was the only product formed with Baylis Hillman acetates containing ester moiety but nitrile containing Baylis Hillman acetates gave the mixture of diastereomers with Z-isomer as the major product. (Scheme 47).
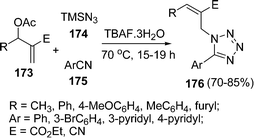 |
| Scheme 47 | |
4.4 Fused heterocycles
4.4.1 Derived from compounds containing one heteroatom.
Condensed pyridines are known for various biological activities, for example, pyrazolo[3,4-b]pyridines are useful for treatment of a wide variety of stress-related illnesses, such as depression, Alzheimer's disease, gastrointestinal disease, anorexia nervosa, haemorrhaged stress, drug and alcohol withdrawal symptoms, drug addition and infertility. The pyridine nucleus is also present in many products such as drugs, vitamins, food, flavorings, plants dyes, adhesives, and herbicides. Mehdi Adib et al.150 reported a procedure for the synthesis of 3-amino-2-arylimidazo[1,2-a]pyridines 180via a one-pot multicomponent reaction of 2-aminopyridines 177, benzaldehydes 178, and imidazoline-2,4,5-trione 179 under solvent-free conditions (Scheme 48).
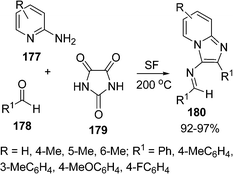 |
| Scheme 48 | |
Indolizines constitute the main structural part of many naturally occurring biologically important alkaloids such as (−)-dendroprimine,151 indalozin 167B,152 (−)-slaframine,153 conceine154etc. General procedures for the synthesis of indolizine are sequential N-quarternisation and intramolecular cyclocondensation or cycloaddition reaction by N-acyl/alkyl pyridinium salts. Bora et al.155 have developed a microwave-mediated three-component reaction of acyl bromide 181, pyridine 182, and acetylene 183 in the presence of basic alumina under solvent-free conditions to give indolizines 184 in excellent yields (Scheme 49).
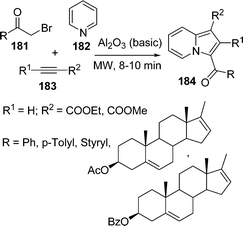 |
| Scheme 49 | |
Liu et al.156 have synthesized substituted aminoindolizines 188 by a gold catalyzed three-component coupling/cycloisomerisation reaction of heteroaryl aldehydes 185, amines 186, and alkynes 187 under solvent-free conditions. The speciality of the reaction is that the coupling of enantiomerically enriched amino-acid derivatives form the corresponding N-indolizines, incorporating amino-acids and maintaining its enantiomeric purity (Scheme 50).
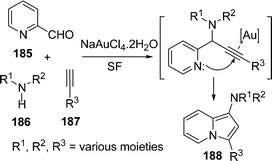 |
| Scheme 50 | |
A microwave procedure was efficiently applied to the synthesis of a series of novel naphthindolizinedione derivatives 189 whose structures somewhat resemble those of known antitumor agents able to interact with DNA by intercalation. An antitumor amide was obtained in a few minutes with high yields through a solventless one-pot cyclization followed by treatment with the suitable amine. This method was also used to access tetracyclic aza-compounds. The one-pot three-component cyclization was found to be more atom-efficient than the N-ylide sequence. To investigate the effect of structural modifications on the naphthindolizinedione skeleton, some indolizino-quinoline-5,12-dione precursors have also been prepared by the cyclization of compound 2,3-dichloro-2,3-dihydro-[1,4]naphthoquinone with ethyl acetoacetate (EAA) and pyridine or picoline (Scheme 51).157
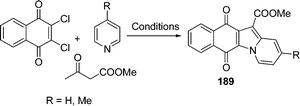 |
| Scheme 51 | |
4.4.2 Derived from compounds containing two heteroatoms.
A three-component synthesis of highly substituted bicyclic pyridines containing ring-junction nitrogen 193 has been described. The cyclocondensation of HKAs 190, triethoxymethane 191, and active methylene compounds 192 was done by refluxing under solvent-free and catalyst-free conditions to afford bicyclic pyridines in excellent yields (78–94%) within 30–59 min depending upon the nature of the substituents (Scheme 52).158
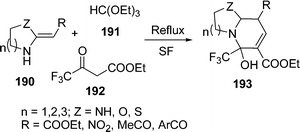 |
| Scheme 52 | |
4.4.3 Derived from compounds containing more than two heteroatoms.
A three-component synthesis of 3-amino-2-arylimidazo[1,2-a]pyridines, 3-amino-2-arylimidazo[1,2-a]pyrazines, and 3-amino-2-arylimidazo[1,2-a]pyrimidines is described by Adib and co-workers.159 Imine derivatives 196 of the title compounds were prepared in excellent yields by heating a mixture of 2-aminopyridine, 2-aminopyrazine or 2-aminopyrimidine 194, a benzaldehyde 195, and imidazoline-2,4,5-trione 179 under solvent-free conditions (Scheme 53).
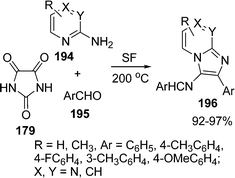 |
| Scheme 53 | |
The synthesis of triazolo[1,2-a]indazole-1,3,8-trione derivatives 199 in good yields (79–90%) has been reported via a three-component condensation of urazole 197, dimedone 166 and aromatic aldehydes 198 under solvent-free conditions at 80 °C in the presence of p-TSA as catalyst. Aliphatic aldehydes reacted poorly under similar conditions (Scheme 54).160
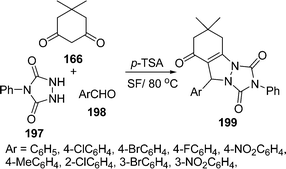 |
| Scheme 54 | |
After the pivotal discovery by Sharpless et al.,161 that Cu(I) catalyzes the formation of triazoles in a 1,4-substituted fashion, the chemistry of triazoles162 was brought from oblivion to renaissance. Triazole chemistry was revisited and has seen exponential growth over the years and an enormous gain in popularity in diverse areas of chemistry such as organic, material, and medicinal chemistry. The 1,2,3-Triazole moiety is present in many compounds exhibiting different biological properties such as antibacterial163 (cefmatilen), anti-HIV,164 antiallergic,165 and inhibitory166 (tazobactam) activities. Triazolobenzodiazepines167 have shown a high affinity toward benzodiazepine receptors. They are also emerging as powerful pharmacophores in their own right.
Furthermore, the importance of triazolopyrimidines is well recognized in the field of medicinal chemistry because these heterocycles have a structure similar to that of purine and adenine, differing in their fused ring systems having the pyrimidine nitrogen atom at the bridgehead position.168 Triazolo pyrimidines are useful building blocks in the synthesis of herbicidal drugs, such as Metosulam, Flumetsulam, Azafenidin, Diclosulam, Penoxsulam, Floransulan, and Cloransulam etc. Richardson et al.169 described the triazolopyrimidines as novel CDK2 inhibitors. In addition, these triazolopyrimidines are also useful potential anticancer,170anti-bronchoconstrictor,171 antiviral,172 diuretic,173 antibacterial,174 and antifungal175 agents.
Various synthetic protocols were reported for the synthesis of triazoles and their ring fused derivatives.176 Privileged scaffolds are uniquely suited to the preparation of molecular libraries for leading development in medicinal chemistry.177,178 Such frameworks are attractive for drug discovery because of the high hit rates and the pharmacological profiles of their derivatives relative to those of other ring systems. By varying substituents on these privileged scaffolds, one can often identify potent and selective binders for multiple biological targets from a single library. Dandia et al.179 have investigated regioselectivity in multicomponent reactions of aminotriazole 200, carbonyl compounds 201, and α-cyanoesters 202 in the synthesis of triazolopyrimidines 203 under solvent-free conditions in excellent yields using a microwave or ultrasonic wave (Scheme 55).
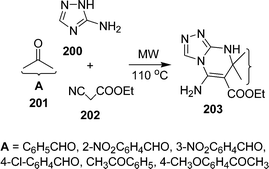 |
| Scheme 55 | |
4.5 Spiro-compounds
The synthesis of indenofused 5- and 6-membered heterocycles such as indenopyrole, indenopyrazole, indenothiophene, indenopyridine and indenopyrimidine has attracted considerable attention in recent years as these classes of compounds constitute structural frameworks of several naturally occurring compounds displaying a wide range of biological activity. The indenopyridine skeleton is present in the 4-azafluorenone group of alkaloids. It is represented by its simplest member, onychnine. Indenopyrazoles and indenopyridazines are known as cyclin-dependent kinase and selective MAO-B inhibitors. The spirooxindole system is the core structure of many pharmacological agents and natural alkaloids. A number of methods have been reported for the synthesis of spirooxindole-fused heterocycles. Recently, Bazgir et al.180 have reported a one-pot simple synthesis of spiro[diindenopyridine-indoline]triones 208, and spiro[acenaphthylene-diindenopyridine]triones 209 through a pseudo four-component reaction among 1,3-indandione 204, aromatic amines 205, and isatins 206 or acenaphthylene-1,2-dione 207 (Scheme 56).
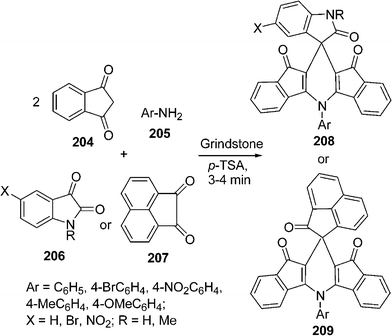 |
| Scheme 56 | |
Raghunathan and co-workers181 have reported a comparative study of the synthesis of novel dispiro pyrrolo/pyrrolizidino ring systems 210, 211 obtained by the cycloaddition of azomethine ylides. They are generated by a decarboxylation from sarcosine/proline and isatin with the dipolarophile 9-arylidine-fluorene using four different methodologies. Among them, the solvent-free microwave-assisted approach gave products with the highest yields in the shortest time. In addition to that, their solvent-free approach allowed the use of 4-N,N-dimethylaminobenzaldehyde, which failed to produce the desired cycloadducts under conventional processes (Scheme 57).
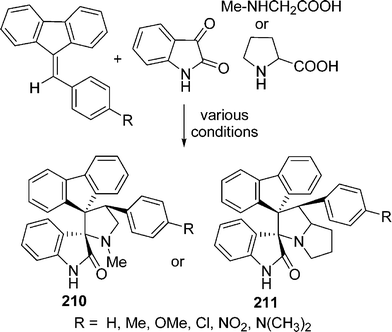 |
| Scheme 57 | |
Spiropyrrolidines/pyrrolizidines have been synthesized utilizing the alkene unit of Baylis–Hillman adducts of ninhydrin with sarcosine/proline and various activated ketones through 1,3-dipolar cycloaddition through microwave-assisted protocol in good yields.182 Raghunathan et al.183 have used TiO2–silica as an efficient solid-supported catalyst for the synthesis of a series of dispiroheterocyclic systems 212 and 213 through cycloaddition of an azomethine ylide generated by decarboxylation from tetrahydroisoquinoline-3-carboxylic acid and acenaphthenequinone/isatin with various unusual dipolarophiles e.g. 2-arylidene-1,3-indanediones and (E)-2-oxoindolino-3-ylidene acetophenones in a one-pot three-component tandem reaction (Scheme 58).
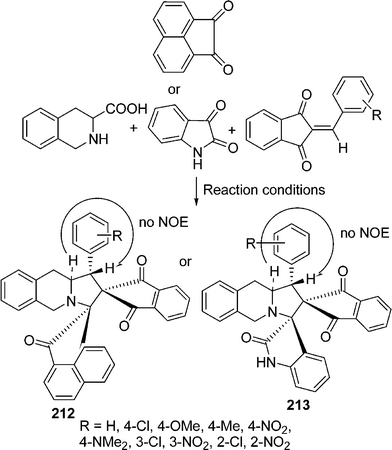 |
| Scheme 58 | |
Spiro-fused heterocycles 217 have been synthesized by Shaabani et al.184 in good yields by the pseudo four-component reaction of an aryl aldehyde 214, urea 215, and a cyclic β-diester or a β-diamide 216 such as Meldrum's acid or barbituric acid derivatives using microwave irradiation under solvent-free conditions. The best yields of the products were obtained in the presence of acetic acid or NaHSO4 (Scheme 59).
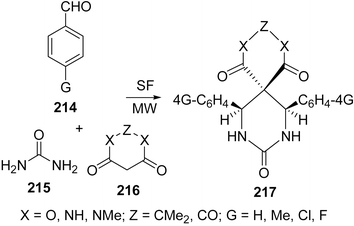 |
| Scheme 59 | |
Very recently, spirocompounds have been synthesized by Mobinikhaledi et al.,185 utilizing a mixture of isatin 206, malononitrile 85 or ethylcyanoacetate 218, and 219 or dimedone 166 in solvent-free media in the presence of a catalytic amount of TBAB to afford 2-amino-5-oxo-7,7-dimethyl spiro[(4H)-5,6,7,8-tetrahydrochromene-4,3′-(3′H)-indol]-(1′H)-2′-one-carbonitrile 220 or 221 in excellent yields. The reaction with malononitrile or ethyl cyanoacetate also proceeded smoothly (Scheme 60).
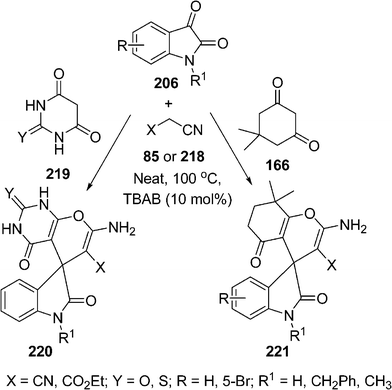 |
| Scheme 60 | |
4.6 Six-membered heterocycles
4.6.1 Containing one heteroatom.
Heterocycles, such as pyridines, pyridones, pyrans, chromenes, quinolines, isoquinolines, acridines, and xanthenes have become increasingly important because they have proven to be extremely useful intermediates for the preparation of new biological materials. These heterocycles are present in numerous pharmacologically and agrochemically important compounds.
4.6.1.1 Pyridines.
Pyridines are the building blocks of various compounds with important medicinal properties such as antitumor, antimicrobial,186 myastyhenia gravis,187 multiple sclerosis,188 spinal cord injuries,189 and botulism.190 For that reason a number of synthetic methodologies for the synthesis of 2-aminopyridines have been reported, including the condensation of α,β-unsaturated ketones with malononitrile in the presence of ammonium acetate,191 nucleophilic substitution of 2-halopyridines with primary or secondary amines,192 aminolysis of 2-alkoxypyridines, and [4 + 2]- or [3 + 3]-type ring formation reactions.193
A three-component cyclocondensation of enaminones 222, 1,3-dicarbonyls 223, and ammonium acetate 129 in the presence of tangstocobaltate salt as the heterogeneous catalyst has been reported by Kantevari et al.194 under solvent-free conditions. The protocol furnished the regioselective formation of 2,3,6-trisubstituted pyridines 224 in good yields (Scheme 61). The reaction was also carried out in solvent but the solvent-free conditions were found to be superior in terms of yield and time.
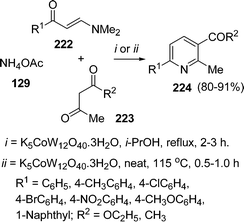 |
| Scheme 61 | |
4-Substituted 1,4-dihydropyridines (1,4-DHPs) are analogs of NADH coenzymes and an important class of drugs. These compounds exhibit various medicinal functions such as neuroprotectant, platelet anti-aggregatory, and cerebral antischemic activity in the treatment of Alzheimer's disease and chemosensitizing activity in tumor therapy. A series of 1,4-dihydropyridines 227 was obtained via sequential Hantzsch condensation catalyzed by morpholine in a one-pot reaction195 of 225, 226, 166, and 129 (Scheme 62). This reaction was also performed in solvent as well as under solvent-free conditions, but the best result was obtained in solvent-free conditions. Both electron-rich and electron-deficient aldehydes as well as heterocyclic aldehyde (furfural) worked well under this protocol.
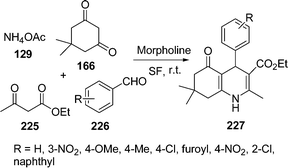 |
| Scheme 62 | |
Ishar and co-workers196 have synthesized some N-aryl-5,6-unsubstituted-1,4-dihydropyridines via a regioselective [4 + 2] cycloaddition of 1-aryl-4-phenyl-1-azadienes with allenic Esters. Sridharan and co-workers197 have synthesized 5,6-unsubstituted dihydropyridines using inert/anhydrous conditions in good yields (61-74%). Similar multicomponent reactions for the synthesis of substituted piperidines, dihydropyridones and tetrahydropyrans were also reported.198 Atul Kumar and Maurya199 have reported the use of organocatalysts for the multicomponent reaction of acetoacetate ester 228, cinnamaldehyde 229, and anilines 230 to yield N-aryl-5-unsubstituted or 5,6-unsubstituted 1,4-dihydropyridines 231 (Scheme 63). Basic amino acids like L-lysine and L-histidine give poor yields. Acidic amino acids were found to be superior but the best results were obtained with neutral amino acids like L-proline (90%) and L-pipecolic acid (85%).
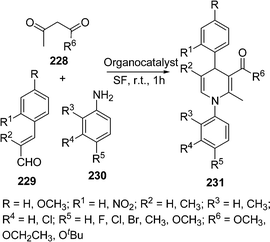 |
| Scheme 63 | |
Recently, Samai et al.200 reported a very simple and highly efficient one-pot method for the synthesis of unsymmetrical dihydro-1H-indeno[1,2-b]pyridine derivatives via a one-pot multicomponent strategy under solvent-free and catalyst-free conditions (Scheme 64). 4-Aryl-4,5-dihydro-1H-indeno[1,2-b]pyridines 234 have been synthesized via four-component cyclocondensation of 1,3-indanedione 204, aldehyde 232, β-ketoester 233 and ammonium acetate 129 in 1
:
1
:
1
:
1.5 ratios at room temperature on grinding.
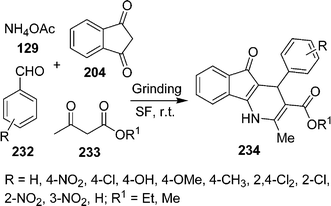 |
| Scheme 64 | |
The synthesis of 1,4-dihydropyridines 236 under solvent-free conditions have been reported by Wang et al.201 The reaction was performed under both with heating as well as with ultrasound irradiation. In case of ultrasound irradiation the mixture of ammonium acetate 129, ethyl acetoacetate 225, and aldehyde 235 in a molar ratio of 1.1
:
2.5
:
1.2 respectively, was irradiated in a water bath of ultrasonic cleaner with a nominal power of 250 W at 28–35 °C for a period of 25–70 min to give the desired products in 80–99% yields (Scheme 65). In all respects, the ultrasonic irradiation method was found to be superior to conventional heating. The reactivity of aldehydes with electron-withdrawing groups was found to be better than those with electron-donating groups.
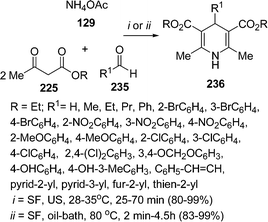 |
| Scheme 65 | |
Zolfigol and Safaiee202a have reported the synthesis of 1,4-dihydropyridines under solvent-free conditions using conventional thermal heating. The mixture of aldehyde, ethyl acetoacetate, and ammonium acetate in 1
:
2
:
1.5 molar ratios respectively, were heated at 80 °C to give the desired products in 83–99% yields. Recently, the synthesis of 2-trifluoromethyl-6-difluoromethylpyridine-3,5-dicarboxylates 239via one-pot three-component reaction of ethyl trifluoroacetoacetate 237, aldehydes 238, and ammonium acetate 129 in the presence of K2CO3 under solvent-free conditions through sequential Hantzsch reaction/dehydration/dehydrofluorination has been described202b (Scheme 66).
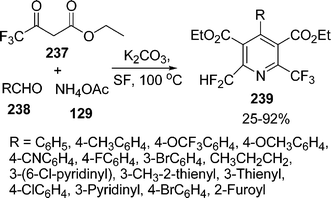 |
| Scheme 66 | |
Very recently, the synthesis of highly functionalized pyridine derivatives 241 has been reported by the domino coupling of readily available malononitrile 85 and cyclic ketones 240 in the presence of ammonium acetate 129 in one-pot under microwave irradiation and solvent-free conditions in high yields. The proposed mechanism involves a novel sequence consisting of deprotonation/imine formation/anionic carbonyl addition. The reaction was also performed in different solvents like HOAc, DMF, ethyl alcohol and water but the solvent-free method was found to be most efficient (Scheme 67).203
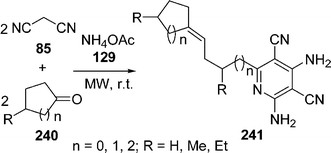 |
| Scheme 67 | |
Recently, one-pot three-component reaction for the synthesis of 1,4-dihydropyridines 245 has been developed by grinding of aldehydes 242, amines 243, DEAD (diethyl acetylenedicarboxylate) 244, and malononitrile 85/ethyl cyanoacetate 218 under catalyst-free and solvent-free domino protocol.204 First coupling occurs between aldehyde and active methylene compound followed by aza-Michael reaction with DEAD and aniline, which undergoes rearrangement to generate the desired product (Scheme 68).
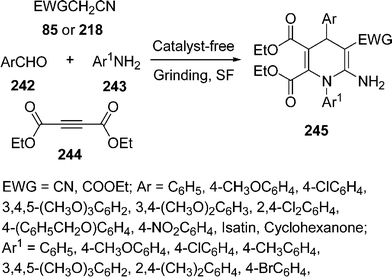 |
| Scheme 68 | |
Recently, Wells–Dawson heteropolyacids (H6P2W18O62·24H2O) are being used as a catalyst in various organic syntheses. Sanchez et al.205 have used it as recyclable catalyst in the synthesis of functionalized dihydropyridines 248 involving the Hantzsch reaction via the one-pot reaction of an aldehyde 246, a β -dicarbonyl compound 247 and an ammonia source i.e. ammonium acetate 129 (taken in a molar ratio of the reactants 3-formylchromone, methyl acetoacetate and ammonium acetate was 1
:
2
:
1, respectively), which provided the desired products in 60–99% yields. The pyridine functionalized at the 2-, 3-, and 5-positions was formed by opening the γ-pyrone ring after nucleophilic attack and subsequent cyclodehydration. Here, due to selectivity reasons, two products are formed in different ratios depending upon the substituents and the ratios of the starting materials (Scheme 69).
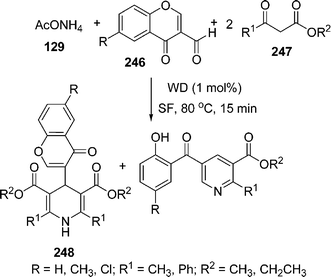 |
| Scheme 69 | |
Another method for the synthesis of 6-amino-5-cyano-1,4-dihydropyridine derivatives has been developed by the one-pot reaction of ethyl acetoacetate, [(2-aryl)methylene]malononitriles, and ammonium acetate at room temperature with grinding.206 Under microwave irradiation, the one-pot multicomponent condensation reaction of three molar aromatic aldehydes 249 with two molar cyclic ketones with free α,α′-methylene ethylene positions such as cyclopentanone or cyclohexanone 250 in the presence of ammonium acetate 129 and acetic acid afforded dicyclocalkenopyridines 251 in good yields. In similar reaction conditions, 1-tetralone, which has only one α-methylene position, results in 10-aryl-2,3:5,6-dibenzoacridines (Scheme 70).207
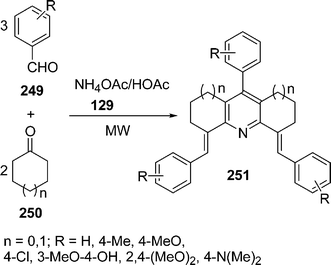 |
| Scheme 70 | |
4.6.1.2 Pyridinones.
Pyridinones constitute an important family of heterocyclic compounds for their potential pharmaceutical applications such as antibacterial and antifungal agents. Recently, the synthesis of 3,4-dihydropyridinones 255 by the one-pot multicomponent reaction of Meldrum's acid 252, methyl acetoacetate 253, and different aldehydes 254 in the presence of ammonium acetate 129 using microwave irradiation under solvent-free conditions (Scheme 71) has been reported.208a Equimolar amounts of the starting compounds were irradiated under microwave at 100–130 °C for 10–15 min providing 3,4-dihydropyridinones in 81–91% yields. Carrying out the above reaction under conventional thermal heating under the same experimental conditions gave lower yields. A one-pot synthesis of 4,6-diaryl-2-oxo-1,2-dihydropyridine-3-carbonitriles 259 has been reported in good yields via the cyclocondensation of aromatic ketones 256, aromatic aldehydes 257, and 2-cyanoacetamide 258 under solvent-free conditions (Scheme 72).208b
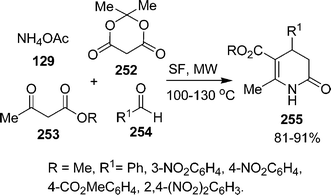 |
| Scheme 71 | |
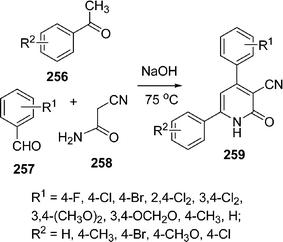 |
| Scheme 72 | |
4.6.1.3 Coumarins.
The coumarins (2H-chromen-2-ones, 2H-1-benzopyran-2-ones), an elite class of lactones, are important oxygen heterocycles that widely present as a structural motif in numerous natural products.209 Among the scaffolds of natural products, coumarins exhibit a broad range of biological210 and therapeutic211 activities together with various applications in technological212 fields. Several synthetic analogs of coumarins such as novobiocin, chlorobiocin, coumermycin, simocyclinone, demiflin, and flavaxate have been developed into useful drugs.213 Xanthotoxol (8-hydroxylpsoralen) is a natural psoralen with a good capability to treat Alzheimer's disease214a and 4-(4-phenoxybutoxy)-7H-furo[3,2-g]chromene-7-thione is a blocker214b of the lymphocyte potassium channel Kv1.3. Dibromo-7-hydroxy-4-methylchromen-2-one215a is the most promising inhibitor of Casein Kinase 2 (CK2). Other coumarin derivatives such as calanolide A, phenprocoumon, and warfarin have been reported as potent anti-HIV agents.215b
Recently, a convenient and one-pot synthesis of 3-aroyl/heteroaroyl-2H-chromene-2-thiones and benzo[f]2H-chromene-2-thiones has been developed by our group via the condensation of β-oxodithioesters and salicylaldehydes/α-hydroxynaphthaldehydes in the presence of indium trichloride216 under solvent-free conditions in high yields. Valizadeh et al.217, using a solventless system, have reported the synthesis of 5-amino-6-cyano-3-hydroxybenzo[c]coumarin derivatives 261via the one-pot three-component reaction of salicylaldehydes 260, malononitrile 85, and ethyl acetoacetate 225 over MgO at room temperature in good yields (Scheme 73).
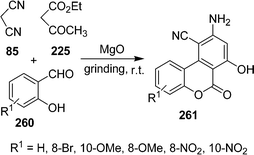 |
| Scheme 73 | |
4.6.1.4 Pyrans.
Pyrans and their derivatives are of special interest due to their biological activities. 4H-Benzo[b]pyrans are an important class of compounds, which have received considerable attention in recent years due to their wide range of biological activities.218 Compounds with this ring system have diverse pharmacological activities such as anti-coagulant, anticancer, spasmolytic, diuretic, anti-ancaphylactia, etc.219 4H-Pyrans also constitute the structural unit of a series of natural products.220 A number of 2-amino-4H-pyrans are useful as photoactive materials.221 In the conventional reported synthesis of 4H-benzo[b]pyrans, the use of organic solvents like DMF/acetic acid make the work-up procedure complicated and lead to poor yields of the products.222 Kaupp et al.223 reported a novel method for the synthesis of benzo[b]pyrans utilising the reactants in a solid or molten state. This reaction has its own merit but some limitations; the two-step reaction was performed at a very high temperature and required a longer period of time and limited scope. Devi and Bhuyan224 have reported a very simple and highly efficient method for the synthesis of 4H-benzo[b]pyrans 263via the three-component cyclocondensation reaction of 166, 262, and 85/218/258 under microwave irradiation using simple and inexpensive sodium bromide as the catalyst under solvent-free conditions (Scheme 74).
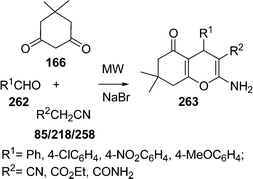 |
| Scheme 74 | |
Recently, Khalilzadeh and co-workers225 have developed the most useful and dependable procedure for the synthesis of S-alkyl dithiocarbamates and 2H-pyran-3,4-dicarboxylate 266 by using 264, 265, and 162/244 in one-pot under solvent-free conditions in the absence of a catalyst (Scheme 75). Radi et al.226 have produced a rapid protocol for the synthesis of 2,3-dihydropyran[2,3-c]pyrazoles 270 by multicomponent microwave-assisted organocatalytic DKHDA from 267, 268, and 269 under both solvent and solvent-free conditions. The above procedure has been utilized for the fast generation of substituted 2,3-dihydropyran[2,3-c]pyrazoles with potential anti-tuberculosis activity (Scheme 76).
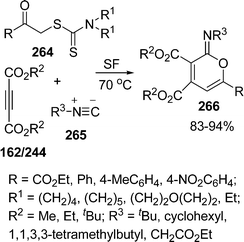 |
| Scheme 75 | |
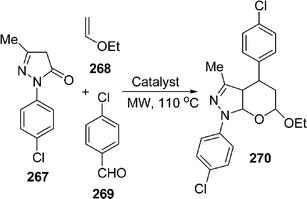 |
| Scheme 76 | |
The microwave-assisted three-component coupling of barbituric acids 271, benzaldehyde 272, and alkyl nitriles 273 has been carried out in the absence or presence of triethylamine under both solvent and solventless conditions to afford pyrano[2,3-d]pyrimidines 274 in good yields (Scheme 77).227 A series of novel 4-aryl-thiopyrano[3,4-b]pyran-5-one derivatives 277 or 278 were synthesized through the one-pot three-component reaction of an aromatic aldehyde 276, Meldrum's acid 252 or 85/218, and 275 under solvent-free conditions at 80 °C. The reaction has also been carried out in different solvents like CH3CN, CHCl3, EtOH, HOAc, and H2O, but the solvent-free method was found to be superior in terms of yields and time (Scheme 78).228
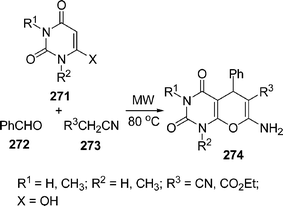 |
| Scheme 77 | |
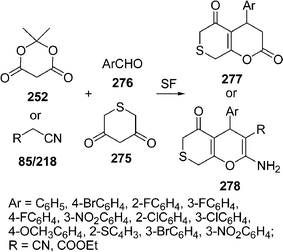 |
| Scheme 78 | |
4.6.1.5 Chromenes.
Chromenes represent an important class of compound as they are the main components of many naturally occurring products, and have been of interest in recent years due to their useful biological and pharmacological aspects, such as anticoagulant, spasmolytic, diuretic, insecticidal, anticancer, and antianaphylactic activity. 2-aminochromenes are widely employed as pigments, cosmetics, potential agrochemicals and also as components of many natural products.229–231 Thus, the development of an efficient methodology for the synthesis of 2-aminochromenes became highly essential. The most straightforward synthesis of this heterocyclic system involves a three-component coupling of aromatic aldehyde, malononitrile, and activated phenol in the presence of piperidine.232 A variety of catalysts such as basic alumina, cetyl trimethyl ammonium chloride, NaOH, InCl3, and I2/K2CO3 have been tested for this multicomponent reaction. Nanosized magnesium oxide has also been shown as an effective catalyst for this reaction.233 A simple, clean, and environmentally benign three-component process for the synthesis of 2-amino-4H-chromenes using N,N-dimethylaminoethylbenzyl dimethyl ammonium chloride, [PhCH2Me2N+CH2CH2NMe2]Cl−, as an efficient catalyst under solvent-free conditions has been described.234a Recently, Surpur and co-workers234b have reported the three-component condensation of aromatic aldehydes 279, malononitrile 85, and 1-naphthol 280 to form 281 by single-mode microwave irradiation under solvent-free conditions catalyzed by Mg/Al HT (Scheme 79).
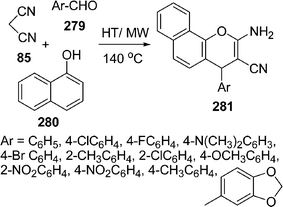 |
| Scheme 79 | |
Elinson and co-workers234c directly heated the mixture of salicylaldehyde 282, malononitrile 85 or methyl cyanoacetate 283, and nitroalkanes 284 at 60 °C in the presence of catalytic amounts of KF or NaOAc, resulting to the formation of 2-amino-4-(1-nitroalkyl)-4H-chromene-3-carbonitriles or methyl 2-amino-4-(1-nitroalkyl)-4H-chromene-3-carboxylates 285 in 80–90% yields (Scheme 80). The three-component coupling (3CC) of Kojic acid 286, aldehyde 287, and dimedone 166 has been achieved in the presence of 10 mol% of InCl3 under solvent-free conditions to afford the corresponding dihydropyrano[3,2-b]chromenedione derivatives 288 in good yields (Scheme 81).235
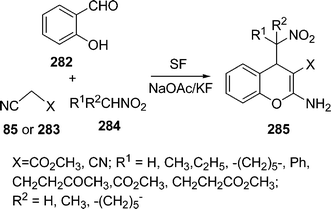 |
| Scheme 80 | |
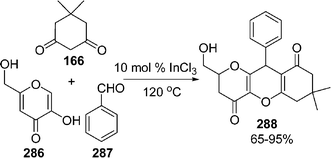 |
| Scheme 81 | |
Recently, Khurana and co-workers236 have developed a novel protocol for the synthesis of biscoumarin 291 and dihydropyrano[c]chromene derivatives 292 by the coupling of a series of aromatic, heteroaromatic and aliphatic aldehydes 289 with 4-hydroxycoumarin 290 and 85 catalyzed by tetrabutylammonium bromide (TBAB, 10 mol%) under solvent-free neat conditions. All aldehydes reacted almost equally well to afford biscoumarins in excellent yields (Scheme 82).
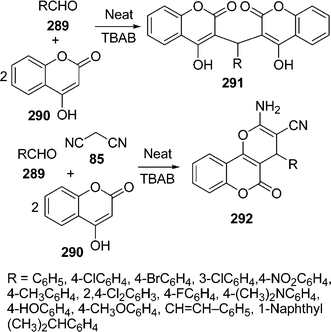 |
| Scheme 82 | |
4.6.1.6 Thiochromones.
Thiochromone derivatives are known to exhibit versatile pharmacological activity. A regioselective synthesis of 4-aryl-3-aroyl-2-methylsulfanyl-4,6,7,8-tetrahydrothiochromen-5-ones 296 has been developed by Singh et al.237via the annulation of β-oxodithioesters 293 with aldehydes 294 and cyclic 1,3-diketones 166/295 under solvent-free conditions promoted by P2O5 in good yields (72–90%). No co-catalyst or activator is needed in this protocol. Aliphatic aldehydes and heterocyclic aldehydes failed to produce the desired product, thus limiting the scope of the reaction to some extent (Scheme 83).
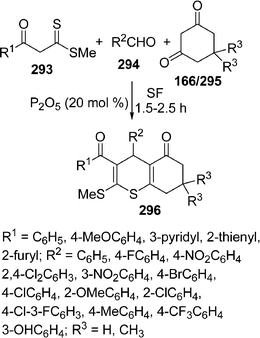 |
| Scheme 83 | |
4.6.1.7 Quinolines.
Quinolines and their derivatives are a very important class of compounds because of their wide occurrence in natural products238 and biologically active compounds.239 The classical method is the three-component coupling of an aldehyde, ethyl acetoacetate, and ammonia in acetic acid or refluxing alcohol.240,241 Kumar et al.242 have developed an efficient synthesis of polyhydroquinolines 299via the four-component reaction of aldehydes 297, dimedone 166, active methylene compounds 298, and ammonium acetate 129 simply by grinding under solvent-free conditions at room temperature (Scheme 84A). Sapkal and co-workers243 have prepared polyhydroquinoline derivatives 299 in one-pot via Hantzsch condensation of 297, 166, 298, and 129 using nanosized Nickel (Ni) as a heterogeneous catalyst. The method does not involve any hazardous organic solvent or catalyst. The smaller size of Ni (80 ± 0.5 nm) with a higher surface to volume ratio has given convenient features for the reaction response (Scheme 84B). Silica gel-supported polyphosphoric acid (PPA–SiO2) was found to be an efficient catalyst for the one-pot four-component Hantzsch condensation reaction of aryl aldehydes, dimedone, ethyl acetoacetate, and ammonium acetate to afford the corresponding polyhydroquinoline derivatives in high yields (Scheme 84C). The main advantages of the present approach are short reaction times, clean reaction profiles and simple experimental and workup procedures. The catalyst was recovered by simple filtration in excellent yields and used in the reaction three times as fresh catalyst without any significant loss of its activity.244 An efficient Hantzsch condensation of polyhydroquinoline derivatives 299 was reported via the four-component coupling reaction of 297, 166, 298, and 129 in the presence of HClO4–SiO2 under solvent-free conditions245 (Scheme 84D).
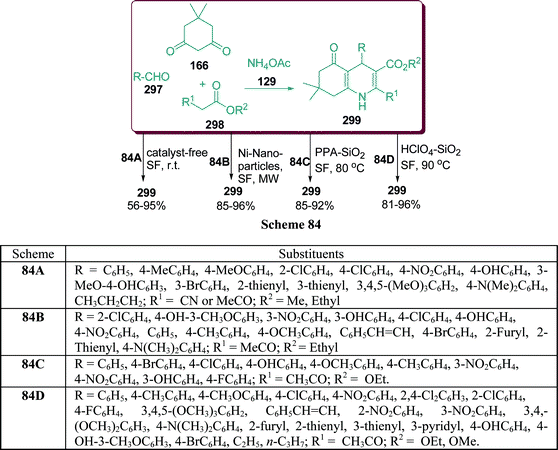 |
| Scheme 84 | |
A three-component cyclocondensation of enaminones 300, cyclic 1,3-dicarbonyl 166, and ammonium acetate 129 in the presence of tangstocobaltate salt as a heterogeneous catalyst has been reported by Kantevari et al.194 under solvent-free conditions. The protocol furnished the regioselective formation of 2,7,7-trisubstituted tetrahydroquinolin-5-ones 301 in good yields (Scheme 85). The reaction was also done in solvent but the solvent-free conditions were found to be superior. Wang et al.246 have reported an uncatalyzed and solvent-free multicomponent procedure for the synthesis of 1,4-arylquinoline derivatives 304 from 302, 85, and 303 (Scheme 86).
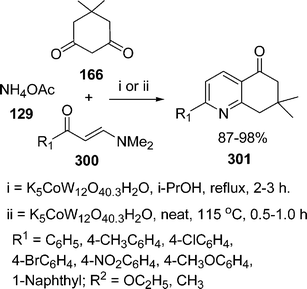 |
| Scheme 85 | |
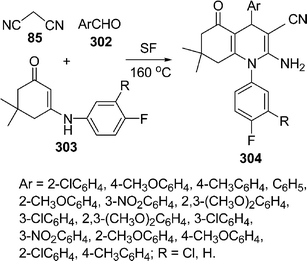 |
| Scheme 86 | |
Quiroga et al.247 have developed a methodology for the synthesis of 6,8-dihydro-5H-benzo[f]pyrazolo[3,4-b]quinolines 308 by the three-component reaction of 2-tetralone 305 or dimedone 166, 5-aminopyrazoles 306, and different benzaldehydes 307 under solvent-free conditions. The isomeric 6,10-dihydro-5H-benzo[h]pyrazolo [3,4-b]quinolines 309 were also obtained by the reaction of amines with benzylidene derivative of β-tetralone under similar conditions (Scheme 87). It has also been observed that sometimes 305 does not react and coupling between 306 and 307 forms 310 (Scheme 88).
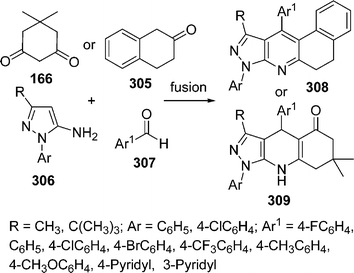 |
| Scheme 87 | |
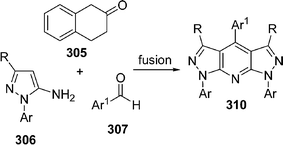 |
| Scheme 88 | |
A series of thieno[3,2-b]quinoline derivatives 314 were designed and synthesized efficiently via the one-pot three-component reaction of 311, 312, and 313 under solvent-free and catalyst-free conditions at 90 °C in 46–86% yields. Aromatic aldehydes with electron-withdrawing groups or electron-donating groups, heterocyclic and aliphatic aldehydes are tolerated well under this protocol. Different solvents such as AcOH, CHCl3, EtOH, DMF, and H2O were also used but solvent-free conditions provided the best results (Scheme 89).248
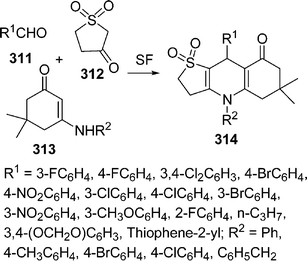 |
| Scheme 89 | |
4.6.1.8 Isoquinolines.
The isoquinoline backbone is present in numerous natural products and has different kinds of bioactivities.249,250 Thus, the isoquinoline family is of vital importance to both synthetic and medicinal chemists.251 Recently, Rong et al.252 reported an efficient MCR involving aromatic aldehyde 315, malononitrile 85, and 1-methylpiperidin-4-one 316 to prepare 6-amino-8-aryl-2-methyl-1,2,3,4-tetrahydroisoquinoline-5,7-dicarbonitrile 317 under solvent-free conditions at 70 °C in the presence of 0.1 g of NaOH as catalyst (Scheme 90).
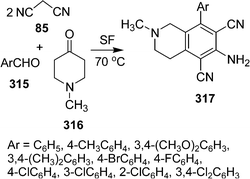 |
| Scheme 90 | |
4.6.1.9 Acridines.
The acridine moiety is an important structural unit present in many biologically important molecules such as amsacrine (cytotoxic, antiviral agent), botiacrine (antiparkinsonian drug), clomacran (tranquilizer), monometacrine (antidepressant), and in natural products such as plakinidine A and B and dercitin. Also, acridine derivatives have been employed or examined as antitumor agents, acetylcholinesterase inhibitors, anticancer agents, and DNA binding agents. Wang and Shen253 have synthesized 9-aryl-3,3,6,6-tetramethyl-hexahydroacridine-1,8-diones or 9,10-diaryl-3,3,6,6-tetramethyl-hexahydroacridine-1,8-diones 320 from 166, 318, and 129 or 319 respectively, under solvent-free conditions in high yields (90%). A rapid and efficient synthesis has been achieved using ammonium acetate 129 and p-toluidine 319 as the nitrogen source (Scheme 91).
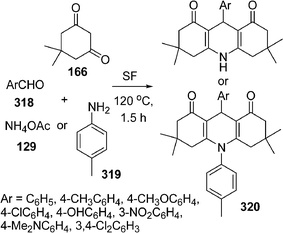 |
| Scheme 91 | |
4.6.1.10 Xanthenes.
Xanthenes and benzoxanthenes are important biologically active heterocyclic compounds. They possess antiviral,254anti-inflammatory,255 and antibacterial256 activities. These are used as antagonists for paralyzing action of zoxazolamine257 and in photodynamic therapy.258 In addition to that, these compounds can be used as dyes,259 in laser technologies260 and as pH sensitive fluorescent materials for the visualization of biomolecules.261 Nandi and co-workers262 have used Indium(III) chloride as a catalyst in the one-pot synthesis of 12-aryl/alkyl-8,9,10,12-tetrahydrobenzo[a]xanthenes-11-one derivatives 323. This has been achieved by the three-component cyclocondensation of aldehydes 321, β-naphthol 28, and the cyclic 1,3-dicarbonyl compound 322 under solvent-free conditions in high yields. P2O5 too has been found to be an effective catalyst towards this transformation (Scheme 92A). Wang et al.263 has also developed an efficient protocol for the synthesis of 12-aryl or 12-alkyl-8,9,10,12-tetrahydrobenzo[a]xanthen-11-one derivatives 323 by the three-component coupling of 321, 28, and 322 in the presence of 12-tungstophosphoric acid (H3PW12O40) under solvent-free conditions (Scheme 92B). The above methodologies both offer several advantages such as high yields, simple procedure, low cost, short reaction times, and mild conditions.
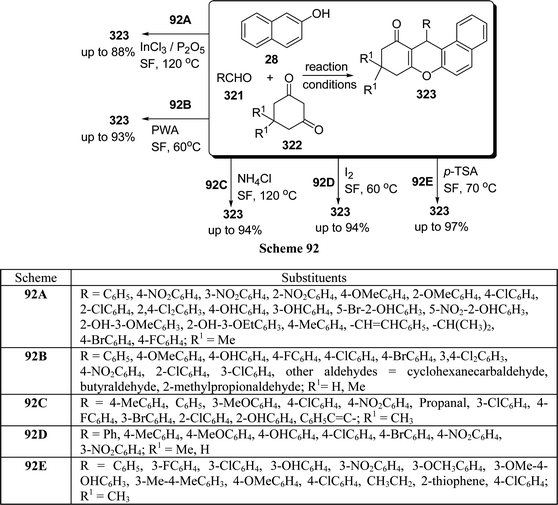 |
| Scheme 92 | |
The preparation of 12-aryl- or 12-alkyl-8,9,10,12-tetrahydrobenzo[a]xanthen-11-one derivatives using ammonium chloride (10 mol%) as a catalyst has been described under solvent-free conditions at 120 °C by the one-pot three-component reaction of 321, 28, and 322 in good yields (Scheme 92C).264 Wang and co-workers265 have described an alternative method for the synthesis of 12-aryl-8,9,10,12-tetrahydro-benzo[a]xanthen-11-one derivatives 323 by the coupling of 321, 28, and 322 catalyzed by iodine in one-pot under solvent-free conditions (Scheme 92D). A facile and efficient procedure has been developed by the one-pot coupling of 321, 28, and 322 for the synthesis of 8,9,10,12-tetrahydrobenzo[a]xanthen-11-one or 8,9-dihydrobenzo[f]cyclopenta[b]chromen-10(11H)-one derivatives 323 catalyzed by p-toluenesulfonic acid under solvent-free and sonication conditions (Scheme 92E).266 Recently, one more convenient multicomponent solvent-free protocol to synthesize this moiety has also been developed by Oskooie et al.267
Shaterian and co-workers268 have used ferric hydrogensulfate as a catalyst for the one-pot preparation of aryl-14H-dibenzo[a,j]xanthene derivatives 325 by the cyclocondensation of β-naphthol 28 and aromatic aldehydes 324 under solvent-free thermal conditions in 85–91% yields (Scheme 93A). Recently, a facile, efficient and environmentally friendly protocol for the synthesis of 14-aryl- or alkyl-14H-dibenzo[a,j]xanthenes 325 has been developed by Singh and co-workers269 through the one-pot condensation of 28 with 324 in the presence of P2O5 or InCl3 as catalysts under solvent-free conditions in high yield (Scheme 93 B).
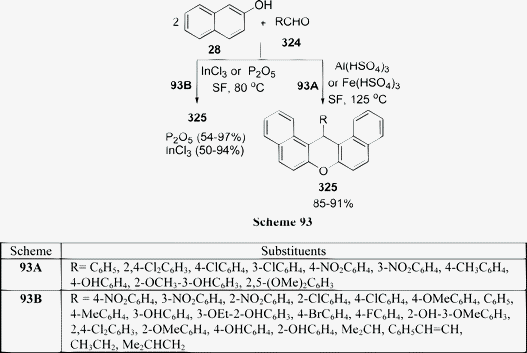 |
| Scheme 93 | |
Shen and co-workers253 have reported the synthesis of 9-aryl-3,3,6,6-tetramethyl-tetrahydroxanthene-1,8-diones 327 in nearly quantitative yields by the coupling of various aromatic aldehydes 326 and 5,5-dimethyl-1,3-cyclohexanedione 166 catalyzed by methanesulfonic acid under solvent-free conditions (Scheme 94).
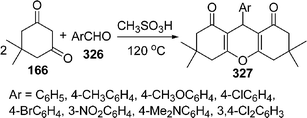 |
| Scheme 94 | |
4.6.2 Containing two heteroatoms.
4.6.2.1 Pyrimidines.
Pyrimidines are of chemical and pharmacological interest and compounds containing the pyrimidine ring system have been shown to possess antitumor, antibacterial, antifungal, antimalarial, and anticonvulsant activities.270–272 Pyrimidines have been synthesized using different procedures273–282 including the reaction of amidines with various functionalities, dimerization-oxidative fragmentation of β-arylvinylimines, condensation of phenacyldimethyl sulfonium salts, aldehydes, and ammonia, reaction of alkynes and nitriles in the presence of TfOH, rearrangement of 2,4,5-trisubstituted imidazolines, one-pot three-component reaction of aryl halides, terminal propargyl alcohols, and amidinium salts based upon a coupling/isomerization/cyclocondensation sequence, arylation of halogenated pyrimidines via Suzuki coupling reaction, the reaction of R,R-dibromo oxime ethers with Grignard reagents, microwave-assisted reaction of amidines and alkynones, and the sequential assembly of aryl groups onto a pyrimidine core (2-methylthiopyrimidine). Zhuang and co-workers283 have reported a novel one-pot three-component synthesis of multifunctionalized 2-amine-4,6-diarylpyrimidine scaffolds 331 through the condensation of aromatic aldehydes 328, aromatic ketones 329, and guanidine carbonate 330 under solvent-free conditions in 88–96% yields (Scheme 95).
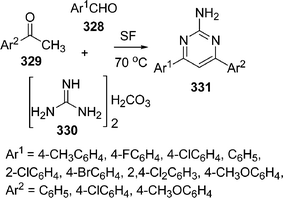 |
| Scheme 95 | |
Prajapati et al.284 have synthesized pyrimido[4,5-d]pyrimidine derivatives 334 of biological significance involving electron rich [(dimethylamino)methylene]amino uracil 332, which undergoes a [4 + 2] cycloaddition reaction with various glyoxylate imine 333, (generated in situ) followed by oxidative aromatisation under solvent-free conditions as well as microwave irradiation (Scheme 96).
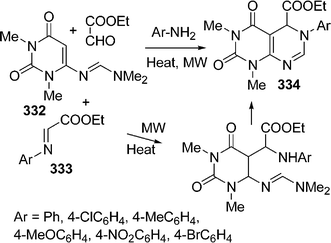 |
| Scheme 96 | |
An efficient and convenient method for the preparation of 2,4-diamino-6-arylpyrimidine-5-carbonitrile derivatives 336 has been reported by the one-pot reaction of aromatic aldehydes 335, malononitrile 85, and guanidine carbonate 330 in the presence of sodium hydroxide under solvent-free conditions in good yields (Scheme 97).285
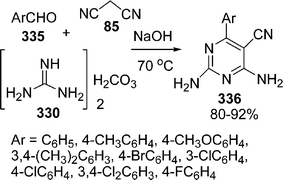 |
| Scheme 97 | |
4.6.2.2 Pyrimidinones and pyrimidinethiones.
3,4-Dihydropyrimidine-2-ones/thiones are useful targets in chemical synthesis as they have been associated with a diverse range of therapeutic and medicinal properties. The dihydropyrimidinone scaffold is also found in various marine alkaloids, which have been shown to possess antiviral, antitumor, antibacterial, and anti-inflammatory activities. Pyrimidinones are of considerable interest both in the industry and in academia because of their promising biological activities as calcium channel blockers, antihypertensive agents, and anticancer drugs.286
The simple and direct method for the synthesis of DHPMs known as the Biginelli reaction involves the one-pot condensation of an aldehyde, a β-ketoester, and urea under strong acidic conditions, which was first reported by Biginelli in 1893.287 However, the yields of products were low (20–50%). Since then, many new techniques such as microwave-assisted synthesis, ionic liquids, ultrasound irradiation, solvent-free conditions, and many new catalysts such as BF3,288a FeCl3,288b InCl3,288c BiCl3,288d LaCl3,288e LiClO4,288f Mn(OAc), CAN289etc., were used to improve this transformation. Dondoni and Massi290 have developed an efficient synthesis of an array of 3,4-dihydropyrimidin-2-(1H)-ones 339 using a solid-supported ytterbium(III) reagent from aldehydes 337, 1,3-dicarbonyl compounds 338, and urea 140/215 (Biginelli reaction) under solvent-free conditions (Scheme 98A). Jain and co-workers291 have reported a PEG-assisted solvent and catalyst-free synthesis of 3,4-dihydropyrimidinones 339 by the coupling of the same components under neutral reaction conditions (Scheme 98B). An efficient synthesis of dihydropyrimidinones 339 from the 337, 338, and 140/215 under solvent-free conditions using fluoroboric acid as the catalyst has been described by Chen et al.292
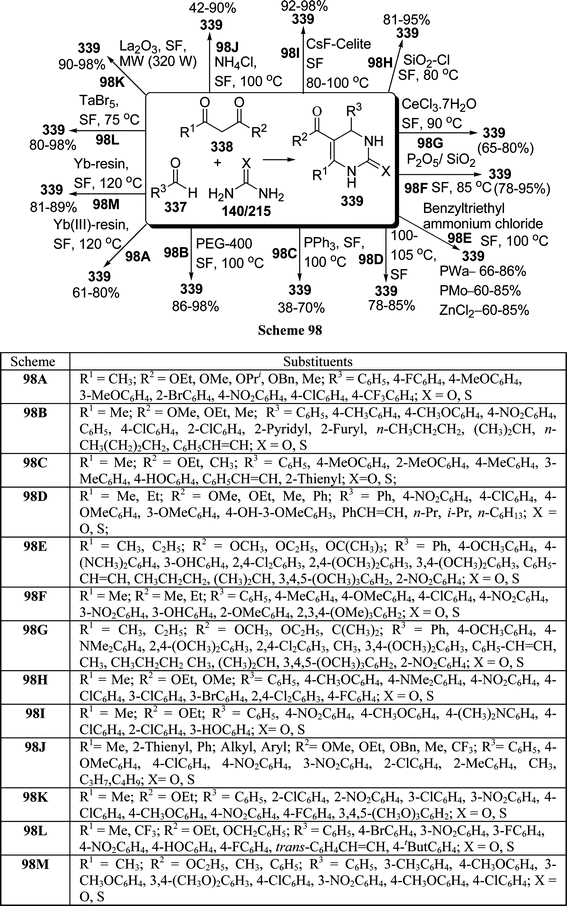 |
| Scheme 98 | |
The use of Lewis base TPP as a new catalyst for the one-pot Biginelli coupling of 337, 338, and 140/215 under neutral and solvent-free conditions to afford the corresponding dihydropyrimidinones 339 in moderate to good yields has been reported by Debache et al. (Scheme 98C).293 A simple, efficient, green, and cost-effective procedure for the synthesis of dihydropyrimidinones 339 has been developed by Ranu et al.294via the coupling of 337, 338, and 140/215 under solvent and catalyst-free conditions (Scheme 98D). Bose and co-workers295 have developed a simple, efficient, and cost-effective method for the synthesis of 3,4-dihydropyrimidin-2(1H)-ones 339via the one-pot three-component cyclocondensation of 337, 338, and 140/215 using benzyltriethylammonium chloride as the catalyst under solvent-free conditions (Scheme 98E). A synthetic procedure for the preparation of 3,4-dihydropyrimidin-2-(1H)-ones/thiones 339 from 337, 338, and 140/215 in the presence of a catalytic amount of silica-supported P2O5 (P2O5/SiO2) at 85 °C has been given by Hasaninejad et al.296 in high yields (Scheme 98F). A solvent-free coupling of 337, 338, and 140/215 in the presence of CeCl3·7H2O (30 mol%) afforded the corresponding dihydropyrimidinones 339 in 65–80% yields (Scheme 98G).297 Karade et al.298 described an improved procedure for the efficient and facile synthesis of 4-aryl substituted 3,4-dihydropyrimidinones 339 from 337, 338, and 140/215 under mild reaction conditions in excellent yields using inexpensive silica chloride under solvent-free conditions (Scheme 98H). Recently, a synthesis of 3,4-dihydropyrimidin-2-(1H)-ones 339via the coupling of 337, 338, and 140/215 catalyzed by CsF–Celite (10 mol%) has been developed by Chancharunee et al.299 (Scheme 98I). Shaabani and co-workers300 performed the Biginelli condensation in one-pot utilizing 337, 338, and 140/215 under solvent-free conditions at 100 °C using ammonium chloride as an efficient catalyst to afford the corresponding 3,4-dihydropyrimidin-2-(1H)-ones 339 in high yields (Scheme 98J). A synthesis of 3,4-dihydropyrimidinone 339 derivatives has been reported using lanthanum oxide as a catalyst, from aldehydes 337, 338, and 140/215 without solvent under the irradiation of microwave (98 K).301 Furthermore, A Biginelli reaction for the syntheses of 3,4-dihydropyrimidin-2-(1H)-ones (DHPMs) and their thione analogs 339, catalyzed by TaBr5 as a novel Lewis acid (5–10 mol%) under solvent-free conditions has been reported (98L).302 The solid catalyst combines the Lewis acid properties of the lanthanide and the advantage of solid phase. Due to the versatility of this new type of solid-phase Lewis acid, A ytterbium(III) reagent supported on ion exchange resin 340 is applied in a number of important organic transformations under solvent-free conditions. Yu et al.303 have developed a novel method to support the lanthanide(III) on ion exchange resin (Scheme 99).
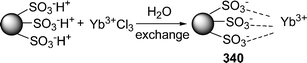 |
| Scheme 99 | |
The one-pot synthesis of 3,4-dihydropyrimidine-2(1H)-thiones 339 was described by Jiang and Chen304 by the cyclocondensation of 337, 338, and 140/215 utilizing recyclable ytterbium(III) reagent in good yields. They have prepared the Yb(III) catalyst supported on Amberlyst 15 resin which is a Yb-resin containing 0.75 mmol g−1 Yb(III), which was determined through EDTA titration. A series of solvents such as toluene, THF, DCM, MeCN, and H2O were tested. In all cases the reaction afforded the product in good yields. The yield of the desired compound was found to be best under solvent-free conditions (Scheme 98M).
Another simple and efficient synthesis of 3,4-dihydropyrimidinones/thiones 339via cyclocondensation of 337, 338, and 140/215 in the presence of silica-supported ceric sulfate [Ce(SO4)2–SiO2] as a heterogeneous catalyst at 110 °C under solvent-free conditions has been described.305 The heterogeneous catalyst Ce(SO4)2–SiO2 can be easily isolated by filtration from the reaction mixture, which was recovered and reused without further activation. Only 5% reduction in the catalytic activity was found during the consecutive use of recovered catalyst. A novel version of the Biginelli reaction using an acylal 341 as masked carbonyl functionality together with ethyl acetoacetate 225 and urea or thiourea 140/215 to give dihydropyrimidinones 342 has been reported by Khabazzadeh et al.306 The reaction is catalyzed by PWA, PMo or zinc chloride and has been performed in one-pot under solvent-free conditions (Scheme 100). In the optimized conditions, 10 mol%, 20 mol%, and 80 mol% of PWA, PMo, and ZnCl2 were used, respectively to get the best yields. The reaction was highly affected by the electronic factors and steric hindrance.
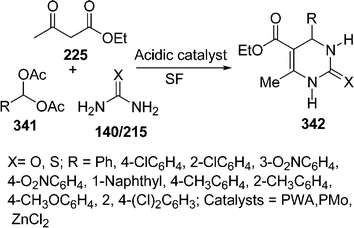 |
| Scheme 100 | |
Singh et al.307 have described a novel multicomponent one-pot protocol for the synthesis of dihydropyrimidinones 344 by the coupling of ketone 343, urea 140, and β-ketoester 225/253 using Zr-PILC as a mild recyclable solid acid catalyst under solvent-free conditions via both thermal and microwave heating (Scheme 101). A novel method for the synthesis of 5-unsubstituted-3,4-dihydropyrimidin-2(1H)-ones 347 has been developed from 215, 345, and 346 by microwave-assisted Biginelli reactions in a short and concise manner employing ZnI2 as a catalyst under solvent-free conditions (Scheme 102).308
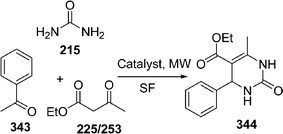 |
| Scheme 101 | |
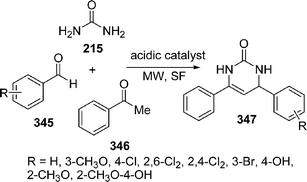 |
| Scheme 102 | |
A zeolite-catalyzed, simple, one-pot, solvent-free and environmentally benign process for the synthesis of dihydropyrimidones 349 from 225, 348, and 140/215 has been described by Kulkarni et al.309 This reaction is scaleable to the multigram scale and the catalyst is recyclable (Scheme 103). Trivalent lanthanides are usually considered hard acids, which can effectively activate the carbonyl and related compounds,310 for example, Ln(OTf)3 can catalyze a variety of important organic reactions under mild conditions.311,312
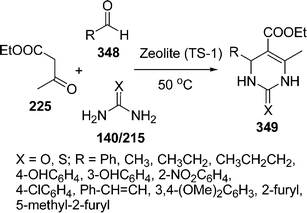 |
| Scheme 103 | |
Singh and co-workers313 have developed a one-pot three-component cyclocondensation reaction between aromatic aldehydes 350, cyclic-1,3-diketones 166/295 or 204 and 1,3-dimethylbarbituric acid 54 for the preparation of 9-oxa-1,3-diazaanthracene-2,4,5-triones 351 and 6,10-dihydro-5-oxa-6,8-diaza-benzo[b]fluorene-7,9,11-triones 352, which are of potential synthetic and pharmacological interest, in the presence of 10 mol% of InCl3 or 20 mol% of P2O5 under solvent-free conditions at 100 °C (Scheme 104). This protocol tolerates a variety of aromatic aldehydes containing both electron-withdrawing and electron-donating substituents at ortho-, meta- or para-positions. However, when some aliphatic aldehydes such as propionaldehyde, isobutyraldehyde, cinnamaldehyde, and cyclohexanecarboxaldehyde were used in this protocol under the above optimized conditions, it led to a mixture of products in low yields. This may be attributed to an aldol condensation as a side reaction.
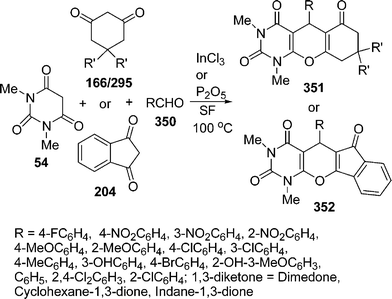 |
| Scheme 104 | |
Nandi and co-workers314 have developed a highly efficient InCl3 catalyzed three-component one-pot protocol for the synthesis of 8,12-dihydro-8,10-dimethyl-12-aryl-9H-naphtho[1′,2′:5,6]pyrano-[2,3-d]pyrimidine-9,11-(10H)-dione 355via the condensation of aldehydes 353, 2-naphthol 28, and 6-amino-1,3-dimethyluracil 354 under solvent-free conditions in good yields (Scheme 105). A synthesis of 3,4-dihydropyrimidin- 2(1H)-ones and thiones by condensation of ethylacetoacetate, aldehydes, and urea or thiourea in the presence of methylimidazolium hydrogensulfate has also been reported. Aromatic and aliphatic aldehydes reacted easily to afford the corresponding 3,4-dihydropyrimidin-2(1H)-ones and thiones under solvent-free conditions.315
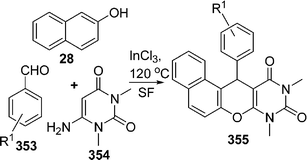 |
| Scheme 105 | |
Pyrimidinone derivatives 358 have also been synthesized from the reaction of aromatic aldehydes 356, cyclopentanone 357, and urea or thiourea 140/215 in the presence of N-(4-sulfonic acid) butyl triethyl ammonium hydrogen sulfate([TEBSA][HSO4]) as the Brønsted acidic ionic liquid as well as an effective catalyst under thermal and solvent-free conditions (Scheme 106).316
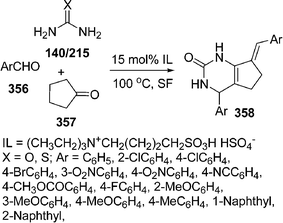 |
| Scheme 106 | |
The one-pot synthesis of substituted pyrimidine derivatives from acetylenedicarboxylate, amine, and orthoformate has been developed recently by Reddy and Nagarajan.317 The solvent and catalyst-free synthesis of pyrano[2,3-d]pyrimidine-2,4(1H,3H)-diones 361 has been described by the ball-milling of aldehyde 359, cyanoacetonitrile 85, and 360 by Naimi-Jamal and Mashkouri (Scheme 107).318
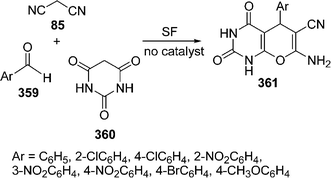 |
| Scheme 107 | |
A solvent-free one-pot three-component coupling between aldehyde 362, ketone 363, and urea 215 in the presence of iodine as a catalyst to give 4,6-diarylpyrimidin-2(1H)-ones (DAPMs) 364 in excellent yields (90–96%) has been described very recently by Reddy et al.319 (Scheme 108). The synthesis of polyfunctional tetrahydropyrimidines 367via a one-pot three-component reaction involving diethylacetylene dicarboxylate 244, amine 365, and aldehyde 366 in the presence of Lewis acid catalyst has been developed by Jiang and Zhang320 in good yields (Scheme 109).
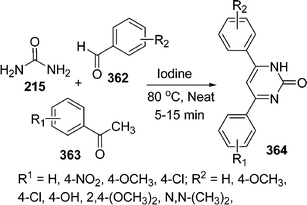 |
| Scheme 108 | |
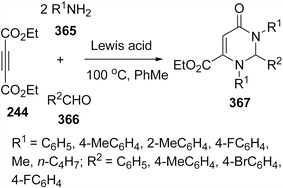 |
| Scheme 109 | |
A three-component one-pot synthesis of 4,6-diarylpyrimidin-2(1H)-ones and 9-phenyl-8-oxa-10,12-diazatricyclo[7.3.1.0]trideca-2(7),3,5-trien-11-one 370 has been reported by the condensation of acetophenone derivatives 368, aldehydes 369, and urea 215 in the presence of trimethylsilyl chloride in 1.5
:
1
:
1
:
1 molar ratios, respectively utilizing H6P2W18O62·18H2O as a catalyst under solvent-free conditions at 70 °C (Scheme 110).321
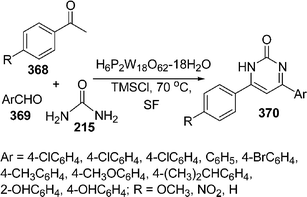 |
| Scheme 110 | |
4.6.2.3 Quinazolines.
Quinazoline compounds are known for their anticonvulsant,322 antihypertensive,323anti-inflammatory,324 and phosphodiesterase inhibitor properties.325 These properties created interest for the synthesis of the quinazolinic structure, which has led to a number of different synthetic pathways (e.g., Niementowski's synthesis,326 Bischler's synthesis,327 and Riedel's synthesis328). Quinazoline derivatives have also been synthesized from anthranilic acid329 and benzonitrile.330
Thus, considering the importance of this class of compounds, Rad-Moghadam and Samavi331 developed microwave-assisted as well as conventional thermal heating methods for the preparation of quinazolines 373 by the coupling of 2-aminobenzonitrile 371, orthoester 372, and ammonium acetate 129 in a molar ratio of 1
:
2
:
1, respectively under solvent-free conditions (Scheme 111). The excess of orthoester was necessary to diminish the side reactions. In the microwave-assisted synthesis, the products were obtained in 82–89% yields and the time needed for the formation of the products was 5–7 min and in the conventional thermal heating method it was observed that the yields were almost parallel (83–92%), although it required higher reaction time (30–80 min). The reaction has also been performed in refluxing ethanol for 180–240 min to furnish the products in 71–81% yields.
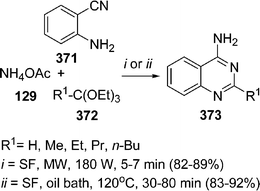 |
| Scheme 111 | |
4.6.2.4 Quinazolinones and quinazolinthiones.
Quinazolinones have various biological activities, including anticancer,332 antidiuretic,333 and anticonvulsant334 properties. The common synthetic methods for aryl-substituted quinazolinone derivatives contain cyclization of o-acylaminobenzamides,335 amidation of 2-aminobenzonitrile followed by oxidative ring closure,336 solid phase synthesis of 2-arylamino-substituted quinazolinones,337 reduction of the azide functionality,338 preparation from isatoic anhydrides and Schiff bases,339 and Pd-catalyzed heterocyclization of nitroarenes.340 The synthesis of 3-substituted quinazolin-4(3H)-ones 377via convergent coupling of formic acid 374, a primary amine 375, and isatoic anhydride 376 under solvent-free microwave irradiation conditions has been described by Rad-Moghadam and co-workers341 (Scheme 112).
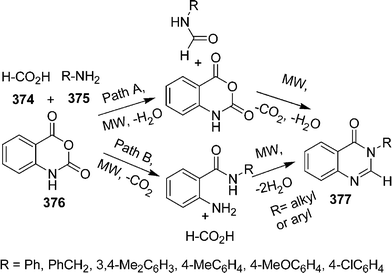 |
| Scheme 112 | |
A simple one-pot synthesis of 2,3-dihydroquinazoline-4(1H)-one derivatives under solvent-free conditions using a catalytic amount of iodine has been reported by Rostamizadeh et al.342 in excellent yields. This methodology provides easy, quantitative access to various 2,3-dihydroquinazoline-4(1H)-one derivatives 379, using 376, 378, and ammonium acetate 129 in the presence of commercially available iodine as a catalyst (Scheme 113).
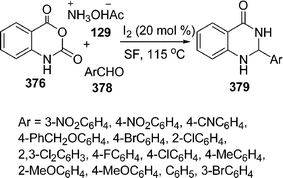 |
| Scheme 113 | |
Kidwai et al.343 reported a microwave-assisted protocol for the preparation of quinazolin-5-ones 381via classical Biginelli reaction using equimolar amounts of aldehyde 380, dimedone 166, and urea or thiourea 140/215 (Scheme 114). The mixture was irradiated in a domestic microwave oven to afford the products in 85–92% yields. When this reaction was performed in refluxing ethanol, the use of the catalyst Nafion-H (100 mol%) was required and the reaction took 10 h to complete, leading to the desired compound in a 72% yield, showing greater efficiency in the solvent-free method. Dandia and co-workers344 synthesized a series of disubstituted quinazolin-4-ones 385 from a multicomponent cyclocondensation reaction between anthranilic acid 382, phenyl acetyl chloride 383, and substituted anilines 384 utilizing equimolar amounts under solvent-free and domestic microwave oven conditions (Scheme 115). Products were obtained in 87–92% yields within a short reaction time (4–5 min).
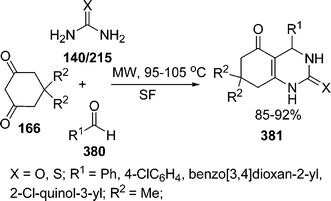 |
| Scheme 114 | |
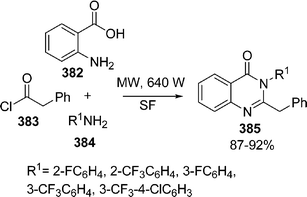 |
| Scheme 115 | |
Siddiqui and co-workers345 have reported the synthesis of 2-aryl-3-(β-D-ribofuranosyl)-3H-quinazolin-4-one 388 in good yields as a novel N-nucleoside by the one-pot three-component coupling of substituted/unsubstituted anthranilic acid 382, ribosylamine 386, and substituted/unsubstituted benzoic acid 387 in the presence of montmorillonite K-10 clay under solvent-free microwave irradiation conditions (Scheme 116).
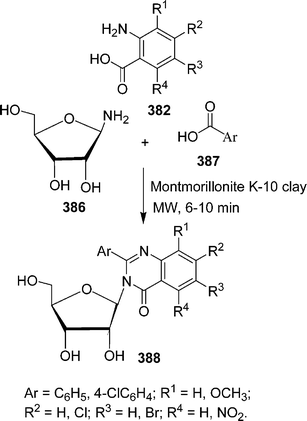 |
| Scheme 116 | |
As an extension of the study on the solid-supported reagent, Jiang et al.304 reported a one-pot synthesis of the derivatives of quinazolin-4(3H)-ones 391 from anthranilic acid 382, trialkyl orthoformate 389, and anilines 390 in the presence of a Yb-resin catalyst under solvent-free conditions (Scheme 117). Anilines with strong electron-withdrawing substitutes such as F and NO2 gave generally lower yields.
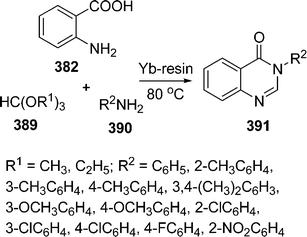 |
| Scheme 117 | |
4.6.2.5 Quinoxalines.
A microwave assisted one-pot three-component procedure was developed by Azizian et al.346 for the synthesis of some dicyanomethylene derivatives of indenoquinoxaline and tryptanthrin 394 under solvent-free conditions by the coupling of 392, 393, and 85 (Scheme 118).
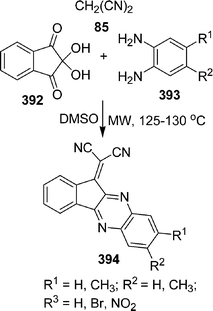 |
| Scheme 118 | |
4.6.2.6 Phthalazines.
The reported methods for the synthesis of phthalazine derivatives show varying degrees of success, and limitations include harsh reaction conditions, expensive catalysts/reagents, toxic organic solvents, low product yields, long reaction times, and co-occurrence of several side products. Therefore, there still remains a high demand for the development of more general, efficient, economically viable, and eco-compatible protocols to assemble such scaffolds. Very recently, we reported a facile one-pot synthesis of 2H-indazolo[2,1-b]phthalazine-1,6,11-triones 396 and 1H-pyrazolo[1,2-b]phthalazine-5,10-diones 397via the three-component coupling of phthalhydrazide 395, 1,3-diketones 166, and aldehydes 348/350 under solvent-free conditions at 80 °C as well as under solvent-free ultrasound irradiation at room temperature (Scheme 119).347
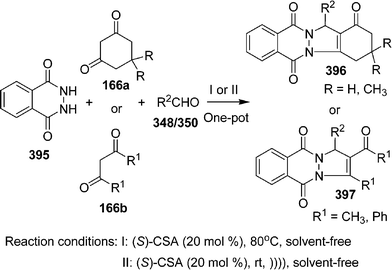 |
| Scheme 119 | |
4.6.2.7 Oxazines.
Oxazines have attracted special attention as pharmaceutical drug candidates and are amply represented in patent literature.348 In particular, 1,4-oxazines show interesting properties including antidepressant, anticancer, and anti-inflammatory activities, that explain the constant interest of synthetic chemists.349 The oxazine core has been synthesized by the Mitsunobu reaction, which involves a diol cyclization.350 Regnier et al.351 reported the synthesis of 2-aminomorpholines 402 under solvent-free and microwave assisted conditions by a straightforward four-component reaction between 1,2-aminoalcohols 398, glyoxal 399, boronic acids 400, and aliphatic or aromatic amines 401 (Scheme 120). The reaction was carried out under microwave irradiation at 110 °C and the reactants were used in a molar ratio of 1
:
1
:
1
:
1.5, respectively to afford the desired products in 43–77% yields.
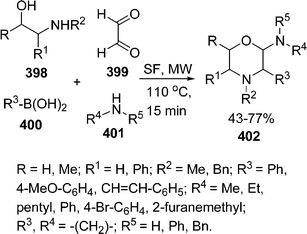 |
| Scheme 120 | |
A mixture of β-naphthol 28, arylaldehydes 403, and urea 215 in the presence of a catalytic amount of iodine as a catalyst under solvent-free conditions was heated for 5 min on a preheated hot plate to get the corresponding 1,2-dihydro-1-aryl-naphtho[1,2-e][1,3]oxazine-3-ones 404 in very good yields (Scheme 121).352
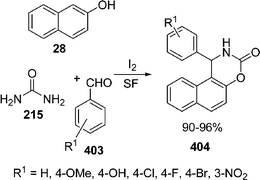 |
| Scheme 121 | |
4.6.2.8 Thiazines.
Thiazines are six-membered heterocycles containing four carbons, one nitrogen and one sulfur atom, which have been used for dyes, tranquillizers and insecticides. In the huge molecular library of heterocyclic compounds, nitrogen and sulfur are ubiquitous elements. The 1,3-thiazine nucleus is the active core of cephalosporins, which are among the most widely used lactam antibiotics. Because of their chemical and biological interest, syntheses of various 1,3-thiazines have been reported.353–356 1,3-Thiazines incorporating a thiol function are not accessible through any of the known synthetic routes.
Yadav et al.357 reported the synthesis of 1,3-thiazines 408 from the reaction of equimolar amounts of 1,3-oxathiolan-5-one 405, aromatic aldehydes 406, and an N-aryl dithiocarbamic acid 407 under microwave irradiation and solvent-free conditions for the first time. Although the authors did not discuss the microwave equipment used in the reaction, they reported that the mixture was irradiated for 8–10 min, affording 1,3-thiazines in 76–90% yields with >96% diastereoselectivity. The reactions were also carried out using a thermostat controlled oil bath at the same temperature (85 °C) for 3–5 h, which led to the respective 1,3-thiazines in 40–54% yields. A diastereomeric ratio of 96
:
4 was found in cases of reactions with MW activation, while a ratio of 55
:
45 was observed for conventional thermal heating (Scheme 122).
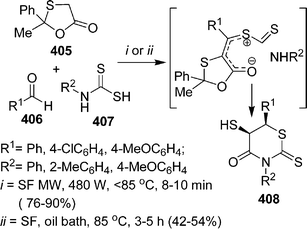 |
| Scheme 122 | |
Microwave irradiation was found to efficiently promote the three-component reaction of aromatic aldehyde 409, thiourea 140, and vinylbenzene 410 for the synthesis of 2-amino-4,6-diaryl-5,6-dihydro-4H-1,3-thiazines 411 in the presence of TMSCl under solvent-free conditions (Scheme 123).358 Both electron-withdrawing and electron-donating groups on both the aromatic aldehydes and vinylbenzene were tolerated well under the reaction conditions with no distinguishable electronic effects. When aliphatic aldehydes are used, no desired products were obtained.
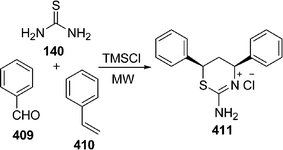 |
| Scheme 123 | |
4.7 Seven-membered heterocycles
4.7.1 Diazepines.
Compounds derived from the 1,4-benzodiazepine ring system, which has been suggested to serve as a structural mimic of peptide β-turns and α-helices bind to a multitude of targets, including G-protein coupled receptors, ligand-gated ion channels, and enzymes. Accordingly, it is hardly surprising that 1,4-benzodiazepines are found in a large number of pharmaceutical agents. Biologically active natural products such as asperlicin and anthramycin also possess 1,4-benzodiazepine substructure.
The synthesis of benzodiazepines is of interest, as they possess large number of pharmacological properties such as anticonvulsant, anti-anxiety, analgesic, hypnotic, sedative, antidepressant and anti-inflamatory agents. The benzodiazepines also used as valuable precursors in the synthesis of various fused heterocyclic systems such as triazolo-, oxazino-, oxadiazolo- and furano-benzodiazepines. In addition to this they also find a commercial use as dyes for acrylic fibers. An efficient and clean synthesis of benzodiazepines is described by the reaction of o-phenylenediamine with different types of methyl/methylene ketones in the presence of catalytic amounts of La(NO3)3·6H2O under solvent-free conditions at room temperature in excellent yields.359
A series of 6-substituted 4-sulfonyl-1,4-diazepane-2,5-diones were designed, synthesized, and evaluated as human chymase inhibitors.360 Structure activity relationship (SAR) studies led to the identification of a potent inhibitor, (6S)-6-(5-chloro-2-methoxybenzyl)-4-[(4-chlorophenyl)sulfonyl]-1,4-diazepane-2,5-dione, with an IC50 of 0.027 μM. A stereoselective one-pot synthesis of polysubstituted 1,4-diazepine derivatives 415 has been achieved by Sotoca et al.361via solvent and catalyst-free multicomponent domino reaction from β-ketoamides 412, aldehydes 413, and diamino compounds 414 (Scheme 124).
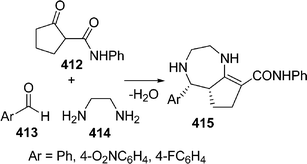 |
| Scheme 124 | |
5 Conclusions and outlook
The broad field of one-pot multicomponent procedures discussed in the sections above prove the fact that this field of research is a general method applicable to various synthesis examples in organic chemistry. The interest in synthesizing heterocycles has always been enormous. Combinatorial chemistry has gained significant importance as a tool for the synthesis of a wide variety of useful compounds including pharmaceuticals. In this context, the multiple component condensation (MCC) approach is especially appealing in view of the fact that products are formed in a single step, and the diversity can be readily achieved simply by varying the reacting components. The generation of small-molecule libraries requires the development of efficient methodologies with special emphasis on manipulative ease of the reaction. In conclusion, this eco-friendly, solvent-free multicomponent approach opens up numerous possibilities for conducting rapid organic synthesis and functional group transformations more efficiently. Additionally, there are distinct advantages of these solvent-free protocols since they provide reduction or elimination of solvents thereby preventing pollution in organic synthesis “at source”. The chemo-, regio- or stereoselective synthesis of high-value chemical entities and parallel synthesis to generate a library of small molecules will add to the growth of multicomponent under solvent-free reactions in the near future. In this review we have summarized the results reported mainly within the last 10 years. It is quite clear from the growing number of emerging publications in this field that the possibility to utilize multicomponent technology allows reaction conditions to be accessed that are very valuable for organic synthesis. Another important issue is the question of scale-up. Most of the reactions described in this review were performed in < 1 g scale, which reflects the current limitation of this technology when it comes to processing larger volumes. In order for multicomponent chemistry to be a valuable tool for the process chemist, new multicomponent scale-up technology needs to be developed, which is both economically viable and environmentally sustainable if one considers the energy balance. However, in comparison to solution-based processes, the solvent-free methods offer several advantages, making them an interesting tool for organic synthesis. In general, increased selectivities and reactivities have been found when working in one-pot multicomponent protocol. In some cases even unique selectivities have been demonstrated, which are not contrivable in solution. Thus, the route is opened for the synthesis of new products with advanced properties. As a consequence multi-component as well as domino or related reactions are witnessing a new spring. Coupling these one-pot processes with solid-phase synthesis offers new perspectives for the preparation of both primary and thematic libraries. The progresses recently made in this field that perfectly suits the needs of modern drug discovery are the subject of the present review.
Future strategies for the development of new MCRs will most likely focus more on the one-pot combination of sequential, orthogonal reactions. For example, (multicomponent) reactions with high functional group compatibility allow their straightforward one-pot combination with other reactions, thereby leading to highly atom- and step-economical procedures. Furthermore, modular reaction sequences allow a stepwise expansion of scaffold diversity. Some MCR purists may claim such sequential one-pot reactions are not true multicomponent reactions, since the reagents can not all be added simultaneously. In our opinion, it is more practical to consider what we wish to achieve with an MCR, that is, a practical, atom-economic, one-pot procedure that delivers complex products with high variability. For this purpose, a true MCR must: 1) involve a true one-pot procedure without intermediate workup; 2) incorporate essentially all of the atoms of the reactants into the product, with the exception of small condensation by-products, and 3) involve only inputs that can be independently varied. In addition, the variability of each of the components should be sufficient to ensure high overall appendage diversity.
6 Abbreviations
AAD | aldehydes, amides, and dienophiles |
Bmim | 1-butyl-3-methyl-1H-imidazolium |
CAN | cerric(IV)ammonium nitrate |
CDK2 | cyclin-dependent kinase 2 |
CMC | comprehensive medicinal chemistry |
DBU | 1,8-diazabicyclo(5.4.0)undec-7-ene |
DCC | 1,3-dicyclohexylcarbodiimide |
DEAD | diethyl azodicarboxylate |
DKHDA | domino Knoevenagel/hetero Diels–Alder reaction |
DMAD | dimethyl acetylenedicarboxylate |
DMAP | 4-(dimethylamino)pyridine |
DMF |
N,N-dimethylformamide |
HKA | heterocyclic ketene aminal |
HIV | Human Immuno deficiency Virus |
MCR | multicomponent reaction |
o-QM |
ortho-quinone methides |
per-6-ABCD | per-6-amino-β-cyclodextrin |
PMA-SiO2 | phosphomolybdic acid-silica |
PMo | 12-molybdophosphoric acid |
PTC | potassium thiocarbonate |
PTSA |
para-toluenesulfonic acid |
PWA | 12-tungstophosphoric acid |
SEM | scanning electron microscope |
TMSCl | trimethylsilyl chloride |
wet-TCT | wet-cyanuric chloride |
Zeolite(TS-1) | Zeolite Titanium silicalite-1 |
Zr-PILC | ZrO2-pillared clay |
Acknowledgements
We sincerely acknowledge the many colleagues and friends for their valuable suggestions and helpful discussions. We would like to thank our present co-workers Dr Ashutosh Gupta, Mr. R. K. Verma, Mr. G. C. Nandi, Mr. S. Samai, Mr. G. K. Verma, Mr. G. Shukla, Mr. T. Chanda, Mr. A. Nagaraju, Mr. Keshav, Mr. B. Jankiramulu and Mr. S. Koley whose significant contributions to the multicomponent reaction are presented or referred in this review. We are grateful to UGC, CSIR, and DST (New Delhi) for funding our research projects at various times. SC thanks UGC (New Delhi) for his research fellowship. Finally, I thank my students and colleagues who have contributed so much in terms of ideas and effort to our research programmes over many years and truly made the journey worthwhile.
References
-
(a) I. Ugi, Pure Appl. Chem., 2001, 73, 187 CrossRef CAS and references therein;
(b)
Multicomponent Reactions, ed. J. Zhu and H. Bienayme, Wiley-VCH, Weinheim, Germany, 2005 Search PubMed;
(c) A. Dömling, Chem. Rev., 2006, 106, 17 CrossRef;
(d) D. M. D'Souza and T. J. J. Mueller, Chem. Soc. Rev., 2007, 36, 3169 RSC;
(e) C. C. A. Cariou, G. J. Clarkson and M. Shipman, J. Org. Chem., 2008, 73, 9762 CrossRef CAS;
(f) A. Alizadeh, F. Movahedi and A. A. Esmaili, Tetrahedron Lett., 2006, 47, 4469 CrossRef CAS;
(g) M. Umkehrer, C. Kalinski, J. Kolb and C. Burdack, Tetrahedron Lett., 2006, 47, 2391 CrossRef CAS;
(h) A. Dömling and I. Ugi, Angew. Chem., Int. Ed., 2000, 39, 3168 CrossRef;
(i) D. J. Ramon and M. Yus, Angew. Chem., Int. Ed., 2005, 44, 1602 CrossRef CAS;
(j) D. Tejedor and F. Garcia-Tellado, Chem. Soc. Rev., 2007, 36, 484 RSC;
(k) R. A. V. Orru and M. de Greef, Synthesis, 2003, 1471 CrossRef CAS;
(l) J. Zhu, Eur. J. Org. Chem., 2003, 1133 CrossRef CAS;
(m) R. Ghahremanzadeh, S. Ahadi and A. Bazgir, Tetrahedron Lett., 2009, 50, 7379 CrossRef CAS;
(n) N. R. Candeias, F. Montalbano, P. M. S. D. Cal and P. M. P. Gois, Chem. Rev., 2010, 110, 6169 CrossRef CAS;
(o) A. V. Artemev, N. K. Gusarova, S. F. Malysheva, V. I. Mamatyuk, Y. V. Gatilov, I. A. Ushakov and B. A. Trofimov, Eur. J. Org. Chem., 2010, 6157 CrossRef CAS;
(p) T. H. Al-Tel, R. A. Al-Qawasmeh and W. Voelter, Eur. J. Org. Chem., 2010, 5586 CrossRef CAS;
(q) L. Banfi, A. Basso, L. Giardini, R. Riva, V. Rocca and G. Guanti, Eur. J. Org. Chem., 2011, 100 CrossRef CAS;
(r) K. Wang, D. Kim and A. Dömling, J. Comb. Chem., 2010, 12, 111 CrossRef CAS.
-
(a) A. Basso, L. Banfi, R. Riva and G. Guanti, J. Org. Chem., 2005, 70, 575 CrossRef CAS;
(b) S. T. Staben and N. Blaquiere, Angew. Chem., Int. Ed., 2010, 49, 325 CAS;
(c) I. Akritopoulou-Zanze, Curr. Opin. Chem. Biol., 2008, 12, 324 CrossRef CAS;
(d) T. Yue, M. -X. Wang, D. -X. Wang, G. Masson and J. Zhu, J. Org. Chem., 2009, 74, 8396 CrossRef CAS;
(e) B. A. Trofimov, L. V. Andriyankova, K. V. Belyaeva, A. G. Malkina, L. P. Nikitina, A. V. Afonin and I. A. Ushakov, Eur. J. Org. Chem., 2010, 1772 CrossRef CAS;
(f) N. Ma, B. Jiang, G. Zhang, S. -J. Tu, W. Wever and G. Li, Green Chem., 2010, 12, 1357 RSC.
-
(a) L. F. Tietze, Chem. Rev., 1996, 96, 115 CrossRef CAS;
(b) X. -H. Duan, X. -Y. Liu, L. -N. Guo, M. -C. Liao, W. -M. Liu and Y. -M. Liang, J. Org. Chem., 2005, 70, 6980 CrossRef CAS;
(c) S. -L. Cui, X. -F. Lin and Y. -G. Wang, J. Org. Chem., 2005, 70, 2866 CrossRef CAS;
(d) S. -J. Tu, B. Jiang, R. -H. Jia, J. -Y. Zhang, Y. Zhang, C. -S. Yao and F. Shi, Org. Biomol. Chem., 2006, 4, 3664 RSC;
(e) S. -J. Tu, B. Jiang, R. -H. Jia, J. -Y. Zhang and Y. Zhang, Tetrahedron Lett., 2007, 48, 1369 CrossRef CAS;
(f) H. -L. Wei, Z. -Y. Yan, Y. -N. Niu, G. -Q. Li and Y. -M. Liang, J. Org. Chem., 2007, 72, 8600 CrossRef CAS;
(g) S. -L. Cui, J. Wang and Y. -G. Wang, Org. Lett., 2007, 9, 5023 CrossRef CAS;
(h) S. -L. Cui, J. Wang and Y. -G. Wang, Org. Lett., 2008, 10, 1267 CrossRef CAS;
(i) X. -H. Chen, W. -Q. Zhang and L.- Z. Gong, J. Am. Chem. Soc., 2008, 130, 5652 CrossRef CAS;
(j) N. Li, X. -H. Chen, J. Song, S. -W. Luo, W. Fan and L. -Z. Gong, J. Am. Chem. Soc., 2009, 131, 15301 CrossRef CAS;
(k) Z. -L. Shen, X. -P. Xu and S. -J. Ji, J. Org. Chem., 2010, 75, 1162 CrossRef CAS;
(l) X. -Y. Guan, L. -P. Yang and W. -H. Hu, Angew. Chem., Int. Ed., 2010, 49, 2190 CrossRef CAS;
(m) C. L. Floch, E. L. Gall, E. Léonel, J. Koubaa, T. Martens and P. Retailleau, Eur. J. Org. Chem., 2010, 5279 CrossRef.
-
(a) I. Ugi, A. Dömling and W. Horl, Endeavour, 1994, 18, 115 CrossRef CAS;
(b) C. Hulme and V. Gore, Curr. Med. Chem., 2003, 10, 51 CrossRef CAS;
(c) J. D. Sunderhaus, C. Dockendorff and S. F. Martin, Org. Lett., 2007, 9, 4223 CrossRef CAS;
(d) R. W. Armstrong, A. P. Combs, P. A. Tempest, S. D. Brown and T. A. Keating, Acc. Chem. Res., 1996, 29, 123 CrossRef CAS;
(e) F. L. Muller, T. Constantieux and J. Rodriguez, J. Am. Chem. Soc., 2005, 127, 17176 CrossRef;
(f) C. Haurena, E. L. Gall, S. Sengmany, T. Martens and M. Troupel, J. Org. Chem., 2010, 75, 2645 CrossRef CAS.
-
(a) L. F. Tietze and M. E. Lieb, Curr. Opin. Chem. Biol., 1998, 2, 363 CrossRef CAS;
(b) S. L. Dax, J. J. McNally and M. A. Youngman, Curr. Med. Chem., 1999, 6, 255 CAS;
(c) B. Willy and T. J. J. Muller, Eur. J. Org. Chem., 2008, 4157 CrossRef CAS;
(d) M. M. Heravi, B. Baghernejad, H. A. Oskooie and R. Hekmatshoar, Tetrahedron Lett., 2008, 49, 6101 CrossRef CAS;
(e) M. Adib, E. Sheikhi, A. Kavoosi and H. R. Bijanzadeh, Tetrahedron, 2010, 66, 9263 CrossRef CAS.
-
(a) A. Dömling, Comb. Chem. High T. Scr., 1998, 1, 1 Search PubMed;
(b) N. M. Evdokimov, A. S. Kireev, A. A. Yakovenko, M. Y. Antipin, I. V. Magedov and A. Kornienko, J. Org. Chem., 2007, 72, 3443 CrossRef CAS;
(c) W. -B. Chen, Z. -J. Wu, Q. -L. Pei, L. -F. Cun, X. -M. Zhang and W. -C. Yuan, Org. Lett., 2010, 12, 3132 CrossRef CAS.
-
(a) Y. -L. Zhu, S. -L. Huang and Y. -J. Pan, Eur. J. Org. Chem., 2005, 2354 CrossRef CAS;
(b) Y. -L. Zhu, Y. -J. Pan and S. -L. Huang, Heterocycles, 2005, 65, 133 CrossRef CAS;
(c) V. A. Chebanov, V. E. Saraev, S. M. Desenko, V. N. Chernenko, I. V. Knyazeva, U. Groth, T. N. Glasnov and C. O. Kappe, J. Org. Chem., 2008, 73, 5110 CrossRef CAS;
(d) Y. I. Sakhno, S. M. Desenko, S. V. Shishkina, O. V. Shishkin, D. O. Sysoyev, U. Groth, C. O. Kappe and V. A. Chebanov, Tetrahedron, 2008, 64, 11041 CrossRef CAS;
(e) F. Sha and X. Huang, Angew. Chem., Int. Ed., 2009, 48, 3458 CrossRef CAS;
(f) E. A. Muravyova, S. V. Shishkina, V. I. Musatov, I. V. Knyazeva, O. V. Shishkin, S. M. Desenko and V. A. Chebanov, Synthesis, 2009, 1375 CAS;
(g) A. Znabet, E. Ruijter, F. J. J. de Kanter, V. Kóhler, M. Helliwell, N. J. Turner and R. V. A. Orru, Angew. Chem., Int. Ed., 2010, 49, 5289 CrossRef CAS;
(h) B. Zhang, L. Cai, H. Song, Z. Wang and Z. He, Adv. Synth. Catal., 2010, 352, 97 CrossRef CAS;
(i) J. Barluenga, M. G. Suero, R. D. la Campa and J. Flórez, Angew. Chem., Int. Ed., 2010, 49, 9720 CrossRef CAS.
-
(a)
K. Tanaka and F. Toda, Solvent-free Organic Synthesis; Wiley-VCH: Weinheim, 2003 Search PubMed;
(b) J. G. Hernández and E. Juaristi, J. Org. Chem., 2010, 75, 7107 CrossRef;
(c)
P. T. Anastas, J. C. Warner, Green Chemistry: Theory and Practice; Oxford University Press: New York 1998 Search PubMed;
(d) S. Li, J. -X. Wang, X. Wen and X. Ma, Tetrahedron, 2011, 67, 849 CrossRef CAS;
(e)
W. M. Nelson, Green Solvents for Chemistry−Perspectives and Practice; Oxford University Press: Oxford, New York, 2003 Search PubMed;
(f) R. A. Sheldon, Green Chem., 2005, 7, 267 RSC.
-
(a) B. M. Trost, Science, 1991, 254, 1471 CAS;
(b) A. Corma and H. Garcia, Chem. Rev., 2003, 103, 4307 CrossRef CAS.
-
(a) F. Toda, Acc. Chem. Res., 1995, 28, 480 CrossRef CAS;
(b) S. J. Ji, Z. L. Shen, D. G. Gu and X. Y. Huang, Ultrason. Sonochem., 2005, 12, 161 CrossRef CAS;
(c) Z. L. Shen, S. J. Ji and T. P. Loh, Tetrahedron Lett., 2005, 46, 507 CrossRef CAS;
(d) Z. L. Shen, S. J. Ji, W. J. Zhou and J. M. Yang, Synth. Commun., 2005, 35, 1903 CrossRef CAS;
(e) J. M. Yang, S. -J. Ji, D. -G. Gu, Z. L. Shen and S.-Y. Wang, J. Organomet. Chem., 2005, 690, 2989 CrossRef CAS;
(f) T. S. Jin, J. S. Zhang, A. Q. Wang and T. S. Li, Synth. Commun., 2005, 35, 2339 CrossRef CAS;
(g) T. S. Jin, G. L. Feng, M. N. Yang and T. S. Li, Synth. Commun., 2004, 34, 1277 CrossRef CAS;
(h) S. J. Ji, J. Lu, X. Zhu, J. Yang, J. P. Lang and L. Wu, Synth. Commun., 2002, 32, 3069 CrossRef CAS;
(i) S. J. Tu, Q. H. Wei, H. J. Ma, D. Q. Shi, Y. Gao and G. Y. Cui, Synth. Commun., 2001, 31, 2657 CrossRef CAS;
(j) Z. L. Shen and S. J. Ji, Synth. Commun., 2009, 39, 775 CrossRef CAS;
(k) R. A. Sheldon, Pure Appl. Chem., 2000, 72, 1233 CrossRef CAS.
- J. M. DeSimone, Science, 2002, 297, 799 CrossRef CAS.
-
P. T. Anastas and T. C. Williamson, Green Chemistry: Frontiers in Benign Chemical Syntheses and Processes; Oxford Science Publications: New York, 1998 Search PubMed.
- R. A. Sheldon, Chem. Ind., 1992, 903 CAS.
- G. W. V. Cave, C. L. Raston and J. L. Scott, Chem. Commun., 2001, 2159 RSC.
- J. O. Metzger, Angew. Chem., Int. Ed., 1998, 37, 2975 CrossRef CAS.
- K. Tanaka and F. Toda, Chem. Rev., 2000, 100, 1025 CrossRef CAS.
- Y. Wang, X. Teng, J. Wang and H. Yang, Nano Lett., 2003, 3, 789 CrossRef CAS.
- B. Nilsson, H. M. Vargas, B. Ringdahl and U. Hacksell, J. Med. Chem., 1992, 35, 285 CrossRef CAS.
-
(a) K. Hattori, M. Miyata and H. Yamamoto, J. Am. Chem. Soc., 1993, 115, 1151 CrossRef CAS;
(b) A. Jenmalm, W. Berts, Y. L. Li, K. Luthman, I. Csoregh and U. Hacksell, J. Org. Chem., 1994, 59, 1139 CrossRef CAS;
(c) M. Miura, M. Enna, K. Okuro and M. Nomura, J. Org. Chem., 1995, 60, 4999 CrossRef CAS;
(d) G. Gao, F. Sanda and T. Masuda, Macromolecules, 2003, 36, 3932 CrossRef CAS;
(e) D. A. Black and B. A. Arndtsen, Org. Lett., 2004, 6, 1107 CrossRef CAS.
-
(a) M. Konishi, H. Ohkuma, T. Tsuno, T. Oki, G. D. VanDuyne and J. Clardy, J. Am. Chem. Soc., 1990, 112, 3715 CrossRef CAS;
(b) K. B. Sanders, A. J. Thomas, M. R. Pavia, R. E. Davis, L. L. Coughenour, S. L. Myers, S. Fischer and W. H. Moos, Bioorg. Med. Chem. Lett., 1992, 2, 803 CrossRef CAS;
(c) M. A. Huffman, N. Yasuda, A. E. DeCamp and E. J. J. Grabowski, J. Org. Chem., 1995, 60, 1590 CrossRef CAS;
(d) L. W. Bieber and M. F. da Silva, Tetrahedron Lett., 2004, 45, 8281 CrossRef CAS.
-
(a) C. W. Ryan and C. Ainsworth, J. Org. Chem., 1961, 26, 1547 CrossRef CAS;
(b) F. Tubery, D. S. Grierson and H. P. Husson, Tetrahedron Lett., 1987, 28, 6457 CrossRef CAS;
(c) M. E. Jung and A. Huang, Org. Lett., 2000, 2, 2659 CrossRef CAS;
(d) T. Murai, Y. Mutoh, Y. Ohta and M. Murakami, J. Am. Chem. Soc., 2004, 126, 5968 CrossRef CAS.
- C. Mannich and F. T. Chang, Ber. Dtsch. Chem. Ges., 1933, 66, 418 CrossRef.
-
(a) M. A. Youngman and S. L. Dax, J. Comb. Chem., 2001, 3, 469 CrossRef CAS;
(b) S. L. Dax, M. A. Youngman, P. Kocis and M. North, Solid-Phase Organic Syntheses, 2001, 1, 45 CrossRef CAS;
(c) A. B. Dyatkin and R. A. Rivero, Tetrahedron Lett., 1998, 39, 3647 CrossRef CAS;
(d) J. J. McNally, M. A. Youngman and S. L. Dax, Tetrahedron Lett., 1998, 39, 967 CrossRef CAS.
-
(a) G. W. Kabalka, L. Wang and R. M. Pagni, Synlett, 2001, 676 CAS;
(b) L. Wang and P. H. Li, Chin. J. Chem., 2003, 21, 710 CAS.
-
(a) F. Colombo, M. Benaglia, S. Orlandi and F. Usuelli, J. Mol. Catal. A: Chem., 2006, 260, 128 CrossRef CAS;
(b) N. Gommermann and P. Knochel, Chem.–Eur. J., 2006, 12, 4380 CrossRef CAS;
(c) A. Bisai and V. K. Singh, Org. Lett., 2006, 8, 2405 CrossRef CAS.
- P. Li and L. Wang, Tetrahedron, 2007, 63, 5455 CrossRef CAS.
- G. W. Kabalka, L. -L. Zhou, L. Wang and R. M. Pagni, Tetrahedron, 2006, 62, 857 CrossRef CAS.
- S. Samai, G. C. Nandi and M. S. Singh, Tetrahedron Lett., 2010, 51, 5555 CrossRef CAS.
-
(a) J. R. Casimir, C. Turetta, L. Ettouati and J. Paris, Tetrahedron Lett., 1995, 36, 4797 CAS;
(b) A. G. Godfrey, D. A. Brooks, L. A. Hay, M. Peters, J. R. McCarthy and D. Mitchell, J. Org. Chem., 2003, 68, 2623 CrossRef CAS.
-
(a) D. Bahulayan, S. K. Das and J. Iqbal, J. Org. Chem., 2003, 68, 5735 CrossRef CAS;
(b) I. N. Rao, E. N. Prabhakaran, S. K. Das and J. Iqbal, J. Org. Chem., 2003, 68, 4079 CrossRef CAS;
(c) E. N. Prabhakaran and J. Iqbal, J. Org. Chem., 1999, 64, 3339 CrossRef CAS;
(d) B. Bhatia, M. M. Reddy and J. Iqbal, J. Chem. Soc., Chem. Commun., 1994, 713 RSC;
(e) R. Ghosh, S. Maiti and A. Chakraborty, Synlett, 2005, 115 CrossRef CAS.
- G. Pandey, R. P. Singh, A. Garg and V. K. Singh, Tetrahedron Lett., 2005, 46, 2137 CrossRef CAS.
- M. M. Khodaei, A. R. Khosropour and P. Fattahpour, Tetrahedron Lett., 2005, 46, 2105 CrossRef CAS.
- R. P. Bhat, V. P. Raje, V. M. Alexander, S. B. Patil and S. D. Samant, Tetrahedron Lett., 2005, 46, 4801 CrossRef CAS.
- E. Rafiee, T. Fariba and M. Joshaghani, Bioorg. Med. Chem. Lett., 2006, 16, 1221 CrossRef CAS.
-
(a) L. Nagarapu, V. N. Cheemalapati, S. Apuri, N. V. Kumari and S. Kantevari, J. Mol. Catal. A: Chem., 2007, 264, 22 CrossRef CAS;
(b) L. Nagarapu, R. Bantu and R. Puttireddy, Appl. Catal., A, 2007, 332, 304 CrossRef CAS.
- R. Ghosh, S. Maiti, S. K. Maity and S. Roy, Synth. Commun., 2008, 38, 1958 CrossRef CAS.
-
(a) J. Barluenga, A. L. Viado, E. Aguilar, S. Fustero and B. Olano, J. Org. Chem., 1993, 58, 5972 CrossRef CAS;
(b) D. Enders, M. Moser, G. Geibel and M. C. Laufer, Synthesis, 2004, 2040 CrossRef CAS.
-
F. Farnworth, S. L. Jones and I. McAlpine, Speciality Inorganic Chemicals; Royal Society of Chemistry: London, 1980, p 40 Search PubMed.
-
(a) U. Dähn, H. Hagenmaier, H. Höhne, W. A. König, G. Wolf and H. Zähner, Arch. Microbiol., 1976, 107, 249 CrossRef;
(b) K. Kobinata, M. Uramoto, M. Nishii, H. Kusakabe, G. Nakamura and K. Isono, Agric. Biol. Chem., 1980, 44, 1709 CrossRef CAS.
-
J. P. Riley and R. Chester, Introduction to Marine Chemistry; Academic: NY, 1971 Search PubMed.
-
(a) U. Bora, Synlett, 2003, 1073 CrossRef CAS;
(b) H. W. Heine, D. L. Cottle and H. L. van Mater, J. Am. Chem. Soc., 1946, 68, 524 CrossRef CAS;
(c) T. Poll, G. Helmchen and B. Bauer, Tetrahedron Lett., 1984, 25, 2191 CrossRef CAS;
(d) D. A. Evans, K. T. Chapman and J. Bisaha, J. Am. Chem. Soc., 1988, 110, 1238 CrossRef CAS;
(e) M. Frank-Neumann, M. Miesch and L. Gross, Tetrahedron Lett., 1990, 31, 5027 CrossRef;
(f) S. E. Denmark, E. J. Weber, T. M. Wilson and T. M. Willson, Tetrahedron, 1989, 45, 1053 CrossRef CAS;
(g) S. Itsuno, Y. Sakurai and K. Ito, Synthesis, 1988, 995 CrossRef CAS;
(h) S. Itsuno, Y. Sakurai, K. Shimizu and K. M. Ito, J. Chem. Soc., Perkin Trans. 1, 1990, 1859 RSC;
(i) K. P. Chary, R. M. Thomas and D. S. Iyengar, Indian J. Chem., 2000, 39B, 57 CAS;
(j) K. P. Chary, S. R. Ram and D. S. Iyengar, Synlett, 2000, 683 CAS;
(k) D. C. Harrowven and R. F. Dainty, Tetrahedron Lett., 1996, 37, 7659 CrossRef CAS;
(l) H. Firouzabadi, N. Iranpoor and B. Karimi, Synlett, 1999, 319 CrossRef CAS;
(m) B. Karimi and H. Seradj, Synlett, 2000, 805 CAS;
(n) S. Smitha and C. S. Reddy, Synthesis, 2004, 834 Search PubMed.
- R. Ghosh, S. Maiti, A. Chakraborty, S. Chakraborty and A. K. Mukherjee, Tetrahedron, 2006, 62, 4059 CrossRef CAS.
- R. I. Kureshy, S. Agrawal, S. Saravanan, N. H. Khan, A. K. Shah, S. H. R. Abdi, H. C. Bajaj and E. Suresh, Tetrahedron Lett., 2010, 51, 489 CrossRef CAS.
- J. Wu, W. Sun, X. Sun and H. -G. Xia, Green Chem., 2006, 8, 365 RSC.
-
(a) P. T. Kaye and X. W. Nocanda, Synthesis, 2001, 2389 CrossRef CAS;
(b) A. R. Katritzky and M. A. C. Button, J. Org. Chem., 2001, 66, 5595 CrossRef CAS;
(c) P. Kumar and M. S. Bodas, Tetrahedron, 2001, 57, 9755 CrossRef CAS;
(d) V. J. Ram, N. Agarwal, A. S. Farhanullah, A. Sharon and P. R. Maulik, J. Chem. Soc., Perkin Trans. 1, 2002, 1, 1426 RSC.
-
(a) T. Al-Nakib, V. Bezjak, S. Rashid, B. Fullam and M. J. Meegan, Eur. J. Med. Chem., 1991, 26, 221 CrossRef;
(b) T. Al-Nakib, V. Bezjak, M. J. Meegan and R. Chandy, Eur. J. Med. Chem., 1990, 25, 455 CrossRef CAS;
(c) L. A. Van Vliet, N. Rodenhuis, D. Dijkstra, H. Wikstroem, T. A. Pugsley, K. A. Serpa, L. T. Meltzer, T. G. Heffner, L. D. Wise, M. E. Lajiness, R. M. Huff, K. Svensson, S. Sundell and M. Lundmark, J. Med. Chem., 2000, 43, 2871 CrossRef CAS.
-
(a) N. H. Eudy, S. R. Safir and J. B. Press, J. Org. Chem., 1980, 45, 497 CrossRef;
(b) S. Pant, A. Sharma, C. K. Sharma, U. C. Pant and A. K. Goel, Indian J. Chem. Sec. B, 1996, 35B, 794 CAS;
(c) G. Desarro, A. Chimirri, A. Desarro, R. Gitto, S. Grasso and M. Zappala, Eur. J. Med. Chem., 1995, 30, 925 CrossRef CAS;
(d) R. U. Braun and T. J. J. Muller, Tetrahedron, 2004, 60, 9463 CrossRef CAS;
(e) A. Levai, J. Heterocycl. Chem., 2004, 41, 399 CrossRef CAS.
- M. Zielinska-Błajet, R. Kowalczyk and J. Skarzewski, Tetrahedron, 2005, 61, 5235 CrossRef.
- A. Kumar and Akanksha, Tetrahedron Lett., 2007, 48, 8730 CrossRef CAS.
-
V. P. Kukhar and H. R. Hudson, Aminophonic and Aminophinic Acids Chemistry and Biological Activity, John Wiley & Sons Ltd, Chichester, 2000 Search PubMed.
- B. C. Ranu, A. Hajra and U. Jana, Org. Lett., 1999, 1, 1141 CrossRef CAS.
- J. S. Yadav, B. V. S. Reddy, K. S. Raj, K. B. Reddy and A. R. Prasad, Synthesis, 2001, 2277 CrossRef CAS.
- S. Lee, J. H. Park, J. Kang and J. K. Lee, Chem. Commun., 2001, 1698 RSC.
- S. Chandrasekhar, S. J. Prakash, V. Jagadeshwar and C. Narsihmulu, Tetrahedron Lett., 2001, 42, 5561 CrossRef CAS.
- F. Xu, Y. Q. Luo, M. Y. Deng and Q. Shen, Eur. J. Org. Chem., 2003, 4728 CrossRef CAS.
- J. Wu, W. Sun, G. H. Xia and Y. X. Sun, Org. Biomol. Chem., 2006, 4, 1663 CAS.
-
(a) C. Y. Yuan, S. J. Chen and G. H. Wang, Synthesis, 1991, 490 CrossRef CAS;
(b) Z. Miao, B. Wang, G. Zhang and R. Chen, Bioorg. Chem., 2006, 34, 167 CrossRef CAS.
- B. C. Ranu and A. Hajra, Green Chem., 2002, 4, 551 RSC.
- K. C. Kumaraswamy, S. Kumaraswamy, K. Senthil Kumar and C. Muthiah, Tetrahedron Lett., 2005, 46, 3347 CrossRef.
-
(a) P. Thirumurugan, A. Nandakumar, N. Sudha Priya, D. Muralidaran and P. T. Perumal, Tetrahedron Lett., 2010, 51, 5708 CrossRef CAS;
(b) B. V. Subba Reddy, A. Siva Krishna, A. V. Ganesh and G. G. K. S. Narayana Kumar, Tetrahedron Lett., 2011, 52, 1359 CrossRef.
- J. Zhang, Z. Cui, F. Wang, Y. Wang, Z. Miao and R. Chen, Green Chem., 2007, 9, 1341 RSC.
-
(a) D. Seebach and J. L. Matthews, Chem. Commun., 1997, 2015 RSC;
(b) Y. F. Wang, T. Izawa, S. Kobayashi and M. Ohno, J. Am. Chem. Soc., 1982, 104, 6465 CrossRef CAS;
(c) S. Knapp, Chem. Rev., 1995, 95, 1859 CrossRef CAS;
(d)
E. Juaristi, In Enantioselective Synthesis of β-Aminoacids; John Wiley & Sons: New York, 1997 Search PubMed.
-
(a)
T. Dingermann, D. Steinhilber and G. Folkers, In Molecular Biology in Medicinal Chemistry, Wiley-VCH, 2004 Search PubMed;
(b) A. Y. Shen, C. T. Tsai and C. L. Chen, Eur. J. Med. Chem., 1999, 34, 877 CrossRef CAS;
(c) A. Y. Shen, C. L. Chen and C. I. Lin, Chin. J. Physiol., 1992, 35, 45 CAS.
-
(a) R. Hulst, H. Heres, N. C. M. W. Peper and R. M. Kellogg, Tetrahedron: Asymmetry, 1996, 5, 1373 CrossRef;
(b) X. Li, C. -H. Yeung, A. S. C. Chan and T. -K. Yang, Tetrahedron: Asymmetry, 1999, 10, 759 CrossRef CAS.
- G. C. Nandi, S. Samai, R. Kumar and M. S. Singh, Tetrahedron Lett., 2009, 50, 7220 CrossRef CAS.
-
(a) K. A. da Silva Rocha, I. V. Kozhevnikov and E. V. Gusevskaya, Appl. Catal., A, 2005, 294, 106 CrossRef CAS;
(b) Z. Li, X. Ma, J. Liu, X. Feng, G. Tian and A. Zhu, J. Mol. Catal. A: Chem., 2007, 272, 132 CrossRef CAS;
(c) M. Vicevic, K. V. K. Boodhoo and K. Scott, Chem. Eng. J., 2007, 133, 31 CrossRef CAS;
(d) K. T. Li, C. L. Dai and C. W. Kuo, Catal. Commun., 2007, 8, 1209 CrossRef CAS;
(e) J. S. Girardon, E. Quinet, A. G. Constant, P. A. Chernavskii, L. Gengembre and A. Y. Khodakov, J. Catal., 2007, 248, 143 CrossRef CAS;
(f) I. M. Vilella, I. Borbáth, J. L. Margitfalvi, K. Lázár, S. R. de Miguel and O. A. Scelza, Appl. Catal., A, 2007, 326, 37 CrossRef CAS;
(g) R. Zavoianu, C. R. Dias, A. P. V. Soares and M. F. Portela, Appl. Catal., A, 2006, 298, 40 CrossRef CAS.
-
R. A. Van Santen and M. Neurock, Molecular Heterogeneous Catalysis: A Conceptual and Computational Approach, Wiley-VCH: Weinheim, Cambridge, 2006 Search PubMed.
- H. R. Shaterian, A. Hosseinian and M. Ghashang, Tetrahedron Lett., 2008, 49, 5804 CrossRef CAS.
- G. H. Mahdavinia and M. A. Bigdeli, Chin. Chem. Lett., 2009, 20, 383 CrossRef CAS.
- H. R. Shaterian, A. Hosseinian and M. Ghashang, Synth. Commun., 2008, 38, 3375 CrossRef CAS.
- H. R. Shaterian, H. Yarahmadi and M. Ghashang, Tetrahedron, 2008, 64, 1263 CrossRef CAS.
- H. R. Shaterian, F. Shahrekipoor and M. Ghashang, J. Mol. Catal. A: Chem., 2007, 272, 142 CrossRef CAS.
- A. K. Chakraborti and R. Gulhane, Chem. Commun., 2003, 1896 RSC.
- B. Das, K. Laxminarayana and B. Ravikanth, J. Mol. Catal. A: Chem., 2007, 271, 131 CrossRef CAS.
- S. Kantevari, R. Bantu and L. Nagarapu, J. Mol. Catal. A: Chem., 2007, 269, 53 CrossRef CAS.
- M. A. Bigdeli, M. M. Heravi and G. H. Mahdavinia, J. Mol. Catal. A: Chem., 2007, 275, 25 CrossRef CAS.
- B. Das, K. Damodar, N. Chowdhury and R. A. Kumar, J. Mol. Catal. A: Chem., 2007, 274, 148 CrossRef CAS.
- V. T. Kamble, V. S. Jamode, N. S. Joshi, A. V. Biradara and R. Y. Deshmukh, Tetrahedron Lett., 2006, 47, 5573 CrossRef CAS.
- B. Das, K. Venkateswarlu, A. Majhi, M. R. Reddy, K. N. Reddy, Y. K. Rao, K. Ravikumar and B. Sridhar, J. Mol. Catal. A: Chem., 2006, 246, 276 CrossRef CAS.
-
(a) B. Das, K. Venkateswarlu, K. Suneel and A. Majhi, Tetrahedron Lett., 2007, 48, 5371 CrossRef CAS;
(b) G. L. Khatik, G. Sharma, R. Kumar and A. K. Chakraborti, Tetrahedron, 2007, 63, 1200 CrossRef CAS.
- G. H. Mahdavinia, M. A. Bigdeli and M. M. Heravi, Chin. Chem. Lett., 2008, 19, 1171 CrossRef CAS.
- H. R. Shaterian and H. Yarahmadi, Arkivoc, 2008, ii, 105 Search PubMed.
- A. Dorehgiraee, H. Khabazzadeh and K. Saidi, Arkivoc, 2009, vii, 303 Search PubMed.
- H. R. Shaterian, A. Amirzadeh, F. Khorami and M. Ghashang., Synth. Commun., 2008, 38, 2983 CrossRef CAS.
- S. B. Patil, P. R. Singh, M. P. Surpur and S. D. Samant, Synth. Commun., 2007, 37, 1659 CrossRef CAS.
- A. Shockravi, M. Sadeghpour and A. Olyaei, Synth. Commun., 2009, 39, 2347 CrossRef CAS.
- N. Foroughifar, A. Mobinikhaledi and H. Moghanian, Synth. Commun., 2009, 39, 3668 CrossRef CAS.
- D. Koszelewski, W. Szymanski, J. Krysiak and R. Ostaszewski, Synth. Commun., 2008, 38, 1120 CrossRef CAS.
- L. El Kaïm, L. Gautier, L. Grimaud, L. M. Harwood and V. Michaut, Green Chem., 2003, 5, 477 RSC.
-
(a) T. R. Van den Ancker, G. W. V. Cave and C. L. Raston, Green Chem., 2006, 8, 50 RSC;
(b) H. Naeimi, F. Salimi and K. Rabiei, J. Mol. Catal. A: Chem., 2006, 260, 100 CrossRef CAS;
(c) D. M. Boghaei and S. Mohebi, Tetrahedron, 2002, 58, 5357 CrossRef CAS;
(d) A. A. Jarrahpour and D. Khalili, Molecules, 2006, 11, 59 CrossRef CAS;
(e) T. Takeuchi, A. Boettcher, C. M. Quezada, M. I. Simon, T. J. Meade and H. B. Gray, J. Am. Chem. Soc., 1998, 120, 8555 CrossRef CAS;
(f) A. Bacchi, M. Carcelli, P. Pelagatti, G. Pelizzi, M. C. Rodriguez-Arguelles, D. Rogolino, C. Solinas and F. Zani, J. Inorg. Biochem., 2005, 99, 397 CrossRef CAS.
- A. Shockravi, M. Sadeghpour and A. Olyaei, Synth. Commun., 2010, 40, 2531 CrossRef CAS.
- P. Srihari, V. K. Singh, D. C. Bhunia and J. S. Yadav, Tetrahedron Lett., 2009, 50, 3763 CrossRef CAS.
- M. L. Deb and P. J. Bhuyan, Tetrahedron Lett., 2007, 48, 2159 CrossRef CAS.
- H. Firouzabadi, N. Iranpoor, M. Jafarpour and A. Ghaderi, J. Mol. Catal. A: Chem., 2006, 253, 249 CrossRef CAS.
- A. Olyaei, B. Shams, M. Sadeghpour, F. Gesmati and Z. Razaziane, Tetrahedron Lett., 2010, 51, 6086 CrossRef CAS.
- L. K. Pandey, U. Pathak, A. Mazumder and S. Mathur, Indian J. Chem., 2010, 49B, 1225 CAS.
- R. Kumar, A. K. Gupta and M. P. Kaushik, Phosphorus, Sulfur Silicon Relat. Elem., 2010, 185, 2064 CrossRef CAS.
- O. Mukherjee Singh and N. Sushuma Devi, Synth. Commun., 2011, 41, 516 CrossRef.
-
(a)
G. Bringmann, C. Gunther, O. Schupp and S. Tesler, In Progress in the Chemistry of Organic Natural Products, W. Herz, H. Falk, G. W. Kirby and R. E. Moore, ed.; Springer-Verlag: New York, 2001, vol. 81, 1 Search PubMed;
(b) J. -M. Becht, A. Gissot, A. Wagner and C. Miosjkowki, Chem.–Eur. J., 2003, 9, 3209 CrossRef CAS.
-
(a) F. Fringuelli, R. Girotti, O. Piermatti, F. Pizzo and L. Vaccaro, Org. Lett., 2006, 25, 5741 CrossRef;
(b) Y. Zhao, J. Li, C. Li, K. Yin, D. Ye and X. Jia, Green Chem., 2010, 12, 1370 RSC.
- D. Strubing, H. Neumann, A. J. V. Wangelin, S. Klaus, S. Hubner and M. Beller, Tetrahedron, 2006, 62, 10962 CrossRef.
- L. Rong, H. Han, H. Jiang and S. Tu, Synth. Commun., 2009, 39, 3493 CrossRef CAS.
- S. W. Kshirsagar, N. R. Patil and S. D. Samant, Tetrahedron Lett., 2010, 51, 2924 CrossRef CAS.
-
(a) A. T. Balaban, D. C. Oniciu and A. R. Katritzky, Chem. Rev., 2004, 104, 2777 CrossRef CAS;
(b) M. A. P. Martins, W. Cunico, C. M. P. Pereira, A. C. F. Flores, A. P. Sinhorin, H. G. Bonacorso and N. Zanatta, Curr. Org. Synth., 2004, 1, 391 CrossRef CAS;
(c) S. V. Druzhinin, E. S. Balenkova and V. G. Nenajdenko, Tetrahedron, 2007, 63, 7753 CrossRef CAS.
-
(a) A. K. Ghose, V. N. Viswanadhan and J. J. Wendoloski, J. Comb. Chem., 1999, 1, 55 CrossRef CAS;
(b) J. Xu and J. Stevenson, J. Chem. Inf. Model., 2000, 40, 1177 CrossRef CAS.
- M. A. P. Martins, C. P. Frizzo, D. N. Moreira, L. Buriol and P. Machado, Chem. Rev., 2009, 109, 4140 CrossRef CAS.
-
(a) V. Estevez, M. Villacampa and J. C. Menendez, Chem. Soc. Rev., 2010, 39, 4402 RSC;
(b) A. Alizadeh, M. Babaki and N. Zohreh, Tetrahedron, 2009, 65, 1704 CrossRef CAS.
-
(a) B. C. Ranu and A. Hajra, Tetrahedron, 2001, 57, 4767 CrossRef CAS;
(b) M. Kidwai, K. Singhal and S. Kukreja, Heteroat. Chem., 2007, 18, 617 CrossRef CAS;
(c) B. C. Ranu, A. Hajra and U. Jana, Synlett, 2000, 75 CAS;
(d) M. Lin, L. Hao, R. -D. Ma and Z. -P. Zhan, Synlett, 2010, 2345 CrossRef CAS.
- O. A. Attanasi, G. Favi, F. Mantellini, G. Moscatelli and S. Santeusanio, J. Org. Chem., 2011, 76, 2860 CrossRef CAS.
- M. Borthakur, S. Gogoi, J. Gogoi and R. C. Boruah, Tetrahedron Lett., 2010, 51, 5160 CrossRef CAS.
- G. W. Kabalka, L. Wang and R. M. Pagni, Tetrahedron Lett., 2001, 42, 6049 CrossRef CAS.
- S. V. Karthikeyan, S. Perumal and K. K. Balasubramanian, Tetrahedron Lett., 2007, 48, 6133 CrossRef CAS.
- M. Jida, S. Malaquin, R. Deprez-Poulain, G. Laconde and B. Deprez, Tetrahedron Lett., 2010, 51, 5109 CrossRef CAS.
-
(a)
Heterocyclic Chemistry, J. A. Joule and K. Mills, ed.; Blackwell Science: Oxford, 2000 Search PubMed;
(b)
A. Padwa and W. H. Pearson, ed.;Synthetic Applications of 1,3-Dipolar Cycloaddition Chemistry Toward Heterocycles and Natural Products; John Wiley & Sons: New York, 2002 Search PubMed;
(c)
B. Stanovnik and J. Svete, Science of Synthesis, Houben-Weyl Methods of Molecular Transformations, vol. 12, Thieme, Stuttgart, 2002, Chap. 12.1p 15 Search PubMed.
-
(a) S. K. Singh, M. S. Reddy, S. Shivaramakrishna, D. Kavitha, R. Vasudev, J. M. Babu, A. Sivalakshmidevi and Y. K. Rao, Tetrahedron Lett., 2004, 45, 7679 CrossRef CAS;
(b) F. A. Rosa, P. Machado, P. S. Vargas, H. G. Bonacorso, N. Zanatta and M. A. P. Martins, Synlett, 2008, 1673 CAS;
(c) B. Stanovnik and J. Svete, Chem. Rev., 2004, 104, 2433 CrossRef CAS;
(d) F. Xie, G. Cheng and Y. Hu, J. Comb. Chem., 2006, 8, 286 CrossRef CAS;
(e) X. Deng and N. S. Mani, Org. Lett., 2008, 10, 1307 CrossRef CAS;
(f) X. Deng and N. S. Mani, J. Org. Chem., 2008, 73, 2412 CrossRef CAS;
(g)
L. Yet, In Comprehensive Heterocyclic Chemistry III, vol. 4 (ed.; A. R. Katritzky, C. A. Ramsden, E. F. V. Scriven and R. J. K.Taylor), Elsevier, Oxford, 2008, p 1 Search PubMed;
(h) B. S. Gerstenberger, M. R. Rauckhorst and J. T. Starr, Org. Lett., 2009, 11, 2097 CrossRef CAS;
(i) R. Muruganantham and I. Namboothiri, J. Org. Chem., 2010, 75, 2197 CrossRef CAS;
(j) J. J. Neumann, M. Suri and F. Glorius, Angew. Chem., Int. Ed., 2010, 49, 7790 CrossRef CAS;
(k) S. Dadiboyena, E. J. Valente and A. T. Hamme II, Tetrahedron Lett., 2009, 50, 291 CrossRef CAS.
-
(a)
J. Elguero, In Comprehensive Heterocyclic Chemistry II; A. R. Katritzky, C. W. Rees and E. F. V. Scriven, ed.; Pergamon: Oxford, 1996, 3, p 1 Search PubMed;
(b) C. Lamberth, Heterocycles, 2007, 71, 1467 CrossRef CAS;
(c) P. Singh, K. Paul and W. Holzer, Bioorg. Med. Chem., 2006, 14, 5061 CrossRef CAS and references cited therein..
-
(a) S. Trofimenko, Prog. Inorg. Chem., 1986, 34, 115 CrossRef CAS;
(b) A. Danel, Z. He, G. H. W. Milburn and P. Tomasik, J. Mater. Chem., 1999, 9, 339 RSC;
(c) H. H. Otto, Arch. Pharm., 1974, 307, 444 CrossRef CAS.
-
(a) H. H. Otto and H. Schmelz, Arch. Pharm., 1979, 312, 478 CrossRef CAS;
(b) G. V. Klokol, S. G. Krivokolysko, V. D. Dyachenko and V. P. Litvinov, Chem. Heterocycl. Compd., 1999, 35, 1183 CrossRef CAS.
-
(a) A. M. Shestopalov, Y. M. Emeliyanova, A. A. Shestopalov, L. A. Rodinovskaya, Z. I. Niazimbetova and D. H. Evans, Tetrahedron, 2003, 59, 7491 CrossRef CAS;
(b) A. M. Shestopalov, Y. M. Emeliyanova, A. A. Shestopalov, L. A. Rodinovskaya, Z. I. Niazimbetova and D. H. Evans, Org. Lett., 2002, 4, 423 CrossRef CAS.
-
(a) D. Shi, J. Mou, Q. Zhuang, L. Niu, N. Wu and X. Wang, Synth. Commun., 2004, 34, 4557 CrossRef CAS;
(b) T. -S. Jin, A. -Q. Wang, Z. -L. Cheng, J. -S. Zhang and T. -S. Li, Synth. Commun., 2005, 35, 137 CrossRef CAS;
(c) Y. Peng, G. Song and R. Dou, Green Chem., 2006, 8, 573 RSC.
- G. Vasuki and K. Kumaravel, Tetrahedron Lett., 2008, 49, 5636 CrossRef CAS.
- F. Lehmann, M. Holm and S. Laufer, J. Comb. Chem., 2008, 10, 364 CrossRef CAS.
-
(a) J. -F. Zhou, S. -J. Tu, H. -Q. Zhu and S. -J. Zhi, Synth. Commun., 2002, 32, 3363 CrossRef CAS;
(b) R. M. Shaker, A. F. Mahmoud and F. F. Abdel-Latif, J. Chin. Chem. Soc., 2005, 52, 563 CAS.
- S. -B. Guo, S. -X. Wang and J. -T. Li, Synth. Commun., 2007, 37, 2111 CrossRef CAS.
- Z. Ren, W. Cao, W. Tong and Z. Jin, Synth. Commun., 2005, 35, 2509 CrossRef CAS.
- A. S. Nagarajan and B. S. R. Reddy, Synlett, 2009, 2002 CAS.
- K. Kanagaraj and K. Pitchumani, Tetrahedron Lett., 2010, 51, 3312 CrossRef CAS.
-
(a) F. Bellina and R. Rossi, Adv. Synth. Catal., 2010, 352, 1223 CrossRef CAS;
(b) S. Kamijo and Y. Yamamoto, Chem.–Asian J., 2007, 2, 568 CrossRef CAS;
(c) F. Bellina, S. Cauteruccio and R. Rossi, Tetrahedron, 2007, 63, 4571 CrossRef CAS.
- A. Y. Usyatinsky and Y. L. Khmelnitsky, Tetrahedron Lett., 2000, 41, 5031 CrossRef CAS.
- S. Kantevari, S. V. N. Vuppalapati, D. O. Biradar and L. Nagarapu, J. Mol. Catal. A: Chem., 2007, 266, 109 CrossRef CAS.
- M. Adib, S. Ansari, S. Feizi, J. A. Damavandi and P. Mirzaei, Synlett, 2009, 3263 CrossRef CAS.
- J. Safari, S. D. Khalili and S. H. Banitaba, Synth. Commun., 2011, 41, 2359 CrossRef CAS.
- B. S. Dawane, S. G. Konda, V. T. Kamble, S. A. Chavan, R. B. Bhosale and S. M. Baseer, E -J. Chem., 2009, 6(S1), S358 CAS.
- M. Anderluh, M. Jukic and R. Petric, Tetrahedron, 2009, 65, 344 CrossRef CAS.
- B. S. Dawane and S. G. Konda, Int. J. Pharma. Sci. Rev. Res., 2010, 3, 96 CAS.
- M. Xia and Y. -D. Lu, Synth. Commun., 2006, 36, 1637 CrossRef CAS.
- J. A. Seijas, M. P. Vazquez Tato and J. Crecente-Campo, Tetrahedron, 2008, 64, 9280 CrossRef CAS.
- S. Kasmi-Mir, A. Djafri, L. Paquin, J. Hamelin and M. Rahmouni, Molecules, 2006, 11, 597 CrossRef CAS.
- I. Yavari, N. Hosseini and L. Moradi, Monatsh. Chem., 2008, 139, 133 CrossRef CAS.
-
(a) F. Al-Assar, K. N. Zelenin, E. E. Lesiovskaya, I. P. Bezhan and B. A. Chakchir, Pharm. Chem. J., 2002, 36, 598 CrossRef CAS;
(b) R. P. Jain and J. C. Vederas, Bioorg. Med. Chem. Lett., 2004, 14, 3655 CrossRef CAS;
(c) L. J. Street, F. Sternfeld, R. A. Jelley, A. J. Reeve, R. W. Carling, K. W. Moore, R. M. McKernan, B. Sohal, S. Cook, A. Pike, G. R. Dawson, F. A. Bromidge, K. A. Wafford, G. R. Seabrook, S. A. Thompson, G. Marshall, G. V. Pillai, J. L. Castro, J. R. Atack and A. M. MacLeod, J. Med. Chem., 2004, 47, 1807 CrossRef.
- S. Grasso, G. De Sarro, A. De Sarro, N. Micale, M. Zappala, G. Puja, M. Baraldi and C. De Micheli, J. Med. Chem., 2000, 43, 2851 CrossRef CAS.
- Y. Nomoto, H. Obase, H. Takai, M. Teranishi, J. Nakamura and K. Kubo, Chem. Pharm. Bull., 1990, 38, 2179 CrossRef CAS.
- N. Watanabe, Y. Kabasawa, Y. Takase, M. Matsukura, K. Miyazaki, H. Ishihara, K. Kodama and H. Adachi, J. Med. Chem., 1998, 41, 3367 CrossRef CAS.
-
(a) T. Sheradsky and R. Moshenberg, J. Org. Chem., 1986, 51, 3123 CrossRef CAS;
(b) H. W. Heine, L. M. Baclawski, S. M. Bonser and G. D. Wachob, J. Org. Chem., 1976, 41, 3229 CrossRef CAS;
(c) Y. K. Ramtohul, M. N. G. James and J. C. Vederas, J. Org. Chem., 2002, 67, 3169 CrossRef CAS;
(d) L. P. Liu, J. M. Lu and M. Shi, Org. Lett., 2007, 9, 1303 CrossRef CAS;
(e) A. CsXmpai, K. Kormendy and F. Ruff, Tetrahedron, 1991, 47, 4457 CrossRef.
- M. Sayyafi, M. Seyyedhamzeh, H. R. Khavasi and A. Bazgir, Tetrahedron, 2008, 64, 2375 CrossRef CAS.
- H. R. Shaterian, A. Hosseinian and M. Ghashang, Arkivoc, 2009, ii, 59 Search PubMed.
-
(a) R. Ghorbani-Vaghei, R. Karimi-Nami, Z. Toghraei-Semiromi, M. Amiri and M. Ghavidel, Tetrahedron, 2011, 67, 1930 CrossRef CAS;
(b) G. Shukla, R. K. Verma, G. K. Verma and M. S. Singh, Tetrahedron Lett., 2011, 52, 7195 CrossRef CAS.
- D. Kundu, A. Majee and A. Hajra, Tetrahedron Lett., 2009, 50, 2668 CrossRef CAS.
- P. Srihari, P. Dutta, R. S. Rao, J. S. Yadav, S. Chandrasekhar, P. Thombare, J. Mohapatra, A. Chatterjee and M. R. Jain, Bioorg. Med. Chem. Lett., 2009, 19, 5569 CrossRef CAS.
- M. Adib, E. Sheibani, L. -G. Zhu and P. Mirzaei, Tetrahedron Lett., 2008, 49, 5108 CrossRef CAS.
- M. Diederich and U. Nubbemeyer, Synthesis, 1999, 286 CrossRef CAS.
- P. Chalard, R. Remuson, Y. Gelas-Mialhe, J. C. Gramain and I. Canet, Tetrahedron Lett., 1999, 40, 1661 CrossRef CAS.
-
(a) M. Pourashraf, P. Delair, M. O. Rasmussen and A. E. Greene, J. Org. Chem., 2000, 65, 6966 CrossRef CAS;
(b) J. Cossy, C. Willis, V. Bellosta and L. S. Jalmes, Synthesis, 2002, 951 CrossRef CAS.
- S. H. Park, H. J. Kang, S. Ko, S. Park and S. Chang, Tetrahedron: Asymmetry, 2001, 12, 2621 CrossRef CAS.
- U. Bora, A. Saikia and R. C. Boruah, Org. Lett., 2003, 5, 435 CrossRef CAS.
- B. Yan and Y. Liu, Org. Lett., 2007, 9, 4323 CrossRef CAS.
- A. Defant, G. Guella and I. Mancini, Synth. Commun., 2008, 38, 3003 CrossRef CAS.
- S. Yan, Y. Chen, L. Liu, N. He and J. Lin, Green Chem., 2010, 12, 2043 RSC.
- M. Adib, E. Sheibani, H. R. Bijanzadeh and L. -G. Zhu, Tetrahedron, 2008, 64, 10681 CrossRef CAS.
- A. Bazgir, M. Seyyedhamzeh, Z. Yasaei and P. Mirzaei, Tetrahedron Lett., 2007, 48, 8790 CrossRef CAS.
-
(a) V. V. Rostovtsev, L. G. Green, V. V. Fokin and K. B. Sharpless, Angew. Chem., Int. Ed., 2002, 41, 2596 CrossRef CAS;
(b) H. C. Kolb, M. G. Finn and K. B. Sharpless, Angew. Chem., Int. Ed., 2001, 40, 2004 CrossRef CAS.
-
E. S. H. El Ashry and N. Rashed, Advances in Heterocyclic Chemistry, A. R.Katrirzky, ed. 1998, 71, 57 Search PubMed.
- M. J. Genin, D. A. Allwine, D. J. Anderson, M. R. Barbachyn, D. E. Emmert, S. A. Garmon, D. R. Graber, K. C. Grega, J. B. Hester, D. K. Hutchinson, J. Morris, R. J. Reischer, C. W. Ford, G. E. Zurenko, J. C. Hamel, R. D. Schaadt, D. Stapert and B. H. Yagi, J. Med. Chem., 2000, 43, 953 CrossRef CAS.
- R. Alvarez, S. Velazquez, A. San-Felix, S. Aquaro, E. De Clercq, C. -F. Perno, A. Karlsson, J. Balzarini and M. J. Camarasa, J. Med. Chem., 1994, 37, 4185 CrossRef CAS.
- D. R. Buckle, C. J. M. Rockell, H. Smith and B. A. Spicer, J. Med. Chem., 1986, 29, 2269 CrossRef.
-
M. Tanaka, T. Yamazaki, M. Kajitani, Penam derivatives. Eur. Pat. 158494, 1985; Chem. Abstr. 1986, 104, 186239d Search PubMed.
-
(a) R. K. Smalley and M. Teguiche, Synthesis, 1990, 654 CrossRef CAS;
(b) L. Bertelli, G. Biagi, I. Giorgi, O. Livi, C. Manera, V. Scartoni, C. Martini, G. Giannaccini, L. Trincavelli and P. L. Barili, Farmaco, 1998, 53, 305 CrossRef CAS.
-
(a) G. Fischer, Adv. Heterocycl. Chem., 1993, 57, 81 CrossRef CAS;
(b) E. S. H. Elashry and N. Rashed, Adv. Heterocycl. Chem., 1999, 73, 127 Search PubMed.
- C. M. Richardson, D. S. Williamson, M. J. Parratt, J. Borgognoni, A. D. Cansfield, P. Dokurno, G. L. Francis, R. Howes, J. D. Moore, J. B. Murray, A. Robertson, A. E. Surgenor and C. J. Torrance, Bioorg. Med. Chem. Lett., 2006, 16, 1353 CrossRef CAS.
- E. -S. A. M. Badawey, J. Heterocycl. Chem., 1996, 33, 229 CrossRef CAS.
- G. E. Davies, J. Pharm. Pharmacol., 1973, 25, 681 CrossRef CAS.
- B. Brdar, M. Japelj and J. Kobe, Biochem. Pharmacol., 1979, 28, 1683 CrossRef CAS.
- H. M. Eisa, M. B. el-Ashmawy, M. M. Tayel, S. A. el-Magd and H. A. el-Kashef, Bull. Chim.
Farm., 1996, 135, 585 CAS.
- O. Prakash, V. Bhardwaj, R. Kumar, P. Tyagi and K. R. Aneja, Eur. J. Med. Chem., 2004, 39, 1073 CrossRef CAS.
- E. B. Moawad, M. Y. Yousif and M. A. Metwally, Pharmazie, 1989, 44, 820 CAS.
-
(a)
J. A. Joule and K. Mills, Heterocyclic Chemistry, 5th Ed., John Wiley & Sons Ltd., 2010 Search PubMed;
(b)
G. W. Gribble and J. A. Joule,Progress in Heterocyclic Chemistry, Elsevier, Oxford OX2 8DP, UK, 2009, vol. 21, Chap. 5.4p 224 Search PubMed.
-
(a) D. A. Horton, G. T. Bourne and M. L. Smythe, Chem. Rev., 2003, 103, 893 CrossRef CAS;
(b) R. W. DeSimone, K. S. Currie, S. A. Mitchell, J. W. Darrow and D. A. Pippin, Comb. Chem. High T. Scr., 2004, 7, 473 CAS;
(c) L. Costantino and D. Barlocco, Curr. Med. Chem., 2006, 13, 65 CrossRef CAS.
-
(a) C. D. Duarte, E. J. Barreiro and C. A. M. Fraga, Mini-Rev. Med. Chem., 2007, 7, 1108 CrossRef CAS;
(b) M. E. Welsch, S. A. Snyder and B. R. Stockwell, Curr. Opin. Chem. Biol., 2010, 14, 347 CrossRef CAS.
- A. Dandia, R. Singh and K. Arya, Lett. Org. Chem., 2009, 6, 100 CrossRef CAS.
- R. Ghahremanzadeh, S. Ahadi, G. I. Shakibaei and A. Bazgir, Tetrahedron Lett., 2010, 51, 499 CrossRef CAS.
- J. Jayashankaran, R. D. R. S. Manian and R. Raghunathan, Tetrahedron Lett., 2004, 45, 7303 CrossRef CAS.
- E. Ramesh, M. Karthiresan and R. Raghunathan, Tetrahedron Lett., 2007, 48, 1835 CrossRef CAS.
- A. R. Suresh Babu and R. Raghunathan, Tetrahedron, 2007, 63, 8010 CrossRef CAS.
- A. Shaabani and A. Bazgir, Tetrahedron Lett., 2004, 45, 2575 CrossRef CAS.
- A. Mobinikhaledi, N. Foroughifar and M. A. Bodaghi Fard, Synth. Commun., 2011, 41, 441 CrossRef CAS.
- D. R. Williams, P. D. Lowder and Y. -G. Gu, Tetrahedron Lett., 1997, 38, 327 CrossRef CAS.
- K. M. McEvoy, A. J. Windebank, J. R. Daube and P. A. Low, N. Engl. J. Med., 1989, 321, 1567 CrossRef CAS.
- S. R. Schwid, M. D. Petrie, M. P. McDermott, D. S. Tierney, D. H. Mason and A. D. Goodman, Neurology, 1997, 48, 817 CAS.
- J. L. Segal and S. R. Brunnemann, Pharmacotherapy, 1997, 17, 415 CAS.
- L. C. Sellin, Med. Biol., 1981, 59, 11 CAS.
-
(a) A. Sakurai and H. Midorikawa, Bull. Chem. Soc. Jpn., 1968, 41, 430 CrossRef CAS;
(b) N. Latif and N. S. Girgis, Indian J. Chem., 1981, 20B, 147 CAS.
-
(a) H. Vorbruggen, Adv. Heterocycl. Chem., 1990, 49, 117 CrossRef;
(b) K. Matsumoto, H. Minatogawa, M. Munakata, M. Toda and H. Tsukube, Tetrahedron Lett., 1990, 27, 3923 Search PubMed.
-
(a)
A. McKillop and A. J. Boulton, Synthesis of Six-Membered Rings, In “Comprehensive Heterocyclic Chemistry”, A. R. Katritzky and C. W. Rees, ed.; Pergamon Press: Oxford, U.K., 1984, vol. 2, p 67 Search PubMed;
(b)
P. A. Keller, Pyridines and their Benzo Derivatives, In “Comprehensive Heterocyclic Chemistry III,”A. R. Katritzky, C. A. Ramsden, E. F. V. Scriven and R. J. K. Taylor, ed.; Pergamon: Oxford, U.K., 2008, vol. 7, p 217 Search PubMed.
- S. Kantevari, M. V. Chary and S. V. N. Vuppalapati, Tetrahedron, 2007, 63, 13024 CrossRef CAS.
- M. M. Heravi, M. Zakeri, S. Pooremamy and H. A. Oskooie, Synth. Commun., 2011, 41, 113 CrossRef CAS.
- M. P. S. Ishar, K. Kumar, S. Kaur, S. Kumar, N. K. Girdhar, S. Sachar, A. Marwaha and A. Kapoor, Org. Lett., 2001, 3, 2133 CrossRef CAS.
- V. Sridharan, P. T. Perumal, C. Avendano and J. C. Menendez, Tetrahedron, 2007, 63, 4407 CrossRef CAS.
-
(a) N. S. Devi and S. Perumal, Tetrahedron Lett., 2007, 48, 5627 CrossRef;
(b) P. A. Clarke, A. V. Zaytzev and A. C. Whitwood, Tetrahedron Lett., 2007, 48, 5209 CrossRef CAS.
- A. Kumar and R. A. Maurya, Tetrahedron, 2008, 64, 3477 CrossRef CAS.
- S. Samai, G. C. Nandi, R. Kumar and M. S. Singh, Tetrahedron Lett., 2009, 50, 7096 CrossRef CAS.
- S. -X. Wang, Z. -Y. Li, J. -C. Zhang and J. -T. Li, Ultrason. Sonochem., 2008, 15, 677 CrossRef CAS.
-
(a) M. A. Zolfigol and M. Safaiee, Synlett, 2004, 827 CrossRef CAS;
(b) L. Shen, S. Cao, J. Wu, H. Li, J. Zhang, M. Wu and X. Qian, Tetrahedron Lett., 2010, 51, 4866 CrossRef CAS.
- B. Jiang, X. Wang, F. Shi, S. -J. Tu and G. Li, Org. Biomol. Chem., 2011, 9, 4025 CAS.
- A. Kumar and S. Sharma, Green Chem., 2011, 13, 2017 RSC.
- L. M. Sanchez, A. G. Sathicq, J. L. Jios, G. T. Baronetti, H. J. Thomas and G. P. Romanelli, Tetrahedron Lett., 2011, 52, 4412 CrossRef CAS.
- A. Moshtaghi Zonouz and D. Moghani, Synth. Commun., 2011, 41, 2152 CrossRef.
- P. Wu, X. -M. Cai, Q. -F. Wang and C. -G. Yan, Synth. Commun., 2011, 41, 841 CrossRef CAS.
-
(a) A. Fassihi, D. Abedi, L. Saghaie, R. Sabet, H. Fazeli, G. Bostaki, O. Deilami and H. Sadinpou, Eur. J. Med. Chem., 2009, 44, 2145 CrossRef CAS;
(b) M. Panunzio, M. A. Lentini, E. Campana, G. Martelli, E. Tamanini and P. Vicennati, Synth. Commun., 2004, 34, 345 CrossRef CAS.
-
(a)
R. D. H. Murray, J. Mendez and S. A. Brown, In The Natural Coumarins: Occurrences, Chemistry and Biochemistry; John Wiley and Sons: New York, USA, 1982 Search PubMed;
(b)
J. D. Hepworth, In Comprehensive Heterocyclic Chemistry; A. R. Katritzky and C. W. Rees, ed.; Pergamon: New York, 1984, vol. 3, p 799 Search PubMed;
(c)
J. D. Hepworth, C. D. Gabbutt and B. M. Heron, In Comprehensive Heterocyclic Chemistry-II, A. R. Katritzky, C. W. Rees and E. F. V. Scriven, ed.; Pergamon: New York, 1996, vol. 5, p 417 Search PubMed;
(d)
F. M. Dean, Naturally Occurring Oxygen Ring Compounds, London, Butterworths, 1963 Search PubMed.
-
(a)
Heterocyclic Chemistry, 4th ed.; J. A. Joule and K. Mills, ed.; Blackwell Science Ltd., Oxford, 2006, p 170 Search PubMed;
(b) R. D. H. Murray, Fortschr. Chem. Org. Naturst., 1978, 35, 199 CrossRef CAS;
(c) H. -X. Xu and S. F. Lee, Phytother. Res., 2001, 15, 39 CrossRef CAS;
(d) J. M. Hamilton miller, Antimicrob. Agents Chemother., 1995, 39, 2375 CAS;
(e) K. C. Fylaktakidou, D. J. Hadjipavlou-Litina, K. E. Litinas and D. N. Nicolaides, Curr. Pharm. Des., 2004, 10, 3813 CrossRef CAS;
(f) J. R. Hwu, R. Singh, S. C. Hong, Y. H. Chang, A. R. Das, I. Vliegen, E. De Clercq and J. Neyts, Antiviral Res., 2008, 77, 157 CrossRef CAS;
(g) S. Sardari, Y. Mori, K. Horita, R. G. Micetich, S. Nishibe and M. Daneshtalab, Bioorg. Med. Chem., 1999, 7, 1933 CrossRef CAS;
(h) D. Egan, P. James, D. Cooke and R. O'Kennedy, Cancer Lett., 1997, 118, 201 CrossRef CAS;
(i) P. Valenti, A. Rampa, M. Recanatini, A. Bisi, F. Belluti, P. Da Re, M. Carrara and L. Cima, Anti-cancer Drug Des., 1997, 12, 443 CAS;
(j) C. Spino, M. Dodier and S. Sotheeswaran, Bioorg. Med. Chem. Lett., 1998, 8, 3475 CrossRef CAS.
-
(a)
R. D. Thornes, In Coumarins: Biology, Application, and Mode of Action, R. O'Kennedy and R. D. Thornes, ed.; Wiley: Chichester, UK, 1997, p 348 Search PubMed;
(b) H. Madari, D. Panda, L. Wilson and R. S. Jacobs, Cancer Res., 2003, 63, 1214 CAS;
(c) G. Finn, B. Creaven and D. Egan, Eur. J. Pharmacol., 2003, 481, 159 CrossRef CAS;
(d) G. J. Finn, B. S. Creaven and D. A. Egan, Cancer Lett., 2004, 214, 43 CrossRef CAS;
(e) G. J. Finn, B. S. Creaven and D. A. Egan, Eur. J. Pharm. Sci., 2005, 26, 16 CrossRef CAS;
(f) M. Basanagouda, K. Shivashankar, M. V. Kulkarni, V. P. Rasal, H. Patel, S. S. Mutha and A. A. Mohite, Eur. J. Med. Chem., 2010, 45, 1151 CrossRef CAS.
-
(a) Perfumery: G. S. Clark, Perfum. Flavor., 1995, 20, 23 CAS;
(b) Fluorescent chemo-sensors: C. -T. Chen and W. -P. Huang, J. Am. Chem. Soc., 2002, 124, 6246 CrossRef CAS;
(c) Laser technology: N. Sekar, Colourage, 2003, 50, 55 Search PubMed;
(d) Fluorescent indicators: M. -P. Brun, L. Bischoff and C. Garbay, Angew. Chem., Int. Ed., 2004, 43, 3432 CrossRef CAS;
(e) L. Shastri, S. Kalegowda and M. Kulkarni, Tetrahedron Lett., 2007, 48, 7215 CrossRef CAS;
(f)
M. Maeda, Laser Dyes: Properties of Organic Compounds for Laser Dyes; Academic Press, Tokyo, 1984, p 335 Search PubMed;
(g)
M. Zabradnik, The Production and Application of Fluorescent Brightening Agent, John Wiley & Sons, New York, 1992 Search PubMed;
(h) D. P. Specht, P. A. Martic and S. Farid, Tetrahedron, 1982, 38, 1203 CrossRef CAS.
- K. S. Atwal, G. J. Grover, S. Z. Ahmed, F. N. Ferrara, T. W. Harper, K. S. Kim, P. G. Sleph, S. Dzwonczyk and A. D. Russell, J. Med. Chem., 1993, 36, 3971 CrossRef CAS.
-
(a) N. H. Zawia, D. K. Lahiri and F. Cardozo-Pelaez, Free Radical Biol. Med., 2009, 46, 1241 CrossRef CAS;
(b) S. B. Bodendiek, C. Mahieux, W. Hansel and H. Wulff, Eur. J. Med. Chem., 2009, 44, 1838 CrossRef CAS.
-
(a) F. Meggio, M. A. Pagano, S. Moro, G. Zagotto, M. Ruzzene, S. Sarno, G. Cozza, J. Bain, M. Elliott, A. D. Deana, A. M. Brunati and L. A. Pinna, Biochemistry, 2004, 43, 12931 CrossRef CAS;
(b) D. Yu, M. Suzuki, L. Xie, S. L. Morris-Natschke and K. -H. Lee, Med. Res. Rev., 2003, 23, 322 CrossRef CAS.
-
(a) G. C. Nandi, S. Samai and M. S. Singh, J. Org. Chem., 2010, 75, 7785 CrossRef CAS;
(b) R. K. Verma, G. K. Verma, K. Raghuvanshi and M. S. Singh, Tetrahedron, 2011, 67, 584 CrossRef CAS.
- H. Valizadeh and A. Fakhari, Mol. Diversity, 2011, 15, 233 CrossRef CAS.
-
G. R. Green, J. M. Evans and A. K. Vong, In Comprehensive Heterocyclic Chemistry II, A. R. Katritzky, C. W. Rees and E. F. V. Scriven, ed.; Pergamon Press: Oxford, 1995, vol. 5, p 469 Search PubMed.
-
(a)
W. O. Foye, Prinicipi di Chemico Farmaceutica; Piccin: Padova, Italy, 1991, p. 416 Search PubMed;
(b) L. L. Andreani and E. Lapi, Bull. Chim. Farm., 1960, 99, 583 Search PubMed;
(c) Y. L. Zhang, B. Z. Chen, K. Q. Zheng, M. L. Xu and X. H. Lei, Yao Xue Xue Bao, 1982, 17, 17 CAS ; Chem. Abstr. 1982, 96, 135383e;
(d) L. Bonsignore, G. Loy, D. Secci and A. Calignano, Eur. J. Med. Chem., 1993, 28, 517 CrossRef CAS;
(e) E. C. Witte, P. Neubert and A. Roesch, Ger. Offen., DE, 1986, 427, 985 Search PubMed ; Chem. Abstr. 1986, 104, 224915f.
-
(a) S. Hatakeyama, N. Ochi, H. Numata and S. Takano, J. Chem. Soc., Chem. Commun., 1988, 1202 RSC;
(b) R. Gonzalez, N. Martin, C. Seoane and J. Soto, J. Chem. Soc., Perkin Trans. 1, 1985, 1202 Search PubMed.
- D. Arnetso, W. M. Horspool, N. Martin, A. Ramos and C. Seaone, J. Org. Chem., 1989, 54, 3069 CrossRef.
-
(a) K. Singh, J. Singh and H. Singh, Tetrahedron, 1996, 52, 14273 CrossRef CAS;
(b) X. S. Wang, D. Q. Shi, S. J. Tu and C. S. Yao, Synth. Commun., 2003, 33, 119 CrossRef CAS.
- G. Kaupp, M. R. Naimi-Zamal and J. Schmeyers, Tetrahedron, 2003, 59, 3753 CrossRef CAS.
- I. Devi and P. J. Bhuyan, Tetrahedron Lett., 2004, 45, 8625 CrossRef CAS.
- M. A. Khalilzadeh, Z. Hossaini, M. M. Baradarani and A. Hasannia, Tetrahedron, 2010, 66, 8464 CrossRef CAS.
- M. Radi, V. Bernardo, B. Bechi, D. Castagnolo, M. Pagano and M. Botta, Tetrahedron Lett., 2009, 50, 6572 CrossRef CAS.
- I. Devi, B. S. D. Kumar and P. J. Bhuyan, Tetrahedron Lett., 2003, 44, 8307 CrossRef CAS.
- C. Yao, C. Wang, B. Jiang, X. Feng, C. Yu, T. Li and S. Tu, Bioorg. Med. Chem. Lett., 2010, 20, 2884 CrossRef CAS.
-
G. P. Ellis, In The Chemistry of Heterocyclic Compounds. Chromenes, Chromanes and Chromones, A. Weissberger and E. C. Taylor, ed.; John Wiley: New York, 1977, Chapter 11, p 11 Search PubMed.
-
(a) E. A. Hafez, M. H. Elnagdi, A. G. A. Elagemey and F. M. A. A. El-Taweel, Heterocycles, 1987, 26, 903 CrossRef CAS;
(b) M. A. Sofan, F. M. A. A. El-Taweel and M. H. Elnagdi, Liebigs Ann. Chem., 1989, 935 CrossRef CAS;
(c) F. M. Abdel Galil, B. Y. Riad, S. M. Sherif and M. H. Elnagdi, Chem. Lett., 1982, 1123 CrossRef CAS.
- M. Kidwai, S. Saxena, M. K. R. Khan and S. S. Thukral, Bioorg. Med. Chem. Lett., 2005, 15, 4295 CrossRef CAS.
-
(a) A. G. A. Elagemey and F. M. A. A. El-Taweel, Indian J. Chem., 1990, 29B, 885 Search PubMed;
(b) A. G. A. Elagemey, F. M. A. A. El-Taweel, M. N. M. Khodeir and M. H. Elnagdi, Bull. Chem. Soc. Jpn., 1993, 66, 464 CrossRef;
(c) J. Bloxham, C. P. Dell and C. W. Smith, Heterocycles, 1994, 38, 399 CrossRef CAS.
- R. Maggi, R. Ballini, G. Sartori and R. Sartorio, Tetrahedron Lett., 2004, 45, 2297 CrossRef CAS.
-
(a) L. Chen, X. -J. Huang, Y. -Q. Li, M. -Y. Zhou and W. -J. Zheng, Monatsh. Chem., 2009, 140, 45 CrossRef CAS;
(b) M. P. Surpur, S. Kshirsagar and S. D. Samant, Tetrahedron Lett., 2009, 50, 719 CrossRef CAS;
(c) M. N. Elinson, A. I. Ilovaisky, V. M. Merkulova, P. A. Belyakov, A. O. Chizhov and G. I. Nikishin, Tetrahedron, 2010, 66, 4043 CrossRef CAS.
- B. V. S. Reddy, M. R. Reddy, G. Narasimhulu and J. S. Yadav, Tetrahedron Lett., 2010, 51, 5677 CrossRef CAS.
- J. M. Khurana and S. Kumar, Tetrahedron Lett., 2009, 50, 4125 CrossRef CAS.
- S. Chowdhury, G. C. Nandi, S. Samai and M. S. Singh, Org. Lett., 2011, 13, 3762 CAS.
- J. V. Vadagama, Y. Wu, D. Shen, S. Hisa and J. Block, Anticancer Res., 2000, 20, 1391 Search PubMed.
- T. Tanaka, B. S. Reddy and K. El-Bayoumy, Jap. J. Cancer Res., 1985, 76, 462 CAS.
-
(a) J. B. Sainani, A. C. Shah and V. P. Arya, Indian J. Chem., 1994, 33B, 526 CAS;
(b) V. K. Ahluwalia, B. Goyal and U. Das, J. Chem. Res. (S), 1997, 266 RSC;
(c) S. Margarita, O. Estael, V. Yamila, P. Beatriz, M. Lourdes, M. Nazario, Q. Margarita, S. Carlos, L. S. Jose, N. Hector, B. Norbert and M. P. Oswald, Tetrahedron, 1999, 55, 875 CrossRef;
(d) V. K. Ahluwalia, B. Goyal and U. Das, J. Chem. Res. Miniprint, 1997, 7, 1501 Search PubMed.
-
(a) V. K. Ahluwalia and B. Goyal, Indian J. Chem., 1996, 35B, 1021 CAS;
(b) S. Margarita, V. Yamila, M. Estael, M. Nazario, M. Roberto, Q. Margaria, S. Carlos, S. Jose, L. N. Hector, B. Norbert, M. Oswald and D. Camiel, J. Heterocycl. Chem., 2000, 37, 735 CrossRef.
- S. Kumar, P. Sharma, K. K. Kapoor and M. S. Hundal, Tetrahedron, 2008, 64, 536 CrossRef CAS.
- S. B. Sapkal, K. F. Shelke, B. B. Shingate and M. S. Shingare, Tetrahedron Lett., 2009, 50, 1754 CrossRef CAS.
- A. Khojastehnezhad, F. Moeinpour and A. Davoodnia, Chin. Chem. Lett., 2011, 22, 807 CrossRef CAS.
- M. Maheswara, V. Siddaiah, G. L. V. Damu and C. V. Rao, Arkivoc, 2006, ii, 201 Search PubMed.
- X. -S. Wang, M. -M. Zhang, H. Jiang, C. -S. Yao and S. -J. Tu, Synth. Commun., 2008, 38, 1355 CrossRef CAS.
- J. Quiroga, J. Portilla, H. Serrano, R. Abonía, B. Insuasty, M. Nogueras and J. Cobo, Tetrahedron Lett., 2007, 48, 1987 CrossRef CAS.
- T. Li, X. Feng, C. Yao, C. Yu, B. Jiang and S. Tu, Bioorg. Med. Chem. Lett., 2011, 21, 453 CrossRef CAS.
- P. Ramesh, N. S. Reddy and Y. Venkateswarlu, J. Nat. Prod., 1999, 62, 780 CrossRef CAS.
-
(a) C. Marchand, S. Antony, K. W. Kohn, M. Cushman, A. Ioanoviciu, B. L. Staker, A. B. Burgin, L. Stewart and Y. Pommier, Mol. Cancer Ther., 2006, 5, 287 CrossRef CAS;
(b) G. R. Pettit, V. Gaddamidi, D. L. Herald, S. B. Singh, G. M. Cragg, J. M. Schmidt, F. E. Boettner, M. Williams and Y. Sagawa, J. Nat. Prod., 1986, 49, 995 CrossRef CAS.
-
(a) J. E. van Muijlwijk-Koezen, H. Timmerman, R. Link, H. van der Goot and A. P. Ijzerman, J. Med. Chem., 1998, 41, 3987 CrossRef CAS;
(b) Q. Zeng, Y. Kwok, S. M. Kerwin, G. Mangold and L. H. Hurley, J. Med. Chem., 1998, 41, 4273 CrossRef CAS.
- L. Rong, L. Gao, H. Han, H. Jiang, Y. Dai and S. Tu, Synth. Commun., 2010, 40, 289 CrossRef CAS.
- Y. -B. Shen and G. -W. Wang, Arkivoc, 2008, xvi, 1 Search PubMed.
- R. W. Lambert, J. A. Martin, J. H. Merrett, K. E. B. Parkes and G. J. Thomas, PCT Int. Appl. WO 9, 1997, 706, 178 Search PubMed.
- J. P. Poupelin, G. Saint-Ruf, O. Foussard-Blanpin, G. Narcisse, G. Uchida-Ernouf and R. Lacroix, Eur. J. Med. Chem., 1978, 13, 67 CAS.
-
T. Hideo and J. Teruomi, Jpn. Patent 1981, 56, 005, 480 Search PubMed.
-
(a) N. P. Buu-Hoi, G. Saint-Ruf, A. De and H. T. Hieu, Bull. Chim. Ther., 1972, 7, 83 CAS;
(b) G. Saint-Ruf, H. T. Hieu and J. P. Poupelin, Naturwissenschaften, 1975, 62, 584 CrossRef CAS.
-
(a) R. M. Ion, Prog. Catal., 1997, 2, 55 Search PubMed;
(b) R. M. Ion, A. Planner, K. Wiktorowicz and D. Frackowiak, Acta Biochim. Pol., 1998, 45, 833 CAS.
-
(a) A. Banerjee and A. K. Mukherjee, Stain Technol., 1981, 56, 83 CAS;
(b) S. M. Menchen, S. C. Benson, J. Y. L. Lam, W. Zhen, D. Sun, B. B. Rosenblum, S. H. Khan and M. Taing, U. S. Patent 6, 2003, 583, 168 Search PubMed.
-
(a) O. Sirkencioglu, N. Talinli and A. Akar, J. Chem. Res., 1995, 502 CAS;
(b) M. Ahmad, T. A. King, D. -K. Ko, B. H. Cha and J. Lee, J. Phys. D: Appl. Phys., 2002, 35, 1473 CrossRef CAS.
- C. G. Knight and T. Stephens, Biochem. J., 1989, 258, 683 CAS.
- G. C. Nandi, S. Samai, R. Kumar and M. S. Singh, Tetrahedron, 2009, 65, 7129 CrossRef CAS.
- H. -J. Wang, X. -Q. Ren, Y. -Y. Zhang and Z. -H. Zhang, J. Braz. Chem. Soc. Short report, 2009, 1 Search PubMed.
- N. Foroughifar, A. Mobinikhaledi, H. Moghanian, R. Mozafari and H. R. M. Esfahani, Synth. Commun., 2011, 41, 2663 CrossRef CAS.
- R. -Z. Wang, L. -F. Zhang and Z. -S. Cui, Synth. Commun., 2009, 39, 2101 CrossRef CAS.
- J. Li, J. Li, J. Fang and W. Su, Synth. Commun., 2010, 40, 1029 CrossRef CAS.
- H. A. Oskooie, M. M. Heravi, N. Karimi and G. Kohansal, Synth. Commun., 2011, 41, 2763 CrossRef CAS.
-
(a) H. R. Shaterian and M. Ghashang, J. Braz. Chem. Soc., 2008, 19, 1053 CrossRef CAS;
(b) H. R. Shaterian, M. Ghashang and N. Mir, Arkivoc, 2007, xv, 1 Search PubMed.
- R. Kumar, G. C. Nandi, R. K. Verma and M. S. Singh, Tetrahedron Lett., 2010, 51, 442 CrossRef CAS.
-
(a)
K. Undheim and T. Benneche, Pyrimidines and their Benzo Derivatives, In Comprehensive Heterocyclic Chemistry II, A. R. Katritzky, C. W. Rees and E. V. F. Scriven, ed.; Pergamon: Oxford, U. K., 1996, vol. 6, p 93 Search PubMed;
(b)
G. W. Rewcastle, Pyrimidines and their Benzo Derivatives, In Comprehensive Heterocyclic Chemistry III, A. R. Katritzky, C. A. Ramsden, E. F. V. Scriven and R. J. K. Taylor, ed.; Pergamon: Oxford, U. K., 2008, vol. 8, p 117 Search PubMed;
(c)
D. J. Brown, R. F. Evans amd W. B. Cowden, The Pyrimidines, E. C. Taylor and A. Weissberger, ed.; John Wiley: New York, 1994, p 52 Search PubMed;
(d) M. Johar, T. Manning, D. Y. Kunimoto and R. Kumar, Bioorg. Med. Chem., 2005, 13, 6663 CrossRef CAS.
- N. Azas, P. Rathelot, S. Djekou, F. Delmas, A. Gellis, C. Di Giorgio, P. Vanelle and P. Timon-David, Farmaco, 2003, 58, 1263 CrossRef CAS.
- A. Agarwal, K. Srivastava, S. K. Puri and P. M. S. Chauhan, Bioorg. Med. Chem., 2005, 13, 4645 CrossRef CAS.
-
(a) R. M. Dodson and J. K. Seyler, J. Org. Chem., 1951, 16, 461 CrossRef CAS;
(b) A. L. Marzinzik and E. R. Felder, J. Org. Chem., 1998, 63, 723 CrossRef CAS.
- A. R. Forrester, M. Gill and R. H. Thomson, J. Chem. Soc., Perkin Trans. 1, 1979, 616 RSC.
- K. C. Gupta and P. Manglum, Curr. Sci., 1989, 58, 1196 CAS.
- A. G. Martinez, A. H. Fernandez, R. M. Alvarez, M. C. S. Losada, D. M. Vilchez, L. R. Subramanian and M. Hanack, Synthesis, 1990, 881 CrossRef.
- M. Seki, H. Kubota, K. Matsumoto, A. Kinumaki, T. Date and K. Okamura, J. Org. Chem., 1993, 58, 6354 CrossRef CAS.
- T. J. J. Muller, R. Braun and M. Ansorge, Org. Lett., 2000, 2, 1967 CrossRef CAS.
- J. M. Schomaker and T. J. Delia, J. Org. Chem., 2001, 66, 7125 CrossRef CAS.
- H. Kakiya, K. Yagi, H. Shinokubo and K. Oshima, J. Am. Chem. Soc., 2002, 124, 9032 CrossRef CAS.
- M. C. Bagley, D. D. Hughes and P. H. Taylor, Synlett, 2003, 259 CrossRef CAS.
- K. Itami, D. Yamazaki and J. Yoshida, J. Am. Chem. Soc., 2004, 126, 15396 CrossRef CAS.
- Q. Zhuang, H. X. Han, S. Wang, S. Tu and L. Rong, Synth. Commun., 2009, 39, 516 CrossRef CAS.
- D. Prajapati, M. Gohain and A. J. Thakur, Bioorg. Med. Chem. Lett., 2006, 16, 3537 CrossRef CAS.
- L. Rong, H. Han, L. Gao, Y. Dai, M. Cao and S. Tu, Synth. Commun., 2010, 40, 504 CrossRef CAS.
-
(a) C. O. Kappe, Tetrahedron, 1993, 49, 6937 CrossRef CAS;
(b) C. O. Kappe, Acc. Chem. Res., 2000, 33, 879 CrossRef CAS.
- P. Biginelli, Gazz. Chim. Ital., 1893, 23, 360 Search PubMed.
-
(a) E. H. Hu, D. R. Sidler and U. -H. Dolling, J. Org. Chem., 1998, 63, 3454 CrossRef CAS;
(b) J. Lu and H. Ma, Synlett, 2000, 63 CAS;
(c) B. C. Ranu, A. Hajra and U. Jana, J. Org. Chem., 2000, 65, 6270 CrossRef CAS;
(d) K. Ramalinga, P. Vijayalakshmi and T. N. B. Kaimal, Synlett, 2001, 863 CrossRef CAS;
(e) J. Lu, Y. Bai, Z. Wang, B. Yang and H. Ma, Tetrahedron Lett., 2000, 41, 9075 CrossRef CAS;
(f) J. S. Yadav, B. V. S. Reddy, R. Srinivas, C. Venugopal and T. Ramalingam, Synthesis, 2001, 1341 CrossRef CAS.
-
(a) K. A. Kumar, M. Kasthuraiah, C. S. Reddy and C. D. Reddy, Tetrahedron Lett., 2001, 42, 7873 CrossRef;
(b) J. S. Yadav, B. V. S. Reddy, K. B. Reddy, K. S. Raj and A. R. Prasad, J. Chem. Soc., Perkin Trans. 1, 2001, 1939 RSC;
(c) F. Bigi, S. Carloni, B. Frullanti, R. Maggi and G. Sartori, Tetrahedron Lett., 1999, 40, 3465 CrossRef CAS;
(d) J. Peng and Y. Deng, Tetrahedron Lett., 2001, 42, 5917 CrossRef CAS;
(e) M. A. Bigdeli, S. Jafari, G. H. Mahdavinia and H. Hazarkhani, Catal. Commun., 2007, 8, 1641 CrossRef CAS.
- A. Dondoni and A. Massi, Tetrahedron Lett., 2001, 42, 7975 CrossRef CAS.
- S. L. Jain, S. Singhal and B. Sain, Green Chem., 2007, 9, 740 RSC.
- W. Chen, S. Qin and J. Jin, Catal. Commun., 2007, 8, 123 CrossRef CAS.
- A. Debache, M. Amimour, A. Belfaitah, S. Rhouati and B. Carboni, Tetrahedron Lett., 2008, 49, 6119 CrossRef CAS.
- B. C. Ranu, A. Hajra and S. S. Dey, Org. Process Res. Dev., 2002, 6, 817 CrossRef CAS.
- D. S. Bose, M. Sudharshan and S. W. Chavhan, Arkivoc, 2005, iii, 228 Search PubMed.
- A. Hasaninejad, A. Zare, F. Jafari and A. R. Moosavi-Zare, E -J. Chem., 2009, 6, 459 CAS.
- D. S. Bose, L. Fatima and H. B. Mereyala, J. Org. Chem., 2003, 68, 587 CrossRef CAS.
- H. N. Karade, M. Sathe and M. P. Kaushik, Molecules, 2007, 12, 1341 CrossRef CAS.
- S. Chancharunee, P. Pinhom, M. Pohmakotr and P. Perlmutter, Synth. Commun., 2009, 39, 880 CrossRef CAS.
- A. Shaabani, A. Bazgir and F. Teimouri, Tetrahedron Lett., 2003, 44, 857 CrossRef CAS.
- A. Kuraitheerthakumaran, S. Pazhamalai and M. Gopalakrishnan, Arabian J. Chem., 2011 DOI:10.1016/j.arabjc.2011.06.005.
- N. Ahmed and J. E. van Lier, Tetrahedron Lett., 2007, 48, 5407 CrossRef CAS.
- L. Yu, D. Chen, J. Li and P. G. Wang, J. Org. Chem., 1997, 62, 3575 CrossRef CAS.
- Z. Jiang and R. Chen, Synth. Commun., 2005, 35, 503 CrossRef CAS.
- W. Pei and Q. Wang, Synth. Commun., 2010, 40, 1209 CrossRef CAS.
- H. Khabazzadeh, K. Saidi and H. Sheibani, Arkivoc, 2008, xv, 34 Search PubMed.
- V. Singh, V. Sapehiyia, V. Srivastava and S. Kaur, Catal. Commun., 2006, 7, 571 CrossRef CAS.
- B. Liang, X. Wang, J. -X. Wang and Z. Du, Tetrahedron, 2007, 63, 1981 CrossRef CAS.
- M. G. Kulkarni, S. W. Chavhan, M. P. Shinde, D. D. Gaikwad, A. S. Borhade, A. P. Dhondge, Y. B. Shaikh, V. B. Ningdale, M. P. Desai and D. R. Birhade, Beilstein J. Org. Chem., 2009, 5, 4 CrossRef.
- R. Chen and C. Qian, Chin. J. Chem., 2002, 20, 407 CAS.
- G. Molander, Chem. Rev., 1992, 92, 29 CrossRef CAS.
-
(a) R. Chen and C. Qian, Synth. Commun., 2002, 32, 2543 CrossRef CAS;
(b) Y. Ma, C. Qian, L. Wang and M. Yang, J. Org. Chem., 2000, 65, 3864 CrossRef CAS;
(c) Y. Ma, C. Qian, M. Xie and J. Sun, J. Org. Chem., 1999, 64, 6462 CrossRef CAS.
- R. Kumar, K. Raghuvanshi, R. K. Verma and M. S. Singh, Tetrahedron Lett., 2010, 51, 5933 CrossRef CAS.
- G. C. Nandi, S. Samai and M. S. Singh, Synlett, 2010, 1133 CAS.
- A. R. Hajipour, L. Khazdooz and A. Zarei, Synth. Commun., 2011, 41, 2200 CrossRef CAS.
- A. R. Hajipour, Y. Ghayeb, N. Sheikhan and A. E. Ruoho, Synth. Commun., 2011, 41, 2226 CrossRef CAS.
- A. S. Nagarajan and B. S. R. Reddy, Synlett, 2010, 2311 CAS.
- S. Mashkouri and M. R. Naimi-Jamal, Molecules, 2009, 14, 474 CrossRef CAS.
- M. B. Madhusudana Reddy and M. A. Pasha, Synth. Commun., 2011, 41, 1875 CrossRef CAS.
- M. Zhang and H. F. Jiang, Eur. J. Org. Chem., 2008, 3519 CrossRef CAS.
- M. M. Heravi, F. Derikvand, L. Ranjbar and F. F. Bamoharram, Synth. Commun., 2010, 40, 1256 CrossRef CAS.
-
(a) D. G. Wenzel, J. Am. Pharm. Assoc., 1955, 44, 550 CAS;
(b) M. Hori, R. Iemura, H. Hara, A. Ozaki, T. Sukamoto and H. Ohtaka, Chem. Pharm. Bull., 1990, 38, 681 CrossRef CAS.
-
(a) S. Hayao, H. J. Havera, W. G. Stryeker, T. J. Leipzig, R. A. Kulp and H. E. Hartzler, J. Med. Chem., 1965, 8, 807 CrossRef CAS;
(b) Y. Nishikawa, T. Shindo, K. Ishii, H. Nakamura, T. Kona and H. Uno, J. Med. Chem., 1989, 32, 583 CrossRef CAS;
(c) H. J. Havera and H. J. Vidrio, J. Med. Chem., 1979, 22, 1548 CrossRef CAS.
- Q. Chao, L. Deng, H. Shih, L. M. Leoni, D. Genini, D. A. Carson and H. B. Cottam, J. Med. Chem., 1999, 42, 3860 CrossRef CAS.
-
(a) A. Witt and J. Bergman, Curr. Org. Chem., 2003, 7, 659 CrossRef CAS;
(b) J. P. Michael, Nat. Prod. Rep., 2005, 22, 627 RSC.
- S. Niementowski, J. Prakt. Chem., 1895, 51, 564 CrossRef CAS.
- A. Bischler, Ber. Dtsch. Chem. Ges., 1891, 24, 506 CrossRef.
-
A. Riedel, German Patent 174941, 1905 Search PubMed.
- P. Rani, Archana, V. K. Srivastava and A. Kumar, Indian J. Chem., 2002, 41B, 2642 Search PubMed.
- K. Ozaki, Y. Yamada, T. Oine, T. Ishizuka and Y. Iwasawa, J. Med. Chem., 1985, 28, 568 CrossRef CAS.
- K. Rad-Moghadam and L. Samavi, J. Heterocycl. Chem., 2006, 43, 913 CrossRef CAS.
- J. B. Jiang, D. P. Hesson, B. A. Dusak, D. L. Dexter, G. J. Kang and E. Hamel, J. Med. Chem., 1990, 33, 1721 CrossRef CAS.
-
(a) J. F. Wolfe, T. L. Rathman, M. C. Sleevi, J. A. Campbell and T. D. Greenwood, J. Med. Chem., 1990, 33, 161 CrossRef CAS;
(b) Y. Takaya, H. Tasaka, T. Chiba, K. Uwai, M. Tanitsu, H. Kim, Y. Wataya, M. Miura, M. Takeshita and Y. Oshima, J. Med. Chem., 1999, 42, 3163 CrossRef CAS.
-
(a) A. J. Bridges, H. Zhou, D. R. Cody, G. W. Rewcastle, A. McMichael, H. D. H. Showalter, D. W. Fry, A. J. Kraker and W. A. Deny, J. Med. Chem., 1996, 39, 267 CrossRef CAS;
(b) Y. Kurogi, Y. Inoue, K. Tsutsumi, S. Nakamura, K. Nagao, H. Yoshitsugu and Y. Tsuda, J. Med. Chem., 1996, 39, 1433 CrossRef CAS.
- W. L. F. Armarego, Adv. Heterocycl. Chem., 1979, 24, 1 CrossRef CAS.
- V. Segarra, M. I. Crespo, F. Pujol, J. Beleta, T. Domenech, M. Miralpeix, J. M. Palacios, A. Castro and A. Martinez, Bioorg. Med. Chem. Lett., 1998, 8, 505 CrossRef CAS.
- Y. Yu, J. M. Ostresh and R. A. Houghten, J. Org. Chem., 2002, 67, 5831 CrossRef CAS.
- A. Kamal, K. V. Ramana, H. B. Ankati and A. V. Ramana, Tetrahedron Lett., 2002, 43, 6861 CrossRef CAS.
- V. B. Rao and C. V. Ratnam, Indian J. Chem., 1979, 18B, 409 CAS.
- M. Akazome, J. Yamamoto, T. Kondo and Y. Watanabe, J. Organomet. Chem., 1995, 494, 229 CrossRef CAS.
- K. Rad-Moghadam, M. Mamghani and L. Samavi, Synth. Commun., 2006, 36, 2245 CrossRef.
- S. Rostamizadeh, A. M. Amani, A. Reza, H. R. Ghaini and N. Shadjou, Synth. Commun., 2008, 38, 3567 CrossRef CAS.
- M. Kidwai, S. Saxena, M. K. R. Khan and S. S. Thukral, Eur. J. Med. Chem., 2005, 40, 816 CrossRef CAS.
- A. Dandia, R. Singh and P. Sarawgi, J. Fluorine Chem., 2004, 125, 1835 CrossRef CAS.
- I. R. Siddiqui, S. A. Siddique, V. Srivastava, P. K. Singh and J. Singh, Arkivoc, 2008, xii, 277 Search PubMed.
- J. Azizian, M. R. Mohammadizadeh, S. Zomorodbakhsh, A. A. Mohammadi and A. R. Karimi, Arkivoc, 2007, xv, 24 Search PubMed.
- G. Shukla, R. K. Verma, G. K. Verma and M. S. Singh, Tetrahedron Lett., 2011, 52, 7195 CrossRef CAS.
-
(a)
K. K. Murthi, R. Koestler, C. Smith, T. Brandstetter and A. F. Kluge, 2006, EP 1674457 20041223 Search PubMed;
(b)
Y. Tanaka, S. Takeda, H. Higashi, M. Matsuura, F. Kobayashi, M. Hamada and M. Tanaka, 2006, WO 2006028284 Search PubMed;
(c)
V. P. Palle, A. K. Verma, M. Salman, R. K. Singh, Y. B. Waman, G. Sharma and A. Ray, 2006, WO 2006016237 Search PubMed;
(d)
M. Cik, G. S. Diels and G. R. Van Lommen, 2006, WO 2006008259 Search PubMed;
(e)
T. B. Durham, P. J. Hahn, T. J. Kohn, J. R. McCarthy, H. B. Broughton, R. D. Dally, M. R. Gonzalez-Garcia, K. J. Henry, T. A. Shepherd, J. A. Erickson and A. B. B. Melendo, 2006, WO 2006034093 Search PubMed.
-
(a) R. Witjmans, M. K. S. Vink, H. E. Schoemaker, F. L. van Delft, R. H. Blaauw and F. P. J. T. Rutjes, Synthesis, 2004, 641 Search PubMed;
(b) A. Amantana and P. L. Iversen, Curr. Opin. Pharmacol., 2005, 5, 550 CrossRef CAS.
- O. Mitsunobu and Y. Yamada, Bull. Chem. Soc. Jpn., 1967, 40, 2380 CrossRef CAS.
- T. Regnier, F. Berree, O. Lavastre and B. Carboni, Green Chem., 2007, 9, 125 RSC.
- A. Nizam and M. A. Pasha, Synth. Commun., 2010, 40, 2864 CrossRef CAS.
- P. Perjesi, A. Foldesi, G. Batta and J. Tamas, Chem. Ber., 1989, 122, 651 CrossRef CAS.
- T. Murai, H. Niwa, T. Kimura and F. Shibahara, Chem. Lett., 2004, 33, 508 CrossRef CAS.
- T. Noshio, Y. Konno, M. Ori and M. Sakamoto, Eur. J. Org. Chem., 2001, 3533 Search PubMed.
- M. Koketsu, K. Tanaka, Y. Takenaka, C. D. Kwong and H. Ishihara, Eur. J. Pharm. Sci., 2002, 15, 307 CrossRef CAS.
- L. D. S. Yadav, S. Yadav and V. K. Rai, Tetrahedron, 2005, 61, 10013 CrossRef CAS.
- J. -P. Wan, Y. -H. Pan, H. Mao, Y. -H. Chen and Y. -J. Pan, Synth. Commun., 2010, 40, 709 CrossRef CAS.
- N. Suryakiran, K. Rajesh, P. Prabhakar, J. J. P. Selvam and Y. Venkateswarlu, Catal. Commun., 2007, 8, 1635 CrossRef CAS.
- T. Tanaka, T. Muto, H. Maruoka, S. Imajo, H. Fukami, Y. Tomimori, Y. Fukuda and T. Nakatsuka, Bioorg. Med. Chem. Lett., 2007, 17, 3431 CrossRef CAS.
- E. Sotoca, T. Constantieux and J. Rodriguez, Synlett, 2008, 1313 CAS.
|
This journal is © The Royal Society of Chemistry 2012 |